Notes
Article history
The research reported in this issue of the journal was funded by the EME programme as project number 08/246/09. The contractual start date was in November 2014. The final report began editorial review in July 2015 and was accepted for publication in April 2016. The authors have been wholly responsible for all data collection, analysis and interpretation, and for writing up their work. The EME editors and production house have tried to ensure the accuracy of the authors’ report and would like to thank the reviewers for their constructive comments on the final report document. However, they do not accept liability for damages or losses arising from material published in this report.
Declared competing interests of authors
Jane E Norman has received research grants from government and charitable bodies for research into pregnancy problems, including a grant from the charity Tommy’s to address the adverse consequences of maternal obesity in pregnancy. Jane E Norman’s institution receives funding from GlaxoSmithKline for Jane E Norman’s participation in a Data Monitoring Committee for a study on preterm birth. Jane E Norman and Gordon D Murray have both served on the Efficacy and Mechanism Evaluation Board during the lifetime of this project. Jane E Norman is a member of the Health Technology Assessment Maternal Newborn and Child Health prioritisation panel.
Permissions
Copyright statement
© Queen’s Printer and Controller of HMSO 2016. This work was produced by Chiswick et al. under the terms of a commissioning contract issued by the Secretary of State for Health. This issue may be freely reproduced for the purposes of private research and study and extracts (or indeed, the full report) may be included in professional journals provided that suitable acknowledgement is made and the reproduction is not associated with any form of advertising. Applications for commercial reproduction should be addressed to: NIHR Journals Library, National Institute for Health Research, Evaluation, Trials and Studies Coordinating Centre, Alpha House, University of Southampton Science Park, Southampton SO16 7NS, UK.
Chapter 1 Introduction and literature review
Rates of obesity, as defined by a body mass index (BMI) of > 30 kg/m2, have risen alarmingly in recent decades. Around 20% of women booking for antenatal care in the UK are obese. The adverse effects of maternal obesity on pregnancy complications for both the mother and the fetus are well established1–4 and there is mounting evidence of a detrimental effect on the longer-term health of offspring. 5–7 Increasingly, data suggest that maternal obesity may programme offspring later-life obesity, with high birthweight being a marker for increased risk. Our own recent work also suggests that offspring of obese pregnant women are at increased risk of premature death in adulthood. 8
The mechanism by which maternal obesity causes excessive neonatal birthweight is not clearly understood but considerable evidence implicates insulin resistance and/or hyperglycaemia. Obese pregnant women are more insulin resistant and hyperglycaemic than their lean counterparts. 9 This enhances nutrient availability for the fetus with consequent excessive growth. There is a strong correlation between the degree of insulin resistance in late pregnancy and both birthweight and fat-free mass (FFM) at birth. 10 The Hyperglycaemia and Adverse Pregnancy Outcomes study11 confirms that there is a linear relationship between hyperglycaemia and birthweight, even at glucose levels considered normal during pregnancy. Finally, treating hyperglycaemia in women with confirmed gestational diabetes mellitus (GDM) reduces the incidence of large-for-gestational-age (LGA) babies and other perinatal complications. 12
The aim of this trial was to see whether or not giving the insulin-sensitising agent metformin to obese pregnant women from 12–16 weeks’ gestation until delivery might reduce the future life risk of obesity and metabolic syndrome in the baby. We used birthweight centile as a surrogate marker for future life events as its predictive value has been shown in large epidemiological studies. 13
Interventions in pregnancy to reduce excess birthweight in offspring of obese pregnant women
To date, all of the interventions that have been trialled in overweight or obese pregnant women to reduce the risk of excess birthweight in the offspring have involved modifications to diet or lifestyle, or a combination of both.
There have been several systematic reviews of studies evaluating such interventions in pregnancy but only two have been limited to overweight and obese women. 14,15 Three further randomised trials have been published since these reviews, the LIMIT trial (limiting weight gain in overweight and obese women during pregnancy to improve health outcomes),16 the LiP (Lifestyle in Pregnancy) study17 and UPBEAT (UK Pregnancies Better Eating and Activity Trial). 18
The review by Dodd et al. 14 examined nine randomised controlled trials (RCTs) including 743 women. Seven trials compared a dietary intervention with standard antenatal care. Two of the trials evaluated the effect of an exercise intervention but outcomes did not include effect on infant birthweight in these studies. Only three trials reported outcome data for the primary outcome of LGA infants, with no significant difference between those who received the intervention and those who did not [366 women; risk ratio 2.20, 95% confidence interval (CI) 0.84 to 4.86]. Four trials examined effect on gestational weight gain and again there was no statistically significant difference between groups for this outcome (416 women; weighted mean difference –3.10 kg, 95% CI –8.32 to 2.13 kg). The overall conclusion of the review was that the evidence of benefit for this type of intervention in overweight or obese women is not clear. However, the authors noted that the quality of all of the included studies was poor to fair and that further high-quality, suitably powered randomised trials are urgently needed.
The review by Oteng-Ntim et al. 15 included 13 randomised trials and six non-randomised trials. Again, the overall quality of the trials was deemed to be suboptimal, with five of the RCTs judged to be of medium quality and the rest of low quality. Six of the studies included LGA as an outcome, but there was no evidence that the interventions were associated with a lower prevalence of this outcome [1008 women; odds ratio (OR) 0.91, 95% CI 0.62 to 1.32]. Seven studies examined the effect on birthweight and, although there was a trend towards an effect of the intervention, this did not reach statistical significance (1133 women; mean difference –56.64 g, 95% CI –120.15 to 6.88 g). The authors reached a similar conclusion that further meta-analyses will be unlikely to refine the quality of the evidence and that large-scale suitably powered trials are required.
One such trial has since been published – the LIMIT trial. 16 This was a multicentre RCT of a diet, exercise and behavioural intervention compared with standard care for overweight or obese women (BMI of > 25 kg/m2, median BMI of cohort 31.1 kg/m2). The primary outcome was LGA infants (> 90th centile for gestation). The trial recruited to target a total of 2212 women and was adequately powered to detect a 30% reduction in LGA infants. There was no significant difference in the risk of infants born LGA in the lifestyle advice group compared with the standard care group (19% vs. 21%; adjusted risk ratio 0.90, 95% CI 0.77 to 1.07; p = 0.24).
The LiP study17 was a smaller trial of 360 women, all of whom were obese (BMI of 30–45 kg/m2, median BMI of 33 kg/m2). The women were randomised to receive a lifestyle intervention that included dietetic advice, gym membership, physical training and personal coaching. The primary end point was a combination of five obstetric and neonatal outcomes: emergency caesarean section, pre-eclampsia, GDM, LGA and admission to the neonatal unit, with a score of 1 point for each outcome. There was no significant difference in combined scores between the groups (0.65 for the intervention group vs. 0.67 for the control group; p = 0.39). Birthweight was, in fact, significantly higher in the intervention group than in the control group (median 3742 g vs. 3596 g; p = 0.039). Gestational weight gain was significantly lower in the intervention group (7.0 kg vs. 8.6 kg; p = 0.01). However, as with many of the previous studies, the authors note that ultimately the study was underpowered, with power calculations being based on the expectation of a larger difference in gestational weight gain between groups than was actually found.
The UPBEAT18 study similarly found no effect of a lifestyle intervention on the incidence of GDM or LGA infants.
At the time of initiation of the EMPOWaR study (Efficacy of Metformin in Pregnant Obese Women, a Randomised controlled trial), there were no RCTs of pharmacotherapy as an intervention for obese pregnant women. Given the evidence of a lack of effect from lifestyle interventions, pharmacotherapy is an important next step. Other than the work presented in this report, we are aware of two other ongoing studies of the effect of metformin as a pharmacological intervention in obese pregnant women [MOP (Metformin in Obese Pregnancy) – NCT01273584; and GRoW (metformin and dietary advice to improve insulin sensitivity and promote Gestational Restriction of Weight in pregnant women who are obese) – ACTRN12612001277831], one of which has now been published. 19
Metformin in pregnancy
The use of metformin is endorsed by the National Institute for Health and Care Excellence for the treatment of GDM. 20 There are no placebo-controlled RCTs of the use of metformin in pregnancy, but several trials have compared metformin with alternative agents for the treatment of GDM. There have been several recent systematic reviews of these trials, including those by Balsells et al. 21 and Zhao et al. ,22 and a ‘literature review’ by Singh et al. 23 Additionally, two other randomised trials24,25 have been published since these meta-analyses were performed.
The meta-analysis by Balsells et al. 21 compared metformin with insulin and with glibenclamide for the treatment of GDM. Fourteen primary outcomes were considered. Compared with insulin, metformin reduced maternal weight gain (mean difference −1.14 kg, 95% CI −2.22 to −0.06 kg), reduced gestational age at delivery (mean difference −0.16 weeks, 95% CI −0.30 to −0.02 weeks) and increased the rate of preterm births (risk ratio 1.50, 95% CI 1.04 to 2.16). Compared with glibenclamide, metformin reduced maternal weight gain (mean difference −2.06 kg, 95% CI −3.98 to −0.14 kg), was associated with lower birthweight (mean difference −209 g, 95% CI −314 to −104 g), reduced the risk of macrosomia (risk ratio 0.33, 95% CI 0.13 to 0.81) and reduced the risk of LGA newborns (risk ratio 0.44, 95% CI 0.21 to 0.92). Zhao et al. 22 demonstrated that, compared with insulin, metformin reduced the risk of pregnancy-induced hypertension (risk ratio 0.54, 95% CI 0.31 to 0.91), but there were no differences in effects on neonatal hypoglycaemia, LGA infants, respiratory distress syndrome, phototherapy or perinatal death.
The literature review23 reported that the majority of studies found no difference in glycaemic control between metformin and insulin and suggested that, although there is a growing body of evidence to suggest a role for metformin in GDM management, much of this came from single-site small studies and that further studies are needed to inform guidelines.
In one of the RCTs not included in the systematic reviews described above,25 and which recruited 159 women, metformin was demonstrated to be superior to glibenclamide because it was associated with a reduction in risk of 16.1% (95% CI 2.5% to 29.7%; p = 0.02) in the primary outcome, a composite of macrosomia, hypoglycaemia need for phototherapy, respiratory distress, stillbirth or neonatal death and birth trauma, largely because of a higher incidence of hypoglycaemia in the glibenclamide group. 25 In the RCT by Beyuo et al. 24 (n = 104), which compared metformin with placebo, with the addition of insulin if required to maintain glycaemic control, postprandial glucose levels were significantly lower in the metformin group.
There are few randomised trials of metformin compared with placebo in pregnant women without GDM. Both published studies were of women with polycystic ovary syndrome (PCOS),26,27 with one being a pilot of the other. Although a significant difference in a composite of severe pregnancy and post-partum complications was seen in the smaller study comparing 850 mg of metformin twice daily with placebo (n = 40),26 there were no significant differences in the outcomes of pre-eclampsia, preterm delivery and GDM in the larger study comparing 2000 mg of metformin daily with placebo (n = 259),27 although women in the metformin group gained less weight.
Chapter 2 Trial design and methods
Study design
This study was a double-blind, randomised, placebo-controlled trial in a population of obese pregnant women to examine the effect of metformin on sex- and age-adjusted birthweight centile of the baby. There were embedded substudies to explore the mechanism of action of metformin. In addition, a qualitative study was carried out to explore reasons for non-participation or non-retention of participants in the trial. A description of the trial protocol and a summary of the clinical trial results have already been published. 28,29
Ethics approval and research governance
A summary of the protocol changes is provided in Table 1.
Protocol version | Date | Summary of changes |
---|---|---|
1 | 6 January 2010 | Initial protocol |
1 | 4 March 2010 | Additional information provided to Medicines and Healthcare products Regulatory Agency after initial non-acceptance |
2 | 20 September 2010 | Protocol modified to version 2 |
Expanded details about the substudies | ||
Patient information leaflet and consent forms amended to version 3 | ||
Addition of new site and principal investigator: Sheffield, Dr H Lashen | ||
Additional documents: treatment diary version 1, 16 June 2010; participant contact information sheet version 1, 13 September 2010; GP letter version 1, 20 September 2010 | ||
3 | 13 April 2011 | Principal investigator contact address and site changed for Professor S Quenby |
Additional site and principal investigator: Nottingham, Dr Bugg | ||
Patient information leaflet and consent forms amended to version 4; all references to obesity removed | ||
Table of assessments errors corrected | ||
1-hour sampling time point in glucose tolerance test removed | ||
Paragraph 6.1 additional text added: ‘where a letter inviting women to participate may be issued’ | ||
GP letter, advert for newspaper, advert for waiting rooms (poster text) and slip for patient notes (invitation letter) updated to version 2 | ||
3 | 19 July 2011 | Additional site and principal investigator: Bradford, Professor Tufnell |
4 | 30 September 2011 | Reference range for alanine aminotransferase changed |
Clarification of exclusion criteria for GDM | ||
Names of recruiting hospitals deleted from general protocol | ||
Reference to Matsuda index deleted; HOMA-IR to be used | ||
4 | 13 February 2012 | Patient advertising leaflet |
Addition of eight new sites | ||
5 | 1 September 2013 | Closure of site |
Revision of protocol for clarifications and addition of new substudy | ||
Revision of patient information leaflet and consent forms to version 5 and advertising leaflet to version 2 | ||
6 | 30 September 2013 | Paragraphs 6.4 amendment and additional documents created for qualitative interviews |
7 | 10 March 2014 | Paragraphs 6.4 and 9.11 updated to include payment for substudy participants and inclusion of lean women as control subjects for vascular function substudy |
8 | 24 September 2014 | Updated protocol to clarify primary and secondary outcomes to agree with statistical analysis plan. Specifically, maternal insulin resistance at 36 weeks’ gestation was originally a coprimary outcome, but was relegated to a secondary outcome when a substantial proportion of participants did not provide a blood sample at 36 weeks |
Removal of substudies to which no subjects were recruited |
Ethics approval was obtained from Scotland A Research Ethics Committee (reference number 10/MRE00/12). The study was conducted in accordance with the principles of Good Clinical Practice. 30
A Data Monitoring Committee oversaw the study. The trial was registered as ISRCTN51279843 (EMPOWaR).
Objectives
Primary objective
The primary objective was to determine the efficacy of metformin (up to 2500 mg daily) given to obese pregnant women from 12–16 weeks’ gestation until delivery in reducing gestational age-, parity- and sex-adjusted birthweight centile of the baby.
Secondary objectives
-
To determine the pattern of association between insulin resistance and adverse pregnancy outcomes including the incidence of pregnancy-induced hypertension, pre-eclampsia, caesarean section and post-partum haemorrhage, maternal weight gain during pregnancy and the incidence of the admission to the neonatal unit.
-
To determine the effect of metformin on maternal body composition.
-
To determine the effect of metformin on neonatal body composition.
-
To determine the effect of metformin on maternal inflammatory and metabolic variables (measured at 28 and 36 weeks’ gestation) and neonatal inflammatory variables (measured in cord blood at birth).
-
To confirm that metformin does not increase the rate of babies with a low birthweight centile.
-
To determine the efficacy (as opposed to the effectiveness) of metformin when analysis is restricted to those with pharmacological circulating levels of the drug.
Substudies
A series of nested substudies was also performed with the following objectives:
-
to determine the effect of metformin on maternal cortisol levels in obese pregnant women
-
to determine the effect of metformin on hepatic and peripheral insulin sensitivity at 36 weeks’ gestation in obese pregnant women
-
to determine the effect of metformin on endothelium-dependent flow-mediated dilatation (FMD) in obese pregnant women
-
to determine the effect of metformin on maternal subcutaneous and visceral adipose tissue deposition and hepatic and skeletal muscle ectopic fat deposition during pregnancy
-
to determine the effect of metformin on fetal liver volume and subcutaneous fat deposition
-
to determine the effect of metformin on myometrial contractility and myometrial glycogen storage in obese pregnant women
-
to determine the effect of metformin on placental glucocorticoid receptor and 11β-hydroxysteroid dehydrogenase (HSD) type 1 and 2 messenger ribonucleic acid (mRNA) levels.
Participants
The study sought to recruit obese pregnant women who met the following eligibility criteria.
Screening phase inclusion criteria
-
Caucasian obese (BMI of ≥ 30 kg/m2) pregnant women between 12+ 0 and 16+ 0 weeks’ (+days) gestation.
-
Aged ≥ 16 years.
-
Signed informed consent form.
Screening phase exclusion criteria
-
Non-Caucasian.
-
BMI of < 30 kg/m2.
-
Gestation > 16 weeks.
-
Pre-existing diabetes mellitus.
-
GDM in a previous pregnancy.
-
Systemic disease at the time of trial entry, with the disease either requiring regular medication or having required treatment with systemic steroids in the past 3 months.
-
Previous delivery of a baby < 3rd centile by weight.
-
Previous pregnancy complicated by pre-eclampsia prompting delivery before 32 weeks’ gestation.
-
Known sensitivity to metformin hydrochloride or any of the known excipients.
-
Acute condition at the time of trial entry with the potential to alter renal function, such as dehydration sufficient to require intravenous infusion, severe infection, shock and intravascular administration of contrast agents.
-
Acute or chronic diseases that may cause tissue hypoxia such as cardiac or respiratory failure, recent myocardial infarction, hepatic insufficiency, acute alcohol intoxication and alcoholism.
-
Lactation.
-
Multiple pregnancy.
Randomisation exclusion criteria following screening
-
GDM in index pregnancy [diagnosed with 75-g oral glucose tolerance test using World Health Organization (WHO) diagnostic criteria of fasting glucose ≥ 7.0 mmol/l, 2-hour glucose ≥ 7.8 mmol/l31]. Participants were also excluded if glucose tolerance testing was diagnostic of GDM based on the criteria used in the recruiting centre [e.g. International Association of the Diabetes and Pregnancy Study Groups (IADPSG) criteria32].
-
Liver or renal dysfunction at the time of trial entry tested prior to randomisation (urea > 6.6 mmol/l, creatinine > 85 mmol/l, sodium > 145 mmol/l, potassium > 5.0 mmol/l, bilirubin > 16 µmol/l, alanine transferase > 60 IU/l) or with abnormal lactate levels (according to local laboratory reference range).
Ineligible and non-recruited participants
No further information was collected on women who were ineligible because of abnormalities in glucose tolerance or liver or renal function, other than the number of such women for trial metrics.
Telephone or face-to-face interviews were carried out to explore the reasons for eligible women declining to participate (see Chapter 5).
Recruitment procedure
Potentially eligible subjects (i.e. women with a BMI of ≥ 30 kg/m2 who booked to have their antenatal care at any of the participating hospitals) were either approached directly by a member of the research team or given written information by their caregiver and their contact details passed to the research team. The recruitment period was 3 February 2011 to 16 January 2014.
Informed consent
Subjects were given at least 24 hours to consider participation. They were then asked to provide written informed consent.
Randomisation, concealment and blinding
Eligible participants were randomly assigned to active treatment with metformin or an identical-looking placebo. This was documented in patients’ paper case record and/or computer file to demonstrate their participation in the trial.
Participants were randomised via a web portal connected to a central randomisation facility based at the trial data centre, the Edinburgh Clinical Trials Unit, University of Edinburgh. Baseline eligibility criteria were required to be entered into the database before randomisation. Participants were randomised in a 1 : 1 ratio of metformin to placebo (block size of two to four). Randomisation was stratified by treatment centre and a BMI of 30–39 kg/m2 compared with a BMI of > 40 kg/m2.
Treatment group allocation
Randomising participants to active or placebo tablets achieved concealment of allocation. Placebo tablets appeared to be identical to active treatment so that participants were masked to treatment allocation. The outcomes were measured by clinicians and investigators masked to treatment allocation. Masking was not broken until after data entry was complete, the validity of the data was checked, all queries were resolved, the patient populations agreed and the database locked. Any clinically indicated unmasking was recorded prospectively.
Intervention
Metformin tablets (or matched placebo) (500 mg) were administered from as soon as practicable after the point of randomisation (and certainly between 12 and 16 weeks’ gestation) until delivery of the baby. The dose regimen was as follows: week 1, 500 mg once daily; week 2, 500 mg twice daily; week 3, 500 mg three times a day; week 4, 500 mg morning and lunchtime and 1000 mg in the evening; week 5, 1000 mg in the morning and evening and 500 mg at lunchtime. All doses were taken with food and dose escalation continued to either the maximum tolerable dose or 2500 mg, whichever was higher.
Dose changes
Local investigators or participants were allowed to alter the treatment regimen at their discretion as long as the maximum daily dose did not exceed 2500 mg. Changes to the treatment dose were recorded in the electronic case report form as soon as was practicable.
Other medications
Alcohol was prohibited because of the increased risk of lactic acidosis. Iodinated contrast agents may increase the risk of renal failure and, hence, if they were required treatment was discontinued for at least 48 hours from immediately prior to contrast administration until after renal function had been re-evaluated and found to be normal. Clinicians prescribing glucocorticoids, beta-2-adrenoreceptor agonists and angiotensin-converting enzyme inhibitors should have been aware that they might amplify or diminish the hypoglycaemic effect of metformin.
Data collection and management
To standardise data collection processes across trial sites, researchers were trained to use detailed standard operating procedures (SOPs) for each element of data collection. Data were entered into the trial database contemporaneously and researchers were also encouraged to keep paper records as reference source data. A number of cross-checks were programmed into the database to automatically raise data queries, for example blank fields and values outwith reference ranges. The data were also checked manually on completion of data collection but prior to unblinding for extreme outliers in an attempt to ensure that biologically implausible data were confirmed or corrected.
Study assessments
Study assessments occurred over nine visits (either face to face or by telephone) throughout pregnancy. These are detailed in the study protocol (see Appendix 1) and are summarised in Table 2.
Purpose | Visit number | ||||||||
---|---|---|---|---|---|---|---|---|---|
1 | 2 | 3 | 4 | 5 | 6 | 7 | 8 | 9 | |
Gestation | 10–16 weeks | 10–16 weeks | 12–16 weeks | 18–20 weeks | 28 weeks | 36 weeks | Term | Labour/delivery/neonatal | 3 months postnatally |
Assessment | Screening | Consent | Randomisation | Study visit (could be by telephone) | Study visit | Study visit | Study visit (could be by telephone) | Study visit | Study visit |
Review inclusion and exclusion criteria | ✗ | ||||||||
Patient information leaflet | ✗ | ||||||||
Consent form | ✗ | ||||||||
Demographics | ✗ | ||||||||
Medical history | ✗ | ||||||||
Height and weight | ✗ | ✗ | |||||||
Maternal anthropometry | ✗ | ✗ | ✗ | ||||||
Bloods for liver function/renal function/full lipid profile/C-reactive protein | ✗ | ✗ | |||||||
75-g oral glucose tolerance test (sampling at baseline and 2 hours) | ✗ | ✗ | ✗ | ||||||
Stored sample for inflammatory and metabolic indices | ✗ | ✗ | ✗ | ||||||
Randomisation | ✗ | ||||||||
Study drug dispensed | ✗ | ✗ | |||||||
Unused study drug/packaging returned | ✗ | ||||||||
Review serious adverse events | ✗ | ✗ | ✗ | ✗ | ✗ | ||||
Complete side effects questionnaire on eCRF | ✗ | ✗ | ✗ | ✗ | ✗ | ||||
Review and record pregnancy complications | ✗ | ✗ | ✗ | ✗ | ✗ | ||||
Saliva samples for cortisol measurements | ✗ | ✗ | ✗ | ||||||
BOD POD® measurementsa | ✗ (or visit 3) | ✗ (or visit 2) | ✗ | ✗ | |||||
Hyperinsulinaemic–euglycaemic clamp | ✗ | ||||||||
FMD | ✗ | ✗ | |||||||
Magnetic resonance scan | ✗ | ✗ | |||||||
Labour/delivery information including birthweight, mode of delivery, estimated blood loss | ✗ | ||||||||
Cord blood and placenta biopsy | ✗ | ||||||||
Myometrium biopsy (if delivered by caesarean section) | ✗ | ||||||||
Adipose tissue biopsy | ✗ | ||||||||
Baby’s weight and anthropometry | ✗ | ✗ | |||||||
PEA POD® measurementsa | ✗ | ✗ |
Maternal anthropometric measurements (waist, hip, upper arm and mid-thigh circumference and bicep, tricep and subscapular skinfold thickness) were recorded at baseline, at 36 weeks’ gestation and at 3 months post partum (see Appendix 2). Neonatal anthropometric measurements (head circumference, length and tricep and subscapular skinfold thickness) were recorded within 72 hours of birth and at 3 months of age (see Appendix 3). All staff making anthropometry measurements were trained by the central trial team. We initially held a study training event on maternal and baby anthropometry for research midwives from Liverpool, Edinburgh and Coventry, during which all staff in attendance, including the trial manager, were trained by the investigators with experience in this area (SF, AD and RR). Further central training events were held with additional training offered and completed during the site initiation visits. All staff trained by the central team were then authorised to train new staff locally. The procedures were also documented in working practice documents, a set of instructions detailing the correct procedures for each measurement required.
Glucose tolerance testing and fasting maternal blood samples were obtained at baseline and 28 and 36 weeks’ gestation to determine the effect of metformin on glucose and insulin resistance. Glucose, C-reactive protein (CRP), liver function tests, urea and electrolytes and lipid indices were all analysed in the recruiting NHS hospital laboratory. These results were available to the clinical team immediately. Other serum and plasma samples were stored for later analysis of inflammatory and metabolic indices. These were insulin, interleukin (IL) 6, leptin, the plasminogen activator inhibitor-1 (PAI-1) : PAI-2 ratio, cortisol and non-esterified fatty acids (NEFAs). Umbilical cord blood was taken at the time of delivery for measurement of glucose and CRP and stored for future measurement of insulin, NEFAs, IL-6, leptin and cortisol.
All blood samples were collected, stored and transferred in accordance with the SOPs (see Appendix 4). The 75-g oral glucose tolerance test was performed in accordance with the SOPs (see Appendix 4). Urea and electrolytes, liver function tests, glucose, CRP, high-density lipoprotein (HDL), low-density lipoprotein (LDL) and cholesterol were analysed by the recruiting NHS hospital laboratory.
Other analytical methods
Insulin
Insulin was measured using a standard sandwich enzyme-linked immunosorbent assay (ELISA) kit from Demeditec Diagnostics (Kiel, Germany). The limit of detection was 1.76 IU/l, with a mean intra-assay coefficient of variation (CV) of 2.2% and a mean inter-assay CV of 4.5%.
Interleukin 6
Interleukin 6 was measured using a high-sensitivity Quantikine® sandwich ELISA from R&D Systems (Abingdon, UK). The limit of detection was 0.039 pg/ml, with a mean intra-assay CV of 7.4% and a mean inter-assay CV of 7.8%.
Leptin
Leptin was measured using a standard sandwich ELISA kit from Alpco® (Salem, NH, USA). The limit of detection was 0.50 ng/ml, with a mean intra-assay CV of 4.6% and a mean inter-assay CV of 6.1%.
Plasminogen activator inhibitor 1
Plasminogen activator inhibitor 1 was measured using a sandwich ELISA kit from Cloud-Clone Corp. (Houston, TX, USA). The limit of detection was 0.063 ng/ml, with a mean intra-assay CV of < 10% and a mean inter-assay CV of < 12%.
Plasminogen activator inhibitor 2
Plasminogen activator inhibitor 2 was measured using a sandwich ELISA kit from Cloud-Clone Corp. The limit of detection was 0.61 ng/ml, with a mean intra-assay CV of < 10% and a mean inter-assay CV of < 12%.
Cortisol
Cortisol was measured using a standard ELISA kit from Demeditec Diagnostics. The limit of detection was 2.5 ng/ml, with a mean intra-assay CV of 5.6% and a mean inter-assay CV of 6.9%.
Non-esterified fatty acids
Non-esterified fatty acids were measured using an enzymatic colorimetric method assay kit from Wako Chemicals (Neuss, Germany). The assay range was 0.01–4.00 mEq/l. The mean intra-assay CV was not more than 1.5%.
Outcomes
Primary outcome
The primary outcome was z-score corresponding to the gestational age-, parity- and sex-adjusted birthweight centile of the baby.
Secondary outcomes
-
Maternal insulin resistance at 36 weeks’ gestation, which will be correlated with adverse pregnancy outcomes.
-
Maternal anthropometry and body composition at 16 and 36 weeks’ gestation and 3 months post-partum.
-
Baby anthropometry and body composition at birth and 3 months of age.
-
Maternal inflammatory markers and lipid and fatty acid indices prior to commencing treatment and again at 28 and 36 weeks’ gestation, including CRP, IL-6, leptin, lipid profile, NEFAs, polyunsaturated fatty acids and PAI-1 : PAI-2 ratio.
-
Neonatal CRP, glucose, insulin and other inflammatory and metabolic indices as previously described (measured in cord blood at birth).
-
Incidence of low birthweight centile.
-
Liquid chromatography-mass spectrometry measurement of metformin in maternal plasma to determine adherence.
Secondary outcomes from nested substudies
-
Maternal salivary cortisol levels at baseline and 28 and 36 weeks’ gestation.
-
Hepatic and peripheral insulin sensitivity at 36 weeks’ gestation as measured by the hyperinsulinaemic–euglycaemic clamp technique.
-
Maternal brachial artery endothelium FMD measured at 16 and 36 weeks’ gestation.
-
Maternal subcutaneous and visceral adipose tissue deposition and hepatic and skeletal muscle ectopic fat deposition assessed using magnetic resonance imaging (MRI) and magnetic resonance spectroscopy (MRS).
-
Fetal liver volume and fetal subcutaneous fat deposition assessed using MRI.
-
In vivo measurements of myometrial contractility on myometrial biopsies obtained at the time of caesarean section.
-
Placental glucocorticoid receptor and 11β-HSD1 and 11β-HSD2 mRNA levels.
Side effects and adverse events reporting
Participants were instructed to contact their investigator at any time after consenting to randomisation if any symptoms developed. In the case of any events, investigators initiated the appropriate treatment according to their medical judgement. Participants with adverse events present at their last visit were followed up until resolution of the event. All adverse events and serious adverse events (SAEs) that occurred after randomisation were recorded in detail in the participants’ medical notes. SAEs occurring in the mother or baby from the time that a participant was randomised until 30 days after stopping taking the study treatment or 28 days after delivery (whichever was later) were reported to the cosponsors using the trial documentation (see Appendix 5). The standard definition of a SAE was used. 30 For the purposes of this study the following events were not considered SAEs: miscarriage; preterm labour; preterm, prelabour spontaneous rupture of membranes; preterm delivery in the maternal interest; preterm delivery in the fetal interest; hospitalisation for pregnancy-induced hypertension; hospitalisation for maternal discomfort; hospitalisation for rest; hospitalisation for observation or monitoring for which the woman was admitted for a period of < 12 hours; delivery complications such as caesarean section or post-partum haemorrhage; or admission of the baby to the neonatal unit for a period of up to 14 days.
Sample size
We calculated that a sample size of 143 participants in each group would have 80% power to detect a difference in mean birthweight centile of 0.33 standard deviations (SDs) (equivalent to the difference between a placebo mean of 4000 g and a metformin mean of 3800 g) at the 5% significance level (two-sided) using a two-group t-test. We initially aimed to randomise 400 participants and anticipated high adherence and participant retention. We increased our sample size to 450 participants when it became apparent that adherence and retention were lower than anticipated.
Statistical analysis
Both intention-to-treat (ITT) analysis and per-protocol analysis are reported. ITT analysis uses data from all randomised participants by allocated treatment. Per-protocol analysis compares outcomes among only those who were compliant with treatment. Adherence was determined prior to review of the data and/or unblinding as follows: the number of weeks from randomisation to delivery was calculated for each participant and those participants reporting (using their study diary) that they took at least one tablet on at least 4 days per week for at least half of those weeks were deemed to have been compliant.
We performed exploratory analysis of secondary outcomes. No formal adjustment was made to any p-values to allow for the large numbers of secondary end points analysed and so the p-values for the secondary analyses should be interpreted conservatively. Post hoc analysis of safety outcomes of all reported SAEs and the combined outcomes of stillbirth, neonatal death, termination of pregnancy and miscarriage was also performed.
Birthweight centiles and z-scores of birthweight centiles (live births only) were derived for each patient after adjustment for sex, gestational age and parity (nulliparous vs. multiparous). 33 Z-scores were compared between the metformin group and the placebo group using a linear regression model, adjusted for treatment centre and BMI band (30–39 kg/m2 vs. ≥ 40 kg/m2) to obtain the adjusted mean difference (with 95% CI). This method was also used for other continuous outcomes including glucose, insulin and homeostatic model assessment – insulin resistance (HOMA-IR) measurement. When necessary, log transformations were performed to achieve a normal distribution of data prior to statistical testing. For umbilical cord blood CRP, Kruskal–Wallis one-way analysis of variance (ANOVA) was used, as this variable could not be transformed into a normal distribution. Unadjusted logistic regression for binary outcomes and Fisher’s exact test were used when event counts were small. Relevant denominators were either all those randomised for whom information was available or those having a live birth for whom information was available.
A statistical analysis plan was finalised and ‘signed off’ before data lock and unblinding (see Appendix 6).
Analysis of the clinical main study outcome data was performed using the statistical programme SAS (version 9.3; SAS Institute Inc., Cary, NC, USA). Analysis of the substudy data was performed using the statistical programmes SAS (version 9.3) and Prism (version 6.0; GraphPad, La Jolla, CA, USA).
Chapter 3 Trial results
Recruitment
In total, 4867 women were approached to participate in the trial. Of these, 4418 were excluded for the following reasons: 2872 declined to participate, 730 did not meet the eligibility criteria, 752 were subsequently uncontactable, 56 were excluded for a variety of other reasons (e.g. they did not speak sufficient English or they were unable to attend extra hospital appointments) and eight did not attend the subsequent screening appointment. The majority of people initially approached to participate in the trial declined to do so. We were unable to formally quantify the reasons for this but anecdotally the most common reasons were a concern that the medication might be harmful to the baby and a lack of appreciation of the adverse effects of obesity on pregnancy outcomes.
Flow of participants through the trial
Figure 1 shows the flow of participants through the trial.
FIGURE 1.
Flow of participants through the trial. a, Other reasons for non-recruitment: change in eligibility from screening of notes to recruitment visit [unable to arrange recruitment visit prior to 16 weeks (n = 26), recruitment stopped prior to screening appointment (n = 14), miscarriage (n = 2), moved out of area (n = 1)], unable to provide informed consent because of lack of spoken English-language ability (n = 5), own doctor or midwife advised against participation (n = 4) and duplicate note screening number issued in error (n = 4).
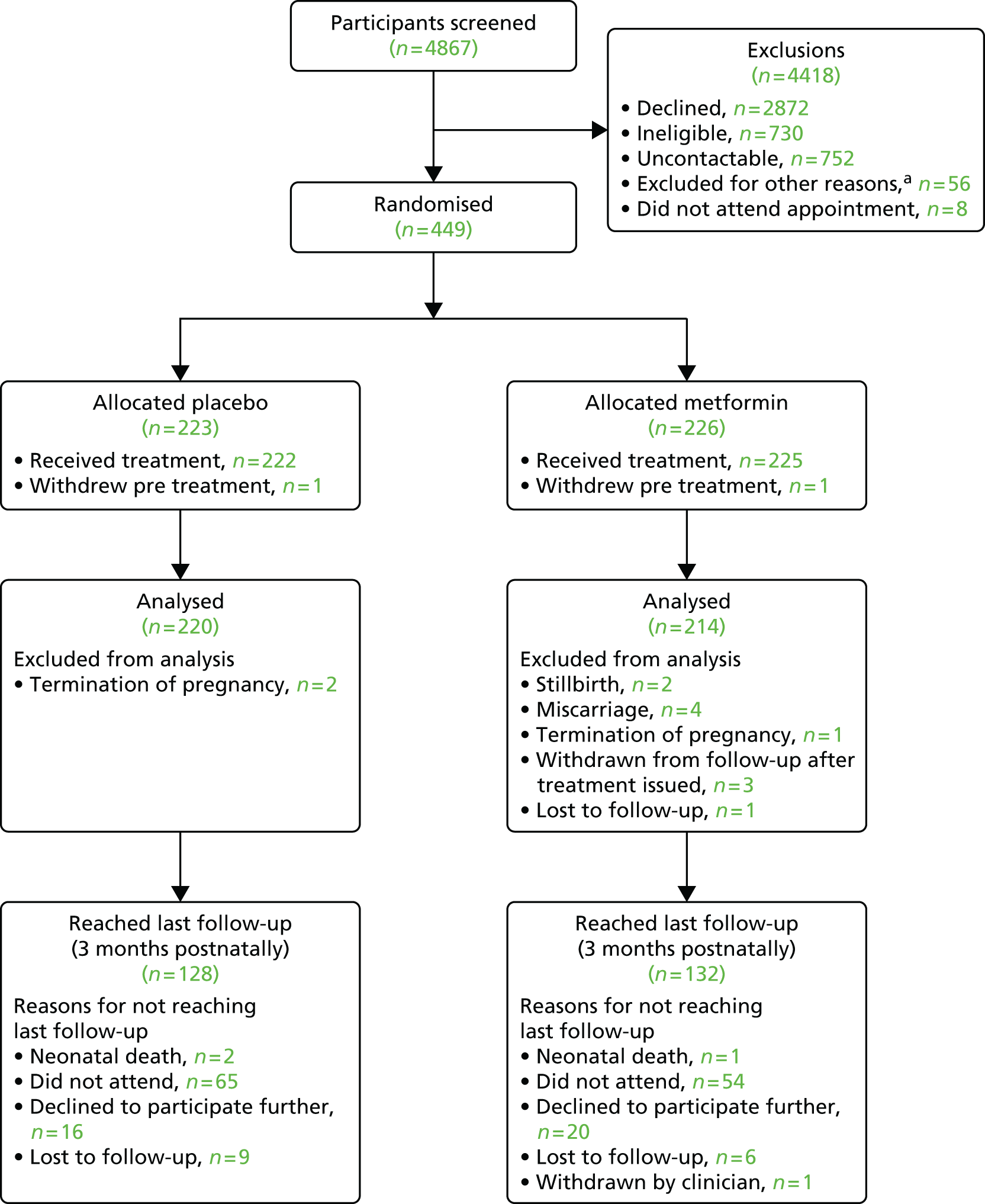
Baseline comparability
There were no differences between the two groups in terms of demographic or anthropometric characteristics at baseline (Table 3). Numbers recruited by each recruiting centre are shown in Table 4.
Characteristics | ITT analysisa | Per-protocol analysisa | ||
---|---|---|---|---|
Placebo (n = 223) | Metformin (n = 226) | Placebo (n = 118) | Metformin (n = 109) | |
Demographics and lifestyle (participant) | ||||
Age (years), mean (SD) | 28.9 (5.1) | 28.7 (5.8) | 29.6 (5.0) | 29.6 (5.6) |
Current smoking, n (%) | 31 (13.9) | 40 (17.7) | 13 (11.0) | 13 (11.9) |
Current alcohol use, n (%) | 9 (4.0) | 3 (1.3) | 6 (5.1) | 0 (0) |
Illicit drug use, n (%) | 1 (0.4) | 0 (0) | 0 (0) | 0 (0) |
Highest educational qualifications, n (%) | ||||
Up to 16 years | 79 (35.4) | 75 (33.2) | 37 (31.4) | 26 (23.9) |
> 16 years | 144 (64.6) | 151 (66.8) | 81 (68.6) | 83 (76.1) |
At least one previous pregnancy of ≥ 12 weeks’ gestation, n (%) | 161 [220] (73.2) | 147 (65.0) | 87 (73.7) | 68 (62.4) |
Systolic blood pressure (mmHg), mean (SD) | 119.4 (10.4) | 117.6 (10.8) | 119.3 (11.2) | 117.1 (11.3) |
Diastolic blood pressure (mmHg), mean (SD) | 68.9 (7.3) | 68.0 (7.8) | 69.0 (7.7) | 68.5 (7.9) |
Gestation at recruitment (days), mean (SD) | 98.9 (8.7) | 99.1 (8.1) | 98.9 (9.0) | 100.0 (7.9) |
Putative father, mean (SD) | ||||
Height (cm) | 178.5 (8.3) | 177.1 (13.7) | 178.5 (7.8) | 177.9 (13.2) |
Weight (kg) | 92.3 (22.5) | 93.5 (25.8) | 92.1 (21.9) | 94.6 (27.7) |
Ethnicity, n (%) | ||||
Caucasian | 214 (96.0) | 210 (93.8) | 114 (96.6) | 101 (92.7) |
Mixed | 4 (1.8) | 4 (1.8) | 1 (0.8) | 2 (1.8) |
Asian | 0 (0) | 3 (1.3) | 0 (0) | 2 (1.8) |
Black | 4 (1.8) | 6 (2.7) | 2 (1.7) | 3 (2.8) |
Chinese | 0 (0) | 0 (0) | 0 (0) | 0 (0) |
Other | 1 (0.4) | 1 (0.4) | 1 (0.8) | 1 (0.9) |
Medical history (participant), n (%) | ||||
Pre-eclampsia or pregnancy-induced hypertension | 7 (3.1) | 10 (4.4) | 3 (2.5) | 6 (5.5) |
Pre-pregnancy hypertension requiring treatment | 2 (0.9) | 1 (0.4) | 1 (0.8) | 1 (0.9) |
PCOS | 21 (9.4) | 28 (12.4) | 14 (11.9) | 16 (14.7) |
Depression requiring treatment | 71 (31.8) | 48 (21.2) | 33 (28.0) | 24 (22.0) |
Anxiety requiring treatment | 20 (9.0) | 15 (6.6) | 7 (5.9) | 7 (6.4) |
Family history (participant), n (%) | ||||
Cardiovascular disease | 69 (30.9) | 71 (31.4) | 41 (34.7) | 31 (28.4) |
Pre-eclampsia | 22 (9.9) | 19 (8.4) | 8 (6.8) | 4 (3.7) |
Diabetes mellitus | 101 (45.3) | 99 (43.8) | 54 (45.8) | 47 (43.1) |
Other | 96 (43.0) | 109 (48.2) | 58 (49.2) | 57 (52.3) |
Participant anthropometry, mean (SD) | ||||
Height (cm) | 165.1 (5.9) | 165.5 (5.9) | 166.1 (6.0) | 165.8 (5.7) |
Weight (kg) | 102.9 (17) | 103.6 (15.5) | 103.7 (17.0) | 104.0 (15.2) |
BMI calculated (kg/m2) | 37.7 (5.6) | 37.8 (5.0) | 37.5 (5.5) | 37.8 (4.7) |
Waist (cm) | 108.7 [222] (13.5) | 110.1 [225] (11.9) | 108.3 (12.6) | 108.6 (11.2) |
Hip (cm) | 126.4 [222] (12.1) | 127.4 [225] (11.8) | 126.8 (11.6) | 127.5 (12.2) |
Mid-arm (cm) | 36.3 [220] (5.0) | 36.7 [221] (4.7) | 36.6 (4.7) | 37.1 (4.4) |
Mid-thigh (cm) | 64.1 [219] (7.7) | 64.2 [222] (6.9) | 64.2 (7.3) | 65.3 (7.0) |
Tricep skinfold (mm) | 31.2 [222] (9.7) | 31.9 [222] (10.8) | 33.3 (9.4) | 32.6 (9.7) |
Bicep skinfold (mm) | 25.7 [222] (10.0) | 27.4 [222] (10.9) | 27.4 (10.1) | 27.8 (10.7) |
Subscapular skinfold (mm) | 32.0 [222] (12.2) | 32.6 [220] (11.8) | 35.3 (11.0) | 34.8 (11.7) |
% fatb | 46.8 [48] (5.6) | 48.2 [53] (5.2) | 46.2 (5.2) | 48.6 (5.0) |
Baseline bloods (recruitment visit), mean (SD) | ||||
Gestation of sampling (days) | 101.1 (8.1) | 100.8 (7.4) | 98.9 (9.0) | 100.0 (7.9) |
Fasting glucose (mmol/l) | 4.39 (0.34) | 4.41 (0.40) | 4.42 (0.36) | 4.41 (0.37) |
2-hour glucose (mmol/l)c | 5.50 (1.09) | 5.20 (1.08) | 5.54 (1.18) | 5.17 (1.10) |
Fasting insulin (µIU/ml) | 22.08 [189] (10.20) | 21.95 [188] (12.26) | 22.96 [101] (10.46) | 21.92 [92] (8.99) |
HOMA-IR scored | 4.36 [189] (2.16) | 4.36 [188] (2.76) | 4.59 [101] (2.32) | 4.34 [92] (1.82) |
CRP (mg/l) | 11.1 (7.4) | 10.7 (6.9) | 11.4 (7.9) | 10.0 (6.3) |
Cholesterol (mmol/l) | 4.87 (1.15) | 4.88 (1.09) | 4.86 [117] (1.16) | 4.82 [108] (1.13) |
HDL (mmol/l) | 1.67 (0.39) | 1.64 (0.38) | 1.67 [117] (0.38) | 1.64 [108] (0.39) |
LDL (mmol/l) | 2.91 (0.78) | 2.89 (0.86) | 2.98 [106] (0.75) | 2.90 [101] (0.90) |
Triglycerides (mmol/l) | 1.51 (0.53) | 1.43 (0.56) | 1.51 [117] (0.54) | 1.45 [108] (0.58) |
Recruiting centre | Placebo (n = 223), n (%) | Metformin (n = 226), n (%) | Overall (n = 449), n (%) |
---|---|---|---|
Royal Infirmary of Edinburgh | 60 (26.9) | 59 (26.1) | 119 (26.5) |
University Hospital Coventry and Warwickshire | 49 (22.0) | 49 (21.7) | 98 (21.8) |
Liverpool Women’s Hospital | 38 (17.0) | 39 (17.3) | 77 (17.1) |
Sheffield Teaching Hospital | 24 (10.8) | 24 (10.6) | 48 (10.7) |
Nottingham City Hospital | 7 (3.1) | 6 (2.7) | 13 (2.9) |
Nottingham Queen’s Medical Centre | 8 (3.6) | 6 (2.7) | 14 (3.1) |
Bradford Royal Infirmary | 4 (1.8) | 4 (1.8) | 8 (1.8) |
Whiston Hospital, St Helens and Knowsley Hospitals | 1 (0.4) | 3 (1.3) | 4 (0.9) |
Chelsea and Westminster Hospital | 0 | 1 (0.4) | 1 (0.2) |
Royal Preston Hospital | 18 (8.1) | 18 (8.0) | 36 (8.0) |
Arrowe Park Hospital, Wirral | 3 (1.3) | 4 (1.8) | 7 (1.6) |
Chesterfield Royal Hospital | 11 (4.9) | 12 (5.3) | 23 (5.1) |
Royal Blackburn Hospital | 0 | 1 (0.4) | 1 (0.2) |
Losses to follow-up
Of the 449 women who were randomised, two withdrew and did not receive their treatment allocation. After the allocated treatment was issued, a further three withdrew from the study (including withdrawing their consent for analysis of the data) and one woman was lost to follow-up (see Figure 1). No participant was considered a protocol violator and no participants were unblinded to the study team before ascertainment of outcomes.
Adherence to the intervention
From participant diary returns and analysis using predefined criteria, 118 out of 177 (67%) in the placebo group and 109 out of 167 (65%) in the metformin group were deemed ‘adherent’. If those who did not return their diary were assumed not to be adherent, calculated adherence would fall to 53% and 48%, respectively. Subsequent analysis of metformin levels showed that detectable levels of metformin were present in the blood of 80 out of 131 (61%) women in the metformin group who gave a blood sample at 36 weeks’ gestation. To explore dosage, we determined the proportion of drug-taking days when 2500 mg or 2000 mg of study drug was taken. Over the entire study, there were 35,686 days when diary data indicated consumption of at least one tablet of study drug. In the placebo group, for 56% of those days the maximum dose of 2500 mg was taken and for 68% of those days a dose of ≥ 2000 mg was taken. The corresponding figures for the metformin group were 38% and 62%.
Primary outcome
Mean (SD) birthweight at delivery was 3463 g (660 g) in the placebo group and 3462 g (548 g) in the metformin group. The primary outcome of z-score of birthweight centile for babies live-born at ≥ 24 weeks’ gestation, adjusted for gestation at delivery, parity and sex, was similar in the placebo and metformin groups for both the ITT analysis (adjusted mean difference –0.029, 95% CI –0.271 to 0.158; p = 0.7597) and the per-protocol analysis (adjusted mean difference 0.068, 95% CI –0.188 to 0.324; p = 0.6001) (Table 5). The distribution of the primary outcome in the two treatment groups is shown in Figure 2.
Outcome | ITT | Per protocol | ||||||||
---|---|---|---|---|---|---|---|---|---|---|
Placebo | Metformin | Adjusted mean difference | 95% CI | p-value | Placebo | Metformin | Adjusted mean difference | 95% CI | p-value | |
Primary outcome [N], mean (SD) | ||||||||||
z-score of birthweight centilea | 0.2680 [220] (1.0055) | 0.2464 [214] (1.0179) | –0.029 | –0.217 to 0.158 | 0.7597 | 0.3130 [117] (0.9781) | 0.3604 [108] (1.0580) | 0.068 | –0.188 to 0.324 | 0.6001 |
Birth outcome (all births) [N], n (%) | ||||||||||
Live births at ≥ 24 weeks’ gestation | 220 [222] (99.1) | 214 [221] (96.8) | 117 [118] (99.2) | 108 [108] (100) | ||||||
Stillbirths at ≥ 24 weeks’ gestation, miscarriage or termination of pregnancy | 2b [222] (0.9) | 7c [221] (3.2) | 3.597d | 0.739 to 17.504 | 0.113 | 1 (0.8) | 0 (0) | < 0.001d | < 0.001 to > 999.999 | 0.9604 |
Birth outcome (babies live-born at ≥ 24 weeks’ gestation) | ||||||||||
Gestational age at delivery (days) [N], mean (SD) | 275.9 [220] (15.9) | 276.6 [214] (11.7) | 277.6 [117] (12.7) | 276.6 [108] (11.5) | ||||||
Male sex [N], n (%) | 109 [220] (49.5) | 109 [214] (50.9) | 58 [118] (49.2) | 54 [108] (50) | ||||||
Birthweight at delivery (g) [N], mean (SD) | 3463 [220] (660) | 3462 [214] (548) | 3539.0 [117] (553.9) | 3503.6 [108] (562.8) | ||||||
Birthweight centile [N], mean (SD) | 57.3 [220] (27.9) | 56.9 [214] (28.6) | 58.527 [117] (27.7) | 59.894 [108] (28.3) |
FIGURE 2.
Distribution of the primary outcome (birthweight) in the (a) placebo and (b) metformin groups.


Secondary outcomes
Maternal outcomes
There was no evidence of a reduction in the main secondary outcome of HOMA-IR at 36 weeks’ gestation (Table 6). Mean HOMA-IR in the placebo and metformin groups was 5.98 and 6.30 molar units, respectively (adjusted mean ratio 0.974, 95% CI 0.865 to 1.097). Similarly, there was no evidence of a statistically significant effect of metformin on fasting or 2-hour glucose (after a 75-g oral glucose challenge) or fasting insulin at 36 weeks’ gestation (see Table 6). However, fasting glucose and the HOMA-IR score at 28 weeks’ gestation were lower in the metformin group (adjusted mean difference/ratio –0.105 mmol/l, 95% CI –0.193 to –0.016 mmol/l; and 0.895 molar units, 95% CI 0.803 to 0.998 molar units, respectively) (data not shown).
Outcome | ITT | Per protocol | ||||||||||||
---|---|---|---|---|---|---|---|---|---|---|---|---|---|---|
Placebo | Metformin | Adjusted mean difference/ratio | 95% CI | p-value | Placebo | Metformin | Adjusted mean difference/ratio | 95% CI | p-value | |||||
Mean (n) | SD | Mean (n) | SD | Mean (n) | SD | Mean (n) | SD | |||||||
Maternal biochemistry at 36 weeks’ gestation | ||||||||||||||
Fasting glucose (mmol/l) | 4.42 (151) | 0.48 | 4.35 (143) | 0.45 | –0.060 | –0.163 to 0.043 | 0.250 | 4.43 (104) | 0.51 | 4.34 (93) | 0.45 | –0.091 | –0.221 to 0.040 | 0.1726 |
2-hour glucose (mmol/l)a | 5.96 (148) | 1.46 | 5.70 (142) | 1.32 | –0.251 | –0.565 to 0.062 | 0.116 | 6.04 (103) | 1.53 | 5.79 (92) | 1.34 | –0.248 | –0.643 to 0.148 | 0.2179 |
Fasting insulin (µIU/ml) | 30.09 (131) | 13.12 | 32.79 (127) | 24.55 | 1.005 | 0.901 to 1.120 | 0.934 | 31.89 (88) | 13.40 | 32.59 (79) | 26.07 | 0.939 | 0.819 to 1.075 | 0.3576 |
HOMA-IR scoreb | 5.98 (131) | 2.89 | 6.30 (123) | 4.78 | 0.974 | 0.865 to 1.097 | 0.666 | 6.36 (88) | 2.96 | 6.22 (77) | 4.90 | 0.912 | 0.784 to 1.060 | 0.2290 |
CRP (mg/l) | 9.20 (150) | 7.10 | 7.47 (140) | 4.62 | 0.860 | 0.743 to 0.996 | 0.043 | 8.91 (104) | 6.39 | 7.48 (93) | 4.58 | 0.901 | 0.760 to 1.070 | 0.2329 |
Cholesterol (mmol/l) | 6.32 (144) | 1.44 | 6.33 (139) | 1.74 | 1.004 | 0.954 to 1.056 | 0.875 | 6.29 (100) | 1.54 | 6.16 (91) | 1.88 | 0.974 | 0.913 to 1.039 | 0.4230 |
HDL (mmol/l) | 1.70 (145) | 0.38 | 1.76 (138) | 0.43 | 0.051 | –0.040 to 0.142 | 0.273 | 1.71 (100) | 0.37 | 1.76 (91) | 0.38 | 0.055 | –0.046 to 0.155 | 0.2866 |
LDL (mmol/l) | 3.57 (126) | 1.13 | 3.77 (118) | 1.25 | 1.064 | 0.982 to 1.152 | 0.127 | 3.67 (89) | 1.09 | 3.71 (80) | 1.22 | 1.013 | 0.923 to 1.113 | 0.7793 |
Triglycerides (mmol/l) | 2.79 (146) | 0.84 | 2.76 (140) | 0.88 | 0.993 | 0.926 to 1.064 | 0.833 | 2.79 (101) | 0.90 | 2.84 (92) | 0.96 | 1.031 | 0.942 to 1.127 | 0.5073 |
IL-6 (mmol/l) | 3.86 (131) | 4.10 | 2.93 (127) | 1.37 | 0.847 | 0.754 to 0.952 | 0.006 | 3.66 (88) | 3.73 | 2.77 (79) | 1.26 | 0.858 | 0.745 to 0.988 | 0.0333 |
Leptin (ng/ml) | 105.0 (131) | 52.4 | 106.6 (127) | 58.8 | 1.005 | 0.902 to 1.120 | 0.927 | 103.80 (88) | 55.34 | 101.26 (79) | 47.02 | 1.007 | 0.886 to 1.145 | 0.9152 |
Serum cortisol (nmol/l) | 821.7 (131) | 232.9 | 867.0 (127) | 225.5 | 1.062 | 0.999 to 1.128 | 0.052 | 806.48 (88) | 225.00 | 888.39 (79) | 250.73 | 1.092 | 1.010 to 1.181 | 0.0281 |
NEFA (mmol/l) | 0.47 (131) | 0.18 | 0.46 (127) | 0.19 | 0.947 | 0.859 to 1.044 | 0.273 | 0.47 (88) | 0.19 | 0.48 (79) | 0.21 | 1.041 | 0.919 to 1.179 | 0.5249 |
PAI1/PAI2 ratio | 3.20 (131) | 2.61 | 2.97 (128) | 2.79 | 0.913 | 0.771 to 1.081 | 0.291 | 3.40 (91) | 2.65 | 3.31 (82) | 3.09 | 0.895 | 0.721 to 1.113 | 0.3167 |
Cord blood biochemical outcomes | ||||||||||||||
Glucose (mmol/l) | 3.89 (79) | 1.24 | 4.06 (74) | 1.08 | 1.067 | 0.974 to 1.170 | 0.164 | 3.94 (62) | 1.25 | 4.02 (54) | 1.05 | 1.062 | 0.955 to 1.181 | 0.2626 |
Insulin (µIU/ml) | 10.95 (47) | 7.49 | 11.41 (57) | 8.80 | 1.060 | 0.767 to 1.463 | 0.722 | 11.14 (37) | 7.48 | 12.04 (45) | 9.21 | 1.137 | 0.805 to 1.607 | 0.4602 |
HOMA-IR scoreb | 1.92 (38) | 1.39 | 1.91 (41) | 2.00 | 1.012 | 0.701 to 1.462 | 0.947 | 1.83 (32) | 1.36 | 1.93 (30) | 2.19 | 1.066 | 0.720 to 1.579 | 0.7436 |
CRP (mg/l)c | 4.32 (78) | 19.55 | 2.36 (73) | 2.29 | 0.741 | 4.85 (62) | 21.89 | 2.15 (53) | 1.82 | 0.7987 |
Metformin had no effect on maternal weight gain during pregnancy (adjusted mean difference in weight gain –0.680 kg, 95% CI –1.863 to 0.503 kg) or maternal weight retention at 3 months post partum (Table 7). We subsequently performed an analysis of weight gain adjusted for baseline weight and again found no significant effect of metformin on weight gain (adjusted mean difference in weight gain –0.637 kg, 95% CI –1.819 to 0.544 kg). There were no differences in the other anthropometric measures of waist, hip, mid-arm and mid-thigh circumference or bicep, tricep and subscapular skinfold thickness (see Table 7).
Outcome | ITT | Per protocol | ||||||||||||||
---|---|---|---|---|---|---|---|---|---|---|---|---|---|---|---|---|
36 weeks | 3 months post-partum | 36 weeks | 3 months post-partum | |||||||||||||
Placebo | Metformin | Placebo | Metformin | Placebo | Metformin | Placebo | Metformin | |||||||||
Mean (n) | SD | Mean (n) | SD | Mean (n) | SD | Mean (n) | SD | Mean (n) | SD | Mean (n) | SD | Mean (n) | SD | Mean (n) | SD | |
Maternal anthropometry | ||||||||||||||||
Height (cm) | 166.0 (153) | 6.0 | 166.3 (142) | 5.6 | 165.3 (125) | 5.9 | 166.1 (127) | 5.8 | 166.2 (105) | 6.1 | 166.4 (94) | 5.7 | 165.6 (89) | 6.0 | 166.2 (89) | 5.7 |
BMI (kg/m2) | 40.4 (153) | 5.4 | 40.6 (141) | 4.9 | 37.4 (124) | 5.2 | 38.3 (124) | 5.6 | 40.2 (105) | 5.4 | 40.4 (93) | 4.7 | 37.1 (89) | 4.9 | 38.0 (87) | 5.5 |
Waist (cm) | 120.0 (155) | 13.2 | 119.0 (142) | 11.1 | 109.2 (124) | 12.8 | 109.9 (125) | 13.9 | 119 (106) | 12.6 | 117.4 (93) | 10.9 | 108.4 (88) | 13.0 | 109.3 (89) | 13.2 |
Hip (cm) | 130.1 (155) | 12.3 | 131.3 (142) | 11.8 | 127.3 (124) | 12.2 | 128.6 (125) | 13.4 | 129.8 (106) | 11.9 | 131.1 (93) | 11.8 | 127.1 (88) | 12.0 | 127.7 (89) | 13.4 |
Mid-arm (cm) | 36.5 (154) | 4.9 | 36.5 (142) | 4.4 | 37.1 (123) | 4.7 | 37.4 (125) | 4.4 | 36.4 (105) | 4.7 | 36.4 (93) | 4.4 | 37.2 (87) | 4.6 | 37.0 (89) | 4.2 |
Mid-thigh (cm) | 65.3 (154) | 7.4 | 65.2 (139) | 6.8 | 64.3 (122) | 6.7 | 65.8 (124) | 6.8 | 64.8 (105) | 7.2 | 65.7 (93) | 6.6 | 64.7 (86) | 6.3 | 65.7 (88) | 6.7 |
Tricep skinfold (mm) | 30.4 (155) | 10.3 | 31.3 (143) | 12.0 | 32.2 (123) | 10.8 | 33.4 (125) | 11.4 | 31.4 (106) | 9.4 | 33.4 (94) | 11.5 | 33.1 (87) | 11.0 | 33.8 (89) | 12.6 |
Bicep skinfold (mm) | 26.0 (155) | 10.5 | 26.9 (143) | 11.6 | 27.2 (123) | 12.1 | 29.7 (125) | 15.1 | 26.7 (106) | 10.3 | 28.4 (94) | 12.2 | 27.5 (87) | 12.5 | 29.5 (89) | 16.5 |
Subscapular skinfold (mm) | 32.7 (154) | 13.5 | 34.5 (141) | 13.9 | 33.2 (123) | 13.1 | 35.9 (124) | 13.2 | 34.9 (105) | 13.1 | 36.3 (92) | 12.6 | 35.1 (87) | 13.3 | 36.4 (89) | 12.8 |
Maternal % fata | 46.3 (31) | 4.84 | 47.8 (30) | 4.63 | 47.45 (29) | 4.97 | 48.35 (30) | 5.31 | 45.6 (22) | 4.7 | 47.4 (29) | 4.7 | 46.8 (21) | 5.0 | 48.5 (27) | 4.8 |
Weight gain during pregnancy (kg) | 7.23 (156) | 4.91 | 6.70 (143) | 6.00 | –0.13 (124) | 6.22 | 0.07 (124) | 9.82 | 7.40 (106) | 4.6 | 6.85 (93) | 6.1 | 0.05 (89) | 6.1 | 0.83 (87) | 11.0 |
Outcome | ITT | Per protocol | ||||||||||||||
Within 72 hours of birth | 3 months of age | Within 72 hours of birth | 3 months of age | |||||||||||||
Placebo | Metformin | Placebo | Metformin | Placebo | Metformin | Placebo | Metformin | |||||||||
Mean (n) | SD | Mean (n) | SD | Mean (n) | SD | Mean (n) | SD | Mean (n) | SD | Mean (n) | SD | Mean (n) | SD | Mean (n) | SD | |
Neonatal anthropometry (live births only) | ||||||||||||||||
Age at which measurements made (days) | 1.04 (157) | 2.44 | 0.97 (145) | 2.44 | 99.59 (128) | 13.12 | 97.72 (129) | 14.01 | 1.34 (97) | 3.0 | 1.12 (89) | 2.6 | 99.95 (91) | 11.9 | 96.84 (90) | 13.9 |
Length (cm)b | 51.2 (150) | 4.0 | 50.7 (139) | 3.3 | 62.13 (124) | 4.4 | 61.69 (125) | 6.33 | 51.4 (94) | 3.0 | 51.1 (83) | 3.3 | 62.41 (89) | 3.9 | 61.51 (90) | 7.2 |
Head circumference (cm) | 34.7 (164) | 4.2 | 34.8 (152) | 3.6 | 41.30 (124) | 2.87 | 41.02 (122) | 4.42 | 35.32 (99) | 1.8 | 34.88 (89) | 4.5 | 41.08 (89) | 2.0 | 41.11 (88) | 5.1 |
Ponderal index [mass (g)/height (cm)3]b | 2.6 (143) | 0.41 | 2.67 (130) | 0.50 | 2.58 (124) | 0.82 | 2.52 (124) | 1.00 | 2.64 (90) | 0.42 | 2.63 (79) | 0.46 | 2.48 (89) | 0.6 | 2.59 (89) | 1.05 |
Tricep skinfold thickness (mm) | 14.3 (111) | 20.6 | 16.4 (99) | 27.9 | 22.05 (106) | 10.40 | 24.61 (104) | 11.00 | 16.26 (79) | 22.0 | 17.29 (74) | 30.1 | 23.98 (83) | 35.6 | 25.67 (83) | 36.0 |
Subscapular skinfold (mm) | 13.5 (113) | 20.4 | 15.7 (98) | 28.0 | 17.00 (104) | 23.95 | 23.11 (104) | 31.33 | 14.84 (80) | 21.1 | 15.88 (73) | 29.5 | 17.80 (82) | 25.1 | 24.70 (83) | 33.1 |
Baby % fata | 12.1 (22) | 5.7 | 12.9 (21) | 4.5 | 25.88 (31) | 6.13 | 23.19 (29) | 5.91 | 13.45 (15) | 5.8 | 12.77 (20) | 4.6 | 25.80 (22) | 5.9 | 23.33 (26) | 5.7 |
Weight at this time (g)b,c | 3707.76 (164) | 2685.66 | 3455.18 (146) | 545.08 | 6085.04 (128) | 1276.59 | 5971.97 (132) | 1724.20 | 3564.96 (97) | 513.5 | 3492.74 (85) | 578.5 | 6075.67 (90) | 1362.0 | 6108.76 (91) | 1664.0 |
Plasma IL-6 and CRP concentrations were both lower in the group treated with metformin at 36 weeks’ gestation (adjusted mean ratio 0.847 mmol/l, 95% CI 0.754 to 0.952 mmol/l; and 0.860 mg/l, 95% CI 0.743 to 0.996 mg/l, respectively). Cholesterol, HDL, LDL, triglycerides, leptin, NEFA and PAI1/2 ratio at 36 weeks’ gestation were similar in the two groups. There was a trend towards higher serum cortisol at 36 weeks’ gestation in the metformin group on ITT analysis and this reached statistical significance in the per-protocol analysis (adjusted mean ratio 0.088, 95% CI 0.010 to 0.167; p = 0.0281) (see Table 6).
Metformin did not appear to prevent GDM. The proportion of women fulfilling either the IADPSG or the WHO criteria for GDM at any time in pregnancy was similar in the two groups. Post hoc analysis of the timing of diagnosis of GDM (IADPSG criteria) showed no statistically significant difference between the two groups: in the placebo group 26 women were diagnosed at 28 weeks’ gestation and 10 at 36 weeks’ gestation, whereas in the metformin group 11 women were diagnosed at 28 weeks’ gestation and 15 at 36 weeks’ gestation (p = 0.0718, Mantel–Haenszel chi-square, post hoc analysis); however, the trend was towards a later diagnosis in the metformin-treated group.
There were no differences in outcomes at other time points between the two groups, with the exception of fasting glucose and HOMA-IR score, as mentioned previously.
Further analysis of the data on a per-protocol basis resulted in similar findings with a few exceptions. For CRP at 36 weeks’ gestation and vomiting, the direction of difference was maintained but statistical significance was lost. Two-hour glucose and fasting insulin at 28 weeks’ gestation were lower in the metformin group (estimated mean difference –0.312 mmol/l, 95% CI –0.620 to –0.004 mmol/l; p = 0.0471; and 0.871 µIU/ml, 95% CI 0.778 to 0.976 µIU/ml; p = 0.0173, respectively). Serum cortisol at 36 weeks’ gestation was higher in the metformin-treated group (estimated mean difference 0.088 nmol/l, 95% CI 0.010 to 0.167 nmol/l; p = 0.0281) (summarised in Table 7).
At 36 weeks’ gestation there were no differences in serum B12 or folate levels between the two groups on ITT analysis (adjusted mean difference: B12 0.952 ng/l, 95% CI 0.879 to 1.032 ng/l; p = 0.2296; folate 1.050 µg/l, 95% CI 0.885 to 1.247 µg/l; p = 0.5737). However, on per-protocol analysis, participants in the metformin group had a lower serum B12 concentration at 36 weeks’ gestation (adjusted mean difference 0.890 ng/l, 95% CI –0.804 to 0.985 ng/l; p = 0.0248). There were no differences between the groups in the proportion of participants with a serum B12 or folate concentration < 5th centile (Table 8).
Outcome | ITT | Per protocol | ||||||||
---|---|---|---|---|---|---|---|---|---|---|
Placebo | Metformin | Adjusted mean difference | 95% CI | p-value | Placebo | Metformin | Adjusted mean difference | 95% CI | p-value | |
Serum B12 (ng/l) [n], mean (SD)a | ||||||||||
Baseline | 260.2 [132] (101.3) | 266.3 [131] (92.4) | 256.6 [90] (108.1) | 259.4 [82] (71.6) | ||||||
36 weeksb | 223.7 [130] (69.6) | 215.0 [132] (73.2) | 0.952 | 0.879 to 1.032 | 0.2296 | 226.1 [88] (75.4) | 205.5 [83] (68.6) | 0.890 | 0.804 to 0.985 | 0.0248 |
Proportion with serum B12 < 5th centile, n (%) | ||||||||||
Baseline | 8 (6.1) | 5 (3.8) | 6 (6.7) | 4 (4.9) | ||||||
36 weeks | 6 (4.6) | 7 (5.3) | 1.157c | 0.378 to 3.541 | 0.7979 | 5 (5.7) | 6 (7.2) | 1.294c | 0.379 to 4.411 | 0.6809 |
Serum folate (µg/l) [n], mean (SD)d | ||||||||||
Baseline | 13.84 [132] (4.6) | 13.77 [131] (4.8) | 13.93 [90] (4.6) | 14.48 [82] (4.4) | ||||||
36 weeksb | 8.29 [132] (5.6) | 8.54 [132] (5.6) | 1.050 | 0.885 to 1.247 | 0.5737 | 9.15 [90] (6.0) | 9.46 [83] (5.7) | 1.114 | 0.897 to 1.384 | 0.3263 |
Proportion with serum folate < 5th centile, n (%) | ||||||||||
Baseline | 0 (0) | 2 (1.5) | 0 (0) | 1 (1.2) | ||||||
36 weeks | 10 (7.6) | 11 (8.3) | 1.109c | 0.454 to 2.708 | 0.8201 | 7 (7.8) | 5 (6.0) | 0.760c | 0.232 to 2.495 | 0.6510 |
Neonatal outcomes
The proportion of live-born babies weighing > 90th centile was similar in the two groups [placebo 38/220 (17%), metformin 31/214 (14%)]. Importantly, we also did not see a difference in the proportion of babies weighing < 10th centile [placebo 11/220 (5%), metformin 14/214 (7%)]. There was no significant difference in neonatal ponderal index at birth between the two groups (adjusted mean ratio 1.032 g/cm3, 95% CI 0.996 to 1.069 g/cm3).
Neonatal cord blood glucose, insulin, HOMA-IR and CRP were similar in the two groups (see Table 6).
Adverse events
Maternal symptoms of diarrhoea and vomiting were more common in the metformin group (OR 1.670, 95% CI 1.064 to 2.621; and 3.113, 95% CI 1.975 to 4.908 in the placebo and metformin groups, respectively).
The incidence of other adverse outcomes, including preterm birth and low birthweight, caesarean section and post-partum haemorrhage, was similar in the two groups.
There were no adverse effects of metformin apparent on post hoc safety analyses comparing the proportion of participants with a recordable SAE between the two groups or the combined adverse outcomes of miscarriage, termination of pregnancy, stillbirth or neonatal death (combined adverse outcomes OR 3.597, 95% CI 0.793 to 17.504).
Two participants in the study delivered a stillborn baby. One of the fetuses had a known congenital cardiac defect and developed severe hydrops. The participant went into spontaneous preterm labour at 31 weeks’ gestation and the baby was stillborn. The other participant presented with an intrauterine death at 38 weeks’ gestation and delivered a stillborn baby weighing 2600 g (< 3rd centile for gestation). Both of the participants were in the metformin group. Neither had any recorded diary entries for tablet taking nor met the adherence criteria for inclusion in the per-protocol analysis.
There were four mid-trimester miscarriages in the metformin group. One was at 20 weeks’ gestation following the participant’s involvement in a road traffic accident. One was an intrauterine fetal demise detected at the 20-week fetal anomaly scan. Two were spontaneous miscarriages at 18 weeks’ gestation, with one of these following a spontaneous rupture of membranes.
Three participants underwent termination of pregnancy following randomisation. One participant was in the metformin group and underwent termination for a fetus with trisomy 21. The other two participants were in the placebo group: one fetus was terminated following a spontaneous rupture of membranes at 18 weeks’ gestation and the other because of a fetal anomaly (split hand and foot syndrome).
On per-protocol analysis of these outcomes, only one participant remained eligible for inclusion (one termination of pregnancy in the placebo group).
Adverse outcomes are summarised in Table 9.
Outcome | ITT | Per protocol | ||||||||||||
---|---|---|---|---|---|---|---|---|---|---|---|---|---|---|
Placebo | Metformin | OR | 95% CI | p-value | Placebo | Metformin | OR | 95% CI | p-value | |||||
n (N) | % | n (N) | % | n (N) | % | n (N) | % | |||||||
Women or their babies with a recorded SAE | 41 (222) | 18.5 | 37 (225) | 16.4 | 0.869 | 0.533 to 1.417 | 0.573 | 22 (118) | 18.6 | 14 (109) | 12.8 | 0.643 | 0.311 to 1.331 | 0.2767 |
Maternal outcomes | ||||||||||||||
Any caesarean section in index pregnancya | 76 (222) | 34.2 | 65 (219) | 29.7 | 0.811 | 0.543 to 1.211 | 0.306 | 43 (118) | 36.4 | 31 (108) | 28.7 | 0.702 | 0.401 to 1.230 | 0.2566 |
Primary caesarean section | 46 (222) | 20.7 | 42 (219) | 19.2 | 0.908 | 0.569 to 1.449 | 0.685 | 25 (118) | 21.2 | 22 (108) | 20.4 | 0.952 | 0.500 to 1.811 | 1.0000 |
Post-partum haemorrhage > 1000 ml | 21 (216) | 9.7 | 20 (212) | 9.4 | 0.967 | 0.508 to 1.842 | 0.919 | 13 (118) | 11.0 | 9 (109) | 8.3 | 0.721 | 0.295 to 1.763 | 0.5079 |
Preterm birthb | 14 (220) | 6.4 | 18 (214) | 8.4 | 1.345 | 0.651 to 2.777 | 0.466 | 4 (117) | 3.4 | 8 (108) | 7.4 | 2.260 | 0.661 to 7.732 | 0.2392 |
Development of GDMc | 36 (153) | 23.5 | 26 (142) | 18.3 | 0.728 | 0.414 to 1.283 | 0.273 | 22 (104) | 21.2 | 15 (92) | 16.3 | 0.726 | 0.351 to 1.501 | 0.3877 |
Pregnancy-induced hypertension | 14 (222) | 6.3 | 21 (221) | 9.5 | 1.56 | 0.772 to 3.152 | 0.22 | 11 (118) | 9.3 | 11 (109) | 10.1 | 1.092 | 0.453 to 2.631 | 0.84 |
Pre-eclampsia | 3 (222) | 1.4 | 7 (221) | 3.2 | 2.39 | 0.61 to 9.36 | 0.21 | 3 (118) | 2.5 | 3 (109) | 2.8 | 1.085 | 0.214 to 5.493 | 0.92 |
Fetal and neonatal outcomes (live births only) | ||||||||||||||
Admission to the neonatal unitd | 29 (219) | 13.2 | 14 (213) | 6.6 | 0.461 | 0.236 to 0.899 | 0.023 | 13 (116) | 11.2 | 8 (108) | 7.4 | 0.634 | 0.252 to 1.595 | 0.3329 |
Congenital anomalyd | 8 (217) | 3.7 | 7 (209) | 3.3 | 0.905 | 0.322 to 2.543 | 0.850 | 4 (115) | 3.5 | 4 (107) | 3.7 | 1.078 | 0.263 to 4.421 | 0.9173 |
Neonatal death in the delivery roomd | 0 (220) | 0 | 0 (214) | 0 | 0 (117) | 0 | 0 (108) | 0 | ||||||
Neonatal death at a later staged | 2 (220) | 0.91 | 1 (214) | 0.5 | 1.000 | 0 (117) | 0 | 0 (108) | 0 | |||||
Incidence of low birthweight < 10th centile | 11 (220) | 5.0 | 14 (214) | 6.5 | 1.330 | 0.590 to 2.999 | 0.492 | 6 (117) | 5.1 | 6 (108) | 5.6 | 1.088 | 0.340 to 3.482 | 0.8867 |
Incidence of low birthweight < 3rd centiled | 3 (220) | 1.4 | 3 (214) | 1.4 | 1.000 | 1 (117) | 0.9 | 1 (108) | 0.9 | 1.0000 | ||||
Maternal symptoms up to 36 weeks’ gestatione | ||||||||||||||
Taste disturbance | 32 (198) | 16.2 (199) | 25 | 12.6 | 0.745 | 0.424 to 1.311 | 0.308 | 20 (118) | 16.9 | 17 (109) | 15.6 | 0.905 | 0.447 to 1.835 | 0.7829 |
Skin reactions | 39 (198) | 19.7 (199) | 36 | 18.1 | 0.900 | 0.545 to 1.489 | 0.683 | 23 (118) | 19.5 | 21 (109) | 19.3 | 0.986 | 0.510 to 1.905 | 0.9658 |
Abdominal pain | 42 (198) | 21.2 (199) | 49 | 24.6 | 1.213 | 0.759 to 1.940 | 0.419 | 26 (118) | 22.0 | 32 (109) | 29.4 | 1.471 | 0.807 to 2.678 | 0.2074 |
Flatulence | 44 (198) | 22.2 (199) | 51 | 25.6 | 1.206 | 0.760 to 1.915 | 0.427 | 28 (118) | 23.7 | 38 (109) | 34.9 | 1.720 | 0.964 to 3.069 | 0.0662 |
Constipation | 57 (198) | 28.8 (199) | 57 | 28.6 | 0.993 | 0.643 to 1.534 | 0.975 | 38 (118) | 32.2 | 37 (109) | 33.9 | 1.082 | 0.622 to 1.882 | 0.7805 |
Diarrhoea | 37 (198) | 18.7 (199) | 83 | 41.7 | 3.113 | 1.975 to 4.908 | < 0.0001 | 24 (118) | 20.3 | 60 (109) | 55.0 | 4.896 | 2.669 to 8.617 | < 0.0001 |
Nausea | 79 (198) | 39.9 (199) | 97 | 48.7 | 1.432 | 0.962 to 2.132 | 0.077 | 46 (118) | 39.0 | 49 (109) | 45.0 | 1.278 | 0.754 to 2.168 | 0.3626 |
Vomiting | 43 (198) | 21.7 (199) | 63 | 31.7 | 1.670 | 1.064 to 2.621 | 0.026 | 24 (118) | 20.3 | 34 (109) | 31.2 | 1.775 | 0.970 to 3.249 | 0.0626 |
Headache | 66 (198) | 33.3 (199) | 65 | 32.7 | 0.970 | 0.638 to 1.474 | 0.887 | 40 (118) | 33.9 | 37 (109) | 33.9 | 1.002 | 0.578 to 1.737 | 0.9941 |
Chapter 4 Substudies
Maternal and neonatal body composition
Introduction
Body mass index is routinely used as a proxy for adiposity. However, a limitation of BMI is that it makes no distinction between fat mass and FFM and indeed other components of total body weight. BMI has been shown to be well correlated with adiposity in pregnant women, although with a wide CI34 and a weakening of correlation closer to term. 35 As fat mass is particularly relevant in the context of insulin sensitivity, we aimed to examine the effect of metformin on fat mass specifically, as well as on overall gestational weight change.
The effect of maternal metformin on the body composition of the infant was also of interest in this study. Body composition at birth, which includes fat mass and FFM as opposed to birthweight alone, is probably a more important predictor for long-term risk of disease such as obesity and metabolic syndrome. Whereas FFM is generally a reflection of genetic effects, fat mass is more variable and susceptible to factors that affect fetal growth such as maternal weight gain in pregnancy, maternal obesity and insulin sensitivity. 10,36,37
There are numerous ways to assess body composition, including underwater weighing, dual-energy X-ray absorptiometry and bioelectrical impedence analysis. These all have limitations, particularly in our two subject groups: pregnant women and infants. Air displacement plethysmography (ADP) is increasingly recognised as the gold standard tool to best assess body composition, particularly in these challenging groups. 38 ADP is based on the principle of Boyle’s law, which states that air compressed will decrease in volume proportional to increasing pressure at a constant temperature. The subject is placed inside a sealed chamber of known volume and any change in pressure and volume is attributable to the volume of the subject. The technique is quick and generally acceptable to all, excepting those with severe claustrophobia.
Methods
Maternal fat mass was measured using ADP at baseline, 36 weeks’ gestation and 3 months post-partum. Infant fat mass was measured using the same technique within 72 hours of birth and at 3 months of age.
Equipment was used in a temperature-controlled room (21–27 °C) and calibrated at the start of each day of use.
Adult ADP tests were performed with the BOD POD [see www.lifemeasurement.com (accessed 21 June 2016)]. Subjects were fasted and had abstained from exercise for at least 2 hours prior to the test. They were asked to empty their bladder and remove all jewellery and glasses. The test was performed with the subject wearing minimal skintight clothing, for example swimwear, and a hair cap. Subjects were in a resting state.
Infant ADP tests were performed with the PEA POD (see www.lifemeasurement.com). The infant’s clothes and nappy were removed and a head cap applied or hair smoothed. The instrument was calibrated to account for the mass of two hospital identification bracelets and an umbilical cord clip for measurements in the newborn babies.
Statistical analysis
Data are expressed as mean ± SD. Outcomes were analysed using a linear regression model, adjusted by BMI band. Significance was set at p < 0.05.
Results
Edinburgh participants only were invited to participate in this substudy. The final cohort numbers following unblinding are provided in Table 10.
Outcome | ITT | Per protocol | ||||||||||||
---|---|---|---|---|---|---|---|---|---|---|---|---|---|---|
Placebo | Metformin | Adjusted mean difference | 95% CI | p-value | Placebo | Metformin | Adjusted mean difference | 95% CI | p-value | |||||
Mean (n) | SD | Mean (n) | SD | Mean (n) | SD | Mean (n) | SD | |||||||
Maternal | ||||||||||||||
Body mass (kg) | ||||||||||||||
Baseline | 101.39 (47) | 16.2 | 103.32 (53) | 16.0 | 100.53 (27) | 12.7 | 106.66 (33) | 17.0 | ||||||
36 weeks’ gestation | 108.79 (31) | 14.9 | 113.37 (30) | 15.9 | 106.92 (22) | 10.7 | 112.95 (29) | 16.9 | ||||||
3 months post-partum | 102.82 (29) | 12.8 | 106.47 (30) | 14.8 | 102.72 (21) | 13.7 | 107.63 (27) | 16.2 | ||||||
Fat mass (kg) | ||||||||||||||
Baseline | 47.93 (48) | 12.1 | 50.32 (53) | 11.9 | 1.212 | –2.111 to 4.535 | 0.4708 | 46.68 (27) | 9.2 | 52.12 (33) | 12.2 | 2.578 | –1.160 to 6.316 | 0.1727 |
36 weeks’ gestation | 50.83 (31) | 10.9 | 54.37 (30) | 12.2 | 1.852 | –2.623 to 6.327 | 0.4108 | 48.98 (22) | 8.1 | 54.15 (29) | 12.3 | 3.225 | –1.510 to 7.960 | 0.1773 |
3 months post-partum | 49.06 (29) | 9.0 | 50.09 (30) | 13.7 | –0.002 | –5.227 to 5.223 | 0.9994 | 48.39 (21) | 9.5 | 50.47 (27) | 13.4 | 0.805 | –5.196 to 6.806 | 0.7883 |
Fat (%) | ||||||||||||||
Baseline | 46.82 (48) | 5.6 | 48.19 (53) | 5.2 | 46.21 (27) | 5.2 | 48.56 (33) | 5.0 | ||||||
36 weeks’ gestation | 46.30 (31) | 4.8 | 47.48 (30) | 4.6 | 45.55 (22) | 4.7 | 47.44 (29) | 4.7 | ||||||
3 months post-partum | 47.45 (29) | 5.0 | 48.35 (30) | 5.3 | 46.78 (21) | 5.0 | 48.49 (27) | 4.8 | ||||||
Fat mass % change from baseline to 36 weeksa | –2.461 | –7.034 to 2.111 | 0.2852 | –3.844 | –8.888 to 1.201 | 0.1319 | ||||||||
Fat mass % change from baseline to 3 months post-partuma | –5.078 | –13.052 to 2.896 | 0.2069 | –5.203 | –13.97 to 3.57 | 0.2382 | ||||||||
Neonatal | ||||||||||||||
Body mass (kg) | ||||||||||||||
Birth | 3.40 (22) | 0.5 | 3.38 (21) | 0.4 | 3.49 (15) | 0.5 | 3.38 (20) | 0.4 | ||||||
3 months | 9.68 (30) | 19.4 | 6.01 (28) | 0.9 | 6.24 (22) | 0.8 | 6.07 (25) | 0.9 | ||||||
Fat mass (kg) | ||||||||||||||
Birth | 0.46 (22) | 0.05 | 0.45 (21) | 0.2 | –0.010 | –0.152 to 0.133 | 0.8905 | 0.49 (15) | 0.3 | 0.45 (20) | 0.2 | –0.073 | –0.237 to 0.091 | 0.3727 |
3 months | 1.60 (30) | 0.08 | 1.44 (29) | 0.08 | –0.164 | –0.398 to 0.070 | 0.1660 | 1.61 (22) | 0.5 | 1.44 (26) | 0.5 | –0.192 | –0.459 to 0.076 | 0.1556 |
Fat (%) | ||||||||||||||
Birth | 12.08 (22) | 5.7 | 12.86 (21) | 4.5 | 13.45 (15) | 5.8 | 12.77 (20) | 4.6 | ||||||
3 months | 25.88 (31) | 6.1 | 23.19 (29) | 5.9 | 25.80 (22) | 5.9 | 23.33 (26) | 5.7 | ||||||
Fat mass % change from birth to 3 monthsa | –0.220 | –0.606 to 0.165 | 0.2511 | –0.253 | –0.671 to 0.165 | 0.2235 |
There were no significant differences in maternal fat mass at baseline, 36 weeks’ gestation and 3 months post partum. There was no significant difference in percentage change in maternal fat mass between early and late pregnancy and 3 months post-partum between the placebo group and the metformin group.
There was no significant difference in percentage change in neonatal fat mass from birth to 3 months of age between the placebo group and the metformin group.
Discussion
Metformin taken during pregnancy does not appear to have had an effect on maternal total body fat percentage in late pregnancy or at 3 months post-partum. In the study population as a whole, we did not see any differences in total body weight or skinfold thickness, which would suggest that metformin has not had a significant effect on the distribution of body fat, although the sample size was small. This is supported by our MRI data (see Magnetic resonance imaging assessment of maternal and fetal adipose distribution) in which we did not see any significant differences in body fat distribution between the two groups in a smaller cohort of subjects.
There was no significant difference in body fat percentage in the babies, either at birth or at 3 months of age. This is similar to the findings of the metformin compared with insulin for the treatment of gestational diabetes trial,39 in which there were no differences at birth in birthweight, upper arm circumference, tricep and subscapular skinfold thickness and ponderal index between the babies exposed to metformin and those whose mothers received insulin. However, when these children were assessed again at age 2 years, those who were exposed to metformin had similar total body fat (assessed by dual-energy X-ray absorptiometry) but larger skinfold thickness,40 suggesting that they had less central fat and may therefore be more insulin sensitive in the longer term. This highlights the need for follow-up studies in our cohort to assess any longer-term effects that metformin exposure may have had on fat distribution in these children.
Hyperinsulinaemic–euglycaemic clamp study
Introduction
Normal pregnancy is associated with marked changes in insulin sensitivity, glucose homeostasis and lipid and protein metabolism. In early pregnancy, fasting glucose decreases by 0.11 mmol/l with little further decrease by the end of pregnancy. 41 Insulin secretion increases but insulin sensitivity remains unchanged. 42,43 This promotes lipogenesis to prepare for the rising energy needs of pregnancy and also to allow lipid storage in preparation for the energy demands of lactation. Glucose tolerance is normal at this stage, as is peripheral insulin sensitivity and hepatic basal glucose production. 43–45 By mid-pregnancy, despite the increase in insulin secretion, basal hepatic glucose production also increases, as does total gluconeogenesis, to meet the increasing demands of the fetoplacental unit. 46–48 By late gestation, peripheral insulin sensitivity is markedly decreased such that insulin is unable to suppress lipolysis, allowing an increase in free fatty acids and therefore more energy available for gluconeogenesis. 49 Overall, the insulin sensitivity of late pregnancy is reduced by 50–70% compared with the non-pregnant state. These mechanisms are important to ensure a ready supply of energy substrates for the developing fetus.
In obese pregnant individuals, these mechanisms are disordered. Obesity is associated with a state of diminished insulin sensitivity and so obese women enter pregnancy already resistant to insulin. The reduction in fasting glucose in very early pregnancy is diminished or absent. 41 By late gestation, a physiological reduction in peripheral insulin sensitivity by 15% has been demonstrated. 50 In addition, there is marked hepatic insulin resistance with reduced insulin-mediated glucose disposal and a reduction in insulin-stimulated suppression of endogenous glucose production (EGP). 51 Thus, there may be an excess of free fatty acids and glucose, which are freely transferred across the placenta and may potentially drive fetal overgrowth and programming of later-life insulin resistance. However, our own work52 has demonstrated differences between lean and severely obese pregnant women only at early and mid-gestation, with a convergence in degree of insulin resistance by late pregnancy.
This mechanistic substudy was designed to examine the effect of metformin on insulin resistance in obese pregnancy. We used the gold standard method of assessing insulin sensitivity, the hyperinsulinaemic–euglycaemic clamp, to assess this in a subgroup of women participating in the trial and who were adherent to treatment. To our knowledge, this is the first study to have employed this technique to examine the effect of metformin in pregnant women. The characteristics of the women were similar to those of the women in the study overall. Importantly, those with GDM were excluded.
Methods
Solutions of 6,6–2H2-glucose (d2-glucose) and 1,1,2,3,3–2H5-glycerol (d5-glycerol) (Cambridge Isotope Laboratories, Inc., Andover, MA, USA) and soluble insulin (Actrapid®, NovoNordisk, Bagsvaerd, Denmark) were prepared in 0.9% saline.
Participants (n = 21) attended the clinical research facility at 08.00 after fasting overnight for 8–10 hours. A 44-mm 20-gauge cannula was inserted into the superficial vein in the dorsum of one hand and kept patent with a slow infusion of 0.9% saline. This hand was wrapped in an electric heated blanket to arterialise the venous blood for sample collection. A second cannula was placed in the antecubital fossa vein of the contralateral arm for the infusates. We infused d2-glucose (prime 25 µmol/kg then continuous infusion of 22 µmol/kg/hour) and d5-glycerol (prime 1.6 µmol/kg then continuous infusion of 6.6 µmol/kg/hour) for 5.5 hours. Four steady-state blood samples were taken at 10-minute intervals at the end of three time periods: (1) 60, 70, 80 and 90 minutes; (2) 180, 190, 200 and 210 minutes; and (3) 300, 310, 320 and 330 minutes with infusion of (1) tracers only (no insulin); (2) 20 mU/m2/minute of insulin (to suppress lipolysis and EGP); and (3) 40 mU/m2/minute of insulin (to stimulate glucose uptake). Following commencement of the insulin infusion at 90 minutes, blood samples were obtained every 5 minutes from the sampling cannula for measurement of whole blood glucose concentration using an Accu-Chek® blood glucose monitor (Roche Products Ltd, Welwyn Garden City, UK). A solution of 20% dextrose was infused as required to maintain arteriolised blood glucose between 4.5 and 5.5 mmol/l. Additional blood samples were obtained every 30 minutes from 90 minutes onwards using fluoride oxalate anticoagulant for formal enzymatic measurement of plasma glucose. The steady-state blood samples for analysis of the tracers and those for insulin and NEFAs were collected on ice, the plasma separated by centrifugation and plasma aliquots stored at –80 °C.
The volume of dextrose infused during the final 30 minutes of the high-dose hyperinsulinaemic–euglycaemic clamp divided by the corresponding insulin concentration at steady state was used to derive the glucose disposal per unit plasma insulin or M/I.
Steele’s equation for steady state was applied to calculate the rate of appearance (Ra) or rate of disappearance (Rd) of the tracee (d2-glucose or d5-glycerol):
where F is the infusion rate of the tracer and TTR is the tracer-to-tracee ratio.
Endogenous glucose production was calculated by subtracting the variable glucose infusion rate from the calculated Ra glucose. Data from glucose infusion studies were corrected for background 13C enrichment. No exogenous unlabelled glycerol was infused and the abundance of other isotopic species within the tracer infusion is negligible; therefore, corrections were not applied in the calculation of Ra glycerol.
Mass spectrometry analysis
Standard curves were prepared for concentrations of glucose, glycerol, d2-glucose and d5-glycerol in plasma with internal standards of 13C6-glucose (Isotec, Southport, UK) and butanetriol as previously described. 53 Standard enrichment curves for glucose and glycerol with d2-glucose and d5-glycerol, respectively, were also prepared. Briefly, samples and standards were prepared in acetonitrile (Sigma Aldrich, Gillingham, Dorset, UK), internal standards were added and incubated for 20 minutes, extracts were collected under vacuum and eluates were dried and incubated with pyridine/acetate anhydride [200 µl, 1 : 1 volume per volume (v/v)] before drying again and reconstituting in 5% acetic anhydride in heptane. These were analysed on a Quantum Ultra GC-MS/MS, operated using Xcalibur™ software version 3.0.63 (ThermoFisher Scientific; Hemel Hempstead, UK) using a HP-INNOWax column (30 m × 0.32 mm × 0.25 µm; Agilent Technologies Ltd, Stockport, UK). Monitored ions were the glycerol triacetate m/z 217, d5-glycerol triacetate m/z 222, butanetriol triacetate m/z 231 (internal standard), glucose pentacetate m/z 287, d2-glucose pentacetate m/z 289 and 13C6-glucose pentacetate m/z 293 (internal standard).
Treatment adherence was measured by determining metformin in plasma using an Aria-TSQ Quantum LC-MS/MS liquid chromatography tandem mass spectrometer (ThermoFisher Scientific). Metformin was extracted from plasma (100 µl) using a SLE+ plate (Biotage GB, Ystrad Mynach, UK) following enrichment with d6-metformin (200 ng) as an internal standard. Calibration standards ranged from 0.5 to 1000 ng of metformin. Analytes were eluted, reduced to dryness under nitrogen (40 °C) and reconstituted in water/acetonitrile (100 µl; 80 : 20 v/v). Chromatographic separation was achieved using an Aria CTC autosampler and Allegros pump on an ACE® Excel™ Super2C18 column (100 × 3 mm; 2 µm; HiChrom, Reading, UK) protected by a Kinetex KrudKatcher® (Phenomenex, Macclesfield, UK) and detected on a TSQ™ Quantum Discovery triple quadrupole mass spectroscopy (ThermoFisher Scientific) operated by selective reaction monitoring in positive electrospray ionisation mode (300 °C, 3 kV). The mobile phase was 0.1% formic acid in water (A), 0.1% formic acid in acetonitrile (B) at a flow rate of 0.2 ml/minute at 30 °C. Gradient elution was achieved by increasing the percentage of acetonitrile from 20% to 90% over a 5-minute run time. Metformin and its isotopically labelled internal standard eluted at 2.1 minutes. Transitions monitored for were m/z 130.1 → 60.1 and 71.1 and m/z 136.2 → 60.1 and 71.1 for metformin and internal standard, respectively. Linear regression analysis of calibration standards, calculated using peak area ratios of metformin to internal standard, was used to determine the concentration of metformin in the samples.
Statistical analysis
Data are expressed as mean ± standard error of the mean unless otherwise stated. Comparisons were made between groups using an unpaired Student’s t-test. HOMA-IR data were log-transformed to achieve a normal distribution and the results back transformed for reporting. Significance was set at p < 0.05. No adjustment was made for multiple comparisons.
Intravenous access failed in one subject and the procedure had to be abandoned. Hence, data were obtained from 20 of the 21 subjects who attended for a clamp study. Clamp studies were performed blind to treatment allocation, with unblinding revealing that final cohort numbers were as follows: placebo group, n = 11; metformin group, n = 9. All subjects were compliant with their study medication according to diary entries. One subject in the metformin group did not have detectable levels of metformin in her blood at 36 weeks’ gestation and her data were, therefore, excluded from the analysis. Hence the final sample size was 11 women in the placebo group and 8 women in the metformin group.
Results
Participant characteristics
Participant characteristics are shown in Table 11.
Characteristic | Placebo (n = 11) | Metformin (n = 8) |
---|---|---|
Age (years), mean (SD) | 29.6 (3.6) | 32.6 (3.7) |
Nulliparity, n (%) | 5 (45) | 3 (38) |
BMI at baseline (kg/m2), mean (SD) | 35.7 (3.5) | 38.5 (4.4) |
Body fat at time of clamp (%), mean (SD) | 46.2 (5.3) | 49.1 (3.9) |
Gestation at time of clamp (days), mean (SD) | 56.25 (4.8) | 57.55 (4.2) |
Effect of metformin on indices of insulin sensitivity for glucose metabolism at 36 weeks’ gestation
Mean plasma glucose and insulin concentrations achieved for the two groups are shown in Figures 3 and 4.
FIGURE 3.
Clamped plasma glucose.

FIGURE 4.
Clamped plasma insulin.

Whole-body glucose disposal (WGD) was calculated in mg of glucose per kg of FFM per minute at steady state during the high-dose clamp. WGD is an indirect measure of whole-body insulin sensitivity, with a greater glucose disposal rate implying greater insulin sensitivity. The M/I was also calculated to correct for slight differences in achieved plasma insulin in each group and expressed in units of mg of glucose per kgFFM per minute.
Glucose disposal per unit plasma insulin but not WGD was higher in the metformin group than in the placebo group (difference between means 0.02 mg/kgFFM/minute, 95% CI 0.001 to 0.03 mg/kgFFM/minute; p = 0.04; and 0.78 mg/kgFFM/minute/µIU/l, 95% CI –0.12 to 1.67 mg/kgFFM/minute/µIU/l; p = 0.08, respectively).
In contrast, HOMA-IR scores for these individuals at baseline were similar in the two groups (difference between means –0.87, 95% CI –3.31 to 1.57; p = 0.46).
In the absence of insulin, EGP (expressed as both milligrams of glucose per kilogram of total body weight per minute and as milligrams of glucose per kilogram of FFM per minute) was greater in the metformin group than in the placebo group (difference between means 0.54 mg/kgFFM/minute, 95% CI 0.08 to 1.00 mg/kgFFM/minute; p = 0.02) (Figure 5). During low-dose insulin infusion, EGP was again higher in the metformin group (difference between means 0.43 mg/kgFFM/minute, 95% CI 0.02 to 0.84 mg/kgFFM/minute; p = 0.04) (see Figure 5). There was no significant difference in the percentage suppression from basal EGP to EGP during low-dose hyperinsulinaemic–euglycaemic clamp between the two groups (difference between means 2.89%, 95% CI –7.65% to 13.42%; p = 0.57) (see Figure 5).
FIGURE 5.
Endogenous glucose production. *p ≤ 0.05.
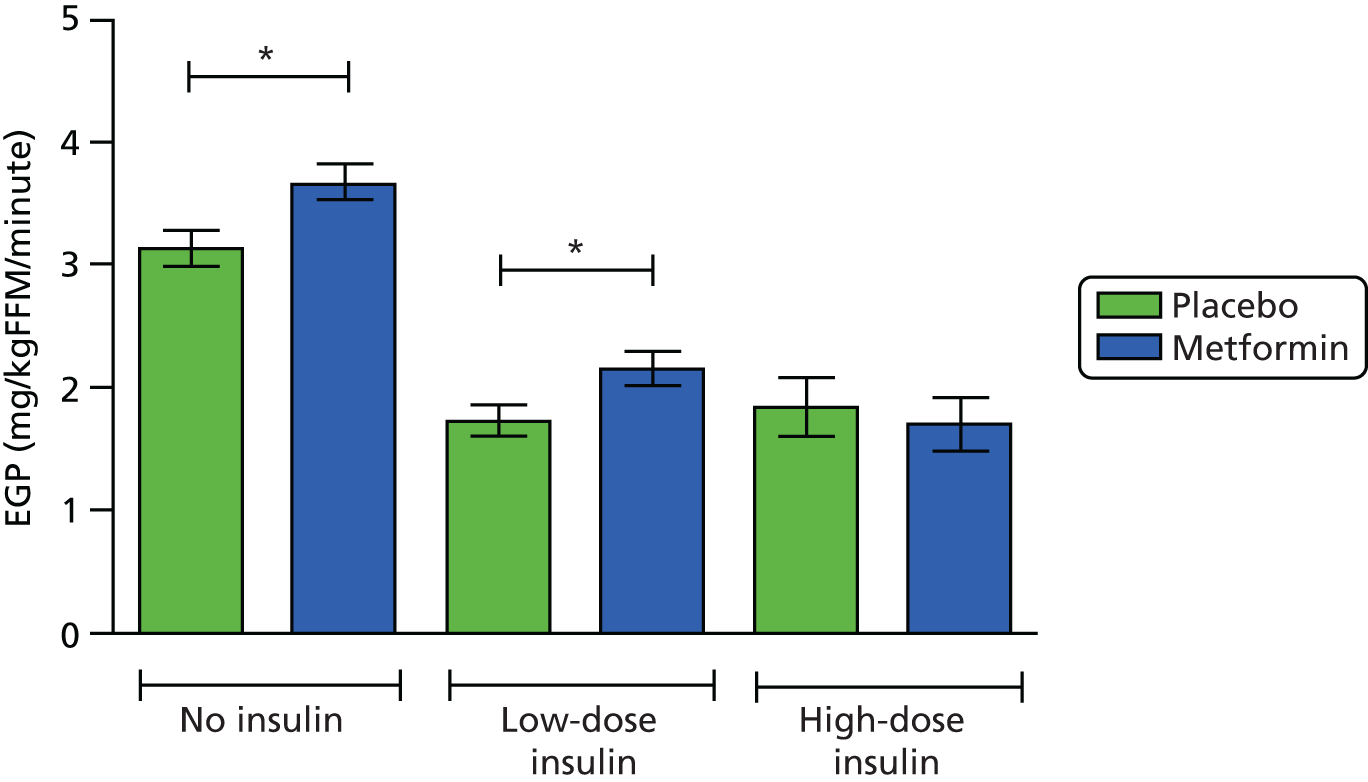
At baseline, prior to insulin infusion, the Rd of glucose and EGP should be equivalent as they are in steady state. The Rd was also significantly greater in the metformin group than the placebo group during the low-dose insulin phase of the clamp (difference between means 0.36 mg/kgFFM/minute, 95% CI 0.22 to 1.18 mg/kgFFM/minute; p = 0.07). At high-dose insulin infusion, Rd was increased further but there was no significant difference between the treatment groups (difference between means 0.35 mg/kgFFM/minute, 95% CI –0.79 to 1.50 mg/kgFFM/minute; p = 0.52) (Figure 6).
FIGURE 6.
Rate of disappearance of glucose. *p ≤ 0.05; **p ≤ 0.01.

Effect of metformin on insulin sensitivity for lipolysis at 36 weeks’ gestation
Mean plasma glycerol and NEFA concentrations achieved for the two groups are shown in Figures 7 and 8. Glycerol turnover per kgFFM is shown in Figure 9. There was no difference in glycerol turnover between the metformin and placebo groups (difference between means: no insulin 0.03 mg/kgFFM/minute, 95% CI –0.12 to 0.18 mg/kgFFM/minute; p = 0.67; low-dose insulin 0.02 mg/kgFFM/minute, 95% CI –0.06 to 0.10 mg/kgFFM/minute; p = 0.64; high-dose insulin –0.01 mg/kgFFM/minute, 95% CI –0.10 to 0.08 mg/kgFFM/minute; p = 0.87). Low-dose insulin infusion resulted in suppression of the Rd of glycerol in both groups; high-dose insulin resulted in no further suppression of Rd in either group. There was no difference in serum NEFAs between the groups at any time.
FIGURE 7.
Clamped plasma glycerol.

FIGURE 8.
Clamped plasma NEFAs.
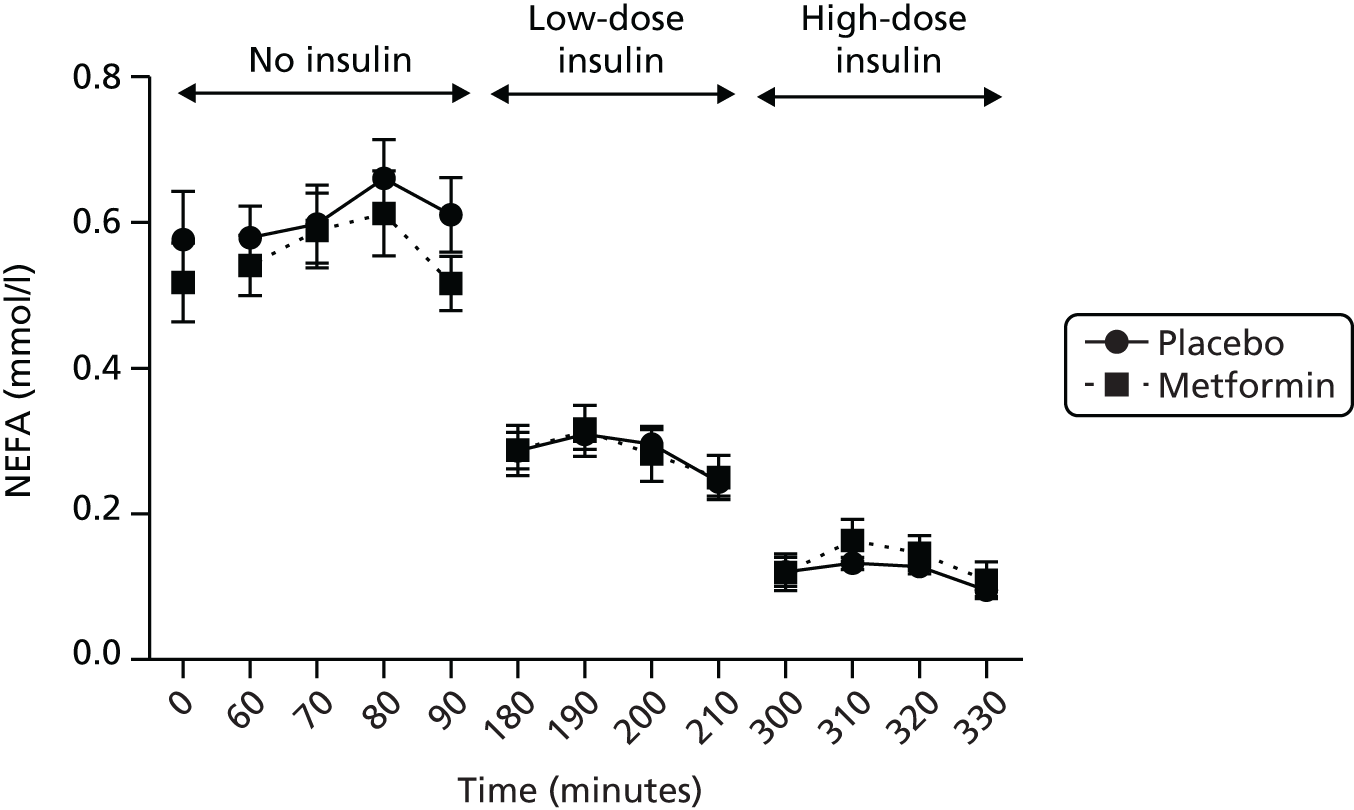
FIGURE 9.
Glycerol turnover.

Discussion
We hypothesised that administration of metformin to obese pregnant women would improve insulin sensitivity in the third trimester. These data show that, as expected, subjects taking metformin demonstrated a greater M/I, which is associated with increased insulin sensitivity. The Rd of glucose was enhanced in the metformin-treated group suggesting improved peripheral insulin sensitivity. However, EGP was higher in the metformin-treated subjects, suggesting that, if anything, those on metformin exhibited a reduced ability to suppress hepatic glucose production in response to insulin and enhanced glucose release on fasting. This is perhaps surprising given that metformin is thought to exert its action principally via the liver, inhibiting gluconeogenesis and reducing hepatic glucose production,54 although an effect mediated via peripheral glucose disposal has also been shown. 55,56 Additionally, metformin has recently been shown to stimulate EGP in healthy fasting individuals who are not pregnant. 57 Metformin’s mechanism of action in pregnant women has, to our knowledge, never been studied. These data suggest enhanced glucose flux in the metformin-treated women (i.e. higher liver production and higher peripheral disposal of glucose). Interpretation of this is perhaps complicated by the presence of the fetoplacental unit, which represents a significant proportion of the FFM at 36 weeks’ gestation and must account for uptake of some of the glucose. These data confirm that the hyperinsulinaemic–euglycaemic clamp is a more sensitive measure of insulin resistance as we demonstrated differences in M/I and EGP but not in HOMA-IR score.
The lipolytic pathway was equally sensitive to exogenous insulin in both the metformin- and the placebo-treated groups. Maximal suppression (around 70%) was achieved by low-dose insulin, with no further suppression at the high-dose infusion. Enhanced rates of lipolysis are thought to be important towards the end of normal pregnancy to provide maternal substrates for gluconeogenesis and triglyceride synthesis and spare glucose to facilitate normal fetal growth. Reduced third-trimester lipolysis is associated with fetal growth restriction58 and so these data are perhaps reassuring on the safety of metformin in pregnancy in terms of not increasing the risk of intrauterine growth restriction.
In conclusion, these substudy data confirm that metformin can improve insulin sensitivity in obese pregnant women. However, this may be offset by increased glucose flux and hence there is a lack of effect on fetal nutrition or growth. Additionally, metabolism at 36 weeks’ gestation may not reflect metabolism in mid-gestation, when differences between lean and obese women are greater.
Endothelial function
Introduction
Hypertensive disorders are more prevalent in obese populations59 including those who are pregnant. 60 Obese women are at greater risk of chronic hypertension, pregnancy-induced hypertension and pre-eclampsia:61–64 for a woman with a BMI of > 35 kg/m2, the risk of pre-eclampsia is twice that of a normal lean woman. 65 The causal mechanisms behind these associations are not clear but insulin resistance may play a role. Women with diabetes mellitus of all types during pregnancy have a heightened risk of pre-eclampsia and women with pre-eclampsia have an increased risk of type 2 diabetes mellitus in later life. 66 Other possible contributory mechanisms include endothelial dysfunction, inflammation, dyslipidaemia and oxidative stress, all of which are associated with both obesity and pre-eclampsia. 67–69
The vascular endothelium is the layer of endothelial cells between the blood vessel wall and the bloodstream. It is a key regulator of vascular homeostasis, acting not only as a barrier but also as an active signal transducer for circulating influences that modify the vessel wall tone and phenotype. 70 Endothelial dysfunction is characterised by a shift of the actions of the endothelium towards reduced vasodilatation and a more pro-inflammatory and pro-thrombotic state. 71 Mechanisms that participate in the reduced vasodilatory responses include reduced nitric oxide bioavailability and oxidative stress. 71 Endothelial dysfunction is associated with most forms of cardiovascular disease, such as hypertension, coronary artery disease, diabetes and chronic renal failure, and often precedes their clinical manifestations. 72 It has also been demonstrated in disease states in the absence of overt cardiovascular complications, such as the metabolic syndrome73 and obesity. 74
Endothelial dysfunction in pregnancy has been most widely studied in the context of pre-eclampsia, in which impaired maternal vascular function has been reported. 75 GDM is associated with increased oxidative stress and overexpression of inflammatory cytokines, both of which contribute to endothelial dysfunction. 76 Obesity in pregnancy shares these features and impaired endothelial function has been demonstrated in obese pregnant women. 67,77 Given that insulin resistance and hyperglycaemia are linked to inflammation, vascular dysfunction and hypertension, these processes are potential mediators for these upstream causative pathways, linking endothelial dysfunction with cardiovascular disease in obese pregnant women.
Metformin might be the ideal agent to address and reverse these abnormalities in obese pregnant women. In addition to its primary function as an insulin-sensitising agent, metformin has beneficial effects on the vascular endothelium, lipid profile and oxidative stress. 78,79 These effects appear to be independent of metformin’s glucose-lowering and insulin-sensitising effects. Metformin also improves vascular function in a variety of clinical syndromes associated with insulin resistance, for example reducing cardiovascular disease risk in patients with type 2 diabetes mellitus80 and improving endothelial function in patients with type 1 diabetes mellitus, metabolic syndrome and PCOS. 79,81–83
Nested within the EMPOWaR trial, this mechanistic substudy was designed to test the hypothesis that, compared with those taking placebo, participants treated with metformin would demonstrate improved endothelial function in late pregnancy.
Methods
We recruited a subset of women participating in the EMPOWaR trial. The characteristics of participants in the substudy were similar to the characteristics of those in the EMPOWaR trial overall. Importantly, those with GDM were excluded. We also included a comparator ‘control’ group of lean pregnant subjects who, with the exception of BMI, were matched for baseline characteristics to the EMPOWaR study population. Participants of the EMPOWaR trial were assessed at 12–16 weeks’ gestation following randomisation but prior to commencing study treatment and at around 36 weeks’ gestation while receiving trial medication. All measurements were performed blind to treatment allocation. Lean control subjects were assessed at both 12–16 and 36 weeks’ gestation.
Endothelial function
We assessed endothelial function by measuring FMD in the brachial artery. Subjects were asked to refrain from eating, smoking or consuming alcohol or caffeine in the preceding 4 hours. Measurements were obtained in a temperature-controlled room with the subject resting in a semi-recumbent position on a bed. Subjects in late pregnancy had a left lateral tilt applied to avoid aortocaval compression.
Measurements were made using ultrasound imaging (CX50 Ultrasound system with a 7-MHz linear array transducer; Philips Medical Systems, Guildford, UK) of the brachial artery, 2–5 cm above the antecubital fossa. A baseline rest image was acquired for a period of 60 seconds. Arterial occlusion was performed using a sphygmomanometric cuff applied below the antecubital fossa, inflated to suprasystolic pressure for 5 minutes and then released to induce hyperaemia. The brachial artery ultrasound was recorded for 30 seconds before and 5 minutes after cuff deflation. Images were acquired with electrocardiogram gating, with measurements made in end-diastole, corresponding to the onset of the R wave. To minimise movement, the scan probe was held in place with a probe-retaining device throughout the period of the study. Images were stored digitally and measurements made using edge-detection software [Vascular Research Tools 5; Medical Imaging Applications LLC, see www.mia-llc.com (accessed 24 June 2016)] (Figure 10).
FIGURE 10.
Representative images from analysis software.

The results were expressed as change in arterial diameter (D) divided by baseline diameter:
The peak diameter (Dpeak) was taken as the mean of the diameter measurements taken 5 seconds either side of the peak diameter. The baseline diameter (Dbaseline) was taken as the mean of the 60 seconds of baseline recording. We then measured endothelium-independent vasodilatation. After 10 minutes of rest, a second baseline image was obtained for 60 seconds and then a single low dose (25 µg) of sublingual nitroglycerin (GTN) spray was given and measurement recorded for 5 minutes. Again, the baseline diameter was taken as the mean of the first 60 seconds and the peak diameter was taken as the mean of the measurements 5 seconds either side of the peak.
Statistical analysis
Graphical data are presented as mean ± standard error of the mean. Comparisons between placebo, metformin and lean groups at both time points were made using one-way ANOVA. Two-way ANOVA was used to examine differences over time in the various treatment groups. Comparisons between early and late pregnancy within groups were made using Student’s unpaired t-tests. Significance was taken as a two-sided p-value of < 0.05.
Results
Forty-one eligible women in the EMPOWaR study agreed to participate in the substudy. However, the majority of EMPOWaR participants were unable or unwilling to attend for the two study visits: only one woman in the placebo group and two in the metformin group attended both visits. In contrast, all women in the lean control group attended both visits except for one lean subject who was ineligible at 36 weeks’ gestation because of a preterm birth. Images from nine subjects had to be discarded as they were of insufficient quality for analysis. The final study population (Table 12), therefore, included 28 subjects (n = 6 placebo, n = 12 metformin and n = 10 lean) at 12–16 weeks’ gestation and 26 subjects (n = 8 placebo, n = 9 metformin and n = 9 lean) at 36 weeks’ gestation. Data are presented for 28 subjects at baseline and 25 subjects at 36 weeks’ gestation.
Characteristics | Placebo (n = 13) | Metformin (n = 19) | Lean (n = 10) |
---|---|---|---|
Age (years), mean (SD) | 31.5 (4.4) | 27.3 (6.1) | 35.6 (4.1) |
Nulliparity, % | 46.7 | 68.8 | 70 |
Current smoking, % | 6.7 | 18.8 | 0 |
BMI baseline (kg/m2), mean (SD) | 38.4 (4.9) | 37.7 (5.7) | 23.05 (3.5) |
SBP baseline (mmHg), mean (SD) | 121.7 (10.8) | 117.3 (9.7) | 109.9 (15.4) |
DBP baseline (mmHg), mean (SD) | 69.1 (7.3) | 69.1 (9.5) | 65.9 (10.3) |
SBP late pregnancy (mmHg), mean (SD) | 123.2 (9.1) | 118.0 (15.3) | 123.8 (11.0) |
DBP late pregnancy (mmHg), mean (SD) | 75.0 (6.0) | 70.4 (8.5) | 73.8 (9.6) |
Mean gestation at time of study (days), mean (SD) | |||
Baseline | 100.2 (10.5) | 105.2 (7.7) | 100.3 (7.7) |
Late pregnancy | 252.1 (4.7) | 253.3 (6.8) | 257.0 (5.6) |
FMD, % [n] (SD) | |||
Baseline | 10.16 [6] (5.0) | 8.58 [12] (6.9) | 10.22 [10] (10.6) |
Late pregnancy | 6.08 [8] (5.3) | 5.10 [9] (4.0) | 5.40 [9] (3.0) |
GTN-mediated dilatation, % [n] (SD) | |||
Baseline | 12.74 [6] (9.7) | 9.07 [12] (5.6) | 11.98 [10] (9.0) |
Late pregnancy | 11.04 [8] (8.1) | 9.61 [8]a (4.4) | 8.50 [9] (4.0) |
There were no differences in FMD between the placebo, metformin and lean groups at baseline or at 36 weeks’ gestation (one-way ANOVA: baseline, p = 0.88; 36 weeks, p = 0.89) (Figure 11; see also Table 12). There was a decline in endothelial function in late pregnancy compared with early pregnancy across all groups (two-way ANOVA p = 0.03). There were no differences in endothelium-independent vasodilatation between groups or within groups in early and late pregnancy (see Table 12 and Figure 11).
FIGURE 11.
(a) FMD and (b) GTN-mediated dilatation. *p ≤ 0.05.
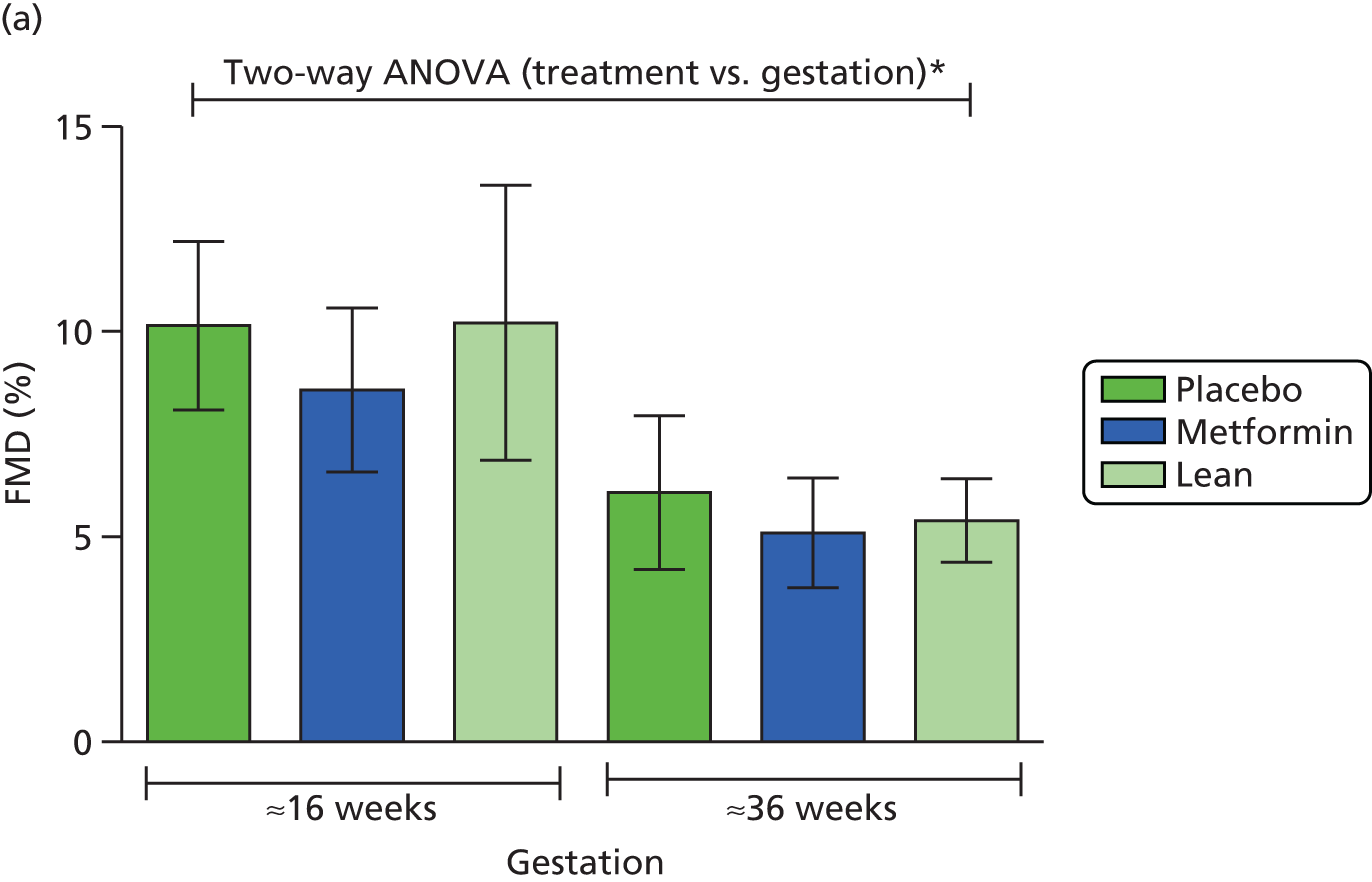

Discussion
We have used flow-mediated vasodilatation, the gold-standard non-invasive method of assessing endothelial function, in obese and lean pregnant women as part of the EMPOWaR study. Although we demonstrated a selective gestation-associated decline in endothelium-dependent vasodilatation, we saw no differences in endothelial function across obese and lean groups, nor any effect of the treatment intervention metformin. This suggests that pregnancy is associated with altered endothelial function and this is independent of body weight or glucose sensitivity.
There was a decline in endothelial function between early and late pregnancy in participants in all three groups. This is contrary to the finding of one longitudinal study of endothelial function in eight normal-weight women that demonstrated increasing FMD across the three trimesters of pregnancy. 84 However, a much larger study of 157 normal-weight pregnant women demonstrated an increase in FMD in pregnant compared with non-pregnant subjects, apparent from 10 weeks’ gestation, but a progressive decline in FMD to pre-pregnancy levels from around 30 weeks’ gestation, in keeping with our data in both lean and obese participants. 85
To our knowledge, this is the first study of the effect of metformin on endothelial function in pregnant women. It is not clear why metformin does not appear to have had an effect on the vascular endothelium in the study population when this has been demonstrated in other insulin-resistant populations. Pregnancy is associated with major vascular and haemodynamic changes, the primary event probably being a fall in peripheral vascular resistance. 86 This is most likely mediated by endothelium-dependent factors including nitric oxide synthesis upregulation by oestradiol and possibly vasodilatory prostaglandins. 87–89 The consequent fall in systemic vascular resistance leads to a compensatory increase in cardiac output. Larger vessels dilate less than smaller ones. 90 It is possible that the increased vessel diameter of pregnancy, along with stimulated nitric oxide activity of pregnancy, may obscure any effect of metformin on the endothelium. However, we did not see any differences in endothelium-independent vasodilatation, which we would expect to see if this was purely a vessel size effect or related to increased nitric oxide consumption.
Flow-mediated dilatation of the brachial artery is accepted as the gold standard for the non-invasive measurement of endothelial function as it is widely used, well tolerated, low risk and, importantly for our study population, suitable for use in pregnant women. There are, however, some limitations. Most notably for our study population, measurement of the vessel diameter can be technically challenging, particularly in the obese in whom visualisation of the intima is difficult as the ultrasound signal is attenuated by subcutaneous fat. Other limitations include the small sample size, the lack of longitudinal data for subjects other than those in the lean group and the variability of the baseline demographics (e.g. smoking status and parity) within the unblinded groups. This makes it difficult to identify differences within the small sample size.
In the larger EMPOWaR cohort as a whole, we saw no differences in blood pressure or the incidence of hypertensive disorders of pregnancy, which we may have expected if the placebo group had impaired endothelial function compared with the metformin group. This suggests that our finding of no difference between the two groups may be correct rather than a type 2 error. In conclusion, we have not demonstrated any effect on endothelial function of metformin in obese pregnant women.
Magnetic resonance imaging assessment of maternal and fetal adipose distribution
Introduction
For many years now it has been recognised that the site rather than the total quantity of body fat is an important determinant of insulin sensitivity and morbidity associated with obesity. 91–94 More recently, it has been recognised that deposition of lipid in ‘ectopic’ sites, namely the liver and skeletal muscle, is a major contributor to the development of insulin resistance. 95,96 It remains unclear whether or not increasing adiposity causes the deterioration in insulin sensitivity or vice versa. The ‘portal hypothesis’ of obesity suggests that an increase in central abdominal fat leads to elevated delivery of free fatty acids and inflammatory cytokines to the liver and that, consequently, hepatic insulin resistance develops and drives glucose upwards. 97 The ‘spillover hypothesis’ suggests that, in the context of obesity, the subcutaneous compartment becomes saturated and leads to the accumulation of visceral fat and deposition of lipid in ectopic sites such as the liver and muscle. 98 Clearly, the two hypotheses are not mutually exclusive and it is likely that both are contributory mechanisms. Regardless of the exact cause, there is no doubt that excess lipid accumulation is associated with impaired insulin sensitivity and morbidity such as type 2 diabetes mellitus.
Fat distribution in normal and obese pregnancy is less well studied and the contribution it pays to maternal and fetal outcomes and longer-term health is not clear. However, gestational weight gain is one of the most important predictors of post-partum weight retention99 and thus contributes significantly to the obesity epidemic among young women. 100 Gestational weight gain is highly variable but in lean women the contribution from fat tends to be predominantly in the subcutaneous compartments, largely the trunk and thighs. 101,102 Obese women actually tend to gain less weight than lean women during pregnancy but the fat mass that they do gain tends to be more central and therefore potentially more metabolically harmful. 103
Gestational weight gain and pre-existing obesity also impact on fetal growth. The conventional strategy for measurement of fetal growth is typically by ultrasound. Estimation of fetal weight is based on measurement of the fetal head circumference, femur length and abdominal circumference, with the abdominal circumference being the most individually sensitive predictor of fetal macrosomia. 104,105 The abdominal circumference is predominantly affected by the size of the fetal liver and is positively correlated with hepatic glycogen stores, which increase towards term. 106,107
Glucose is the major substrate that determines fat accumulation in the fetus, with the greater the glucose supply the greater the deposition of fat. 108 There is a linear association between maternal glucose tolerance and neonatal adiposity at birth,109 and a strong positive correlation between degree of maternal insulin resistance and neonatal fat mass at birth. 10 Increasing maternal BMI has also been shown to be associated with increased intrahepatocellular lipid in the newborn, assessed by magnetic resonance proton spectroscopy. 110
Magnetic resonance imaging has been used for many years in pregnant women with no apparent adverse effects on the mother or developing fetus. 111,112 It enables the quantification of the different maternal fat depots, in contrast to other techniques that can measure only total body fat mass (e.g. ADP; see Maternal and neonatal body composition). This substudy also provided the opportunity to develop scanning protocols to assess the body composition of the fetus in utero.
The aim of this mechanistic substudy was to examine the effect of metformin on maternal and fetal fat deposition in the early and late third trimester. We hypothesised that improving insulin sensitivity with metformin would result in less deposition of fat in the more insulin-sensitive sites (i.e. visceral, hepatic and skeletal muscle) and potentially protect the fetus from accumulation of excess fat.
Methods
Participants in the EMPOWaR study were invited to undergo a MRI scan at 28 and 36 weeks’ gestation. We aimed to recruit 40 participants to have a scan at both time points. Whole-body MRI and 1H-MRS studies were performed on a Siemens MAGNETOM® Verio 3 Tesla MRI system (Siemens AG, Healthcare Sector, Erlangen, Germany). Participants were positioned in the magnet in a full left lateral position to avoid aortocaval compression. Data from the abdomen and thigh were acquired using a combination of spine and body matrix coils elements. Aural protection was provided by use of earplugs and headphones. Contact between the participant and scanning staff was maintained at all times. Heart rate and oxygen saturation were monitored continuously throughout the scan period; blood pressure was measured at the start of the scan and every 10 minutes throughout the procedure.
Scan sequences
Standard localising images were acquired to confirm organ and fetal position.
1H-magnetic resonance spectroscopy
For 1H-MRS measurement of intramyocellular and intrahepatocellular lipid, single-voxel spectra localised to the right quadriceps muscle and the right lobe of the liver were acquired using a point-resolved spectroscopy sequence (repetition time 5000 milliseconds/echo time 30 milliseconds) with and without water suppression and with eight signal averages. The voxel size was 2 cm3 in the muscle and 3 cm3 in the liver. The voxel site was chosen to avoid large blood vessels or subcutaneous adipose tissue. Lipid concentration was calculated from the water-suppressed acquisition using the spectroscopy analysis tool jMRUI (MRUI Consortium, Brno, Czech Republic).
In- and out-of-phase imaging (Dixon method)
In addition to MRS, the Dixon method113–115 was used for calculation of hepatic and skeletal muscle fat fraction in the participant and also fetal hepatic fraction. The lipid signal was calculated by subtraction of in- and out-of-phase images (2.46 milliseconds and 8.61 milliseconds) and T2* decay during this time, corrected using the two in-phase images (2.46 milliseconds and 4.92 milliseconds) according to protocols that are well established for use in the adult liver. 113–115 These protocols are not well established in fetal imaging and method development was required during the study to optimise this technique for the fetal measurements.
Assessment of maternal abdominal adipose tissue
To quantify maternal subcutaneous and visceral fat, a three-dimensional T1-weighted volumetric interpolated breath-hold examination sequence was acquired axially through the liver. Lipid signals were defined using a semiquantitative thresholding technique using the commercial software SliceOmatic™ (TomoVision, Magog, QC, Canada).
Adipose tissue appears bright on T1-weighted images. Regions of interest with attenuation above an investigator-defined threshold were coloured to define visceral adipose tissue and subcutaneous adipose tissue (Figure 12). The areas of the coloured regions were extracted, converted into a unit of volume (mm3) and expressed as a percentage of the total abdominal volume of the region being examined. Volumes were calculated using the extracted areas of the regions of interest (mm2) multiplied by the width of each slice (2 mm).
FIGURE 12.
Maternal adipose tissue images. (a) Uncoloured and (b) coloured axial slices to show subcutaneous (green) and visceral (red) fat distribution.
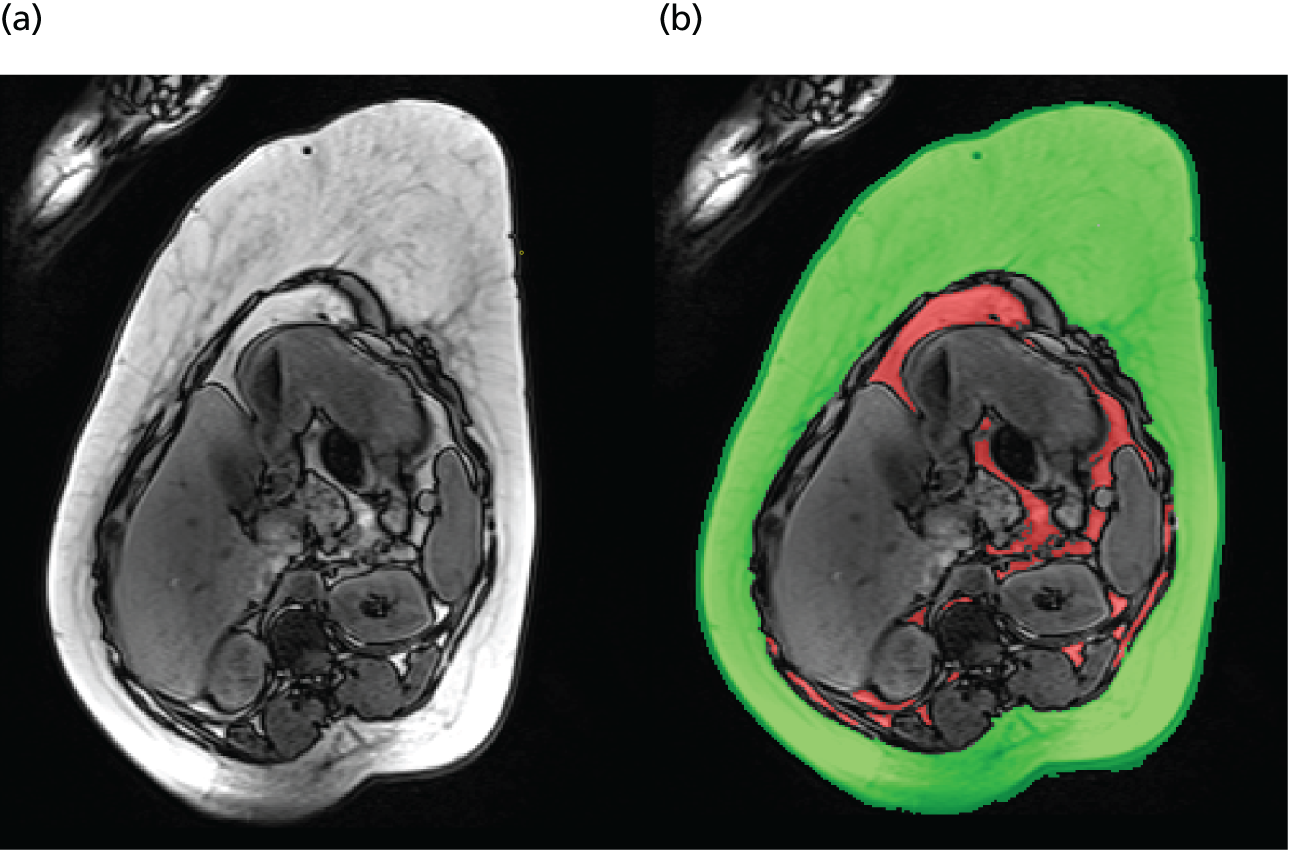
A multislice approach was used. The left renal pelvis was identified in all subjects and adipose tissue was measured in 20 2-mm slices cranial to this level.
Estimation of fetal liver volume
A T2 half-Fourier acquisition single-shot turbo spin-echo sequence was acquired of the fetus to cover the fetal liver in the axial, sagittal and coronal planes (dependent on degree of fetal movement during the acquisition period).
The fetal liver is identifiable by the investigator on these images using standard anatomical landmarks. The entire liver was coloured as the area of interest on every slice in which it was visible using the same software as was used for the maternal fat measurements (Figure 13). The area of the coloured region was extracted and converted into a unit of volume (mm3) by multiplying the area (mm2) by the width of each slice (2 mm).
FIGURE 13.
Fetal liver images. Uncoloured (a and c) and coloured (b and d) axial (a and b) and sagittal (c and d) slices to show the fetal liver.

Estimation of fetal hepatic fat and fetal subcutaneous fat
A T1-weighted fast low-angle shot (FLASH) sequence was acquired for the in- and out-of-phase fetal liver fat fraction. The slice thickness was 8 mm. For fetal subcutaneous fat, a fat excitation FLASH sequence was used, again with an 8-mm slice width. Shoulder-to-shoulder coverage of the fetus was obtained in the sagittal plane and a single slice at the level of the umbilical cord insertion was used in the axial plane. The subcutaneous fat was coloured on every available slice in the sagittal plane (Figure 14) and on the single slice in the axial plane using the same technique as described for the maternal subcutaneous and visceral fat and fetal liver volume. The amount of fat was expressed as a percentage of the total volume of the area examined. These protocols were subject to method development during the study process.
FIGURE 14.
Fetal subcutaneous fat images. (a) Uncoloured and (b) coloured sagittal slices through the fetus to show the subcutaneous fat.

Statistical analysis
Comparisons between groups were made using unpaired t-tests or Mann–Whitney tests when data were not normally distributed. Comparisons within groups were made using paired t-test or Wilcoxon tests when data were not normally distributed. The Kruskal–Wallis test was used to compare differences between the groups over the two time points. Significance was set at p < 0.05.
Reproducibility
The intra- and interobserver variability for measurement of maternal abdominal adipose tissue using the same method as in this study has previously been validated and been found to be highly correlated. 116 Intraobserver variability for measurements of fetal liver volume and fetal subcutaneous fat was assessed by the same observer (CC) defining the region of interest on all relevant slices from five subjects on two separate occasions. Interobserver variability was assessed by comparison of data from all relevant slices for five subjects for two independent observers (CC and SS).
For each paired data set, a correlative plot of the data sets around the line of equality is presented. Agreement was assessed by construction of Bland–Altman plots with 95% limits of agreement as follows: upper 95% limit of agreement = mean difference + 2 SDs; lower 95% limit of agreement = mean difference – 2 SDs.
Results
The demographic characteristics of the participants are provided in Table 13. In total, 37 participants (n = 18 and n = 19 in the placebo and metformin groups, respectively) underwent MRI and 1H-MRS studies at both 28 and 36 weeks’ gestation. A further 10 participants (n = 6 and n = 4 in the placebo and metformin groups, respectively) underwent MRI at 28 weeks’ gestation only and 10 participants (n = 6 and n = 4 in the placebo and metformin groups, respectively) underwent MRI at 36 weeks’ gestation only.
Characteristic | Placebo (n = 30) | Metformin (n = 27) |
---|---|---|
Maternal | ||
Age (years), mean (SD) | 29.4 (4.5) | 30.1 (5.5) |
Nulliparity, n (%) | 11 (36.7) | 11 (40.7) |
BMI at baseline (kg/m2), mean (SD) | 38.2 (5.6) | 39.4 (4.7) |
Neonatal | ||
Male sex, n (%) | 14 (46.7) | 11 (40.7) |
Birthweight (g), mean (SD) | 3493.0 (512.4) | 3596.1 (494.7) |
Birthweight centile, mean (SD) | 51.7 (29.6) | 63.4 (25.8) |
Ponderal index at birth, mean (SD) | 3.44 (4.6) | 2.60 (0.32) |
Fat (%), mean (SD) | 12.53 (5.7) | 12.63 (4.3) |
Tricep skinfold at birth (mm), mean (SD) | 11.38 (18.1) | 8.30 (2.5) |
Subscapular skinfold at birth (mm), mean (SD) | 10.06 (13.9) | 7.11 (2.3) |
Reproducibility
Fetal liver volume
Following repeated measurements by the same investigator (M1 and M2; intrarater) and of the same images by two investigators (CC and SS; inter-rater), measurements of fetal liver volume were found to be well correlated in both the sagittal (Figures 15–18) and axial (Figures 19–22) planes, with the majority of points scattered evenly around the line of no difference and within the upper and lower 95% limits of agreement. Measurement in the axial plane showed the best reproducibility. This may be because there is less rotation of the fetus on this axis and more reliable images were obtained.
FIGURE 15.
Sagittal fetal liver volume intrarater reproducibility. (a) Observer 1; and (b) observer 2. M1, measurement 1; M2, measurement 2, in reference to repeated measures by the same observer.


FIGURE 16.
Sagittal fetal liver volume intrarater reproducibility: Bland–Altman analysis. (a) Observer 1. n = 93 pairs, mean difference (lower to upper 95% limits of agreement) –1015 (–3228 to 1198) mm3; and (b) observer 2. n = 99 pairs, mean difference (lower to upper 95% limits of agreement) –495 (–3003 to 2014) mm3.
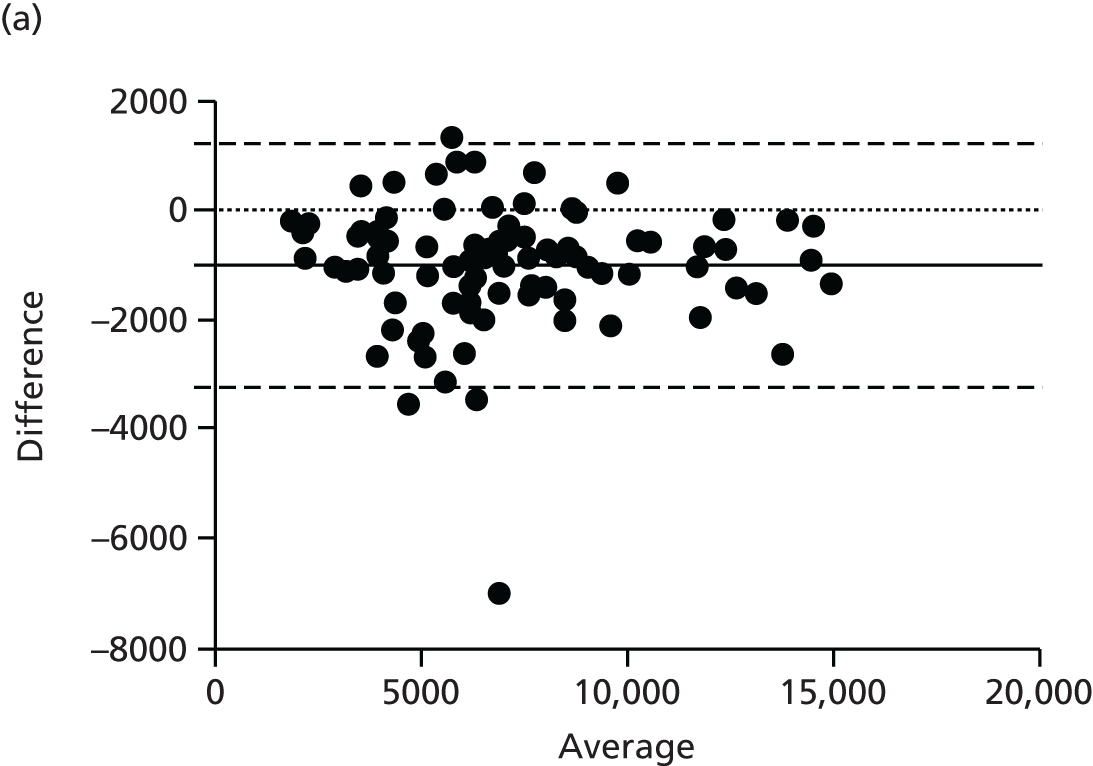

FIGURE 17.
Sagittal fetal liver volume inter-rater reproducibility. CC, observer 1; SS, observer 2.
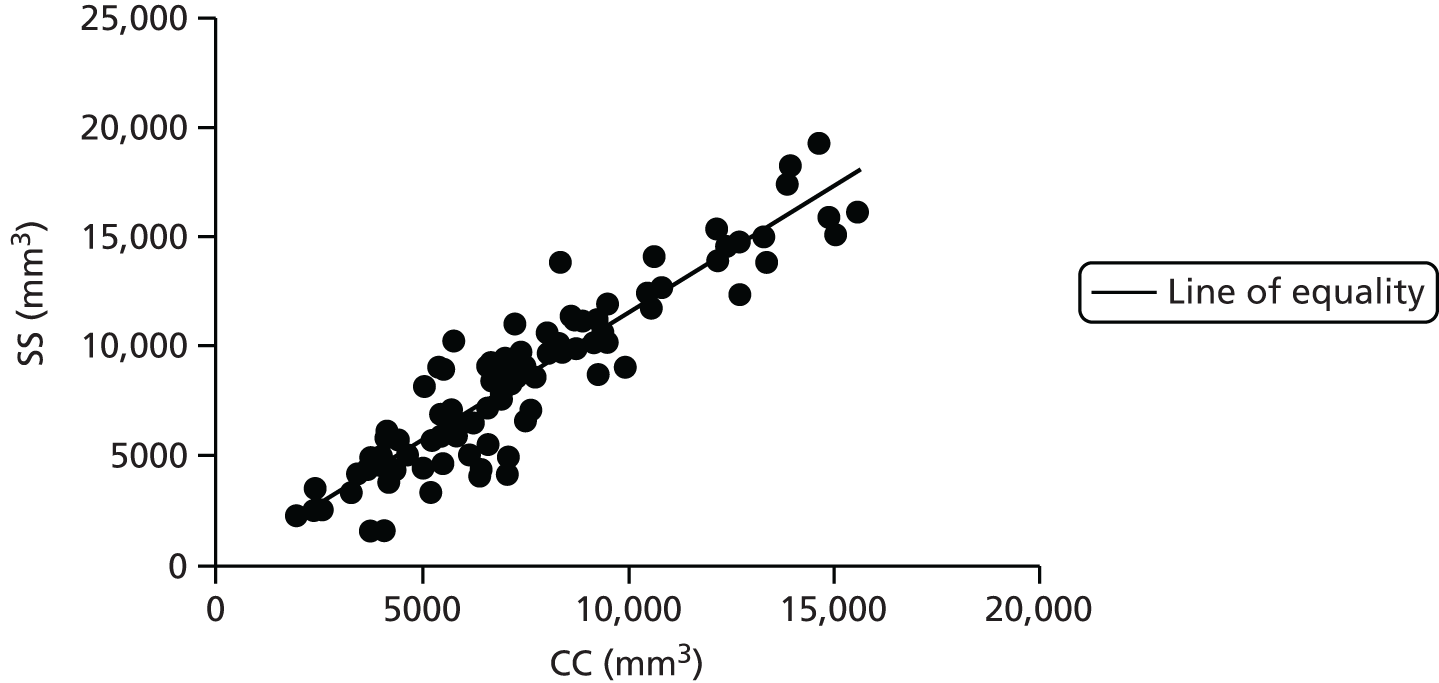
FIGURE 18.
Sagittal fetal liver volume inter-rater reproducibility: Bland–Altman analysis. n = 96 pairs, mean difference (lower to upper 95% limits of agreement) –1110 (4284 to 2065) mm3.

FIGURE 19.
Axial fetal liver volume intrarater reproducibility. (a) Observer 1; and (b) observer 2. M1, measurement 1; M2 measurement 2, in reference to repeated measures by the same observer.


FIGURE 20.
Axial fetal liver volume intrarater reproducibility: Bland–Altman analysis. (a) Oberver 1: n = 71 pairs, mean difference (lower to upper 95% limits of agreement) –2965 (–8335 to 2404) mm3; and (b) observer 2: n = 80 pairs, mean difference (lower to upper 95% limits of agreement) –853 (–8575 to 6869) mm3.
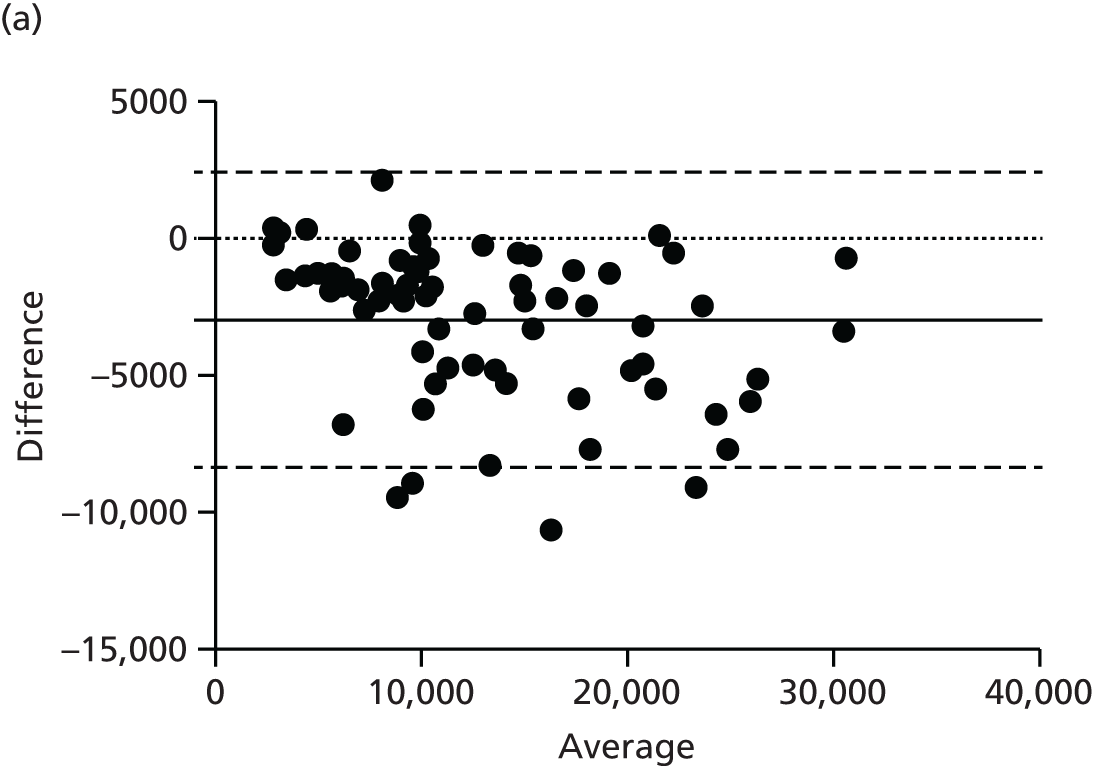
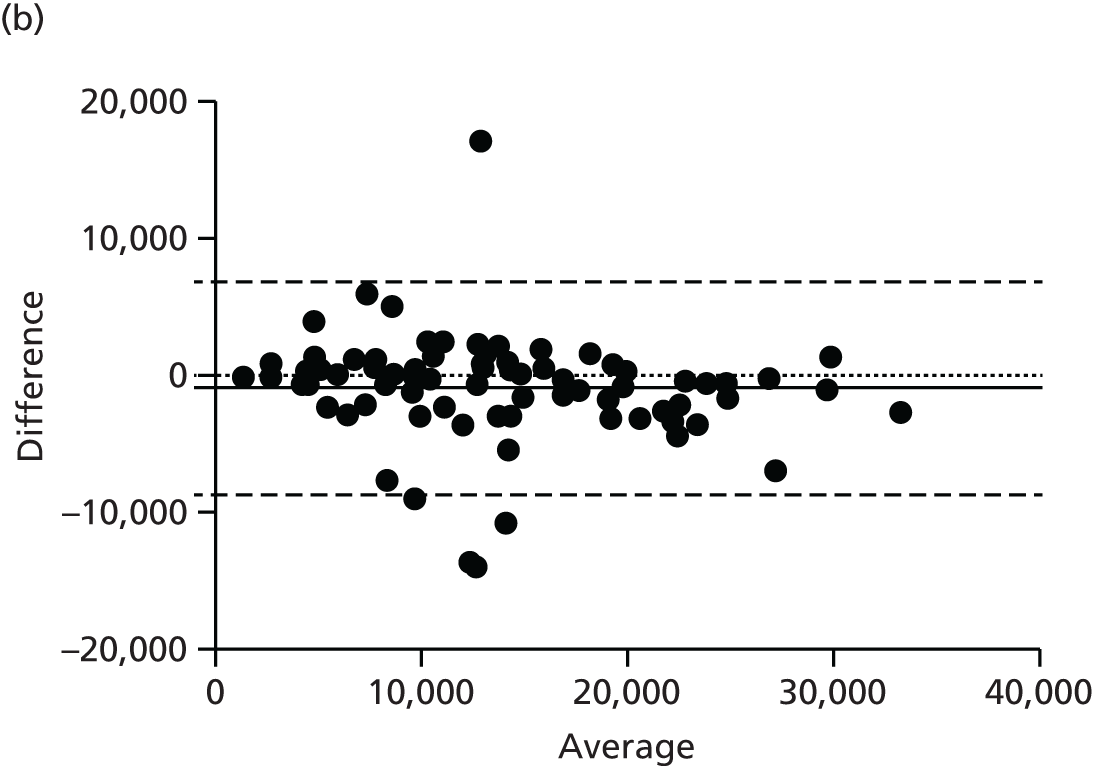
FIGURE 21.
Axial fetal liver volume inter-rater reproducibility. CC, observer 1; SS, observer 2: n = 93 pairs, mean difference (lower to upper 95% limits of agreement) –1015 (–3228 to 1198) mm3.

FIGURE 22.
Axial fetal liver volume inter-rater reproducibility: Bland Altman analysis. n = 77 pairs, mean difference (lower-upper 95% limits of agreement) –92.84 (–8255 to 8069) mm3.

Fetal subcutaneous fat, intrarater variability
Repeated measures by the same investigator were performed. Repeated measures for this parameter were less highly correlated, as demonstrated by a wider scatter of points around the line of equality (Figure 23) and between the 95% limits of agreement (Figure 24). This is likely to reflect both the smaller number of suitable images for analysis of fetal subcutaneous fat and the fact that this was a novel technique that required significant method development during the study period.
FIGURE 23.
Fetal subcutaneous fat intrarater reproducibility. M1, measurement 1; M2, measurement 2, in reference to repeated measures by the same observer.

FIGURE 24.
Fetal subcutaneous fat intrarater reproducibility: Bland–Altman analysis. n = 67 pairs, mean difference (lower to upper 95% limits of agreement) 1530 (–5630 to 8691) mm3.
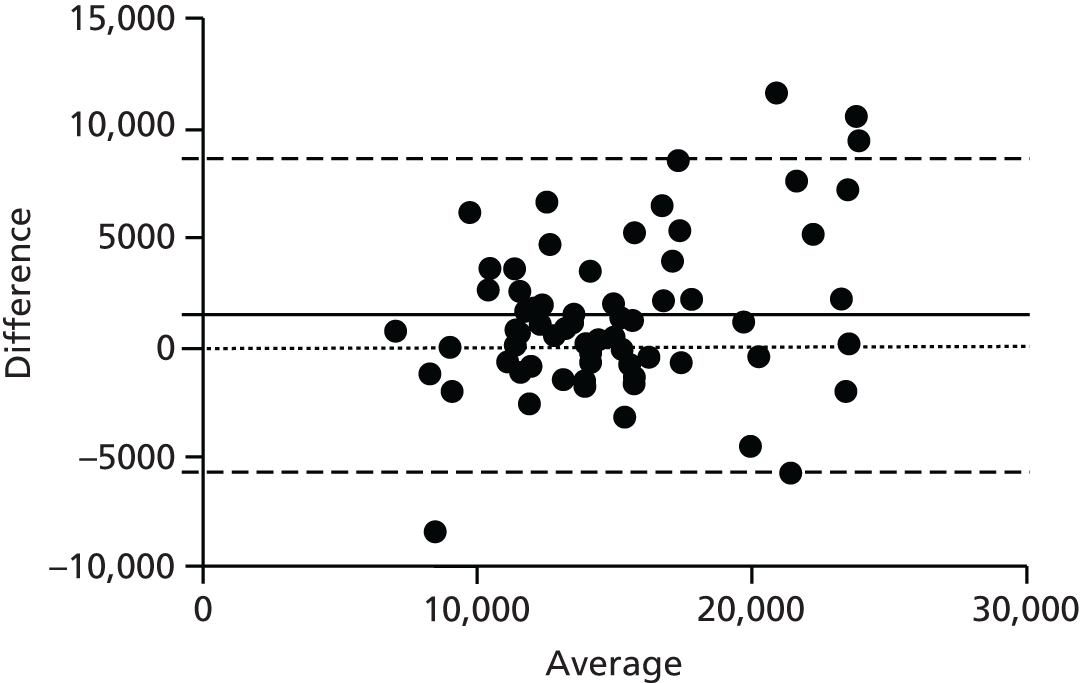
Maternal subcutaneous and visceral fat masses
There was no difference in the subcutaneous fat mass (expressed as a percentage of the abdominal volume examined) between the placebo group and the metformin group at 28 weeks’ gestation (difference between means –3.45%, 95% CI –7.63% to 0.73%; p = 0.10) or at 36 weeks’ gestation (difference between means –3.43%, 95% CI –7.63% to 0.76%; p = 0.11). There was no difference in visceral fat mass (expressed as a percentage of the abdominal volume examined) at 28 weeks’ gestation (difference between means –0.02%, 95% CI –1.93% to 1.89%; p = 0.98) or at 36 weeks’ gestation (difference between means 0.18%, 95% CI –2.17% to 1.82%; p = 0.86) (Figure 25).
FIGURE 25.
Maternal subcutaneous (SC) and visceral (V) fat mass at 28 and 36 weeks’ gestation. **p ≤ 0.01, ***p ≤ 0.001.
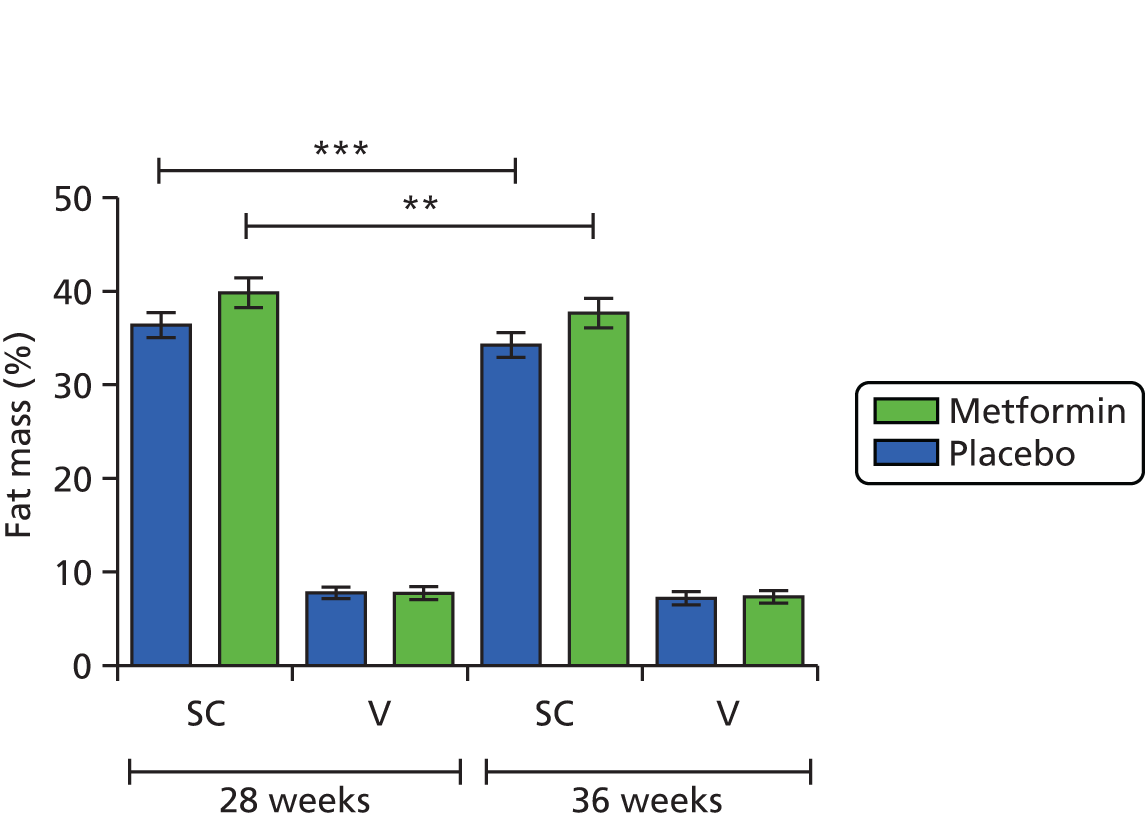
Both groups demonstrated a significant loss of subcutaneous fat mass between 28 and 36 weeks’ gestation (paired t-test: placebo difference between means –2.14, 95% CI –3.27% to –1.01%; p = 0.0009; metformin difference between means –2.15, 95% CI –3.38% to –0.923%; p = 0.0018). There was no significant difference in the percentage change in subcutaneous fat mass from 28 to 36 weeks’ gestation between the placebo group and the metformin group (difference between means –0.45, 95% CI –4.71% to 3.82%; p = 0.83) (Figure 26).
FIGURE 26.
Percentage change in (a) subcutaneous and (b) visceral fat mass between 28 and 36 weeks’ gestation.

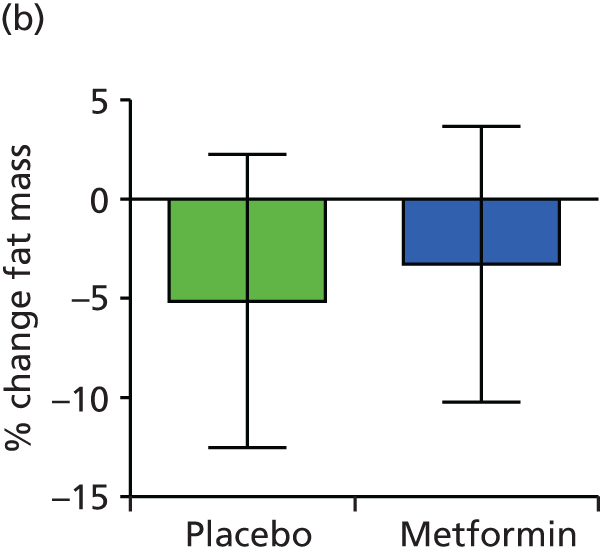
Neither group demonstrated any change in visceral fat mass between 28 and 36 weeks’ gestation and thus there was no difference in change in visceral fat mass between 28 and 36 weeks’ gestation between the groups (Mann–Whitney test, p = 0.60) (see Figure 26).
Maternal skeletal muscle and hepatic fat fraction
Dixon method
The mean (SD) hepatic fat fraction (%) at 28 weeks’ gestation was 3.93 (1.35) and 4.90 (2.76) in the placebo and metformin groups, respectively. At 36 weeks’ gestation the equivalent figures were 5.16 (2.78) and 5.00 (2.54).
The mean (SD) skeletal muscle fat fraction (%) at 28 weeks’ gestation was 2.84 (0.90) and 3.20 (1.33) in the placebo and metformin groups, respectively. At 36 weeks’ gestation the equivalent figures were 3.43 (1.29) and 3.62 (1.82).
There were no significant differences in fat fraction measured by the Dixon method by gestation or treatment group in either the skeletal muscle (p = 0.55) or the liver (p = 0.50) (Figure 27).
FIGURE 27.
Maternal (a) hepatic and (b) skeletal muscle fat fraction measured by the Dixon method.
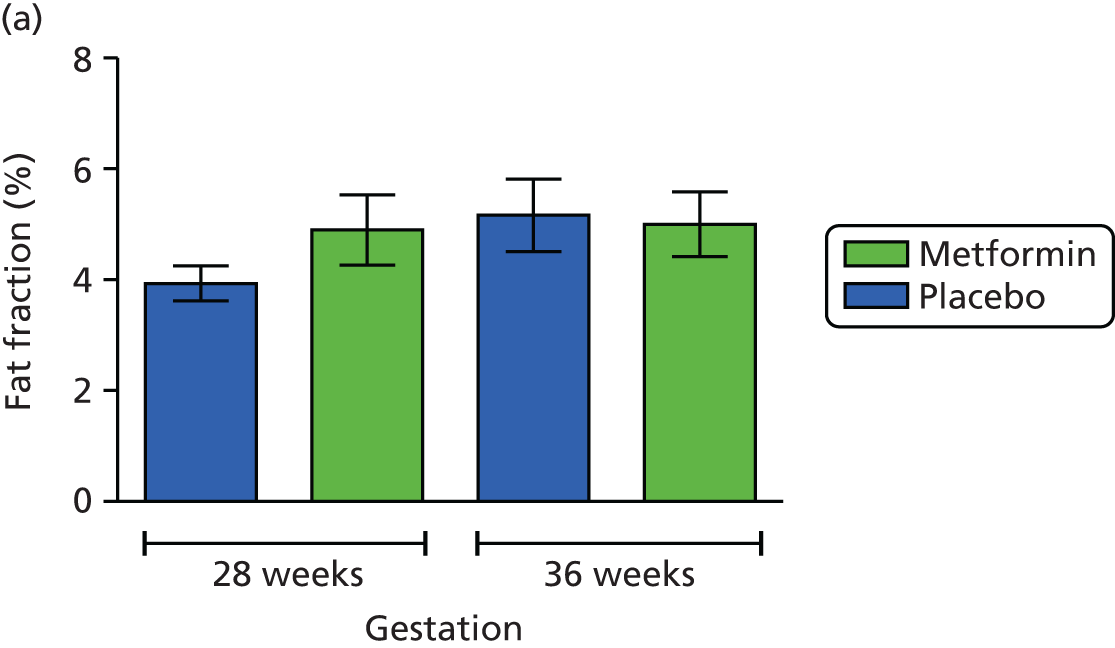

1H-magnetic resonance spectroscopy method
The mean (SD) hepatic fat fraction (%) at 28 weeks’ gestation was 1.24 (1.26) and 0.87 (1.22) in the placebo and metformin groups, respectively. At 36 weeks’ gestation the equivalent figures were 1.11 (1.80) and 0.86 (0.71).
The mean (SD) skeletal muscle fat fraction (%) at 28 weeks’ gestation was 7.13 (3.77) and 7.55 (4.86) in the placebo and metformin groups, respectively. At 36 weeks’ gestation the equivalent figures were 10.99 (9.60) and 9.10 (4.46).
There were no significant differences in fat fraction measured by 1H-MRS by gestation or treatment group in either the skeletal muscle (p = 0.64) or the liver (p = 0.42) (Figure 28).
FIGURE 28.
Maternal (a) hepatic and (b) skeletal muscle fat fraction measured by 1H-MRS.
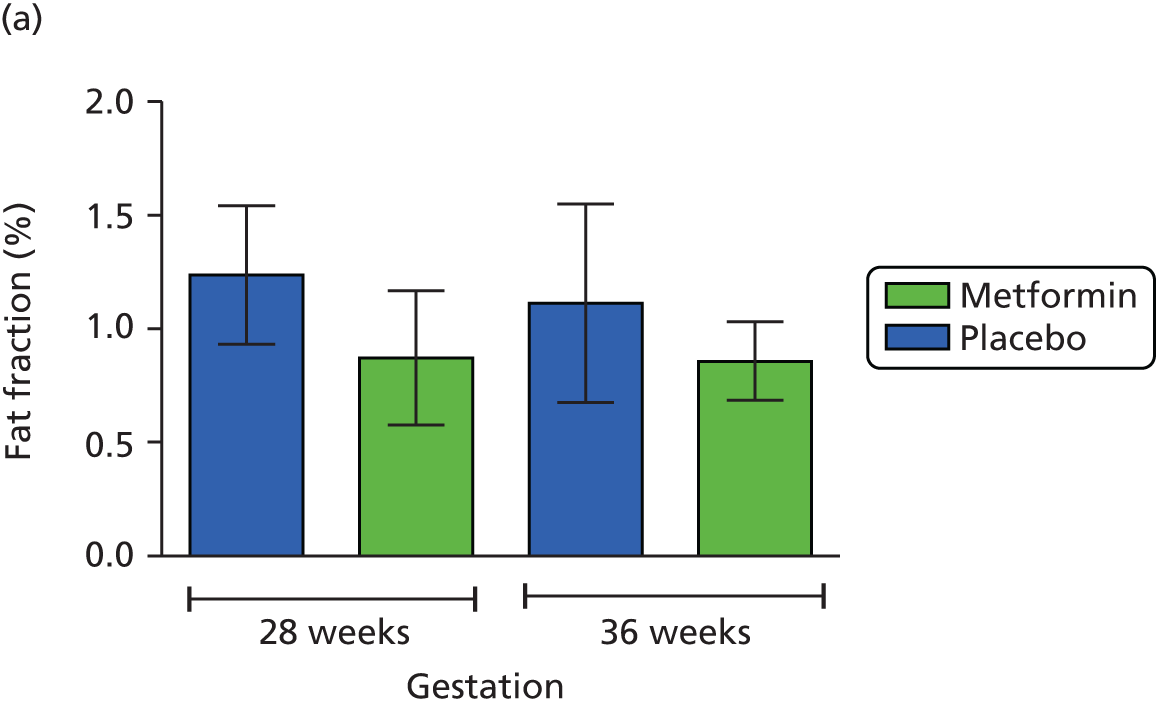

Fetal liver volume, hepatic fat fraction and subcutaneous fat
There was no statistically significant difference in fetal liver volume at 28 or 36 weeks’ gestation between the placebo group and the metformin group. Both groups demonstrated a significant increase in liver volume over time (p < 0.0001), as would be expected, but the percentage increase was not significantly different between the two groups when measured in either plane (Figures 29 and 30). There was no change in the fetal hepatic fat fraction by gestation or treatment group (Figure 31). There was no difference in subcutaneous fat, measured in the sagittal and axial planes (expressed as a percentage of body volume), at 36 weeks’ gestation between the two treatment groups (Figure 32).
FIGURE 29.
Fetal liver volume: axial plane. Placebo: 28 weeks, n = 25; 36 weeks, n = 22; metformin: 28 weeks, n = 22; 36 weeks, n = 22.

FIGURE 30.
Fetal liver volume: sagittal plane. Placebo: 28 weeks, n = 25; 36 weeks, n = 23; metformin: 28 weeks, n = 23; 36 weeks, n = 22.
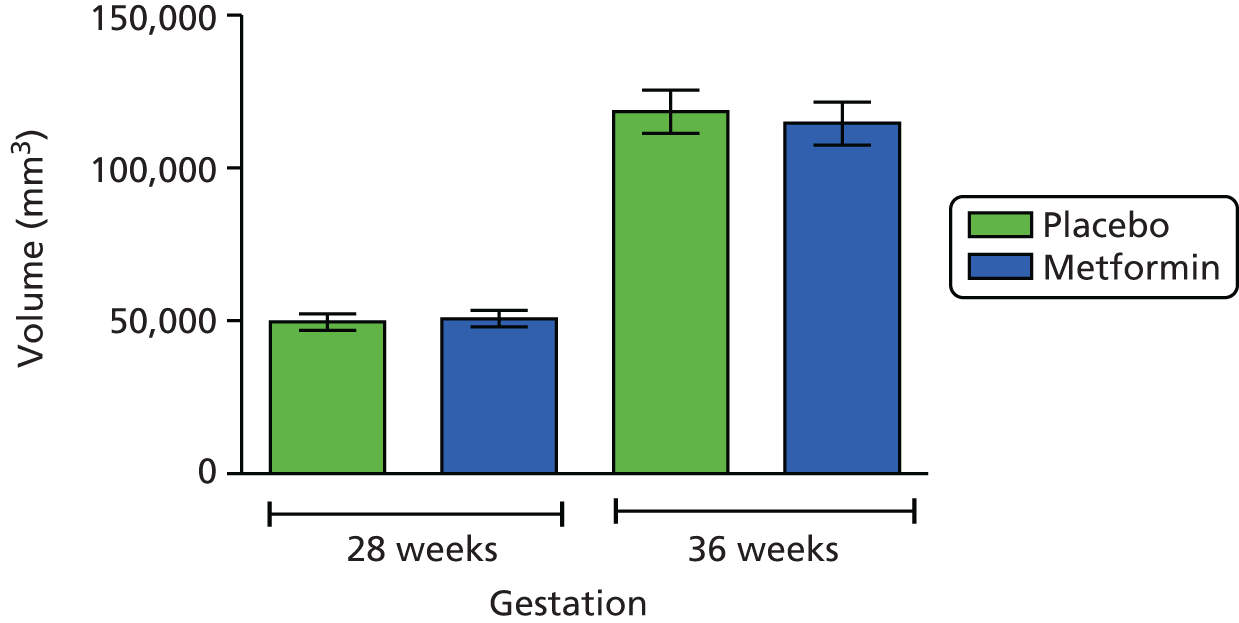
FIGURE 31.
Fetal hepatic fat fraction. Placebo, n = 17; metformin, n = 16 (paired samples).

FIGURE 32.
Fetal subcutaneous (s/c) fat volume: (a) sagittal plane; and (b) axial plane. Subcutaneous fat sagittal plane: placebo, n = 14; metformin, n = 25; subcutaneous fat axial plane: placebo, n = 8; metformin, n = 5.
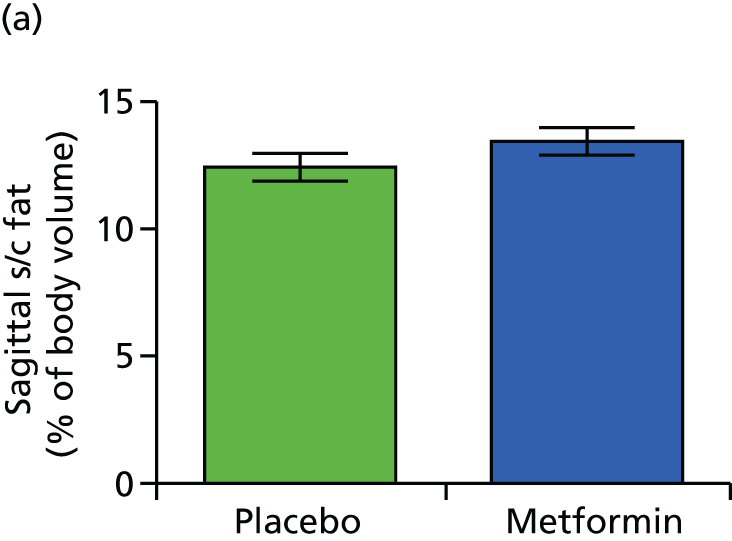

Discussion
The purpose of this substudy was to assess whether or not distribution of body fat in obese pregnant women was altered by metformin. We also examined the fetus with the aim of assessing effect on liver volume, hepatic fat content and fetal subcutaneous fat deposits. We aimed to scan 40 participants at both 28 and 36 weeks’ gestation. Ultimately, we scanned 57 participants, with longitudinal maternal data available for 37 participants (those who attended for both scans). We obtained longitudinal fetal hepatic lipid data in 17 and 16 of the participants allocated to the placebo and metformin groups, respectively. Liver volume was successfully measured in the axial plane in 25 and 22 fetuses at 28 and 36 weeks, respectively, in participants allocated to placebo and in 22 fetuses at both time points in participants allocated to metformin. Liver volume was successfully measured in the sagittal plane in 25 and 23 fetuses at 28 and 36 weeks, respectively, in participants allocated to placebo and in 22 fetuses at both time points in participants allocated to metformin. Subcutaneous fat mass was measured in the sagittal plane in 14 and 25 subjects allocated to placebo and metformin, respectively. In the axial plane it was successfully measured in eight and five subjects in the placebo and metformin groups, respectively.
We have demonstrated that the maternal scanning protocols work well in obese pregnant women, with good inter- and intrarater correlation. We have shown that it is possible to obtain the fetal data as we had planned, although not in every subject scanned because of fetal movement and time limitations, with priority being given to acquisition of the maternal data. There was reasonably good inter- and intrarater correlation for the fetal liver volume data. Correlation was less good for the subcutaneous fat measurements but the cohort numbers were small for this study.
In summary, participants in both the placebo and the metformin arms of the study lost subcutaneous fat over the course of pregnancy but there was no difference in the percentage change between the two groups. We saw no differences in the amounts of visceral fat either between treatment groups or by gestation. Ectopic lipid deposition in both the liver and skeletal muscle was also the same in both groups and did not change between 28 and 36 weeks’ gestation.
Mean hepatic fat fraction in both groups was relatively low, particularly when measured by 1H-MRS. When measured using the Dixon method, it was higher than we have previously seen in a cohort of 10 lean and 10 obese non-diabetic pregnant women116 but still lower than in a cohort of obese non-pregnant women117 and certainly below the diagnostic threshold for non-alcoholic fatty liver disease. 118 Mean skeletal muscle fat fraction measured by 1H-MRS was similar to that in our previous cohort of obese pregnant women116 and to that of a group of normally glucose-tolerant obese (mean BMI 30 kg/m2) non-pregnant women. 119 This suggests that pregnancy itself, rather than metformin, may be exerting a protective effect on the liver, which deters accumulation of lipid in this site.
The fetal data must be interpreted with a greater degree of caution. The scanning protocols are not well established and were subject to some method development during the study period. Fetal movement during the image acquisition period is an extra challenge. We aimed to limit the scan duration to 60 minutes, which was the limit of acceptability for the participants, and we prioritised the acquisition of maternal data in this time. However, we still acquired a reasonable amount of data suitable for analysis and have not demonstrated any differences in fetal liver volumes or hepatic and subcutaneous fat depots between the placebo group and the metformin group. This is in keeping with the primary outcome of the EMPOWaR trial, which demonstrated no difference in birthweight of the babies, and also the secondary outcomes, for which we saw no difference in neonatal fat mass measured by ADP or in neonatal skinfold thicknesses (see Maternal and neonatal body composition).
In conclusion, we have not demonstrated any effect of metformin in obese pregnant women on maternal or fetal body fat distribution at 28 and 36 weeks’ gestation.
The effect of metformin on the hypothalamic–pituitary–adrenal axis
Background
It is now well recognised that the intrauterine environment is a key time for determining not only fetal growth and consequent birthweight but also future life health, a concept known as ‘early life programming’. 120 One of the key mechanisms thought to be responsible for programming is overexposure of the fetus to glucocorticoids, with consequent alterations to the fetal hypothalamic–pituitary–adrenal (HPA) axis. 121,122
The maternal HPA axis undergoes significant activation during pregnancy, resulting in a substantial increase in maternal cortisol levels. 123,124 This is partly because of placental secretion of large quantities of corticotrophin-releasing hormone. 125 These physiological changes in the HPA axis are essential for normal fetal growth and development and promotion of fetal organ maturation and are also thought to have a role in a gestational clock mechanism signalling the appropriate time for the onset of labour. 126 However, over- and possibly underexposure to glucocorticoids in utero can have adverse effects on the fetus that persist into adult life. Glucocorticoids are lipophilic and readily cross the placenta, yet fetal glucocorticoid levels are tenfold lower than maternal levels because of the action of placental 11β-HSD2. 127 11β-HSD2 acts as a placental enzyme barrier that converts active cortisol into inactive cortisone,127 thus protecting the fetus from overexposure to excess glucocorticoid. Both animal and human studies have suggested that the efficiency of placental 11β-HSD2 is variable and may be weakened by factors such as diet, infection, inflammation, hypoxia and stress,121,122 thus allowing greater transplacental passage of cortisol to the fetus. Even modest changes in placental 11β-HSD activity appear to have the potential to significantly alter fetal exposure. 128,129
Glucocorticoids exert their effect through the glucocorticoid receptor (GR), which acts as a transcription factor. The activated GR translocates to the nucleus, binds to GR response elements in the promoters of target genes and influences their transcription. 130 11β-HSD1 is the enzyme that converts cortisone back to cortisol. Alterations in levels of GR could also affect the sensitivity of the placenta to cortisol and alterations in levels of 11β-HSD1 will affect cortisol availability.
The impact of undernutrition in pregnancy on the HPA axis has been extensively studied in both animal models and human cohorts. It is difficult to ascertain the impact of diet alone because of potential confounding from the impact of stress but it would appear that prenatal exposure to undernutrition has an adverse impact on long-term health, mediated in part through overexposure to intrauterine glucocorticoid. 131 The impact of overnutrition or obesity in pregnancy on the HPA axis is not yet known. In the non-pregnant population, obesity is associated with activation of the HPA axis but there is associated increased hepatic metabolism and renal excretion of cortisol results in near-normal levels of circulating cortisol. 132–134 If dysregulation is maintained during pregnancy, fetuses of obese women may be exposed to altered levels of glucocorticoids compared with fetuses of lean women, with consequent impact on birthweight and health in later life. The current data on the impact of obesity are conflicting. One study found that women who were obese at the start of pregnancy had elevated evening salivary cortisol in the third trimester, particularly women who had gestational weight gain greater than that in Institute of Medicine guidelines. 135 Another study demonstrated higher hair cortisol (a measure of longer-term cortisol exposure) in obese women. 136 Contrary to this, our own recently published data suggest that obese women have lower total serum levels of cortisol and lower calculated free levels of cortisol through pregnancy than lean women,137 suggesting that cortisol exposure to the fetus may be lower in obese pregnancy. A better understanding of the impact of obesity on offspring birthweight and the maternal and fetal HPA axis is clearly required.
As the primary outcome of our study was birthweight, and fetal exposure to cortisol is a likely determinant of birthweight, this mechanistic substudy was designed to examine the effect of metformin on maternal salivary cortisol levels (as a measure of cortisol exposure). Furthermore, as placental glucocorticoid metabolism is known to be tightly regulated by changes in inflammation, and in a rodent model metformin altered expression of genes involved in glucocorticoid metabolism in skeletal muscle and adipose tissue, we tested whether or not treatment with metformin had any effect on the placental expression of genes regulating fetal glucocorticoid exposure, including GR, 11β-HSD1 and 11β-HSD2.
Methods
Salivary cortisol
For the measurement of diurnal cortisol patterns, all participants in the study were invited to submit bedtime and waking saliva samples at the baseline visit (after randomisation but before starting study treatment) and at 28 and 36 weeks’ gestation. Saliva samples were collected in Salivette® (Sarstedt, Nümbrecht, Germany) containers at bedtime and on waking, and time of collection was recorded on the containers. Participants were asked not to eat, drink, smoke or brush their teeth for the half-hour preceding collection. Participants were asked to store the samples in their home refrigerator for no more than 1 week and post them back to our laboratory. Samples were then stored at –80 °C.
Salivary cortisol was measured by ELISA using a standard kit from Demeditec Diagnostics (Kiel, Germany) according to the manufacturer’s protocol. The limit of detection was 2.5 ng/ml, with a mean intra-assay CV of 5.6% and a mean interassay CV of 6.9%.
Statistical analysis
Data were compared between treatment groups using a linear regression model, adjusted by BMI band (30–39 kg/m2 vs. > 40 kg/m2). Data were log-transformed for analysis and transformed back to natural numbers for presentation of the results.
Placental biopsies
A subset of women participating in the study consented to placental biopsy. The characteristics of participants in the substudy were similar to those of the women in the study overall. Samples were collected and the study carried out blind to treatment allocation.
Sample preparation
Placental biopsies were obtained at delivery from consenting women participating in the EMPOWaR trial in accordance with the SOP (see Appendix 7). Samples were stored in RNA Later® solution (ThermoFisher Scientific) for 24 hours at 4 °C. The RNA Later solution was then removed and the tissue stored at –80 °C prior to analysis.
Ribonucleic acid extraction and quantitative real-time reverse transcription polymerase chain reaction
Ribonucleic acid (RNA) was extracted from 40–60 mg of tissue using the RNeasy® Mini RNA extraction kit (Qiagen, Manchester, UK), according to the manufacturer’s protocol. A deoxyribonuclease step was included to remove genomic deoxyribonucleic acid (DNA) from the samples. The RNA obtained was assessed for quality and quantity using the RNA 6000 Nano Kit and Bioanalyzer (Agilent Technologies, Stockport, UK), according to the manufacturer’s protocol. Complementary deoxyribonucleic acid (cDNA) was synthesised from RNA (1 µg) using the High Capacity cDNA Reverse Transcription Kit (Applied Biosystems™, Life Technologies, Loughborough, UK) in a 50-µl reaction, according to the manufacturer’s protocol. Negative controls [no RNA (water) and no reverse transcriptase (RT)] were included.
The expression of GR (NR3C1), 11β-HSD1 and 11β-HSD2 was quantified using Taqman® Gene Expression Assays: GR (Hs00353740_m1), HSD1 (Hs01547870_m1) and HSD2 (Hs00388669_m1), with tyrosine 3-mono-oxygenase/tryptophan 5-mono-oxygenase activation protein, zeta polypeptide (YWHAZ, Hs03044281_g1) used as an endogenous control (ThermoFisher, Renfrew, UK). All probes spanned exons so would not amplify any residual genomic DNA present. Samples were assayed in triplicate on 384-well plates. Samples from the same patient for the different gene expression assays were run on the same plate. Negative controls [no RNA, no RT, no cDNA (water)] were included on every plate. A cDNA sample from one patient (chosen as the sample had high levels of good-quality RNA) was included on every plate to show interplate variation and act as a calibrator sample. Plates were run on a 7900HT bioanalyser (Applied Biosystems, Foster City, CA, USA). Gene expression was calculated using the 2-ΔΔCt method of analysis. 138
Statistical analysis
Gene expression data were compared between treatment groups using a linear regression model, adjusted by BMI band (30–39 kg/m2 vs. > 40 kg/m2) and mode of delivery (vaginal delivery vs. caesarean section) as these factors are known to affect gene expression in the placenta. 139 Data were log-transformed for analysis and transformed back to natural numbers for presentation of results.
Results
Salivary cortisol
Samples were obtained from 235 participants. Unblinding after data lock revealed final cohort numbers as detailed in Table 14. Baseline demographics were similar between the two groups and are also shown in Table 14.
Characteristic | ITT | Per protocol | ||
---|---|---|---|---|
Placebo (n = 121) | Metformin (n = 114) | Placebo (n = 77) | Metformin (n = 79) | |
Age (years), mean (SD) | 29.9 (5.0) | 29.1 (5.6) | 29.8 (5.0) | 29.9 (5.5) |
Nulliparity, mean (SD) | 50 (41.3) | 52 (45.6) | 30 (39.0) | 38 (48.1) |
BMI (kg/m2), mean (SD) | 36.9 (5.0) | 37.5 (5.2) | 36.7 (5.0) | 37.2 (4.6) |
Current smoking, n (%) | 9 (7.4) | 15 (13.2) | 5 (6.5) | 8 (10.1) |
Highest educational qualification, n (%) | ||||
Up to 16 years | 41 (33.9) | 31 (27.2) | 23 (29.9) | 16 (20.3) |
> 16 years | 80 (66.1) | 83 (72.8) | 54 (70.1) | 63 (79.7) |
There was no difference in the increment of mean cortisol on waking at each gestation time point between the placebo group and the metformin group (Table 15 and Figure 33).
Time point | ITT | Per protocol | ||||||||||||
---|---|---|---|---|---|---|---|---|---|---|---|---|---|---|
Placebo | Metformin | Adjusted mean difference | 95% CI | p-value | Placebo | Metformin | Adjusted mean difference | 95% CI | p-value | |||||
Mean | SD | Mean | SD | Mean | SD | Mean | SD | |||||||
Baseline | ||||||||||||||
n | 107 | 101 | 70 | 72 | ||||||||||
Bedtime | 2.23 | 8.0 | 1.99 | 2.38 | 1.51 | 3.7 | 2.00 | 2.7 | ||||||
Waking | 9.03 | 24.6 | 6.4 | 3.9 | 10.23 | 30.2 | 6.42 | 3.5 | ||||||
Mean difference | –6.80 | 22.5 | –4.36 | 5.1 | 1.023 | 0.978 to 1.071 | 0.3111 | –8.72 | 26.7 | –4.42 | 5.0 | 1.040 | 0.973 to 1.111 | 0.2429 |
28 weeks | ||||||||||||||
n | 48 | 52 | 36 | 47 | ||||||||||
Bedtime | 3.17 | 4.0 | 2.04 | 1.6 | 3.19 | 4.0 | 2.04 | 1.7 | ||||||
Waking | 8.68 | 6.9 | 9.26 | 4.3 | 9.28 | 7.7 | 9.26 | 4.5 | ||||||
Mean difference | –5.51 | 7.8 | –7.22 | 3.7 | 0.994 | 0.984 to 1.004 | 0.2223 | –6.09 | 8.2 | –7.22 | 3.9 | 0.997 | 0.987 to 1.008 | 0.6153 |
36 weeks | ||||||||||||||
n | 36 | 41 | 28 | 35 | ||||||||||
Bedtime | 3.40 | 3.15 | 2.98 | 1.8 | 3.63 | 3.5 | 2.89 | 1.7 | ||||||
Waking | 8.90 | 4.2 | 8.74 | 4.8 | 8.99 | 4.3 | 9.04 | 5.1 | ||||||
Mean difference | –5.50 | 3.7 | –5.74 | 5.6 | 0.999 | 0.990 to 1.008 | 0.7780 | –5.35 | 3.7 | –6.15 | 5.9 | 0.996 | 0.986 to 1.007 | 0.4921 |
FIGURE 33.
Bedtime and waking salivary cortisol: (a) baseline; (b) 28 weeks; and (c) 36 weeks.
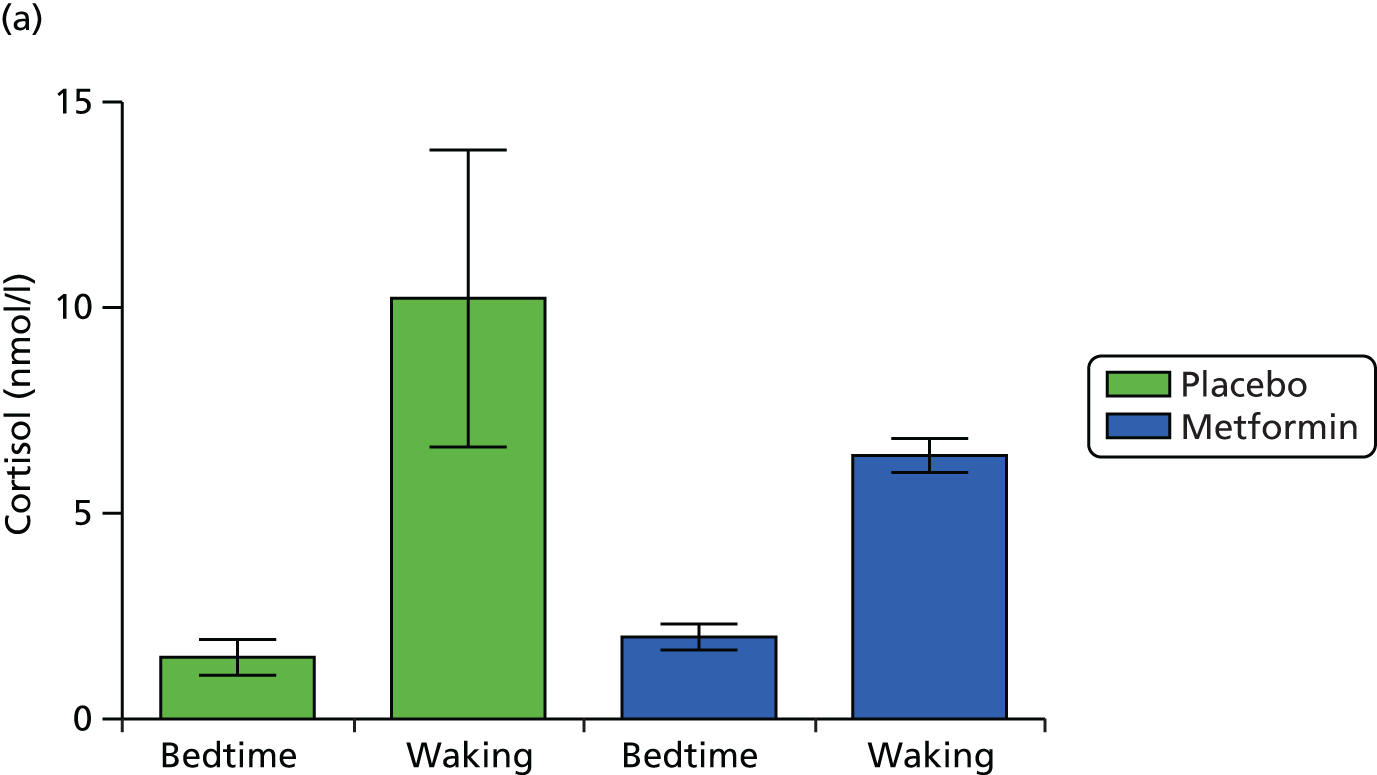


Placental biopsies
Samples were obtained from 125 participants from six participating centres. Unblinding after data lock revealed final cohort numbers as follows: placebo group n = 64 and metformin group n = 61 for ITT analysis; placebo group n = 53 and metformin group n = 52 for per-protocol analysis (as previously defined). Baseline demographics were similar between the two groups and are shown in Table 16.
Characteristic | ITT | Per protocol | ||
---|---|---|---|---|
Placebo (n = 64) | Metformin (n = 61) | Placebo (n = 53) | Metformin (n = 52) | |
Age (years), mean (SD) | 29.2 (5.5) | 29.7 (5.3) | 29.1 (5.4) | 30.3 (5.3) |
BMI (kg/m2), mean (SD) | 37.0 (5.2) | 38.3 (5.3) | 36.4 (4.7) | 37.8 (4.8) |
Current smoking, n (%) | 7 (10.9) | 10 (16.4) | 5 (9.4) | 7 (13.5) |
Highest educational qualification, n (%) | ||||
Up to 16 years | 20 (31.3) | 13 (21.3) | 18 (34.0) | 9 (17.3) |
> 16 years | 44 (68.8) | 48 (78.7) | 35 (66.0) | 43 (82.7) |
Mode of delivery, n (%) | ||||
Vaginal | 37 (57.8) | 41 (67.2) | 31 (58.5) | 37 (71.2) |
Caesarean section | 27 (42.2) | 20 (32.8) | 22 (41.5) | 15 (28.8) |
Gestation at delivery (days), mean (SD) | 277.5 (9.4) | 277.6 (9.6) | 277.3 (9.5) | 277.8 (10.1) |
Male baby sex, n (%) | 31 (48.4) | 34 (55.7) | 24 (45.3) | 28 (53.8) |
There were differences in RNA quality but not RNA yield according to recruitment centre. However, all of the RNA samples were used for cDNA synthesis and all samples gave results for gene expression. There was no indication of any variation between plates. None of the negative controls showed contamination in the reverse transcription polymerase chain reaction process.
The gene expression results are shown in Table 17. There was no difference in GR, 11β-HSD1 or 11β-HSD2 mRNA levels (relative to control gene expression) between the two treatment groups after adjustment for mode of delivery and BMI band. This was the same for both the ITT analysis and the per-protocol analysis.
Gene expression | ITT | Per protocol | ||||||||||||
---|---|---|---|---|---|---|---|---|---|---|---|---|---|---|
Placebo | Metformin | Estimated mean difference | 95% CI | p-value | Placebo | Metformin | Estimated mean difference | 95% CI | p-value | |||||
Mean | SD | Mean | SD | Mean | SD | Mean | SD | |||||||
GRa,b | 0.8404 | 0.49 | 0.8088 | 0.44 | 0.975 | 0.790 to 1.206 | 0.82 | 0.7963 | 0.41 | 0.8410 | 0.45 | 1.060 | 0.857 to 1.311 | 0.59 |
11β-HSD1b,c | 2.2783 | 3.09 | 2.4612 | 3.20 | 1.142 | 0.725 to 1.802 | 0.56 | 2.2935 | 3.09 | 2.4747 | 3.38 | 1.117 | 0.666 to 1.876 | 0.67 |
11β-HSD2b,c | 4.2637 | 7.28 | 3.3163 | 5.40 | 0.855 | 0.588 to 1.241 | 0.41 | 3.5363 | 5.50 | 3.6518 | 5.78 | 0.970 | 0.662 to 1.423 | 0.88 |
Discussion
We have not demonstrated any differences in bedtime or waking salivary cortisol between obese women taking metformin and those taking placebo. The findings should be interpreted with caution as the sample size was small and there was considerable variation in the waking levels of cortisol (as is well documented in other studies). Nevertheless, these findings accord with the lack of change in fasting morning plasma cortisol between groups and suggest that metformin treatment had no effect on the activity of the maternal HPA axis (see Table 6). In addition, we found no difference in the expression of GR, 11β-HSD1 or 11β-HSD2 in the placentae of women who took metformin during pregnancy compared with those who took placebo once BMI band and mode of delivery were taken into account. In our analyses we adjusted for mode of delivery, which is recognised to alter placental gene expression of genes related to fetal glucocorticoid exposure. We acknowledge that there may be other confounding factors such as time between delivery and placental sample collection that we did not adjust for in the statistical analyses. However, all samples were collected using standard protocols and good-quality RNA was extracted from the tissues used. Although 11β-HSD2 is known to be sensitive to inflammation and we observed changes in circulating inflammatory markers at 36 weeks’ gestation between women taking metformin and those taking placebo, the lack of change observed here could be consistent with a compensatory change in placental metabolism in women taking metformin. Alternatively, as our primary outcome measure of birthweight was the same in the two treatment groups, and fetal glucocorticoid exposure is a key determinant of birthweight, it is perhaps unsurprising that we have not seen an effect on this determinant of birthweight.
Myometrial contractility and glycogen storage
Introduction
Maternal obesity is well recognised to be associated with an increased risk of caesarean section. 61,140–143 Although this association is widely reported, little is known about the mechanism behind it. There is a commonly held clinical assumption that this is related to fat dystocia from the maternal tissues and/or dystocia as a result of larger babies. These are indeed likely to be contributory factors but myometrial contractility is known to be impaired in obese women, even after adjustment for birthweight. 144 Additionally, contractility is impaired in women with diabetes mellitus. 145 Of particular note is that the response to oxytocin is reduced in these women. Thus, if the diabetic environment has already reduced contractility, for example because of glycosylation of proteins and fibre damage, leading to a poorly progressing labour in these women, then oxytocin is likely to be needed. The reduced responsiveness to oxytocin would suggest that its efficacy is reduced and this may contribute to the increased rate of caesarean sections in this group of women. In addition, there is also evidence that insulin per se is detrimental to contractility, possibly by causing hyperpolarisation. 146
Metabolic changes in the uterus are a recognised preparation for labour. These include changes in lactate dehydrogenase isoforms to those favouring hypoxic conditions and, of interest to this study, increased glycogen storage from glucose uptake into myometrial cells, along with fatty droplet inclusions. 147,148 These metabolic changes are expected to maintain forceful contraction, necessary for labour, in the face of repetitive, transient ischaemic episodes, consequent to occlusion of the blood vessels within the myometrium with each contraction. It could therefore be suggested that, if insulin sensitivity improvement occurs with metformin, then more glycogen would be stored and labour outcome improved. This suggestion can be tested directly by determining glycogen levels and also by challenging the myometrial tissue with a solution lacking glucose and monitoring contractile activity.
This mechanistic substudy was designed to examine whether or not improving insulin sensitivity with metformin improved myometrial contractility in obese pregnant women and increased myometrial glycogen content.
Methods
At caesarean section, biopsy of lower-segment myometrium was obtained from consenting women participating in the EMPOWaR trial, according to the SOP (see Appendix 8). All biopsies were immediately placed in physiological saline (PSS), stored at 4 °C and used for experimentation within 24 hours of collection for contractility studies or snap frozen at –80 °C for later analysis of glycogen storage.
Contractility measurements
Contractions were measured as previously described. 149 Briefly, in the laboratory, biopsies were cleared of endometrium, excess blood and any fetal membranes. Strips of myometrium 5-mm long, 2-mm wide and 1-mm thick were cut so that the longitudinal axis was aligned with the direction of the muscle fibres. Four strips were then simultaneously mounted, secured by aluminium clips, in a 1-ml chamber bath. To ensure the amount of stretch applied was standardised across experiments, all strips were placed under isometric conditions with a resting tension of 2 mN. Contractility was recorded using a tension transducer (FORT25; WPI, Sarasota, FL, USA) attached to one end of the strip, which was connected to a data acquisition system (DataTrax; WPI). The strips were superfused with PSS at a rate of 1.5 ml/minute at pH7.4 and 37 °C. Under these conditions, most strips developed a steady baseline tension and achieve spontaneous contractions within 2 hours of continual superfusion.
After strips began to contract, a control period was established of between four and six contractions, each of similar amplitude and frequency, and then strips were superfused with PSS (control), PSS lacking glucose (PSS 0-glucose) or PSS and 0.5 nM oxytocin (oxytocin) for the remainder of the experiment, as detailed below.
Measurements of mean amplitude, duration and frequency of contractions, as well as total integral of force (measured as area under the curve, AUC), were made at 30-minute intervals for each myometrial strip. Simultaneous experiments (4–12 strips per biopsy) enabled the contractility of ‘test’ strips to be compared with that of time-matched control strips that were contracting with similar amplitude and frequency at the start of each experiment.
Experiments in zero glucose
After 30 minutes of control contractions, test strips were bathed in PSS 0-glucose. Contractility measurements were made from test strips at 30-minute intervals and compared with those of time-matched controls.
Glycogen determination
Glycogen determination was performed following established methodologies. 148,150 Myometrial biopsy samples were snap frozen in liquid nitrogen in each hospital and stored at −80 °C. Prior to analysis, each biopsy was freeze-dried for 48 hours in a Modulyo® 4K freeze dryer (Edwards, Crawley, UK) to enable inspection and accurate dissection of approximately 10-mg aliquots of myometrium. Each aliquot was then carefully weighed and homogenised in 500 µl of water for 3 minutes (oscillation frequency of 30 Hz) using a Retsch MM 400 milling system (Haan, Germany). The homogenate was then boiled for 5 minutes to remove enzyme activity and centrifuged (at 4 °C) for 5 minutes at 13,000 g.
The lysates were analysed using a commercially obtained glycogen assay kit (Sigma-Aldrich, St Louis, MO, USA). In brief, the assay utilises a coupled enzyme reaction that hydrolyses the glycogen present in the samples into glucose. The glucose is then oxidised to yield a colorimetric product proportional to the amount of glycogen present (detectable at 570 nm) after subtraction of background signals. The lysates were diluted 10- and 20-fold in water before hydrolysis to align with the optical density range of the assay standard curve (standard glycogen concentration range 0.4–2.0 µg/well). The samples were assayed according to the manufacturer’s protocol and the colorimetric product was quantified using a ThermoFisher Scientific Multiskan Ascent 354 microplate reader.
Results
All sample collection and analysis were performed blind to treatment allocation group. Eight samples were obtained for the contractility studies. Unblinding following data lock revealed final cohort numbers as follows: placebo, n = 2; metformin, n = 6. Twenty-eight samples were obtained for the glycogen storage study with final cohort numbers as follows: placebo, n = 17; metformin, n = 11. Baseline characteristics are shown in Table 18.
Characteristic | ITT | Per protocol | ||
---|---|---|---|---|
Placebo | Metformin | Placebo | Metformin | |
Contractility study | ||||
n | 2 | 6 | 2 | 5 |
Age (years), mean (SD) | 34.5 (7.8) | 27.7 (4.3) | 34.5 (7.8) | 28.6 (4.0) |
Gestation at delivery (days), mean (SD) | 274.0 (1.4) | 272.5 (3.7) | 274.0 (1.4) | 273.0 (3.9) |
Nulliparity, n (%) | 0 (0) | 4 (66.7) | 0 (0) | 4 (80.0) |
BMI (kg/m2), mean (SD) | 37.6 (7.1) | 38.1 (3.7) | 37.6 (7.1) | 37.8 (4.1) |
Glycogen storage study | ||||
n | 17 | 11 | 15 | 7 |
Age (years), mean (SD) | 29.8 (5.8) | 30 (4.7) | 29.2 (5.9) | 31.0 (5.0) |
Gestation at delivery (days), mean (SD) | 274.9 (7.7) | 272.5 (4.2) | 275.7 (7.6) | 273.6 (3.2) |
Nulliparity, n (%) | 6 (35.3) | 4 (36.4) | 5 (33.3) | 4 (57.1) |
BMI (kg/m2), mean (SD) | 37.5 (4.6) | 40.6 (4.2) | 37.7 (4.7) | 40.5 (4.7) |
Contractility
Responses to oxytocin were calculated and showed the expected increases in amplitude and overall force (AUC, integral). The mean increases in amplitude and force from control values (preceding spontaneous contractions, 100%) for all samples (n = 8) were 186% ± 21% and 216% ± 31% (standard error of the mean), respectively. In the metformin samples (n = 6) these values were 170% ± 12% and 210% ± 26%, respectively. In the placebo samples (n = 2) the averages were 261% and 296.1%, respectively (Figure 34).
FIGURE 34.
Example of myometrium contraction trace. Continuous trace of spontaneous contractions from paired strips of myometrium. Spontaneous contractions were established and then oxytocin (0.5 nM) was superfused throughout the experiment. After 30 minutes of control contractions, test strips were bathed in PSS 0-glucose. Contractility measurements were made from test strips at 30-minute intervals and compared with those of time-matched controls.

We also examined the effects of glucose removal on the above sample (see Figure 34). After 120–150 minutes in PSS 0-glucose, contractions in the eight samples decreased from control values (100%) to an amplitude of 83% ± 6% and overall force (AUC) was 57% ± 9%. In the metformin samples these values were 82% ± 6% and 69% ± 10% and in the placebo samples these values were 94% and 30%, respectively.
Formal statistical analyses to compare the results between the metformin group and the placebo group were not performed because of low sample numbers.
Glycogen
Glycogen content was determined in 25 samples. Mean glycogen in myometrium was 12.8 ± 0.7 µg/mg tissue. Of the 25 samples, 13 were placebo samples and five were metformin samples, with the remaining seven samples not per the protocol. Subsequent analysis was retrospectively restricted to data obtained from compliant women. In the placebo samples (n = 13) mean glycogen was 13.3 ± 1.1 µg/mg and in the metformin samples (n = 5) it was 11.8 ± 0.7 µg/mg (unpaired t-test).
Discussion
It is not possible to draw any robust conclusions from this substudy as the sample size was so small and the distribution of treatment allocation following unblinding was too uneven. The unequal distribution of treatment allocations between the two groups is a consequence of an unavoidable risk when carrying out mechanistic studies blind to treatment allocation. Clearly, a large sample size will minimise the possibility of this being a problem. We had hoped to obtain a much larger sample of myometrial biopsies but the provision of expertise for ‘out-of-hours’ tissue collection was limited. We had anticipated that we would see improved myometrial contractility responsiveness to oxytocin and higher glycogen storage in samples from participants taking metformin. We were unable to demonstrate any differences but, as stated, our sample size was very small. There are few data on the effect of metformin on smooth muscle contractility. One small study of human myometrial biopsies did not demonstrate any differences in in vitro contractility with the addition of metformin. 151 However, there is evidence from animal models that metformin improves contractility in vascular and penile smooth muscle, mediated through activation of adenosine monophosphate-activated protein kinase or inhibition of the production of reactive oxygen intermediates. 152–155 We have previously shown impaired uterine contractility in the presence of increased cholesterol. 156 Increased cholesterol is well recognised to be associated with obesity and we hypothesised that this may be one of the contributory mechanisms behind the excess risk of caesarean delivery in obese women. In non-pregnant populations metformin has a beneficial effect on the cholesterol profile. 79 However, we did not see any effect on cholesterol in our study population and therefore it is not possible to confirm this hypothesis from these data.
Although our sample size in this substudy was insufficient to draw any conclusions, we did not see any differences in rates of caesarean section or post-partum haemorrhage in the main trial cohort, which would perhaps suggest that metformin has not had either a beneficial or an adverse effect on myometrial contractility.
Chapter 5 Qualitative study
Introduction
Recruitment and retention of trial participants is fundamental to the success of any RCT. A high number of eligible women declined to participate in the EMPOWaR study. In addition, a number of eligible women agreed to participate, signed a consent form and underwent baseline visits and screening, but subsequently did not take trial medication or began to take trial medication and stopped.
We proposed to conduct interviews both with women who did not wish to participate and with women who consented but who did not comply with the treatment regime. However, we were able to recruit only one woman who had declined to participate in the study. Thus, our sample consisted only of women who consented to participate in the study but who did not comply with the treatment regime.
Methods
Twenty-three women who initially consented to participate in the trial but who did not comply with the treatment regime were telephoned to ask if they would participate in an interview to discuss the reasons why they had not taken the trial tablets. Of these, six women were successfully contacted and sent a recruitment pack and three women returned a signed consent form. Telephone interviews took place between February and June 2014 and were audio-recorded and transcribed with the participants’ consent. Pseudonyms are used throughout.
Results
The women’s ages ranged from 26 to 40 years. One of the women (Sam) was expecting her first baby when she took part in the EMPOWaR trial. A second (Penny) had two other children and the third (Janet) was pregnant with her fourth baby at the time that she was recruited to the trial.
Janet and Penny were initially offered an information sheet by their community midwife at their booking appointment and then were telephoned by a trial research midwife. Sam said that her community midwife had mentioned the study to her but no written information or referral was offered. She was telephoned later by a research midwife to discuss the study and agreed to be sent an information sheet in the post.
All three women said that they were happy to be offered information about the study. All three stated that the written information was clear and easy to understand. All three also said that they were very satisfied with the level of information given during their initial telephone conversation and in appointments with the research midwives.
The women recalled having few questions to ask the research midwives about the study. Penny said that her main question was whether or not it was safe for her to take them during pregnancy and said that she felt reassured by the answers she was given.
The reasons the women gave for agreeing to participate in the study initially were varied. Sam said that as it was her first baby she liked the idea of the extra antenatal check-ups that she would have as a result of participating in the study. She also said that she was motivated by the idea that she would be helping other women through her participation. Janet had previously participated in pregnancy-related research while attending the antenatal metabolic clinic at Edinburgh Royal infirmary in a previous pregnancy and was therefore familiar with the research team. She cited this as a reason for agreeing to participate in the trial. Penny said that she had a friend who had participated in the trial and who spoke positively to her about it; thus, Penny described herself as ‘quite keen’ and ‘excited’ to take part. Penny also said that she would like to help other women in the future and said that this was another reason why she agreed to take part in the study.
Sam did not take any of her trial medication. She said that when it was explained to her that she might receive a placebo she decided not to take part, as she wanted only to take an active treatment not a placebo. Sam was taking folic acid and iron tablets at the time she was recruited to the study and felt that she did not want to take a placebo tablet in addition to this. Sam said, ‘If I was guaranteed a [metformin] tablet I would take it, but as it wasn’t guaranteed, I thought, “no thanks” ‘. Sam said that she discussed her decision with her mother and her partner, but she said that she attended her trial appointments alone and it was her choice not to take the trial medication. She continued to attend her data collection appointments during pregnancy.
Janet said that she did not initially understand that the study would involve taking medication when she agreed to attend for screening. She mistakenly believed that the nature of her participation would involve only physical measurements and data collection, similar to those recorded in her previous pregnancy when she had also participated in clinical research. Janet said that she was not happy to take metformin during pregnancy. She said that, as this was her fourth baby and she had never been offered metformin during previous pregnancies, she did not feel comfortable taking it during this pregnancy. She said, ‘They could do anything they wanted to me, but I wasn’t willing to take any risk with my baby’. Janet spoke to her husband about the study and said that he also ‘wasn’t happy’ for her to take the trial medication. Janet also cited the fact that she lived some distance away from the trial centre and the study necessitated several visits to the hospital as another reason for her withdrawing from the study. She said that, although she had participated in research at the same hospital in a previous pregnancy, she had only had one other child at that time. She now had three other children, making childcare arrangements during hospital visits much more challenging.
Penny took her trial tablets for approximately 5 weeks. When her pregnancy reached 21 weeks she had a premature spontaneous rupture of membranes. She was initially admitted to hospital to be monitored and said that she ‘panicked’ about her participation in the study. She discussed it with her husband and stopped taking the study medication. Penny continued to attend data collection appointments during her pregnancy but did not recommence trial medication.
When asked if they would participate in research in the future, all three women said that they would be willing to consider it. Penny said that she would be particularly open to participating in any trial that might help her to lose weight. Both Janet and Penny suggested that clinical community midwives should have more knowledge and information regarding pregnancy-related research, as they were not able to answer all of their questions when initially offering study information.
Discussion
It is possible that the two women in this sample who did not take the medication would have declined to participate had they fully understood the implications, risks and benefits at the time their consent was obtained. It has been observed elsewhere that participants have difficulty understanding the true meaning of a RCT and the rationale behind randomisation. 157–159 Like Sam, participants have expressed a preference for certain treatment arms. 160–162 Janet said that once she understood that she would be taking tablets during pregnancy, her concern was the potential risk of participating in the trial. Her concerns echo those of others who have declined to participate in other RCTs. 161,163
The study sample was very small. However, the results suggest that particular attention should be given to designing appropriate trial information materials and processes that fully inform potential participants of the benefits and risks of participation. In particular, when recruiting trial participants, attention should be paid to ensuring that trial information has been understood before obtaining consent.
Chapter 6 Discussion and conclusions
Summary of findings
This was the first RCT of a pharmacological intervention, metformin, to reduce the risk of excessive birthweight offspring in non-diabetic obese pregnant women. Contrary to our hypothesis, metformin had no effect on our primary outcome of birthweight. In addition, we did not see any effect on our secondary outcomes of insulin sensitivity at 36 weeks’ gestation; maternal and neonatal anthropometry; and neonatal CRP, glucose and insulin measured in cord blood. Since publishing the results of this trial28 another group has published a similar study with a slightly smaller sample size and has again found no effect of metformin on birthweight in obese pregnant women without diabetes mellitus. 19
The inflammatory markers CRP and IL-6 were both lower in the metformin group. These markers are known to be elevated in obese pregnant women compared with lean pregnant women67 and may be associated with adverse pregnancy outcomes such as preterm birth and pre-eclampsia. 164,165
Fasting glucose and insulin were lower in the metformin group at 28 weeks’ gestation in the ITT analysis. On per-protocol analysis, fasting and 2-hour glucose, insulin and HOMA-IR score were all lower in the metformin group. The lack of effect at 36 weeks’ gestation may reflect the changes in glucose homeostasis throughout pregnancy.
The lack of effect was evident in both the ITT and the per-protocol analyses. Our study was adequately powered and we can conclude that our results reflect a true lack of effect of the intervention, rather than a type 2 error.
Effectiveness and acceptability of the intervention
Despite the lack of an effect on our primary outcome, we believe that metformin had its expected pharmacodynamic effect given the differences in measures of insulin sensitivity at 28 weeks’ gestation.
Recruitment to the trial was challenging. The majority of women declined to participate. We were unable to formally assess the reasons for this but anecdotally there is an understandable reluctance among pregnant women to take medication in pregnancy and also a lack of awareness of the potential harm associated with obesity in pregnancy.
The intervention was acceptable to the women who agreed to participate in the trial. No participants were withdrawn specifically because of treatment side effects. Overall adherence was around 60% by both diary entries and detectable levels of metformin in the 36-week blood sample. The median dose taken was 2000 g, which suggests that the treatment regimen was acceptable to most participants.
Strengths and limitations
This was a multicentre study with a double-blind, randomised controlled design, making the findings robust and generalisable. Despite challenges with recruitment, we were still able to recruit our target sample size and we had adequate power to address our hypothesis.
We have used a recognised surrogate, birthweight centile, as a marker of future life risk of obesity in the offspring. A limitation of the study is that follow-up was limited to the early postnatal period and longer-term conclusions about the effect of metformin on the offspring will require long-term follow-up studies. Additionally, the large number of secondary outcomes means that conclusions about these results (even when p < 0.05) are potentially subject to a type 1 error.
We did not attempt to assess whether or not masking/blinding was effective. Metformin may cause gastrointestinal side effects and so it is possible that some women (and their caregivers) may have correctly inferred their treatment allocation from their side effect profile. However, the majority of the clinical outcomes (e.g. birthweight centile) are unlikely to be significantly affected by observer bias and so we do not think that this will have adversely affected the results.
We used a starting dose of metformin of 500 mg and a maximum dose of 2000 mg and up-titrated by 500 mg per week. In the MOP study,19 the starting dose was 1000 mg, the maximum dose was 3000 mg and the up-titration rate was similar. In clinical practice, some clinicians up-titrate more rapidly. It is possible that different dose regimens may have produced a different result, although the MOP study also did not demonstrate any effect of metformin on the primary outcome of birthweight centile. 19
As part of our study protocol we performed a glucose tolerance test at baseline (12–16 weeks) and excluded those who fulfilled the criteria for GDM. We anticipated that women diagnosed with GDM after enrolment would wish to withdraw and be treated with metformin as a first-line agent for their GDM. Hence, a glucose tolerance test at baseline would prevent withdrawals (and protocol violations) by identifying early women who would later be diagnosed with GDM. In practice, however, our approach has likely excluded women who are most likely to have a macrosomic baby. Glucose tolerance tests are not performed until 28 weeks in current clinical practice and so most women ultimately diagnosed with GDM have 28 weeks of pregnancy during which their babies are exposed to high levels of maternal glucose. Such women were excluded from our study.
Difficulties with recruitment reported by staff were twofold. First, although we were formally unable to quantify this, our impression was that women felt stigmatised at being identified as obese and immediately rejected the study on that basis.
Second, it was difficult to explain to potential participants the risks of maternal obesity and high birthweight for their offspring. Neither outcome was seen by women as an adverse outcome and so the concept of taking a tablet to prevent this was insufficient to overturn the generic advice not to take medications in pregnancy unless absolutely necessary.
Our study was restricted to Caucasian women to minimise the effect of ethnicity on birthweight. Over 30% of participants in the MOP study were of other ethnic groups,19 but again no effect of metformin was seen on birthweight.
Implications for health care and recommendations for future research
Obesity is a major public health concern of our time. Rates of obesity among young women of reproductive age are ever increasing and the cycle of disadvantage is thus being perpetuated to the next generation. On the basis of this research, we can conclude that metformin should not be used to improve pregnancy outcomes in obese pregnant women without GDM. Follow-up studies of the babies born to the women who participated in the EMPOWaR trial will determine whether or not there are any longer-term benefits (or indeed harms) of metformin taken during pregnancy. Our findings of no beneficial effect are similar to those of other trials of various dietary and lifestyle interventions aimed at reducing birthweight in obese individuals. An alternative approach would be to optimise the diagnosis of GDM in obese pregnant women. Although national recommendations are that obese women should have a glucose tolerance test at 28 weeks to test for GDM, these recommendations are incompletely applied. Additionally, given that glucose levels are high in obese women from the beginning of pregnancy, deferring diagnosis until 28 weeks allows high maternal glucose to impact adversely on fetal growth for the first two-thirds of pregnancy. Hence, earlier diagnoses for GDM might be appropriate. It seems that the focus of intervention must shift towards reducing weight and optimising health in young girls and women prior to embarking on pregnancy – arguably a much greater challenge. Increasing awareness among the general public of the impact of obesity on both immediate and long-term pregnancy outcomes must also be addressed.
Acknowledgements
This study would not have been possible without the commitment of the pregnant women who participated and we are enormously grateful to them.
We are grateful to the members of the Trial Steering Committee – Siladitya Bhattacharya (chairperson), Robbie Lindsay and Gary Mires – and the Data Monitoring Committee – Peter Brocklehurst (chairperson), Graeme MacLennan, Andrew Thomson and Catherine Williamson – who generously gave their time to support the study.
We are also grateful to the principal investigators: Derek Tuffnell (Bradford), Aamod Nawathe (Chelsea and Westminster), Janet Cresswell and Santhi Chidambaram (Chesterfield), George Bugg (Nottingham), Fiona Crosfill (Preston), Tom Farrell (Sheffield), Cally Nwosu, Sandhya Rao, Nidhi Srivastava and Vicky Cording (Whiston); the study midwives: Julie Grindey (Arrowe Park), Diane Farrar, Vicky Jones, Jennifer Syson, Gillian Butterfield, Rebecca Palethorpe and Tracey Germaine-Rylance (Bradford), Kathryn McCormick and Sarah Ladd (Chelsea and Westminster), Mary Kelly-Baxter, Louise Underwood and Claire Wood (Chesterfield), Lynsdey Prue and Natasha Kaba (Coventry), Jennifer Devlin, Yvonne Grieg, Hayley Moir, Alice Keeley and Mary Simpson (Edinburgh), Falak Diab, Caroline Cunningham and Katherine Day (Liverpool Women’s Hospital), Natasha Singh and Suzanne Ridley (Imperial), Yvonne Toomassi, Yvette Davis and Yvette Gunn (Nottingham), Katrina Rigby (Preston), Pam Inniss (Royal Blackburn), Hilary Rosser, Siobhan Gillespie, Sarah Senbeto, Alison Carey and Anne Chamberlain (Sheffield), Jane Hillen and Debbie Bullen (University Hospitals Coventry and Warwickshire), Lauren Lacey (University College London), Zoe Grindlay (Whitson); and the pharmacists: Kathryn Hayes (Blackburn), Martin Shepherd (Chestefield), Hazel Milligan (Edinburgh), Elaine Willis (Liverpool Women’s Hospital), Margaret Hargreaves (Whitson) and Louise Hough (Preston).
We are grateful to Nanette Hibbert, Graham Harrold, Rose Leask, Ana Calarrao, Forbes Howie and Linda Nicol for providing expert technical assistance; to Ruth Andrew, Natalie Homer and Sanjay Kothiya for their expertise in gas chromatography mass spectrometry; and to Karen Noble for carrying out the myometrial work. We are grateful to the staff at the Clinical Research Imaging Centre, Edinburgh, particularly Annette Cooper (senior magnetic resonance radiographer) and her team for carrying out the MRI scans and Dr Jane Walker (consultant radiologist) for reviewing the scans for clinical reporting. We are also grateful to Allan Walker and Garry Milne for their help in generating the database and Lorraine Adamson, Tariq Derdeb and Carolyn Newton for excellent administrative assistance. Finally, we are grateful to the administrators in participating NHS research and development departments and to all others who have helped with the study but who we have been unable to name.
Funding
This study was funded by the Efficacy and Mechanism Evaluation (EME) programme, a Medical Research Council (MRC) and NIHR partnership (grant reference number 08/246/09). The EME programme is funded by the MRC and NIHR, with contributions from the Chief Scientist Office in Scotland and National Institute for Social Care and Health Research in Wales and the Health and Social Care Research and Development Division, Public Health Agency in Northern Ireland.
Active and placebo treatments were supplied without charge by Merck KGaA and we are grateful to them. Neither the funder nor the supplier of the active and placebo drugs had any role in study design, data collection, data analysis, data interpretation or writing of the report. The joint study sponsor in terms of the European Union Clinical Trials Directive was the University of Edinburgh and NHS Lothian and had no role in analysis of the data. The corresponding author had full access to all of the data in the study and had final responsibility for the decision to submit for publication.
Contributions of authors
Carolyn A Chiswick wrote the manuscript. Carolyn A Chiswick, Rebecca M Reynolds, Fiona C Denison, Amanda J Drake, Shareen Forbes, David E Newby, Brian R Walker, Siobhan Quenby, Andrew Weeks, Hany Lashen, Sonia Whyte, Natalie Homer and Jane E Norman acquired the data. Rebecca M Reynolds, Fiona C Denison, Amanda J Drake, Shareen Forbes, David E Newby, Brian R Walker, Siobhan Quenby, Susan Wray, Gordon D Murray, Sonia Whyte and Jane E Norman designed the study. Susan Wray and Karen Noble carried out the analysis and interpretation of the myometrial contractility and glycogen storage studies. Aryelly Rodriguez and Gordon D Murray analysed the data. Ruth Andrew provided expertise in analysis and interpretation of the gas chromatography–mass spectrometry and liquid chromatography–mass spectrometry data. Scott Semple and Calum Gray provided expertise for the analysis and interpretation of the MRI data. Marian C Aldhous carried out the analysis for the placental 11β-HSD1 and 2 and glucocorticoid receptor studies. Sarah Cunningham-Burley and Alice Keely were responsible for the qualitative study. Jane E Norman conceived the study. All authors interpreted the data, revised the manuscript critically for important intellectual content and approved the final version.
Publications
Norman JE. The adverse effects of obesity on reproduction. Reproduction 2010;140:343–5.
Norman JE, Reynolds RM. The consequences of obesity and excess weight gain in pregnancy. Proc Nutr Soc 2011;70:450–6.
Chiswick C, Reynolds RM, Denison F, Drake AJ, Forbes S, Newby DE, et al. Effect of metformin on maternal and fetal outcomes in obese pregnant women (EMPOWaR): a randomised, double-blind, placebo-controlled trial. Lancet Diabetes Endocrinol 2015;3:778–86.
Chiswick CA, Reynolds RM, Denison FC, Whyte SA, Drake AJ, Newby DE, et al. Efficacy of metformin in pregnant obese woman: a randomised controlled trial. BMJ Open 2015;5:e006854.
Data sharing statement
All available data can be obtained from the corresponding author.
Disclaimers
This report presents independent research. The views and opinions expressed by authors in this publication are those of the authors and do not necessarily reflect those of the NHS, the NIHR, the MRC, NETSCC, the EME programme or the Department of Health. If there are verbatim quotations included in this publication the views and opinions expressed by the interviewees are those of the interviewees and do not necessarily reflect those of the authors, those of the NHS, the NIHR, NETSCC, the EME programme or the Department of Health.
References
- Chu SY, Kim SY, Lau J, Schmid CH, Dietz PM, Callaghan WM, et al. Maternal obesity and risk of stillbirth: a metaanalysis. Am J Obstet Gynecol 2007;197:223-8. http://dx.doi.org/10.1016/j.ajog.2007.03.027.
- Kristensen J, Vestergaard M, Wisborg K, Kesmodel U, Secher NJ. Pre-pregnancy weight and the risk of stillbirth and neonatal death. BJOG 2005;112:403-8. http://dx.doi.org/10.1111/j.1471-0528.2005.00437.x.
- O’Brien TE, Ray JG, Chan WS. Maternal body mass index and the risk of preeclampsia: a systematic overview. Epidemiology 2003;14:368-74. http://dx.doi.org/10.1097/01.EDE.0000059921.71494.D1.
- Sebire NJ, Jolly M, Harris JP, Wadsworth J, Joffe M, Beard RW, et al. Maternal obesity and pregnancy outcome: a study of 287,213 pregnancies in London. Int J Obes Relat Metab Disord 2001;25:1175-82. http://dx.doi.org/10.1038/sj.ijo.0801670.
- Curhan GC, Chertow GM, Willett WC, Spiegelman D, Colditz GA, Manson JE, et al. Birth weight and adult hypertension and obesity in women. Circulation 1996;94:1310-15. http://dx.doi.org/10.1161/01.CIR.94.6.1310.
- Curhan GC, Willett WC, Rimm EB, Spiegelman D, Ascherio AL, Stampfer MJ. Birth weight and adult hypertension, diabetes mellitus, and obesity in US men. Circulation 1996;94:3246-50. http://dx.doi.org/10.1161/01.CIR.94.12.3246.
- Parsons TJ, Power C, Logan S, Summerbell CD. Childhood predictors of adult obesity: a systematic review. Int J Obes Relat Metab Disord 1999;23:1-107.
- Reynolds RM, Allan KM, Raja EA, Bhattacharya S, McNeill G, Hannaford PC, et al. Maternal obesity during pregnancy and premature mortality from cardiovascular event in adult offspring: follow-up of 1 323 275 person years. BMJ 2013;347. http://dx.doi.org/10.1136/bmj.f4539.
- Catalano PM, Ehrenberg HM. The short- and long-term implications of maternal obesity on the mother and her offspring. BJOG 2006;113:1126-33. http://dx.doi.org/10.1111/j.1471-0528.2006.00989.x.
- Catalano PM, Thomas A, Huston-Presley L, Amini SB. Increased fetal adiposity: a very sensitive marker of abnormal in utero development. Am J Obstet Gynecol 2003;189:1698-704. http://dx.doi.org/10.1016/S0002-9378(03)00828-7.
- Metzger BE, Lowe LP, Dyer AR, Trimble ER, Chaovarindr U, Coustan DR, et al. Hyperglycemia and adverse pregnancy outcomes. N Engl J Med 2008;358:1991-2002. http://dx.doi.org/10.1056/NEJMoa0707943.
- Crowther CA, Hiller JE, Moss JR, McPhee AJ, Jeffries WS, Robinson JS. Australian Carbohydrate Intolerance Study in Pregnant Women (ACHOIS) Trial Group . Effect of treatment of gestational diabetes mellitus on pregnancy outcomes. N Engl J Med 2005;352:2477-86. http://dx.doi.org/10.1056/NEJMoa042973.
- Boney CM, Verma A, Tucker R, Vohr BR. Metabolic syndrome in childhood: association with birth weight, maternal obesity, and gestational diabetes mellitus. Pediatrics 2005;115:e290-6. http://dx.doi.org/10.1542/peds.2004-1808.
- Dodd JM, Grivell RM, Crowther CA, Robinson JS. Antenatal interventions for overweight or obese pregnant women: a systematic review of randomised trials. BJOG 2010;117:1316-26. http://dx.doi.org/10.1111/j.1471-0528.2010.02540.x.
- Oteng-Ntim E, Varma R, Croker H, Poston L, Doyle P. Lifestyle interventions for overweight and obese pregnant women to improve pregnancy outcome: systematic review and meta-analysis. BMC Med 2012;10. http://dx.doi.org/10.1186/1741-7015-10-47.
- Dodd JM, Turnbull D, McPhee AJ, Deussen AR, Grivell RM, Yelland LN, et al. Antenatal lifestyle advice for women who are overweight or obese: LIMIT randomised trial. BMJ 2014;348. http://dx.doi.org/10.1136/bmj.g1285.
- Vinter CA, Jensen DM, Ovesen P, Beck-Nielsen H, Jørgensen JS. The LiP (Lifestyle in Pregnancy) study: a randomized controlled trial of lifestyle intervention in 360 obese pregnant women. Diabetes Care 2011;34:2502-7. http://dx.doi.org/10.2337/dc11-1150.
- Poston L, Bell R, Croker H, Flynn AC, Godfrey KM, Goff L, et al. Effect of a behavioural intervention in obese pregnant women (the UPBEAT study): a multicentre, randomised controlled trial. Lancet Diabetes Endocrinol 2015;3:767-77. http://dx.doi.org/10.1016/S2213-8587(15)00227-2.
- Syngelaki A, Nicolaides KH, Balani J, Hyer S, Akolekar R, Kotecha R, et al. Metformin versus placebo in obese pregnant women without diabetes mellitus. N Engl J Med 2016;374:434-43. http://dx.doi.org/10.1056/NEJMoa1509819.
- National Institute for Health and Care Excellence . Diabetes in Pregnancy: Management from Preconception to the Postnatal Period n.d. www.nice.org.uk/guidance/ng3 (accessed 12 July 2016).
- Balsells M, García-Patterson A, Solà I, Roqué M, Gich I, Corcoy R. Glibenclamide, metformin, and insulin for the treatment of gestational diabetes: a systematic review and meta-analysis. BMJ 2015;350. http://dx.doi.org/10.1136/bmj.h102.
- Zhao LP, Sheng XY, Zhou S, Yang T, Ma LY, Zhou Y, et al. Metformin versus insulin for gestational diabetes mellitus: a meta-analysis. Br J Clin Pharmacol 2015;80:1224-34. http://dx.doi.org/10.1111/bcp.12672.
- Singh KP, Rahimpanah F, Barclay M. Metformin for the management of gestational diabetes mellitus. Aust N Z J Obstet Gynaecol 2015;55:303-8. http://dx.doi.org/10.1111/ajo.12311.
- Beyuo T, Obed SA, Adjepong-Yamoah KK, Bugyei KA, Oppong SA, Marfoh K. Metformin versus insulin in the management of pre-gestational diabetes mellitus in pregnancy and gestational diabetes mellitus at the Korle Bu teaching hospital: a randomized clinical trial. PLOS ONE 2015;10. http://dx.doi.org/10.1371/journal.pone.0125712.
- George A, Mathews JE, Sam D, Beck M, Benjamin SJ, Abraham A, et al. Comparison of neonatal outcomes in women with gestational diabetes with moderate hyperglycaemia on metformin or glibenclamide – a randomised controlled trial. Aust N Z J Obstet Gynaecol 2015;55:47-52. http://dx.doi.org/10.1111/ajo.12276.
- Vanky E, Salvesen KA, Heimstad R, Fougner KJ, Romundstad P, Carlsen SM. Metformin reduces pregnancy complications without affecting androgen levels in pregnant polycystic ovary syndrome women: results of a randomized study. Hum Reprod 2004;19:1734-40. http://dx.doi.org/10.1093/humrep/deh347.
- Vanky E, Stridsklev S, Heimstad R, Romundstad P, Skogøy K, Kleggetveit O, et al. Metformin versus placebo from first trimester to delivery in polycystic ovary syndrome: a randomized, controlled multicenter study. J Clin Endocrinol Metab 2010;95:E448-55. http://dx.doi.org/10.1210/jc.2010-0853.
- Chiswick C, Reynolds RM, Denison F, Drake AJ, Forbes S, Newby DE, et al. Effect of metformin on maternal and fetal outcomes in obese pregnant women (EMPOWaR): a randomised, double-blind, placebo-controlled trial. Lancet Diabetes Endocrinol 2015;3:778-86. http://dx.doi.org/10.1016/S2213-8587(15)00219-3.
- Chiswick CA, Reynolds RM, Denison FC, Whyte SA, Drake AJ, Newby DE, et al. Efficacy of metformin in pregnant obese women: a randomised controlled trial. BMJ Open 2015;5. http://dx.doi.org/10.1136/bmjopen-2014-006854.
- Directive 2001/20/EC of the European Parliament and of the Council of 4 April 2001 on the Approximation of the Laws, Regulations and Administrative Provisions of the Member States Relating to the Implementation of Good Clinical Practice in the Conduct of Clinical Trials on Medicinal Products for Human Use. Official Journal of the European Communities. Brussels: European Commission; 2001.
- Diagnostic Criteria and Classification of Hyperglycaemia First Detected in Pregnancy. 2013.
- International Association of Diabetes and Pregnancy Study Groups Consensus Panel . International Association of Diabetes and Pregnancy Study Groups Recommendations on the diagnosis and classification of hyperglycemia in pregnancy. Diabetes Care 2010;33:676-82. http://dx.doi.org/10.2337/dc09-1848.
- Bonellie S, Chalmers J, Gray R, Greer I, Jarvis S, Williams C. Centile charts for birthweight for gestational age for Scottish singleton births. BMC Pregnancy Childbirth 2008;8. http://dx.doi.org/10.1186/1471-2393-8-5.
- Lindsay CA, Huston L, Amini SB, Catalano PM. Longitudinal changes in the relationship between body mass index and percent body fat in pregnancy. Obstet Gynecol 1997;89:377-82. http://dx.doi.org/10.1016/S0029-7844(96)00517-0.
- Sewell MF, Huston-Presley L, Amini SB, Catalano PM. Body mass index: a true indicator of body fat in obese gravidas. J Reprod Med 2007;52:907-11.
- Hull HR, Dinger MK, Knehans AW, Thompson DM, Fields DA. Impact of maternal body mass index on neonate birthweight and body composition. Am J Obstet Gynecol 2008;198:416.e1-6. http://dx.doi.org/10.1016/j.ajog.2007.10.796.
- Sewell MF, Huston-Presley L, Super DM, Catalano P. Increased neonatal fat mass, not lean body mass, is associated with maternal obesity. Am J Obstet Gynecol 2006;195:1100-3. http://dx.doi.org/10.1016/j.ajog.2006.06.014.
- Fields DA, Gunatilake R, Kalaitzoglou E. Air displacement plethysmography: cradle to grave. Nutr Clin Pract 2015;30:219-26. http://dx.doi.org/10.1177/0884533615572443.
- Rowan JA, Hague WM, Gao W, Battin MR, Moore MP. MiG Trial Investigators . Metformin versus insulin for the treatment of gestational diabetes. N Engl J Med 2008;358:2003-15. http://dx.doi.org/10.1056/NEJMoa0707193.
- Rowan JA, Rush EC, Obolonkin V, Battin M, Wouldes T, Hague WM. Metformin in gestational diabetes: the offspring follow-up (MiG TOFU): body composition at 2 years of age. Diabetes Care 2011;34:2279-84. http://dx.doi.org/10.2337/dc11-0660.
- Mills JL, Jovanovic L, Knopp R, Aarons J, Conley M, Park E, et al. Physiological reduction in fasting plasma glucose concentration in the first trimester of normal pregnancy: the diabetes in early pregnancy study. Metab Clin Exp 1998;47:1140-4. http://dx.doi.org/10.1016/S0026-0495(98)90290-6.
- Catalano PM, Huston L, Amini SB, Kalhan SC. Longitudinal changes in glucose metabolism during pregnancy in obese women with normal glucose tolerance and gestational diabetes mellitus. Am J Obstet Gynecol 1999;180:903-16. http://dx.doi.org/10.1016/S0002-9378(99)70662-9.
- Catalano PM, Tyzbir ED, Wolfe RR, Calles J, Roman NM, Amini SB, et al. Carbohydrate metabolism during pregnancy in control subjects and women with gestational diabetes. Am J Physiol 1993;2644:E60-7.
- Catalano PM, Tyzbir ED, Wolfe RR, Roman NM, Amini SB, Sims EA. Longitudinal changes in basal hepatic glucose production and suppression during insulin infusion in normal pregnant women. Am J Obstet Gynecol 1992;167:913-19. http://dx.doi.org/10.1016/S0002-9378(12)80011-1.
- Catalano PM, Tyzbir ED, Roman NM, Amini SB, Sims EA. Longitudinal changes in insulin release and insulin resistance in nonobese pregnant women. Am J Obstet Gynecol 1991;165:1667-72. http://dx.doi.org/10.1016/0002-9378(91)90012-G.
- Assel B, Rossi K, Kalhan S. Glucose metabolism during fasting through human pregnancy: comparison of tracer method with respiratory calorimetry. Am J Physiol 1993;265:351-6.
- Kalhan S, Rossi K, Gruca L, Burkett E, O’Brien A. Glucose turnover and gluconeogenesis in human pregnancy. J Clin Invest 1997;100:1775-81. http://dx.doi.org/10.1172/JCI119704.
- Kalhan SC, D’Angelo LJ, Savin SM, Adam PA. Glucose production in pregnant women at term gestation. Sources of glucose for human fetus. J Clin Invest 1979;63:388-94. http://dx.doi.org/10.1172/JCI109314.
- Homko CJ, Sivan E, Reece EA, Boden G. Fuel metabolism during pregnancy. Semin Reprod Endocrinol 1999;17:119-25. http://dx.doi.org/10.1055/s-2007-1016219.
- Endo S, Maeda K, Suto M, Kaji T, Morine M, Kinoshita T, et al. Differences in insulin sensitivity in pregnant women with overweight and gestational diabetes mellitus. Gynecol Endocrinol 2006;22:343-9. http://dx.doi.org/10.1080/09513590600724836.
- Sivan E, Chen X, Homko CJ, Reece EA, Boden G. Longitudinal study of carbohydrate metabolism in healthy obese pregnant women. Diabetes Care 1997;20:1470-5. http://dx.doi.org/10.2337/diacare.20.9.1470.
- Forbes S, Barr SM, Reynolds RM, Semple S, Gray C, Andrew R, et al. Convergence in insulin resistance between very severely obese and lean women at the end of pregnancy. Diabetologia 2015;58:2615-26. http://dx.doi.org/10.1007/s00125-015-3708-3.
- Upreti R, Hughes KA, Livingstone DE, Gray CD, Minns FC, Macfarlane DP, et al. 5α-reductase type 1 modulates insulin sensitivity in men. J Clin Endocrinol Metab 2014;99:E1397-406. http://dx.doi.org/10.1210/jc.2014-1395.
- Giannarelli R, Aragona M, Coppelli A, Del Prato S. Reducing insulin resistance with metformin: the evidence today. Diabetes Metab 2003;29:6S28-35.
- Goodarzi MO, Bryer-Ash M. Metformin revisited: re-evaluation of its properties and role in the pharmacopoeia of modern antidiabetic agents. Diabetes Obes Metab 2005;7:654-65. http://dx.doi.org/10.1111/j.1463-1326.2004.00448.x.
- Inzucchi SE, Maggs DG, Spollett GR, Page SL, Rife FS, Walton V, et al. Efficacy and metabolic effects of metformin and troglitazone in type II diabetes mellitus. N Engl J Med 1998;338:867-72. http://dx.doi.org/10.1056/NEJM199803263381303.
- Christensen MM, Hojlund K, Hother-Nielsen O, Stage TB, Damkier P, Beck-Nielsen H, et al. Endogenous glucose production increases in response to metformin treatment in the glycogen-depleted state in humans: a randomised trial. Diabetologia 2015;58:2494-502. http://dx.doi.org/10.1007/s00125-015-3733-2.
- Diderholm B, Stridsberg M, Nordén-Lindeberg S, Gustafsson J. Decreased maternal lipolysis in intrauterine growth restriction in the third trimester. BJOG 2006;113:159-64. http://dx.doi.org/10.1111/j.1471-0528.2005.00825.x.
- Kannel WB, Brand N, Skinner JJ, Dawber TR, McNamara PM. The relation of adiposity to blood pressure and development of hypertension. The Framingham study. Ann Intern Med 1967;67:48-59. http://dx.doi.org/10.7326/0003-4819-67-1-48.
- Fisher JJ, Frey I. Pregnancy and parturition in the obese patient. Obstet Gynecol 1958;11:92-4.
- Cedergren MI. Maternal morbid obesity and the risk of adverse pregnancy outcome. Obstet Gynecol 2004;103:219-24. http://dx.doi.org/10.1097/01.AOG.0000107291.46159.00.
- Bhattacharya S, Campbell DM, Liston WA, Bhattacharya S. Effect of body mass index on pregnancy outcomes in nulliparous women delivering singleton babies. BMC Public Health 2007;7. http://dx.doi.org/10.1186/1471-2458-7-168.
- Seely EW, Ecker J. Clinical practice. Chronic hypertension in pregnancy. N Engl J Med 2011;365:439-46. http://dx.doi.org/10.1056/NEJMcp0804872.
- Jensen DM, Ovesen P, Beck-Nielsen H, Mølsted-Pedersen L, Sørensen B, Vinter C, et al. Gestational weight gain and pregnancy outcomes in 481 obese glucose-tolerant women. Diabetes Care 2005;28:2118-22. http://dx.doi.org/10.2337/diacare.28.9.2118.
- Duckitt K, Harrington D. Risk factors for pre-eclampsia at antenatal booking: systematic review of controlled studies. BMJ 2005;330. http://dx.doi.org/10.1136/bmj.38380.674340.E0.
- Libby G, Murphy DJ, McEwan NF, Greene SA, Forsyth JS, Chien PW, et al. Pre-eclampsia and the later development of type 2 diabetes in mothers and their children: an intergenerational study from the Walker cohort. Diabetologia 2007;50:523-30. http://dx.doi.org/10.1007/s00125-006-0558-z.
- Stewart FM, Freeman DJ, Ramsay JE, Greer IA, Caslake M, Ferrell WR. Longitudinal assessment of maternal endothelial function and markers of inflammation and placental function throughout pregnancy in lean and obese mothers. J Clin Endocrinol Metab 2007;92:969-75. http://dx.doi.org/10.1210/jc.2006-2083.
- Sattar N, Gaw A, Packard CJ, Greer IA. Potential pathogenic roles of aberrant lipoprotein and fatty acid metabolism in pre-eclampsia. Br J Obstet Gynaecol 1996;103:614-20. http://dx.doi.org/10.1111/j.1471-0528.1996.tb09827.x.
- Roberts JM, Bodnar LM, Patrick TE, Powers RW. The role of obesity in preeclampsia. Pregnancy Hypertens 2011;1:6-16. http://dx.doi.org/10.1016/j.preghy.2010.10.013.
- Vita JA, Keaney JF. Endothelial function: a barometer for cardiovascular risk?. Circulation 2002;106:640-2. http://dx.doi.org/10.1161/01.CIR.0000028581.07992.56.
- Endemann DH, Schiffrin EL. Endothelial dysfunction. J Am Soc Nephrol 2004;15:1983-92. http://dx.doi.org/10.1097/01.ASN.0000132474.50966.DA.
- Ross R. The pathogenesis of atherosclerosis: a perspective for the 1990s. Nature 1993;362:801-9. http://dx.doi.org/10.1038/362801a0.
- Engler MM, Engler MB, Malloy MJ, Chiu EY, Schloetter MC, Paul SM, et al. Antioxidant vitamins C and E improve endothelial function in children with hyperlipidemia: Endothelial Assessment of Risk from Lipids in Youth (EARLY) trial. Circulation 2003;108:1059-63. http://dx.doi.org/10.1161/01.CIR.0000086345.09861.A0.
- Raitakari M, Ilvonen T, Ahotupa M, Lehtimäki T, Harmoinen A, Suominen P, et al. Weight reduction with very-low-caloric diet and endothelial function in overweight adults: role of plasma glucose. Arterioscler Thromb Vasc Biol 2004;24:124-8. http://dx.doi.org/10.1161/01.ATV.0000109749.11042.7c.
- Poston L. Endothelial dysfunction in pre-eclampsia. Pharmacol Rep 2006;58:69-74.
- Di Fulvio P, Pandolfi A, Formoso G, Di Silvestre S, Di Tomo P, Giardinelli A, et al. Features of endothelial dysfunction in umbilical cord vessels of women with gestational diabetes. Nutr Metab Cardiovasc Dis 2014;24:1337-45. http://dx.doi.org/10.1016/j.numecd.2014.06.005.
- Ramsay JE, Ferrell WR, Crawford L, Wallace AM, Greer IA, Sattar N. Maternal obesity is associated with dysregulation of metabolic, vascular, and inflammatory pathways. J Clin Endocrinol Metab 2002;87:4231-7. http://dx.doi.org/10.1210/jc.2002-020311.
- Scarpello JH, Howlett HC. Metformin therapy and clinical uses. Diab Vasc Dis Res 2008;5:157-67. http://dx.doi.org/10.3132/dvdr.2008.027.
- Nagi DK, Yudkin JS. Effects of metformin on insulin resistance, risk factors for cardiovascular disease, and plasminogen activator inhibitor in NIDDM subjects. A study of two ethnic groups. Diabetes Care 1993;16:621-9. http://dx.doi.org/10.2337/diacare.16.4.621.
- Effect of intensive blood-glucose control with metformin on complications in overweight patients with type 2 diabetes (UKPDS 34) . UK Prospective Diabetes Study (UKPDS) Group. Lancet 1998;352:854-65. http://dx.doi.org/10.1016/S0140-6736(98)07037-8.
- Vitale C, Mercuro G, Cornoldi A, Fini M, Volterrani M, Rosano GM. Metformin improves endothelial function in patients with metabolic syndrome. J Intern Med 2005;258:250-6. http://dx.doi.org/10.1111/j.1365-2796.2005.01531.x.
- Diamanti-Kandarakis E, Alexandraki K, Protogerou A, Piperi C, Papamichael C, Aessopos A, et al. Metformin administration improves endothelial function in women with polycystic ovary syndrome. Eur J Endocrinol 2005;152:749-56. http://dx.doi.org/10.1530/eje.1.01910.
- Pitocco D, Zaccardi F, Tarzia P, Milo M, Scavone G, Rizzo P, et al. Metformin improves endothelial function in type 1 diabetic subjects: a pilot, placebo-controlled randomized study. Diabetes Obes Metab 2013;15:427-31. http://dx.doi.org/10.1111/dom.12041.
- Dørup I, Skajaa K, Sørensen KE. Normal pregnancy is associated with enhanced endothelium-dependent flow-mediated vasodilation. Am J Physiol 1999;276:H821-5.
- Savvidou MD, Kametas NA, Donald AE, Nicolaides KH. Non-invasive assessment of endothelial function in normal pregnancy. Ultrasound Obstet Gynecol 2000;15:502-7. http://dx.doi.org/10.1046/j.1469-0705.2000.00131.x.
- Nelson-Piercy C. Handbook of Obstetric Medicine. London: Informa Healthcare; 2007.
- Hashimoto M, Akishita M, Eto M, Ishikawa M, Kozaki K, Toba K, et al. Modulation of endothelium-dependent flow-mediated dilatation of the brachial artery by sex and menstrual cycle. Circulation 1995;92:3431-5. http://dx.doi.org/10.1161/01.CIR.92.12.3431.
- Lieberman EH, Gerhard MD, Uehata A, Walsh BW, Selwyn AP, Ganz P, et al. Estrogen improves endothelium-dependent, flow-mediated vasodilation in postmenopausal women. Ann Intern Med 1994;121:936-41. http://dx.doi.org/10.7326/0003-4819-121-12-199412150-00005.
- Williams DJ, Vallance PJ, Neild GH, Spencer JA, Imms FJ. Nitric oxide-mediated vasodilation in human pregnancy. Am J Physiol 1997;272:H748-52.
- Celermajer DS, Sorensen KE, Gooch VM, Spiegelhalter DJ, Miller OI, Sullivan ID, et al. Non-invasive detection of endothelial dysfunction in children and adults at risk of atherosclerosis. Lancet 1992;340:1111-15. http://dx.doi.org/10.1016/0140-6736(92)93147-F.
- Canoy D, Boekholdt SM, Wareham N, Luben R, Welch A, Bingham S, et al. Body fat distribution and risk of coronary heart disease in men and women in the European prospective investigation into cancer and nutrition in Norfolk cohort: a population-based prospective study. Circulation 2007;116:2933-43. http://dx.doi.org/10.1161/CIRCULATIONAHA.106.673756.
- Kaess BM, Pedley A, Massaro JM, Murabito J, Hoffmann U, Fox CS. The ratio of visceral to subcutaneous fat, a metric of body fat distribution, is a unique correlate of cardiometabolic risk. Diabetologia 2012;55:2622-30. http://dx.doi.org/10.1007/s00125-012-2639-5.
- Kissebah AH, Krakower GR. Regional adiposity and morbidity. Physiol Rev 1994;74:761-81.
- Lapidus L, Bengtsson C, Larsson B, Pennert K, Rybo E, Sjöström L. Distribution of adipose tissue and risk of cardiovascular disease and death: a 12 year follow up of participants in the population study of women in Gothenburg, Sweden. Br Med J 1984;289:1257-61. http://dx.doi.org/10.1136/bmj.289.6454.1257.
- Bozzetto L, Prinster A, Mancini M, Giacco R, De Natale C, Salvatore M, et al. Liver fat in obesity: role of type 2 diabetes mellitus and adipose tissue distribution. Eur J Clin Invest 2011;41:39-44. http://dx.doi.org/10.1111/j.1365-2362.2010.02372.x.
- Goodpaster BH, Wolf D. Skeletal muscle lipid accumulation in obesity, insulin resistance, and type 2 diabetes. Pediatr Diabetes 2004;5:219-26. http://dx.doi.org/10.1111/j.1399-543X.2004.00071.x.
- Björntorp P. ‘Portal’ adipose tissue as a generator of risk factors for cardiovascular disease and diabetes. Arteriosclerosis 1990;10:493-6. http://dx.doi.org/10.1161/01.ATV.10.4.493.
- Jakobsen MU, Berentzen T, Sørensen TI, Overvad K. Abdominal obesity and fatty liver. Epidemiol Rev 2007;29:77-8. http://dx.doi.org/10.1093/epirev/mxm002.
- Siega-Riz AM, Herring AH, Carrier K, Evenson KR, Dole N, Deierlein A. Sociodemographic, perinatal, behavioral, and psychosocial predictors of weight retention at 3 and 12 months postpartum. Obesity 2010;18:1996-2003. http://dx.doi.org/10.1038/oby.2009.458.
- Rasmussen KM, Abrams B, Bodnar LM, Butte NF, Catalano PM, Maria Siega-Riz A. Recommendations for weight gain during pregnancy in the context of the obesity epidemic. Obstet Gynecol 2010;116:1191-5. http://dx.doi.org/10.1097/AOG.0b013e3181f60da7.
- Sohlström A, Wahlund LO, Forsum E. Total body fat and its distribution during human reproduction as assessed by magnetic resonance imaging. Basic Life Sci 1993;60:181-4. http://dx.doi.org/10.1007/978-1-4899-1268-8_41.
- Stevens-Simon C, Thureen P, Barrett J, Stamm E. Skinfold caliper and ultrasound assessments of change in the distribution of subcutaneous fat during adolescent pregnancy. Int J Obes Relat Metab Disord 2001;25:1340-5. http://dx.doi.org/10.1038/sj.ijo.0801685.
- Soltani H, Fraser RB. A longitudinal study of maternal anthropometric changes in normal weight, overweight and obese women during pregnancy and postpartum. Br J Nutr 2000;84:95-101. http://dx.doi.org/10.1017/S0007114500001276.
- Landon MB, Mintz MC, Gabbe SG. Sonographic evaluation of fetal abdominal growth: predictor of the large-for-gestational-age infant in pregnancies complicated by diabetes mellitus. Am J Obstet Gynecol 1989;160:115-21. http://dx.doi.org/10.1016/0002-9378(89)90101-4.
- Wladimiroff JW, Bloemsma CA, Wallenburg HC. Ultrasonic diagnosis of the large-for-dates infant. Obstet Gynecol 1978;52:285-8.
- Evans MI, Mukherjee AB, Schulman JD. Animal models of intrauterine growth retardation. Obstet Gynecol Surv 1983;38:183-92. http://dx.doi.org/10.1097/00006254-198304000-00001.
- Vintzileos AM, Neckles S, Campbell WA, Andreoli JW, Kaplan BM, Nochimson DJ. Fetal liver ultrasound measurements during normal pregnancy. Obstet Gynecol 1985;66:477-80.
- Symonds ME, Mostyn A, Pearce S, Budge H, Stephenson T. Endocrine and nutritional regulation of fetal adipose tissue development. J Endocrinol 2003;179:293-9. http://dx.doi.org/10.1677/joe.0.1790293.
- HAPO Study Cooperative Research Group . Hyperglycemia and Adverse Pregnancy Outcome (HAPO) study: associations with neonatal anthropometrics. Diabetes 2009;58:453-9. http://dx.doi.org/10.2337/db08-1112.
- Modi N, Murgasova D, Ruager-Martin R, Thomas EL, Hyde MJ, Gale C, et al. The influence of maternal body mass index on infant adiposity and hepatic lipid content. Pediatr Res 2011;70:287-91. http://dx.doi.org/10.1203/PDR.0b013e318225f9b1.
- Patenaude Y, Pugash D, Lim K, Morin L, Lim K, Bly S, et al. The use of magnetic resonance imaging in the obstetric patient. J Obstet Gynaecol Can 2014;36:349-63. http://dx.doi.org/10.1016/S1701-2163(15)30612-5.
- Strizek B, Jani JC, Mucyo E, De Keyzer F, Pauwels I, Ziane S, et al. Safety of MR imaging at 1.5 T in fetuses: a retrospective case–control study of birth weights and the effects of acoustic noise. Radiology 2015;275:530-7. http://dx.doi.org/10.1148/radiol.14141382.
- Kim H, Taksali SE, Dufour S, Befroy D, Goodman TR, Petersen KF, et al. Comparative MR study of hepatic fat quantification using single-voxel proton spectroscopy, two-point dixon and three-point IDEAL. Magn Reson Med 2008;59:521-7. http://dx.doi.org/10.1002/mrm.21561.
- Irwan R, Edens MA, Sijens PE. Assessment of the variations in fat content in normal liver using a fast MR imaging method in comparison with results obtained by spectroscopic imaging. Eur Radiol 2008;18:806-13. http://dx.doi.org/10.1007/s00330-007-0801-0.
- Hussain HK, Chenevert TL, Londy FJ, Gulani V, Swanson SD, McKenna BJ, et al. Hepatic fat fraction: MR imaging for quantitative measurement and display – early experience. Radiology 2005;237:1048-55. http://dx.doi.org/10.1148/radiol.2373041639.
- Barr SM, Chiswick C, Semple S, Gray C, Cooper A, Forbes S, et al. Fat distribution and ectopic lipid distribution in morbidly obese pregnant women in the third trimester: MRI analyses. Arch Dis Child Fetal Neonatal Ed 2012;97:A34-5. http://dx.doi.org/10.1136/fetalneonatal-2012-301809.110.
- Vega GL, Chandalia M, Szczepaniak LS, Grundy SM. Metabolic correlates of nonalcoholic fatty liver in women and men. Hepatology 2007;46:716-22. http://dx.doi.org/10.1002/hep.21727.
- Szczepaniak LS, Nurenberg P, Leonard D, Browning JD, Reingold JS, Grundy S, et al. Magnetic resonance spectroscopy to measure hepatic triglyceride content: prevalence of hepatic steatosis in the general population. Am J Physiol Endocrinol Metab 2005;288:E462-8. http://dx.doi.org/10.1152/ajpendo.00064.2004.
- Sprung VS, Jones H, Pugh CJ, Aziz NF, Daousi C, Kemp GJ, et al. Endothelial dysfunction in hyperandrogenic polycystic ovary syndrome is not explained by either obesity or ectopic fat deposition. Clin Sci 2014;126:67-74. http://dx.doi.org/10.1042/CS20130186.
- Barker DJ. Fetal origins of coronary heart disease. BMJ 1995;311:171-4. http://dx.doi.org/10.1136/bmj.311.6998.171.
- Cottrell EC, Seckl JR. Prenatal stress, glucocorticoids and the programming of adult disease. Front Behav Neurosci 2009;3. http://dx.doi.org/10.3389/neuro.08.019.2009.
- Seckl JR. Prenatal glucocorticoids and long-term programming. Eur J Endocrinol 2004;151:U49-62. http://dx.doi.org/10.1530/eje.0.151U049.
- Duthie L, Reynolds RM. Changes in the maternal hypothalamic–pituitary–adrenal axis in pregnancy and postpartum: influences on maternal and fetal outcomes. Neuroendocrinology 2013;98:106-15. http://dx.doi.org/10.1159/000354702.
- Lindsay JR, Nieman LK. The hypothalamic–pituitary–adrenal axis in pregnancy: challenges in disease detection and treatment. Endocr Rev 2005;26:775-99. http://dx.doi.org/10.1210/er.2004-0025.
- Jung C, Ho JT, Torpy DJ, Rogers A, Doogue M, Lewis JG, et al. A longitudinal study of plasma and urinary cortisol in pregnancy and postpartum. J Clin Endocrinol Metab 2011;96:1533-40. http://dx.doi.org/10.1210/jc.2010-2395.
- Sandman CA, Glynn L, Schetter CD, Wadhwa P, Garite T, Chicz-DeMet A, et al. Elevated maternal cortisol early in pregnancy predicts third trimester levels of placental corticotropin releasing hormone (CRH): priming the placental clock. Peptides 2006;27:1457-63. http://dx.doi.org/10.1016/j.peptides.2005.10.002.
- Edwards CR, Benediktsson R, Lindsay RS, Seckl JR. Dysfunction of placental glucocorticoid barrier: link between fetal environment and adult hypertension?. Lancet 1993;341:355-7. http://dx.doi.org/10.1016/0140-6736(93)90148-A.
- Gitau R, Cameron A, Fisk NM, Glover V. Fetal exposure to maternal cortisol. Lancet 1998;352:707-8. http://dx.doi.org/10.1016/S0140-6736(05)60824-0.
- Gitau R, Fisk NM, Teixeira JM, Cameron A, Glover V. Fetal hypothalamic–pituitary–adrenal stress responses to invasive procedures are independent of maternal responses. J Clin Endocrinol Metab 2001;86:104-9. http://dx.doi.org/10.1210/jc.86.1.104.
- Reynolds RM. Corticosteroid-mediated programming and the pathogenesis of obesity and diabetes. J Steroid Biochem Mol Biol 2010;122:3-9. http://dx.doi.org/10.1016/j.jsbmb.2010.01.009.
- Reynolds RM. Glucocorticoid excess and the developmental origins of disease: two decades of testing the hypothesis – 2012 Curt Richter Award Winner. Psychoneuroendocrinology 2013;38:1-11. http://dx.doi.org/10.1016/j.psyneuen.2012.08.012.
- Mårin P, Darin N, Amemiya T, Andersson B, Jern S, Björntorp P. Cortisol secretion in relation to body fat distribution in obese premenopausal women. Metab Clin Exp 1992;41:882-6. http://dx.doi.org/10.1016/0026-0495(92)90171-6.
- Strain GW, Zumoff B, Kream J, Strain JJ, Levin J, Fukushima D. Sex difference in the influence of obesity on the 24 hr mean plasma concentration of cortisol. Metab Clin Exp 1982;31:209-12. http://dx.doi.org/10.1016/0026-0495(82)90054-3.
- Strain GW, Zumoff B, Strain JJ, Levin J, Fukushima DK. Cortisol production in obesity. Metab Clin Exp 1980;29:980-5. http://dx.doi.org/10.1016/0026-0495(80)90043-8.
- Aubuchon-Endsley NL, Bublitz MH, Stroud LR. Pre-pregnancy obesity and maternal circadian cortisol regulation: moderation by gestational weight gain. Biol Psychol 2014;102:38-43. http://dx.doi.org/10.1016/j.biopsycho.2014.07.006.
- Braig S, Grabher F, Ntomchukwu C, Reister F, Stalder T, Kirschbaum C, et al. Determinants of maternal hair cortisol concentrations at delivery reflecting the last trimester of pregnancy. Psychoneuroendocrinology 2015;52:289-96. http://dx.doi.org/10.1016/j.psyneuen.2014.12.006.
- Stirrat LI, O’Reilly JR, Barr SM, Andrew R, Riley SC, Howie AF, et al. Decreased maternal hypothalamic–pituitary–adrenal axis activity in very severely obese pregnancy: associations with birthweight and gestation at delivery. Psychoneuroendocrinology 2016;63:135-43. http://dx.doi.org/10.1016/j.psyneuen.2015.09.019.
- Livak KJ, Schmittgen TD. Analysis of relative gene expression data using real-time quantitative PCR and the 2(-Delta Delta C(T)) method. Methods 2001;25:402-8. http://dx.doi.org/10.1006/meth.2001.1262.
- Burton GJ, Sebire NJ, Myatt L, Tannetta D, Wang YL, Sadovsky Y, et al. Optimising sample collection for placental research. Placenta 2014;35:9-22. http://dx.doi.org/10.1016/j.placenta.2013.11.005.
- Crane SS, Wojtowycz MA, Dye TD, Aubry RH, Artal R. Association between pre-pregnancy obesity and the risk of cesarean delivery. Obstet Gynecol 1997;89:213-16. http://dx.doi.org/10.1016/S0029-7844(96)00449-8.
- Loverro G, Greco P, Vimercati A, Nicolardi V, Varcaccio-Garofalo G, Selvaggi L. Maternal complications associated with cesarean section. J Perinat Med 2001;29:322-6. http://dx.doi.org/10.1515/JPM.2001.046.
- Young TK, Woodmansee B. Factors that are associated with cesarean delivery in a large private practice: the importance of prepregnancy body mass index and weight gain. Am J Obstet Gynecol 2002;187:312-18. http://dx.doi.org/10.1067/mob.2002.126200.
- Weiss JL, Malone FD, Emig D, Ball RH, Nyberg DA, Comstock CH, et al. Obesity, obstetric complications and cesarean delivery rate – a population-based screening study. Am J Obstet Gynecol 2004;190:1091-7. http://dx.doi.org/10.1016/j.ajog.2003.09.058.
- Zhang J, Bricker L, Wray S, Quenby S. Poor uterine contractility in obese women. BJOG 2007;114:343-8. http://dx.doi.org/10.1111/j.1471-0528.2006.01233.x.
- Al-Qahtani S, Heath A, Quenby S, Dawood F, Floyd R, Burdyga T, et al. Diabetes is associated with impairment of uterine contractility and high Caesarean section rate. Diabetologia 2012;55:489-98. http://dx.doi.org/10.1007/s00125-011-2371-6.
- Tack CJ, Lutterman JA, Vervoort G, Thien T, Smits P. Activation of the sodium-potassium pump contributes to insulin-induced vasodilation in humans. Hypertension 1996;28:426-32. http://dx.doi.org/10.1161/01.HYP.28.3.426.
- Chew CS, Rinard GA. Glycogen levels in the rat myometrium at the end of pregnancy and immediately postpartum. Biol Reprod 1979;20:1111-14. http://dx.doi.org/10.1095/biolreprod20.5.1111.
- Steingrímsdóttir T, Ronquist G, Ulmsten U, Waldenström A. Low myometrial glycogen content compared with rectus muscle in term pregnant women before labor. Gynecol Obstet Invest 1999;47:166-71. http://dx.doi.org/10.1159/000010086.
- Arrowsmith S, Quenby S, Weeks A, Burdyga T, Wray S. Poor spontaneous and oxytocin-stimulated contractility in human myometrium from postdates pregnancies. PLOS ONE 2012;7. http://dx.doi.org/10.1371/journal.pone.0036787.
- Lust WD, Passonneau JV, Crites SK. The measurement of glycogen in tissues by amylo-alpha-1,4-alpha-1,6-glucosidase after the destruction of preexisting glucose. Anal Biochem 1975;68:328-31. http://dx.doi.org/10.1016/0003-2697(75)90712-5.
- Hehir MP, Morrison JJ. Metformin and human uterine contractility. Endocrine 2012;42:761-3. http://dx.doi.org/10.1007/s12020-012-9687-y.
- Pyla R, Osman I, Pichavaram P, Hansen P, Segar L. Metformin exaggerates phenylephrine-induced AMPK phosphorylation independent of CaMKKbeta and attenuates contractile response in endothelium-denuded rat aorta. Biochem Pharmacol 2014;92:266-79. http://dx.doi.org/10.1016/j.bcp.2014.08.024.
- Sung JY, Choi HC. Metformin-induced AMP-activated protein kinase activation regulates phenylephrine-mediated contraction of rat aorta. Biochem Biophys Res Commun 2012;421:599-604. http://dx.doi.org/10.1016/j.bbrc.2012.04.052.
- Wu Y, Liu L, Zhang Y, Wang G, Han D, Ke R, et al. Activation of AMPK inhibits pulmonary arterial smooth muscle cells proliferation. Exp Lung Res 2014;40:251-8. http://dx.doi.org/10.3109/01902148.2014.913092.
- Labazi H, Wynne BM, Tostes R, Webb RC. Metformin treatment improves erectile function in an angiotensin II model of erectile dysfunction. J Sex Med 2013;10:2154-64. http://dx.doi.org/10.1111/jsm.12245.
- Smith RD, Babiychuk EB, Noble K, Draeger A, Wray S. Increased cholesterol decreases uterine activity: functional effects of cholesterol alteration in pregnant rat myometrium. Am J Physiol Cell Physiol 2005;288:C982-8. http://dx.doi.org/10.1152/ajpcell.00120.2004.
- Altman DG, Schulz KF, Moher D, Egger M, Davidoff F, Elbourne D, et al. The revised CONSORT statement for reporting randomized trials: explanation and elaboration. Ann Intern Med 2001;134:663-94. http://dx.doi.org/10.7326/0003-4819-134-8-200104170-00012.
- Featherstone K, Donovan JL. ‘Why don’t they just tell me straight, why allocate it?’ The struggle to make sense of participating in a randomised controlled trial. Soc Sci Med 2002;55:709-19. http://dx.doi.org/10.1016/S0277-9536(01)00197-6.
- Moher D, Schulz KF, Altman DG. The CONSORT statement: revised recommendations for improving the quality of reports of parallel-group randomised trials. Lancet 2001;357:1191-4. http://dx.doi.org/10.1016/S0140-6736(00)04337-3.
- Canvin K, Jacoby A. Duty, desire or indifference? A qualitative study of patient decisions about recruitment to an epilepsy treatment trial. Trials 2006;7. http://dx.doi.org/10.1186/1745-6215-7-32.
- Madsen SM, Holm S, Riis P. Attitudes towards clinical research among cancer trial participants and non-participants: an interview study using a grounded theory approach. J Med Ethics 2007;33:234-40. http://dx.doi.org/10.1136/jme.2005.015255.
- Snowdon C, Garcia J, Elbourne D. Making sense of randomization; responses of parents of critically ill babies to random allocation of treatment in a clinical trial. Soc Sci Med 1997;45:1337-55. http://dx.doi.org/10.1016/S0277-9536(97)00063-4.
- Eborall HC, Stewart MC, Cunningham-Burley S, Price JF, Fowkes FG. Accrual and drop out in a primary prevention randomised controlled trial: qualitative study. Trials 2011;12. http://dx.doi.org/10.1186/1745-6215-12-7.
- Catov JM, Bodnar LM, Ness RB, Barron SJ, Roberts JM. Inflammation and dyslipidemia related to risk of spontaneous preterm birth. Am J Epidemiol 2007;166:1312-19. http://dx.doi.org/10.1093/aje/kwm273.
- Xu L, Lee M, Jeyabalan A, Roberts JM. The relationship of hypovitaminosis D and IL-6 in preeclampsia. Am J Obstet Gynecol 2014;210:149.e1-7. http://dx.doi.org/10.1016/j.ajog.2013.09.037.
Appendix 1 Study protocol
The study protocol can be found at www.nets.nihr.ac.uk/projects/eme/0824609 (accessed 11 July 2016).
Appendix 2 Maternal anthropometry measurements
Appendix 3 Neonatal anthropometry measurements
Appendix 4 Collection, storage and transfer of blood samples
Appendix 5 Serious adverse event form
Appendix 6 Statistical analysis plan
Appendix 7 Placental biopsy sample collection
Appendix 8 Myometrial biopsy collection
Appendix 9 Public and patient involvement
Recruitment of study participants was challenging. The majority of women approached declined to participate. Anecdotally, this was predominantly because of a concern about taking medication during pregnancy. However, it was also evident that there was a lack of awareness of the problems associated with obesity during pregnancy, particularly potential longer-term harms for the offspring. We held education sessions for study midwives to enable them to pass on this message to their patients and the wider midwifery community. We distributed posters advertising the study to all clinical areas where potentially eligible women might see them. Following feedback from the public and potential patients that they found the word ‘obese’ offensive, we revised all of our materials and used the more acceptable terminology ‘high BMI’. We employed the use of social media and a website to disseminate information to the public and participants about the progress of the trial, for example updates when recruitment targets had been reached. We included a qualitative substudy to explore the reasons behind patients’ reluctance to participate in the trial and why participants withdrew from the trial once they had been recruited.
Patients and the public were not formally involved in the trial at the design and concept stage. However, the clinical investigators (including the lead investigator) were strongly influenced by the pregnant women (patients) they look after and these influences were key to the trial.
Patient/public involvement was formally instituted during the conduct of the trial. A patient/public representative was appointed to the Trial Steering Committee. She made helpful comments on the patient information leaflet and attended several meetings of the Trial Steering Committee. She resigned in the last year of the study and, given that the trial was ending, she was not formally replaced.
List of abbreviations
- ADP
- air displacement plethysmography
- ANOVA
- analysis of variance
- AUC
- area under the curve
- BMI
- body mass index
- cDNA
- complementary deoxyribonucleic acid
- CI
- confidence interval
- CRP
- C-reactive protein
- CV
- coefficient of variation
- DNA
- deoxyribonucleic acid
- EGP
- endogenous glucose production
- ELISA
- enzyme-linked immunosorbent assay
- EMPOWaR
- Efficacy of Metformin in Pregnant Obese Women, a Randomised controlled trial
- FFM
- fat-free mass
- FLASH
- fast low-angle shot
- FMD
- flow-mediated dilatation
- GDM
- gestational diabetes mellitus
- GR
- glucocorticoid receptor
- GTN
- nitroglycerin
- HDL
- high-density lipoprotein
- HOMA-IR
- homeostatic model assessment – insulin resistance
- HPA
- hypothalamic–pituitary–adrenal
- HSD
- hydroxysteroid dehydrogenase
- IADPSG
- International Association of the Diabetes and Pregnancy Study Groups
- IL
- interleukin
- ITT
- intention to treat
- LDL
- low-density lipoprotein
- LGA
- large for gestational age
- LiP
- Lifestyle in Pregnancy
- M/I
- glucose disposal per unit plasma insulin
- MOP
- Metformin in Obese Pregnancy
- MRC
- Medical Research Council
- MRI
- magnetic resonance imaging
- mRNA
- messenger ribonucleic acid
- MRS
- magnetic resonance spectroscopy
- NEFA
- non-esterified fatty acid
- OR
- odds ratio
- PAI
- plasminogen activator inhibitor
- PCOS
- polycystic ovary syndrome
- PSS
- physiological saline
- PSS 0-glucose
- physiological saline lacking glucose
- Ra
- rate of appearance
- RCT
- randomised controlled trial
- Rd
- rate of disappearance
- RNA
- ribonucleic acid
- RT
- reverse transcriptase
- SAE
- serious adverse event
- SD
- standard deviation
- SOP
- standard operating procedure
- UPBEAT
- UK Pregnancies Better Eating and Activity Trial
- v/v
- volume per volume
- WGD
- whole-body glucose disposal
- WHO
- World Health Organization