Notes
Article history
The research reported in this issue of the journal was funded by the EME programme as project number 12/127/127. The contractual start date was in January 2015. The final report began editorial review in June 2020 and was accepted for publication in December 2020. The authors have been wholly responsible for all data collection, analysis and interpretation, and for writing up their work. The EME editors and production house have tried to ensure the accuracy of the authors’ report and would like to thank the reviewers for their constructive comments on the final report document. However, they do not accept liability for damages or losses arising from material published in this report.
Permissions
Copyright statement
© Queen’s Printer and Controller of HMSO 2021. This work was produced by Haldar et al. under the terms of a commissioning contract issued by the Secretary of State for Health and Social Care. This issue may be freely reproduced for the purposes of private research and study and extracts (or indeed, the full report) may be included in professional journals provided that suitable acknowledgement is made and the reproduction is not associated with any form of advertising. Applications for commercial reproduction should be addressed to: NIHR Journals Library, National Institute for Health Research, Evaluation, Trials and Studies Coordinating Centre, Alpha House, University of Southampton Science Park, Southampton SO16 7NS, UK.
2021 Queen’s Printer and Controller of HMSO
Chapter 1 Introduction
Background
Atrial fibrillation (AF) is an important public health concern because it is the most common arrhythmia, affecting 1–2% of the population. Its prevalence is on the increase with the rising age of populations. 1–5 AF leads to lower quality of life, as patients with this condition have increased risk of morbidity (thromboembolic stroke, heart failure) and mortality, evidenced by the large number of hospital admissions. 4–8 Treating AF requires considerable resources and long-term medications; therefore, around 2% of the NHS budget in the UK is spent on treating and managing AF. 2,8–10
Stroke prevention with anticoagulants is the main concern when managing patients with AF. In addition, pharmacological agents for heart rhythm or rate control may be used, depending on clinical indications and patient characteristics. Rhythm control is preferred in patients with persistent AF who continue to experience symptoms despite adequate rate control. 2,5,11,12
Traditionally, agents to control rhythm are antiarrhythmic drugs (AADs) and/or direct-current cardioversion (DCCV), but the long-term efficacy of these treatments is poor and they are associated with drug side effects and the risk of pro-arrhythmia.
Percutaneous catheter ablation (CA) has been shown to be more effective than AAD therapy in achieving and maintaining normal sinus rhythm (SR) and is now routinely offered as standard management for patients with symptomatic AF. 2,4,5,11–18
In a large proportion of patients (up to 78%) with paroxysmal AF, conventional CA can reliably establish and maintain SR. 8,12,19–22 Similar results can be achieved in patients with short-lasting persistent AF, but not in those with long-standing persistent atrial fibrillation (LSPAF). 23–27 The success in this group is poor, being only 32–40%, and more than one procedure is often required to establish normal heart rhythm. 8,28–32
Minimally invasive thoracoscopic surgical ablation (SA) is another option for treatment of AF, and there are indications that it may be significantly more effective than CA in restoring SR. This technique offers direct access to cardiac tissues and can deliver transmural and contiguous lesions. Ablation of ganglionic plexuses and occlusion of the left atrial appendage (LAA) may contribute to its greater efficacy. 33–36 However, risk of morbidity and complications need to be included in the assessment of this procedure before it can be considered a treatment option for patients with LSPAF.
Methods/design
The Catheter Ablation versus thoracoscopic Surgical Ablation in long-standing persistent Atrial Fibrillation (CASA-AF) trial is a prospective, multicentre, randomised clinical trial to assess the effectiveness and safety of thoracoscopic SA compared with CA (usual care) in patients with LSPAF. The study protocol was developed in accordance with current guidelines for clinical trials37,38 and was published in 2018. 39
The study took place in four tertiary cardiovascular specialist NHS hospitals in England with proven expertise in both of the interventions and a large pool of potentially eligible participants (Royal Brompton Hospital, Harefield Hospital, Liverpool Heart and Chest Hospital, and Brighton and Sussex University Hospital).
The conduct of the study was overseen by an independent Data Monitoring Committee (DMC) and an independent Trial Steering Committee (TSC), which both met twice per year throughout the study.
Outcomes of the study
Primary
The primary hypothesis of this study was that thoracoscopic SA is clinically more effective than percutaneous CA in achieving freedom from atrial arrhythmia. The primary outcome was therefore absence of atrial arrhythmia (fibrillation or tachycardia lasting ≥ 30 seconds) after a single treatment and without AADs within the follow-up period (from the end of the 3 months’ blanking period to 12 months after the ablation). Data collected by the implanted loop recorder (ILR) over the follow-up period was used to detect the recurrence of AF/atrial tachycardia (AT) lasting ≥ 30 seconds.
Secondary
The most important among the secondary outcomes was the safety of the two interventions. The safety end point in this trial was intervention-related major complication [serious adverse event (SAE)], defined as permanent injury or death, an event that requires unplanned intervention for treatment, or an event that prolongs or requires unplanned hospitalisation for > 48 hours.
In addition, we wanted to evaluate:
-
The secondary efficacy outcome, defined as the clinical success of the two interventions, comparing the reduction of AF burden (≥ 75%) over the follow-up period in each treatment arm. The 75% burden reduction was evaluated by calculating the percentage of time that patients spent in AF/AT each month. If this percentage was ≥ 25% in any of the months of follow-up, the burden reduction of 75% was not achieved.
-
The requirement for multiple procedures during 12 months’ follow-up.
-
The effect of arrhythmia interventions on patients’ symptoms and quality of life through changes in European Heart Rhythm Association (EHRA), EuroQol-5 Dimensions, five-level version (EQ-5D-5L) and Atrial Fibrillation Effect on QualiTy-of-life (AFEQT) questionnaire scores. 40,41
-
Quality-adjusted life-years (QALYs) accrued during the 12-month period and cost-effectiveness [incremental cost (IC) per QALY gained] for SA compared with CA, estimated over the 12-month period (‘within-trial’ analysis) and over a lifetime horizon (estimated by modelling).
-
Changes in atrial size and function following ablation using echocardiography and cardiac magnetic resonance imaging (CMRI) parameters.
Characteristics of participants
Adults with symptomatic LSPAF considered for treatment (DCCV or CA) at the participating trial centres were considered for inclusion in the study. The complete list of inclusion and exclusion criteria is presented in Box 1.
Age ≥ 18 years.
Long-standing persistent AF (> 12 months’ duration).
EHRA symptom score > 2. a
Left ventricular ejection fraction ≥ 40%.
Suitable for either ablation procedure.
Exclusion criteriaValvular heart disease with a severity greater than mild.
Contraindication to anticoagulation.
Thrombus in the left atrium despite anticoagulation in the therapeutic range.
Cerebrovascular accident within the previous 6 months.
Previous thoracic or cardiac surgery (including surgical interventions for AF).
Prior left atrial CA for AF.
Unable to provide informed written consent.
Active malignancy, another severe concomitant condition or presence of implanted cardiac devices that would preclude patient undergoing study-specific procedures.
Pregnant or breastfeeding, or women of childbearing age not using a reliable contraceptive method.
Patients included in the study had symptoms classed as severe (i.e. had a EHRA symptom score of 3), which affected their normal daily activity.
The consultant cardiologists at participating sites referred patients from outpatient clinics or through DCCV and ablation waiting lists for inclusion in the trial.
CASA-AF trial schedule
Patients considered eligible to take part in the study were given a participant information sheet (PIS) and provided with opportunities to discuss details of participation with the study team. When they confirmed their interest in taking part, a hospital appointment was arranged for them to sign an informed consent form and complete baseline assessments. Participants’ progress through the study is shown schematically in Figure 1.
FIGURE 1.
Schematic representation of the CASA-AF study design. ECG, electrocardiography; HEQ, health economic questionnaire; TTE, transthoracic echocardiogram.

Baseline study visit
As part of the baseline visit, we collected blood samples to assess full blood count; electrolytes; renal, liver and thyroid function; coagulation profile; levels of C-reactive protein; tests for diabetes (glycated haemoglobin levels); and lipid profile (high-density lipoproteins, low-density lipoproteins).
Transthoracic echocardiogram (TTE) and cardiac magnetic resonance assessments were performed to ensure that patients did not have poor left ventricular function, valvular disease or other pathologies meeting the exclusion criteria.
A list of additional clinical and study data collected at baseline, as well as at other study time points, [Standard Protocol Items: Recommendations for Interventional Trials (SPIRIT) figure] is shown in Appendix 1, Table 30.
Treatment allocation
Patients with confirmed eligibility were randomly assigned to a treatment arm by a computer-generated sequence in a 1 : 1 ratio using minimisation. A secure 24-hour web-based system hosted by the King’s Clinical Trials Unit provided named research team members with access to the allocation system. The system used the study site code, participant’s sex and the diameter of the left atrium (LA) (< 50 or ≥ 50 mm) as minimisation variables.
Treatment allocation was concealed from the researchers undertaking randomisation, but blinding was not possible in this trial. The primary outcome(s) assessors were blinded to treatment arms, as were the trial statistician and the health economist.
Pre ablation
Anticoagulation
Current guidelines were used to manage anticoagulation. 2,9,42–45 Patients randomised to CA were usually on uninterrupted warfarin therapy. Those treated with novel oral anticoagulants (NOACs) could be converted to warfarin for 4 weeks before the CA or stop NOAC therapy 24–36 hours before the procedure. The choice depended on the type of NOAC and the clinician’s preference.
For patients in the SA group, warfarin therapy was stopped 5 days before the procedure and bridging with enoxaparin was introduced for 3 days at a dose of 1.5 mg/kg once daily. Patients had no anticoagulation treatment for 24 hours before the procedure. NOAC treatments were also discontinued in the SA group 2–5 days before surgery, depending on the type of NOAC and the patient’s renal function parameters.
Treatment groups
Thoracoscopic surgical atrial fibrillation ablation
This procedure was performed as previously described by Yilmaz et al. 46–48 In this trial, the presence of a cardiac electrophysiologist in theatre during SA was mandated to ensure that conduction blocks were tested and achieved for all lesions to ensure better outcomes. 49 Cardiac surgeons participating in this study were required to have experience in video-assisted thoracoscopic surgery. In addition, they also had to have conducted at least 20 thoracoscopic ablations to treat AF as the primary operator.
Prior to the start of the procedure, transoesophageal echocardiography (TOE) was conducted under general anaesthetic to exclude thrombus in the LA. Three thoracoports were made on each side of the chest. Pericardiotomy exposed pulmonary veins (PVs) and the left atrial antrum. Blunt dissection using the LumitipTM dissector (AtriCure, Inc., Mason, OH, USA) lead into the transverse and oblique sinuses. A bipolar radiofrequency ablation clamp (AtriCure, Inc.) was used to isolate PVs from the epicardial surface, with additional overlapping applications around the LA antrum. Entrance and exit blocks of lesions were confirmed at the end of the procedure. If pulmonary vein isolation (PVI) was incomplete, further ablation was performed. Ganglionic plexuses were located by high-frequency stimulation and an isolator multifunctional pen (AtriCure, Inc.) was used to ablate them. The multifunctional pen was also used to create additional ablation lines to connect the contralateral superior PVs (roof line) and the inferior PVs (inferior line), thus creating a posterior wall box lesion. SR was restored by external DCCV if AF was not terminated by ablation. Verification of electrical isolation of the posterior box was conducted using sensing and pacing manoeuvres in SR. Finally, the LAA was excluded using the AtriClip® LAA exclusion system (AtriCure, Inc., Mason, OH, USA). 50–53 At the end of the procedure, the patients had an ILR implanted and were extubated in the operating theatre.
Catheter atrial fibrillation ablation (usual care treatment)
With the patient under general anaesthesia, TOE was first used to exclude a thrombus and then to guide transeptal puncture. The CARTO® 3 three-dimensional (3D) electroanatomical mapping system (Biosense Webster, Inc., Diamond Bar, CA, USA) was used to create the left atrial geometry with a 20-pole circular mapping catheter (CARTO LASSO® 2515 NAV; Biosense Webster, Inc.). Simultaneous voltage maps were created using CARTO® CONFIDENSETM (Biosense Webster, Inc.). Ablation was conducted with a CARTO® THERMOCOOL SMARTTOUCHTM 3.5-mm irrigated-tip catheter (Biosense Webster, Inc.). We did not use oesophageal temperature monitoring during the procedure. A stepwise ablation strategy was used to electrically isolate the PVs at the antral level, and then linear ablation was performed at the left atrial roof and a posterior line was created (to create a ‘box lesion’). A lateral mitral isthmus line and the cavotricuspid isthmus were also ablated. Electrical isolation of the PVs was confirmed through testing for both entrance and exit blocks with the circular sensing catheter. The integrity of the linear lesions was assessed using differential pacing manoeuvres with further ablation, if necessary, to achieve bidirectional block. If AT occurred at any point, it was mapped and ablated when possible. 54 An ILR was implanted at the end of the procedure once the activated clotting time was normalised. Patients were extubated in the cardiac catheterisation laboratories.
Post ablation
Postoperative analgesia
Participants in the SA arm received intercostal nerve block at each port site (bupivacaine or similar agents; dosage depending on patient characteristics and tolerance), paracetamol (1 g four times each day, intravenous/per rectum and then per os) and codeine (30–60 mg four times each day).
One day after surgery, if the patient’s renal function was normal and they were able to tolerate oral medication, analgesia could be provided by non-steroidal anti-inflammatory drugs for 1 week with or without opiates.
Following discharge, patients were prescribed analgesics (e.g. paracetamol, codeine) to be taken as needed.
Postoperative anticoagulation
Anticoagulation therapy was restarted soon after ablation if there were no contraindications. In the SA group, anticoagulation was restarted with enoxaparin (1.5 mg per kg once daily) or other heparin derivatives until the chest drains were removed. Subsequent anticoagulation was the same as before the ablation and continued for the duration of the trial in both treatment arms.
Postoperative antiarrhythmic drugs
Antiarrhythmic drug therapy (flecainide, procainamide, amiodarone or sotalol) was allowed for a maximum of 3 months after the ablation procedure (blanking period). Medications that have an effect on heart rhythm but are used to treat conditions like hypertension (i.e. beta-blockers and non-dihydropyridine calcium channel blockers) were not stopped.
Postoperative antibiotic prophylaxis
In both treatment arms, patients usually received one dose of antibiotic on induction of anaesthesia, one dose at the end of the procedure, and one dose up to 5 days postoperatively, depending on local practices.
Early postoperative discharge period
During the first month after discharge, study participants were contacted by the research team once per week to assess their health status. They were asked about pain management, cough, raised temperature, difficulties swallowing and any other symptoms that may have been early indications of possible complications. In addition, patients were advised to contact the study team if they had any concerns regarding their health at any point in the study. If appropriate, the patients had a full assessment, evaluation and treatment of reported health issues even if they were not related to study participation.
Implanted loop recorder
Continuous cardiac monitoring using implanted loop recorders is shown to have overall high accuracy and excellent diagnostic yield in identifying AF/AT compared with non-invasive, intermittent cardiac monitoring. 55,56 The Medtronic Reveal LINQTM ILR (Medtronic plc, Dublin, Ireland) was chosen because it was, at the time, the smallest, latest generation of ILRs available, with the most reliable remote monitoring system that helps minimise data loss. It was inserted at the end of the index ablation procedure subcutaneously in parasternal location.
Patients were registered on the Medtronic CareLink® Network (Medtronic plc) following ILR insertion and were instructed on how to perform manual data downloads using their remote monitoring equipment. During manual download, the encrypted data from the ILR were sent via mobile networks to a central server and routed to the CareLink® website, which has restricted user access. In addition, automatic event reports were generated by the CareLink® system when the device detected any arrhythmia. A symptom activator was also provided to the patients to allow recording and transmission of heart rhythm prior to and after the event associated with the patient-experienced symptom.
A dedicated cardiac physiologist, blinded to treatment allocation, analysed downloaded data on a regular basis to produce monthly heart rhythm assessment reports for each patient. The data collected by the ILR allowed detection of the AF/AT recurrence as well as providing the duration of each episode. The duration of all episodes of AF/AT during each month of follow-up (30 days) was used to calculate the proportion of time a patient spent in arrhythmia. Patients had to be free from AT/AF burden ≥ 75% of the time each month to be classed as a clinical success.
Programming
The ILR has an automatic AF detection algorithm that continuously analyses beat-to-beat variability of cardiac cycles and an additional ‘P-SENSE’ algorithm, leading to an accurate detection of arrhythmia recurrence, with timing of episodes, as well as an accurate quantification of AF burden (duration of AF and percentage of overall time in AF).
This automatic AF detection algorithm is based on the pattern of R-wave interval variability within 2-minute periods. The differences between consecutive R-wave intervals are plotted in a Lorenz plot. Pattern recognition is used to identify the AF episodes because R-wave intervals during AF episodes are highly irregular and uncorrelated. In case of symptomatic AF/AT, when the recording is triggered by the patient, the ILR transmits 6.5 minutes of pre-trigger electrocardiography (ECG) and 1 minute of post-trigger ECG, where uninterrupted episodes of AF/AT lasting ≥ 30 seconds are defined as recurrent. 57–59
The device was programmed to detect AT and AF with high sensitivity. AT was triggered when the heart rate (HR) was ≥ 100 b.p.m. (beats per minute) and the ectopic rejection was set to nominal so that any arrhythmia could be captured, minimising the chance of missing short episodes. We chose greater sensitivity at the cost of a reduction in specificity, so sinus tachycardia was often recorded as well as atrial arrhythmia. All other ILR parameters were programmed as nominal.
Guided by CareLink® alerts for symptomatic and automated arrhythmia episodes detected, post-ablation therapy optimisation was managed by cardiology clinic follow-ups, outside the study-specific visits. The research team monitored CareLink® alerts to ensure that patients with a large number of arrhythmia episodes downloaded their data more frequently to reduce potential data loss.
Follow-up schedule
Details of the assessments during study participation are given in Figure 1 and in Appendix 1, Table 27, in accordance with the SPIRIT 2013 recommendations. 37,38
Symptomatic AF recurring during the blanking period (up to 3 months after ablation) was treated with DCCV with or without AADs tailored to patient characteristics. Patients were offered percutaneous CA if the AF recurred at a later point in the follow-up period, as shown in Figure 1.
Chapter 2 Analysis
Statistical analysis
All statistical analyses are based on a modified intention-to-treat principle; data from randomised participants who received treatment were included in the analyses. Sensitivity analyses were used to explore the impact of missing data, non-compliance and withdrawals. R statistical software, version 4.0.0 (The R Foundation for Statistical Computing, Vienna, Austria), was used to analyse data.
Sample size (n = 120) was calculated based on the data from our pilot study with 6 months’ follow-up: 76% of participants in the SA group were free from AT/AF, compared with 44% of participants in the CA group. Using these results, a sample size of 48 participants per group was required to detect this difference in the primary outcome with 90% power and a 5% significance level. We added a margin of error of 25% to include a 10% dropout rate and account for the possible greater sensitivity of ILR to detect short episodes of atrial arrhythmias.
The primary outcome of the trial is the proportion of LSPAF patients undergoing ablation who are free from atrial arrhythmias (defined as a single episode of ≥ 30 seconds) within 1 year of a single ablation procedure. Arrhythmia-free patients were identified through monthly ILR data assessments from the end of the blanking period to the end of the 12 months’ follow-up. Burden of AF/AT reduction was assessed from the ILR monthly reports, in which the blinded cardiac physiologist reported the percentage of time that patients spent in atrial arrhythmia. A chi-squared test was used for comparison of the treatment groups. To estimate the probability of being free from AF/AT 1 year after ablation, we developed a logistic regression model, which was used to check for robustness and to control for the variables used in the randomisation algorithm [sex, study site and left atrial diameter (LAD)]. The primary measure to be reported was the odds ratio (OR) of being AF free for the surgical group after controlling for the other factors in the model. The recurrence of AF and duration of AF freedom were analysed using Kaplan–Meier survival curves. The heart rhythm outcomes are also expressed as relative risks (RRs) in the supplementary analyses (see Appendix 2). RRs and their confidence intervals (CIs) were estimated using R’s epitools library. 60
Binary secondary outcomes (reduction in arrhythmia burden, freedom from arrhythmia following multiple procedures) were analysed in the same manner as the primary outcome using a combination of chi-squared tests and logistic regression.
Continuous data were analysed by either Student’s t-test or Mann–Whitney U-test and presented as mean ± standard deviation (SD), mean (95% CI) or median [interquartile range (IQR)], depending on the distribution of obtained data. All tests were two-sided and a p-value of < 0.05 is considered significant.
Missing data
Using an approach similar to the economic analysis, we used the multivariate imputation via chained equations (MICE) predictive mean matching (PMM) procedure to simultaneously impute missing outcome variables. Instead of using predicted outcome values from a parametric regression as the imputed values, PMM identifies a number of ‘near neighbours’ (n = 5 in our analysis) – cases with outcomes similar to the prediction for the missing case – and randomly selects one of these for the imputed value. However, unlike the economic analysis, our multiple imputation (MI) was imputed in R’s mice package. 61 The approach of Aragon60 was not suitable for estimating RR on multiply imputed data.
For the MI data, RRs were estimated using a log-binomial RR regression model implemented in the logbin library. 62 Results were pooled with the mice library. 61
The statistical analysis plan was written before the first DMC and TSC meetings and the final version was signed off by the DMC statistician and the chairperson of the TSC prior to the database lock (see the Statistical Analysis Plan on the NIHR Journals Library study web page; URL: https://fundingawards.nihr.ac.uk/award/12/127/127).
Health economic analysis
The aim of the economic analysis is to compare the relative costs and health effects of SA and CA for people with LSPAF, with health effects measured in terms of QALYs. Our original intention was to use two methods of analysis:
-
a within-trial analysis to compare costs and QALYs estimated over the 1-year follow-up period only
-
a model-based analysis to extrapolate costs and QALYs and estimate the long-term relative cost-effectiveness of the interventions.
We conducted an economic analysis using data collected in the trial. We also report adherence to the Consolidated Health Economic Evaluation Reporting Standards (CHEERS) reporting criteria. 63
Data sources
Health outcomes
Quality-adjusted life-years were estimated at the individual patient level from EQ-5D-5L data collected at baseline and quarterly assessments up to 12 months. Following the position statement from the National Institute for Health and Care Excellence (NICE),64 we used the van Hout ‘crosswalk’ algorithm to map from the five-level EuroQol-5 Dimensions (EQ-5D) questionnaire to the three-level version, and hence to utility values based on the UK social tariff. 64–67
Quality-adjusted life-years were estimated for each participant over the 12-month period by estimating the area under the EQ-5D index score curves. There are four 3-month time periods defined by the five EQ-5D-5L observations. For each period, QALYs accrued were estimated by taking the mean of the scores at adjacent time points and multiplying by the duration of the period. Values for the four 3-month periods were then added to calculate the total QALYs accrued. To avoid bias due to any differences between the treatment arms in the actual timing of the follow-up visits, we assumed a fixed duration for each time period (0.25 of 1 year). One patient died during the trial period; QALYs for this individual were estimated by setting the utility to zero at the date of death.
We compared the EQ-5D index scores with the EHRA symptom score, AFEQT disease-related score and EQ-5D visual analogue scale (VAS) score to assess the consistency of changes over time and differences between the treatment arms.
Resource use
Information about the index admission, in which patients received the initial SA or CA intervention, was collected in the discharge form. This included the dates of admission, procedure and discharge and whether or not patients received the intervention to which they were randomised. The economic analysis was conducted following an intention-to-treat principle.
Information about medications, repeat ablation procedures and DCCVs performed during follow-up were also collected by research staff on case report forms. Information about the use of other health and social care services was collected from patients at their 3-, 6-, 9- and 12-month assessments using a specially designed questionnaire. This questionnaire asked participants whether or not they had used any of a range of resources in the last 3 months ‘for reasons that might be related to your heart condition or treatment’ and, if so, how many times and why. Resources listed in the questionnaire included:
-
visits to the accident and emergency department and use of ambulance services
-
hospital inpatient admissions and day-case attendances
-
hospital outpatient visits and tests
-
primary care and community services (including consultations with a general practitioner, primary care or community nurse, cardiology community nurse, physiotherapist and occupational therapist)
-
personal social care funded by local authorities (including home help, help with personal care, Meals on Wheels, admission to residential or nursing care, provision of specialist equipment and consultations with a social worker).
Each section of the form also included a question about the use of other related services, including social care.
The protocol stated that we would collect costs for all health and social care, but we changed this to collecting related costs only to reduce the burden of assessment for patients and the research team. This is unlikely to have had any impact on the relative costs of SA compared with CA.
Costing
Costs were estimated from an NHS payer perspective, based on estimated payments to providers.
For the index admission, we used individually coded costs for patients treated at the Royal Brompton Hospital and Harefield Hospital, which were available for 70 out of the 94 patients who were admitted for CA or SA at these sites. Cost data were not available for the 21 patients treated at the other study sites. We consider hospital-coded data to be more accurate than an inferred cost for the admissions based on Healthcare Resource Group (HRG) codes. This is because the hospital-coded costs include adjustment for an individual’s length of stay (LOS) and for more than one episode of care during the admission, which allows for additional critical care, recovery time, procedures and diagnostic tests, when required. Costs for patients for whom individually coded costs were not available were imputed in the MI procedure as described in Missing data and imputation.
Costs for other hospital, primary and community health services were estimated from patient-reported use with unit costs from the National Schedule of NHS Costs for 2018/19. 68 The only exceptions were consultations with a doctor or nurse in primary care, for which unit costs were based on estimates in the Personal Social Services Research Unit’s Unit Costs of Health and Social Care 2019. 69
Medicine use during follow-up was costed using the NHS list price as reported in the Monthly Index of Medical Specialities (MIMS) Online 2020, accessed in March 2020. 70
Analysis set
We used an intention-to-treat approach for the economic analysis. This included all 120 randomised patients, including five patients randomised to SA who did not undergo a procedure and another six patients randomised to SA who had CA.
Missing data and imputation
Missing data are a particular problem for trial-based economic evaluations, even in studies with good follow-up and data collection, as in this trial. The area under the curve (AUC) approach to QALY estimation requires utility data from five time points. Similarly, cost estimates over the trial period are based on estimates of various resource quantities used over the four 3-month time periods. The proportion of participants that can be included in a complete-case analysis of costs and QALYs is limited, as each person with any missing data point must be excluded.
Multiple imputation was therefore used to reduce the potential for bias due to missing data. 71,72 We used the Stata® version 16 (StataCorp LP, College Station, TX, USA) chained MICE PMM procedure to simultaneously impute missing EQ-5D index scores and cost estimates. The chained approach imputes missing values for multiple variables iteratively, using a sequence of predictive equations that is fully conditional: it accounts for possible dependence between the imputed variables, as well as with any specified non-imputed covariates. Instead of using predicted outcome values from a parametric regression as the imputed values, PMM identifies a number of ‘near neighbours’ (five in our analysis) – cases with outcomes similar to the prediction for the missing case – and randomly selects one of these for the imputed value. This can give imputed values with a distribution more like that of the real values. PMM has been recommended for cost data, which must be greater than or equal to zero, and usually has a large positive skew. 73 It may also be more appropriate than linear regression-based imputation for utilities, which are not normally distributed and are constrained between a lower and upper bound. However, the validity of the results is dependent on the specification of the imputation equation and requires an assumption that data does not depend on unobserved data (i.e. it is missing at random).
We included the following variables in the imputation equation for the EQ-5D index scores and cost variables (separate variables for index procedure, emergency department, inpatient, day case, outpatient, diagnostic tests and primary care) at the follow-up time points (3, 6, 9 and 12 months):
-
Baseline EQ-5D index score. This was complete and one would expect patients’ quality of life in follow-up to be related to their baseline quality-of-life values. It is also likely that health-care use, and hence costs, is related to the EQ-5D index score as an indicator of health status.
-
Treatment arm (CA or SA), study site and LAD above or below 50 mm. Study site and LAD were stratification variables.
-
Baseline patient characteristics: age, sex and Index of Multiple Deprivation (IMD). Health-related quality of life (HRQoL) and use of health services are expected to differ by age, sex and level of deprivation.
These variables are indicators of the main demographic, socioeconomic, health status and clinical factors likely to be related to missingness. We did consider including ethnicity, but as a categorical variable with little variation (the study population was largely white) the MI model would not converge when this variable was included.
In addition, the equation for the cost of the index admission included LOS and indicators for patients who did not have the index procedure or who did not have the procedure to which they were allocated.
We used 40 sets of imputed data for our base-case analysis.
Total costs and AUC QALYs were calculated at the individual patient level from the MI data sets. Mean costs, utilities and QALYs were then re-estimated using the Stata version 16 ‘mi estimate’ procedures to adjust coefficients and standard errors for the variability between imputations. We used the following procedures to check the face validity and stability of the imputations:
-
Comparison of complete-case and MI estimates of summary statistics (means, ranges and CIs) for the imputed variables.
-
Comparison of histograms for the observed data and randomly selected MI iterations.
-
Comparison of total cost and QALY results with complete-case and MI estimates. This is our preferred approach because it adjusts for the likelihood that data are not missing completely at random, as well as for potential bias due to differences in the baseline characteristics of the study groups. The adjusted MI analysis cannot control for unobserved causes of missing data or differences between the study groups, but we consider it to make the best use of available data.
-
Sensitivity analysis with different random numbers, numbers of imputations and model specifications for the MI.
We present estimated means and CIs for intermediate outcomes measures (including AFEQT and EQ-5D scores at the end of follow-up) and costs (by type of cost and quarter) as well as QALYs and total costs. Estimates are presented for the complete-case and MI analyses.
Differences between the groups were estimated by regression. We used ordinary least squares (OLS) for health outcomes and generalised linear models (GLMs), with distribution and identity link function for costs. For each summary variable, we show three model specifications:
-
A simple regression of complete-case data, including a treatment group indicator (1 for CA and 2 for SA) without covariates.
-
An adjusted regression of complete-case data. For these models, we considered a set of possible covariates, which all included the stratification variables (study site and LAD of > 50 or < 50 mm) and sociodemographic indicators (age, sex and IMD). A relevant baseline value was also tested; this was the baseline EQ-5D index score for QALYs and costs. We tested the inclusion of LOS in the equation for the cost of the index admission, but the GLMs iteration would not resolve. We used backward stepwise regression to select covariates from the predefined list (coefficient p-value < 0.2) and checked that the included covariates improved the model fit, as indicated by adjusted R2 or Akaike information criterion/Bayesian information criterion statistics.
-
The adjusted regression equation with MI estimation. This is our preferred approach.
The key results for the economic analysis are the between-group difference in mean QALYs, the incremental effect (IE), and the between-group difference in mean total costs (the IC). These results would usually be summarised as a ratio: the incremental cost-effectiveness ratio (IC/IE). However, this is not meaningful in a situation, as here, where one intervention yields more QALYs at lower cost than the comparator. We therefore present results as an incremental net benefit (INB) statistic. This is calculated using a ‘willingness-to-pay’ threshold (λ) to place a monetary value on QALYs:
A positive INB indicates that the intervention is cost-effective at the defined threshold, λ. We present INB results calculated at threshold values of £20,000 per QALY gained and at £30,000 per QALY gained, which are the bounds of the range usually used by NICE for the assessment of cost-effectiveness of interventions in the NHS. 67
Non-parametric bootstrap
We also used non-parametric bootstrapping to estimate the joint distribution of costs and QALYs. This is potentially important because health outcomes (QALYs) are often correlated with costs. We used the Stata bsample command to draw 10,000 non-parametric bootstrap samples (random with replacement and stratified by treatment arm), each drawn from a different imputed data set (using the chained MI PMM procedure described in Missing data). This combines uncertainty due to sampling variation with uncertainty over the imputed missing data. The bootstrap results are summarised as 10,000 pairs of IC and IE estimates, which we illustrate graphically on a cost-effectiveness plane. We also estimate the probability that SA is dominated by CA (the proportion of bootstrap samples in which IC is > 0 and IE is < 0) and the probability that SA is cost-effective (the proportion of bootstrap samples in which INB is < 0).
Clinical end-points verification
Heart rhythm data (implanted loop recorder)
Implanted loop recorder data analysis
Heart rhythm data were regularly assessed by a blinded cardiac physiologist based at the Royal Brompton Hospital. Events were classified into three groups: SR, AF/AT or unclassified. Given that we programmed the device to maximise sensitivity for arrhythmia detection, a number of automated events were falsely labelled as AT by the device. Differentiating sinus tachycardia and AT was challenging with a single-lead ECG recording from the ILR. ECG strips that were undetermined were sent for review to two electrophysiology (EP) consultants, who were blinded to the patients’ characteristics and treatment allocation, to help with assessments.
Quality control
We completed a quality control process of ILR data evaluation, which involved an independent review of 10% of all collected data (2700/27,088 ECG traces) by a senior, blinded physiologist at Barts Health NHS Trust. The evaluations from this external, blinded physiologist were compared with the original assessor’s evaluations and showed an interobserver variability rate of 18%. The discordant ECG strips were then assessed by three senior EP consultants, who were also blinded to patients’ characteristics, treatment allocation and the results of earlier evaluations. At the end of the expert group adjudication process, we recorded an interobserver variability rate of 8%.
Importantly, none of the patients’ heart rhythm primary end-point status changed as a result of additional scrutiny.
Based on our quality control processes and end-point verification of ILR data, we calculated that evaluations done by the Royal Brompton physiologist had sensitivity and specificity of 96.4% and 88.1%, respectively. The positive predictive value was 86.6% and the negative predictive value was 96.9%.
Adverse events
All adverse events and their classifications were regularly reviewed by the DMC, which met twice a year. Towards the end of the follow-up period, we also convened an independent expert group to review adverse events in the study. The group consisted of two cardiothoracic surgeons and one EP consultant. They familiarised themselves with the protocol and were each sent a subset of adverse events to classify on their own. The group was blinded to the treatment allocation of the patients whose anonymised data were reviewed. We then scheduled a meeting in person with the Trial Management Group to discuss and classify these events. The aim of the meeting was to ensure that every SAE was identified as such and that its relatedness to the study procedure was ascertained. A consensus outcome was used in cases where a unanimous decision was not reached.
The safety outcome we report in the study is a major complication (SAE) related to the study procedure within 30 days and within the length of the follow-up. The study protocol defined SAEs as those complications resulting in hospitalisation and leading to permanent injury or death. 38
Expected adverse events and their classification, as listed in the PIS and the study protocol, are shown in Table 1.
Adverse events (minor complications) | SAEs (major complications) |
---|---|
Bruising, haematoma, vascular injury not requiring intervention | Vascular complications requiring blood transfusion or intervention |
Pericardial/pleural effusion (observation only) | Symptomatic pericardial/pleural effusion or requiring intervention |
Broken rib | Stroke/transient ischaemic attack |
Pneumothorax requiring observation | Pneumothorax requiring chest drain |
Infection (i.e. pneumonia) | Empyema |
Pulmonary oedema | Myocardial infarction |
Temporary phrenic nerve damage | Permanent phrenic nerve damage |
Pain near surgical sites | PV stenosis (> 50% reduction in diameter from baseline) |
Requirement to insert permanent pacemaker (with or without prior conduction tissue damage) | |
Cardiac trauma requiring surgical intervention | |
Radiation-induced skin damage | |
Oesophageal atrial fistula | |
Death |
Chapter 3 Recruitment, treatment and follow-up
Study recruitment started in September 2015 and was completed in July 2018. The last patient in the trial completed their last follow-up visit in November 2019.
The outcomes of screening and recruitment and patients’ progress through the study are shown in Figure 2.
FIGURE 2.
The CONSORT flow diagram. EF, ejection fraction.
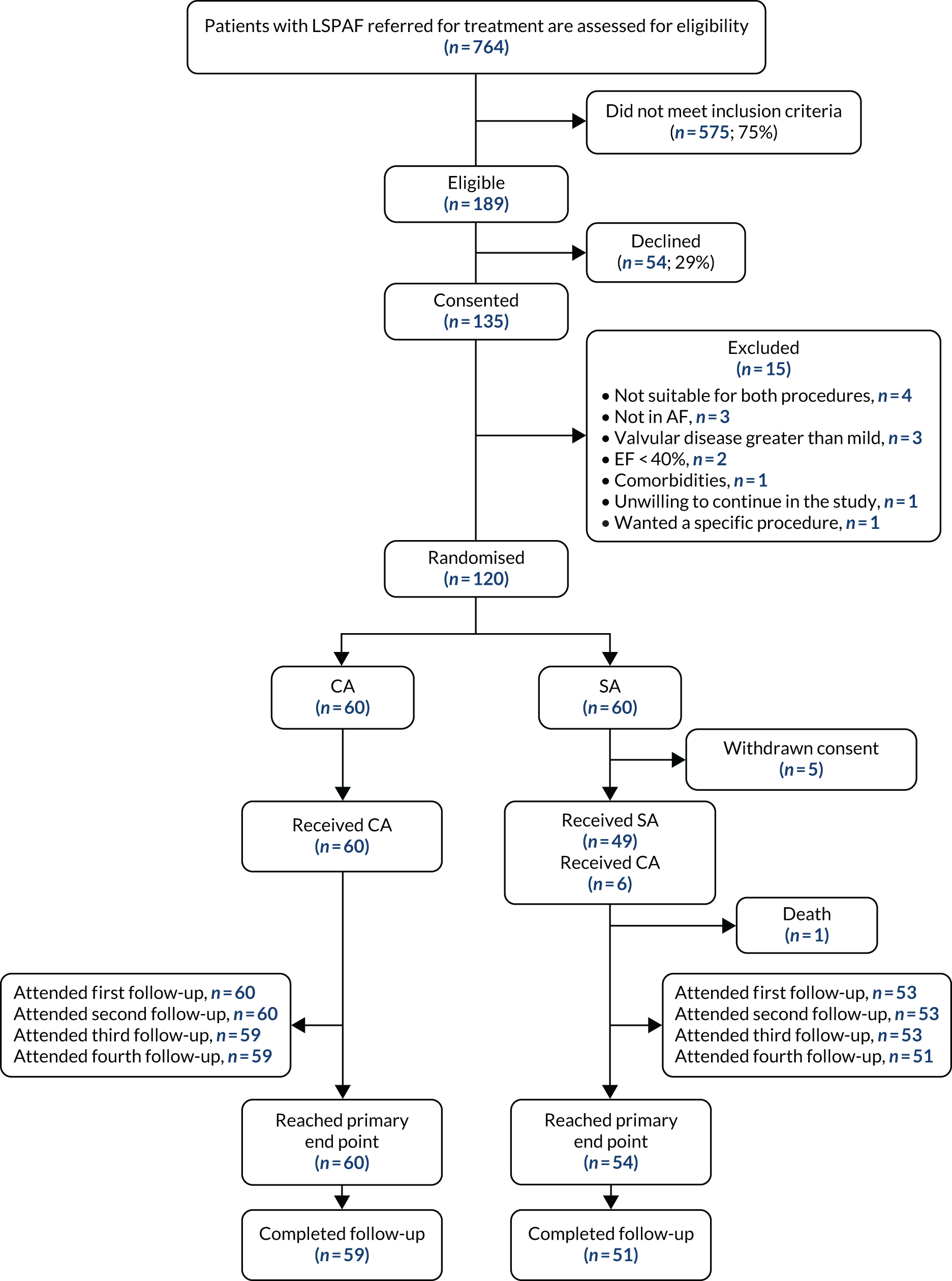
Of the 764 patients with LSPAF referred for DCCV or CA in the four participating hospitals, 575 (75%) did not meet the inclusion criteria based on the screening of medical notes. Previous ablation or cardiac/thoracic surgery accounted for 43% of the screening failures, 18% of patients were not suitable for both treatments, 12% had a low EHRA score and 10% had an ejection fraction (EF) of < 40%. The remaining exclusions at the screening stage were because of comorbidities (including valvular disease) and because patients were already scheduled for CA.
Those deemed eligible (n = 189) were given a PIS to consider taking part in the study. Of those 189, 135 (74%) expressed their interest and agreed to attend a hospital visit for baseline assessments.
Following completion of baseline assessments, we identified 15 patients who did not meet the inclusion criteria (see Figure 2) and 120 patients were subsequently randomised in the study.
Most of the study participants were recruited at the Royal Brompton Hospital (58/120, 48%) and Harefield Hospital (39/120, 33%). Liverpool Heart and Chest Hospital recruited 18 patients (15%) and Brighton and Sussex University Hospital recruited five patients (4%) to the study.
The baseline characteristics of patients randomised in the study are shown in Table 2.
Characteristic | All (N = 120) | Treatment arm | |
---|---|---|---|
CA (N = 60) | SA (N = 60) | ||
Age (years) | 62.3 (9.6) | 60.8 (10.1) | 63.8 (8.9) |
Sex (male), n (%) | 89 (74.2) | 45 (75) | 44 (73.3) |
BMI (kg/m2) (IQR) | 30.2 (27 to 32.8) | 30.6 (27.6 to 33.3) | 29.7 (26.1 to 32.8) |
Townsend Deprivation Index (IQR) | –0.4 (–2.2 to 1.4) | –0.7 (–2.5 to 0.6) | –0.1 (–2 to 1.8) |
IMD score (IQR) | 12.7 (7.7 to 22.3) | 11.1 (5.5 to 21.5) | 14 (8.7 to 22.6) |
Ethnicity, n (%) | |||
White | 112 (93) | 57 (95) | 55 (92) |
Bangladeshi | 1 (0.8) | 0 (0) | 1 (2) |
Other Asian | 0 (0) | 0 (0) | 0 (0) |
Black | 1 (0.8) | 1 (1.67) | 0 (0) |
Middle Eastern | 1 (0.8) | 1 (1.67) | 0 (0) |
African Caribbean | 2 (1.7) | 0 (0) | 2 (3) |
Not stated | 3 (2.5) | 1 (1.67) | 2 (3) |
Systolic blood pressure (mmHg) | 127.9 (16.7) | 126.6 (16.3) | 129.1 (17.2) |
Diastolic blood pressure (mmHg) | 79.8 (12) | 81.5 (13.1) | 78 (10.6) |
EF (%) | 56.9 (8.9) | 55.2 (8.9) | 58.8 (8.7) |
LAD (mm) | 44.6 (5.9) | 44.6 (6) | 44.7 (5.8) |
Diagnosis of persistent AF to randomisation (months) (IQR) | 20 (14 to 30) | 18 (14 to 27.2) | 23.5 (17 to 34) |
Diagnosis of persistent AF to procedure (months) (IQR) | 22 (16 to 31) | 19.5 (15 to 29.2) | 25 (19 to 35.5) |
Medical history, n (%) | |||
Hypertension | 56 (46.7) | 23 (38.3) | 33 (55) |
Diabetes | 9 (7.5) | 4 (6.7) | 5 (8.3) |
Coronary artery disease | 13 (10.8) | 7 (11.7) | 6 (10) |
Stroke, TIA or thromboembolism | 5 (4.2) | 1 (1.7) | 4 (6.7) |
CHA2DS2-VASc score, n (%) | |||
0 | 34 (28.3) | 21 (35) | 13 (21.7) |
1 | 34 (28.3) | 18 (30) | 16 (26.7) |
2 | 27 (22.5) | 11 (18.3) | 16 (26.7) |
≥ 3 | 25 (20.8) | 10 (16.7) | 15 (25) |
HAS-BLED score, n (%) | |||
0 | 23 (19.2) | 12 (20) | 11 (8.3) |
1 | 49 (40.8) | 30 (50) | 19 (31.7) |
2 | 39 (32.5) | 15 (25) | 24 (40) |
≥ 3 | 9 (7.5) | 3 (5) | 6 (10) |
Study participants were, on average, aged 62 years, and 74% were men. Patients had a history of AF lasting around 3 years on average, but were diagnosed with LSPAF (with evidence from Holter monitoring) a median of 20 months (IQR 14–30 months) before randomisation or 22 months (IQR 16–31 months) prior to ablation. All patients were in AF at the time of baseline assessments, as measured by 12-lead ECG.
At study enrolment, 13% of the participants used AADs and all were treated with anticoagulants, as shown in Table 3.
Medications | All (N = 120), n (%) | Treatment arm, n (%) | |
---|---|---|---|
CA (N = 60) | SA (N = 60) | ||
AADs | |||
Amiodarone | 6 (5) | 2 (3.33) | 4 (6.67) |
Dronedarone | 1 (0.83) | 1 (1.67) | 0 |
Flecainide | 6 (5) | 6 (10) | 0 |
Sotalol | 3 (2.5) | 1 (1.67) | 2 (3.33) |
Rate control | |||
Beta-blocker (atenolol/bisoprolol) | 91 (75) | 49 (81.67) | 42 (70) |
Calcium channel blocker (verapamil/diltiazem) | 16 (13.33) | 7 (11.67) | 9 (15) |
Cardiac glycoside (digoxin) | 26 (21.67) | 14 (23.33) | 12 (20) |
Others | |||
ACEi/ARB | 55 (45.83) | 26 (43.33) | 29 (48.33) |
Aldosterone antagonist | 5 (4.17) | 2 (3.33) | 3 (5) |
Calcium channel blocker (amlodipine/felodipine/lercanidipine) | 17 (14.17) | 7 (11.67) | 10 (16.67) |
Diuretics | 21 (17.5) | 10 (16.67) | 11 (18.33) |
Other antihypertensives (bendroflumethiazide, indapamide, alpha-blockers) | 12 (10) | 5 (8.3) | 7 (11.67) |
Statins | 32 (26.67) | 15 (25) | 17 (28.33) |
Anticoagulants | |||
Vitamin K antagonist | 43 (35.83) | 21 (35) | 22 (36.67) |
Factor Xa inhibitors | 76 (63.33) | 38 (63.33) | 38 (63.33) |
Dabigatran | 9 (7.5) | 5 (8.33) | 4 (6.67) |
Antiplatelets | |||
Aspirin | 2 (1.67) | 2 (3.33) | 0 |
Clopidogrel | 2 (1.67) | 1 (1.67) | 1 (1.67) |
Five patients from the SA group withdrew their consent following randomisation (two patients from Royal Brompton Hospital, one from Harefield Hospital and two from Liverpool Heart and Chest Hospital). Their data were included in baseline characteristics analysis.
Before randomisation, baseline assessments were completed an average of 27 (SD ± 36) days after the date of consent. There was considerable variation between trial centres and treatment arms, with most of the delays occurring as a result of difficulties in scheduling echocardiography and magnetic resonance imaging (MRI).
We expected ablation to be scheduled within 4 weeks of randomisation, but it took longer in practice. The median (IQR) time required to schedule the index ablation was 48 days (34–69 days). Mean time to index ablation was significantly longer in the SA arm [63 (IQR 42–100) days] than in the CA arm [41 (IQR 33–54) days; p < 0.001].
Delays in scheduling ablation affected 38 out of 60 (47%) participants in the CA group and 39 out of 55 participants (71%) in the SA group. The main reason for delays in arranging an ablation for our study participants was a long list of clinical cases that were deemed urgent. The other reasons are listed in Table 4.
Reasons for delays | Treatment arm (n) | |
---|---|---|
CA | SA | |
Ablation postponed because of urgent clinical cases | 15 | 20 |
Ablation slots not available | 5 | 6 |
Medical conditions needing further investigation | 3 | 5 |
Patient on holiday | 2 | 2 |
Pause in randomisationa | 2 | 1 |
Thrombus in LA | 1 | 5 |
Six patients randomised to the SA arm could not complete the treatment owing to pericardial or lung adhesions (four patients from Harefield Hospital, one patient from the Royal Brompton Hospital and one patient from Liverpool Heart and Chest Hospital). They underwent CA once they recovered from the attempted surgery and their data are considered as per intention-to-treat analyses. The delays in scheduling the alternative treatment have not been accounted for in Table 4.
Follow-up visits were more regularly distributed throughout the follow-up period and the intervals were, on average, a mean (± SD) of 97 (± 22) days between the first and second follow-up; 90 (± 17) days between the second and third follow-up visit and 96 (± 24) days between the third and fourth follow-up visits. The last study visit was, on average, scheduled a mean (± SD) of 370 (± 27) days after the index ablation in both treatment arms.
As can be seen in Figure 2, patient compliance with study visits following ablation was very good, as 59 out of 60 (98%) participants in the CA arm and 51 out of 55 (93%) participants in the SA arm completed 12 months’ study follow-up.
Ablation characteristics
The median time taken to complete index ablation in the CA group was significantly shorter than in the SA group: 219 (IQR 191.5–261.2) minutes and 260 (IQR 219–307.5) minutes, respectively (p = 0.0019).
However, PV isolation took significantly longer in the CA arm than in the SA arm. The mean (± SD) ablation time for left PVI was 16.4 (± 5.9) minutes in the CA arm, compared with 2.2 (± 1.4) minutes in the SA arm (95% CI 12.7 to 15.8 minutes; p < 0.001). Similarly, the mean (± SD) ablation time for right PVI was, on average, 16.8 (± 7.6) minutes in the CA arm and 2.5 (± 2.2) minutes in the SA arm (95% CI 12.1 to 16.4 minutes; p < 0.001).
Significantly less power was used in the SA arm than in the CA arm to execute all lesions, as shown in Table 5.
Ablation site | Treatment arm | Unpaired t-test | |||
---|---|---|---|---|---|
CA | SA | ||||
n | Mean (± SD) | n | Mean (± SD) | ||
LPV line (duration, minutes) | 60 | 16.4 (5.9) | 48 | 2.2 (1.4) | p < 0.001 |
LPV line (power, W) | 60 | 32 (5) | 44 | 16.6 (3.4) | p < 0.001 |
RPV line (duration, minutes) | 60 | 16.8 (7.9) | 49 | 2.5 (2.2) | p < 0.001 |
RPV line (power, W) | 60 | 31.4 (4.9) | 46 | 15.6 (3.9) | p < 0.001 |
Roof line (duration, minutes) | 59 | 6.2 (4.2) | 49 | 6 (3.9) | p = 0.81 |
Roof line (power, W) | 60 | 31.6 (5.9) | 45 | 18.8 (2.2) | p < 0.001 |
Inferior line (duration, minutes) | 60 | 5.8 (3.7) | 49 | 6.4 (3.8) | p = 0.34 |
Inferior line (power, W) | 60 | 31.9 (13.6) | 45 | 18.5 (3.2) | p < 0.001 |
The mean (± SD) fluoroscopy time in the CA arm was 19.6 (± 12.3) minutes, with a mean (± SD) radiation exposure of 1461 (± 1129) cGy cm2.
In the CA group, electrical isolation of the left PV, right PV, and the cavotricuspid isthmus line was achieved in 97% (58/60) of patients. Electrical isolation of the mitral valve isthmus and box lesion was achieved in 82% (49/60) of patients.
Of the 60 patients randomised to the SA group, 55 underwent treatment and six of those patients had to be treated by CA owing to lung or cardiac adhesions. Of the 49 patients who completed SA treatment, two did not have the complete lesion sets ablated: one patient did not have their left PV isolated and LAA occluded because of unfavourable anatomical features, and another patient did not have their LAA ligated for the same reason.
Electrical isolation in the SA group, tested with a surgical pen, was achieved in 96% (47/49) of patients for left PV, 92% (45/49) of patients for right PV and 88% (43/49) of patients for box lesions. Isolation of ganglionic plexuses was based on positive identification (vagal response) using high-frequency stimulation. Coronary sinus ganglionic plexus (GP) was ablated in 71% (35/49) of patients, right inferior GP in 35% (17/49) of patients and right superior GP in 29% (14/49) of patients. At the end of the procedure there was an abolition of vagal response in all of the ganglionic plexuses.
The time and power required to ablate ganglionic plexuses in the SA group are shown in Table 6.
GP | Patients with lesion (n) | Number of lesions, median (IQR) | Ablation time (minutes), median (IQR) | Power (W), median (IQR) |
---|---|---|---|---|
Left superior | 2 | 2.5 (2.2–2.8) | 0.8 (0.7–0.8) | 20 (20–20) |
Left inferior | 2 | 7 (6.5–7.5) | 0.8 (0.8–0.9) | 20 (20–20) |
Right superior | 14 | 2.5 (2–7.5) | 0.8 (0.6–1.6) | 19.2 (17.5–20) |
Right inferior | 17 | 4 (2–8) | 1 (0.5–1.5) | 20 (17–20) |
Right posterior | 7 | 4 (4–11.5) | 1 (0.7–2.1) | 20 (19.8–20) |
Coronary sinus | 36 | 11 (6.8–16.2) | 1.7 (1.1–2.6) | 20 (15.2–20) |
Left bifurcation | 1 | 4 | 0.5 | 20 |
Right anterior | 1 | 2 | 0.4 | 20 |
Electrophysiological testing in the SA arm was intended to be performed using two methods: first, by multipoint testing using the surgical pen and, second, by multipolar electrophysiological catheter. In our trial, this proved to be a very demanding process that was attempted in 11 patients, but was successful in only eight patients. This was largely because of anatomical features that increased the risk of serious complications (e.g. enlarged superior vena cava). For patients in whom we were able to conduct electrophysiological testing using both methods, multipolar catheter identified only one additional site of PV connectivity than the surgical pen, so we abolished this testing in the remaining procedures.
The median length of hospital stay for patients undergoing SA was significantly longer than for those undergoing CA: 6 (IQR 5–7) days compared with 2 (IQR 2–2) days, respectively. In the SA arm, we had 19 patients (35%) admitted to an intensive treatment unit following the procedure, and they spent, on average, a mean (± SD) of 1.4 nights (± 0.9) there. None of the patients in the CA arm needed admission to an intensive treatment unit. Thirty-six patients in the SA group (66%) spent, on average, a mean (± SD) of 2.5 nights (± 1.6) in a high-dependency unit, compared with just one patient in the CA arm (p < 0.001).
Chapter 4 Efficacy and safety outcomes
Efficacy
At the end of the 12 months’ follow-up, of the 115 patients who had received treatment, 114 were included in the efficacy analyses (as one patient in the SA arm died within 30 days of the procedure). In the SA arm, 26% (14/54) of patients were free from AF/AT after a single procedure and without taking AADs, compared with 28% (17/60) of patients in the CA arm (OR 1.13, 95% CI 0.46 to 2.28; p = 0.84) (Figure 3a).
After one procedure and without taking AADs, the burden of atrial arrhythmias was reduced by ≥ 75% in 36 out of 54 (67%) patients in the SA arm, compared with 46 out of 60 (77%) patients in the CA arm (OR 1.64, 95% CI 0.67 to 4.08; p = 0.3) (Figure 3b).
FIGURE 3.
Proportion of patients (a) free from AF/AT (≥ 30 seconds) and (b) with the burden of AF/AT (≥ 30 seconds) reduced by ≥ 75%, following single procedure from the end of the blanking period to the end of follow-up.


Including patients with AADs, 39 out of 54 (72%) participants in the SA arm and 49 out of 60 (82%) participants in the CA arm had the burden of AF/AT reduced by ≥ 75% after a single procedure (OR 1.71, 95% CI 0.71 to 4.15; p = 0.23).
Sensitivity analyses, including per-protocol and MI technique for missing data and logistic regression (adjusting for variables used during randomisation: sex, trial site and LAD), did not substantially affect these findings (see Appendix 2).
One patient in the study did not have ILR data available for the duration of follow-up owing to non-compliance; therefore, we used the results of their 3-, 6-, 9- and 12-month 12-lead ECGs, which were obtained at clinical follow-up, to establish freedom from AF/AT.
Direct-current cardioversion was required for some patients in both groups: 10 out of 55 (18%) participants in the SA arm and 11 out of 60 (18%) participants in the CA arm (OR 0.99, 95% CI 0.38 to 2.55; p = 0.98).
Patients in both treatment arms underwent additional CA ablation for AF/AT recurrence during follow-up: 10 out of 55 (18%) participants in the SA arm and 9 out of 60 (15%) participants in the CA arm (OR 1.26, 95% CI 0.47 to 3.38; p = 0.65).
Safety
Procedure-related serious complications (SAEs) occurred within 30 days of the ablation in 8 out of 55 (15%) patients in the SA arm and 6 out of 60 (10%) patients in the CA arm (p = 0.46). Some patients had more than one complication: 10 SAEs were recorded in the SA arm and eight SAEs were recorded in the CA arm, as shown in Table 7.
SAE | Treatment arm (n) | |
---|---|---|
CA | SA | |
Anaphylactic shock | 1 | |
Chest infection | 4 | 2 |
Congestive heart failure | 1 | 1 |
Death | 1 | |
Gastric ileus | 1 | |
Hemidiaphragmatic paralysis | 1 | |
Hemidiaphragmatic paresis | 1 | |
Infection | 1 | |
Pacemaker insertion | 1 | |
Pain at wound site | 1 | |
Pleural effusion | 1 | |
Pseudoaneurysm of femoral artery | 1 |
One death in the SA group occurred at 3 weeks post procedure. The patient presented with sepsis complicated by subsequent and progressive multiorgan failure, and bleeding oesophageal and gastric ulcers. The possibility of atrio-oesophageal fistula was considered, but was not confirmed in the post mortem examination.
Procedure-related SAEs (major complications) over the follow-up period occurred in 10 out of 55 (18.2%) patients in the SA arm (14 events) and 8 out of 60 (13.3%) patients in the CA arm (11 events).
Over the follow-up period, significantly more patients with adverse events (major and minor complications) attributable to the study intervention were recorded in the SA arm than the CA arm [22/55 (40%) vs. 9/60 (15%) patients; OR 3.78, 95% CI 1.55 to 9.21; p = 0.003] (Table 8).
Procedure-related adverse event | Treatment arm (n) | |
---|---|---|
CA (N = 9) | SA (N = 22) | |
Acute kidney injury | 2 | |
Anaphylactic shock | 1 | |
Chest infection | 4 | 11 |
Congestive heart failure | 1 | 1 |
Death | 1 | |
Gastric ileus | 1 | |
Hemidiaphragmatic paralysis | 1 | |
Hemidiaphragmatic paresis | 2 | |
Infection | 1 | 6 |
Pacemaker insertion | 2 | 1 |
Pain at wound site | 1 | |
Pleural effusion | 1 | |
Pseudoaneurysm of femoral artery | 1 | |
PV occlusion | 1 | |
Surgical emphysema | 1 | |
TIA | 1 | 1 |
Total number of events | 11 | 31 |
One of the undesired effects of AF ablation is the narrowing of PVs. Using cardiac magnetic resonance, we were able to compare pre- and post-ablation sizes of PVs in 56 patients in the CA arm and 50 patients in the SA arm.
One patient in the SA arm (1/50; 2%) had severe PV stenosis (≥ 50% stenosis of the left inferior PV and occlusion of the left superior PV) that required treatment. The patient remains under regular follow-up. One patient in the CA arm (1/56; 2%) had 50% stenosis of the left inferior PV, as measured by MRI. The patient was asymptomatic and did not require any treatment.
Chapter 5 Quality of life and health economic analyses
We used an intention-to-treat approach for the economic analysis. This included all 120 randomised patients, including five patients randomised to SA who did not receive a procedure and another six patients randomised to SA who received CA.
Health effects
Health-related quality of life
The measures of HRQoL are summarised in Table 9. This includes the disease-specific AFEQT questionnaire for comparison, as well as the generic EQ-5D-5L questionnaire, which is used to estimate QALYs for the economic analysis. The AFEQT overall score and EQ-5D VAS score are both measured on a scale from 0 to 100, with a higher score indicating a better outcome. The EQ-5D index score (UK ‘crosswalk’ value set) has a minimum score of –0.594 and a maximum of 1.000, with a higher score reflecting a better outcome.
Variable | Month | No imputation for missing data | MI | ||||||||
---|---|---|---|---|---|---|---|---|---|---|---|
CA | SA | CA (N = 60) | SA (N = 60) | ||||||||
n | Mean | (95% CI) | n | Mean | (95% CI) | Mean | (95% CI) | Mean | (95% CI) | ||
EHRA | 0 | 60 | 3.00 | (3.00 to 3.00) | 60 | 3.02 | (2.98 to 3.05) | 3.00 | (3.00 to 3.00) | 3.02 | (2.98 to 3.05) |
3 | 60 | 1.63 | (1.45 to 1.82) | 54 | 1.72 | (1.50 to 1.94) | 1.63 | (1.45 to 1.82) | 1.73 | (1.51 to 1.95) | |
6 | 58 | 1.59 | (1.40 to 1.77) | 51 | 1.51 | (1.32 to 1.70) | 1.60 | (1.42 to 1.79) | 1.51 | (1.33 to 1.70) | |
9 | 54 | 1.37 | (1.18 to 1.56) | 52 | 1.60 | (1.38 to 1.81) | 1.35 | (1.18 to 1.53) | 1.63 | (1.41 to 1.85) | |
12 | 59 | 1.34 | (1.21 to 1.47) | 54 | 1.70 | (1.46 to 1.94) | 1.35 | (1.20 to 1.49) | 1.71 | (1.47 to 1.96) | |
AFEQT | 0 | 60 | 52.0 | (48.2 to 55.8) | 60 | 53.1 | (47.8 to 58.3) | 52.0 | (48.2 to 55.8) | 53.0 | (47.3 to 58.7) |
3 | 58 | 77.0 | (72.1 to 81.9) | 52 | 72.8 | (66.5 to 79.2) | 76.9 | (72.1 to 81.8) | 71.3 | (64.6 to 77.9) | |
6 | 58 | 81.4 | (75.8 to 87.0) | 51 | 77.7 | (71.8 to 83.6) | 80.9 | (75.3 to 86.5) | 76.1 | (69.8 to 82.3) | |
9 | 54 | 81.3 | (76.2 to 86.4) | 50 | 79.5 | (73.2 to 85.8) | 81.5 | (76.7 to 86.3) | 78.2 | (72.0 to 84.4) | |
12 | 59 | 84.7 | (80.5 to 88.8) | 54 | 77.4 | (70.9 to 83.8) | 84.5 | (80.3 to 88.6) | 77.6 | (71.2 to 83.9) | |
EQ-5D VAS | 0 | 60 | 64.4 | (59.6 to 69.2) | 60 | 64.2 | (60.0 to 68.5) | 64.4 | (59.6 to 69.3) | 64.2 | (59.7 to 68.8) |
3 | 58 | 76.9 | (72.3 to 81.4) | 52 | 76.1 | (71.5 to 80.7) | 76.9 | (72.3 to 81.4) | 75.9 | (71.3 to 80.5) | |
6 | 58 | 78.6 | (73.8 to 83.4) | 50 | 76.3 | (71.1 to 81.5) | 78.2 | (73.3 to 83.1) | 75.6 | (70.3 to 80.9) | |
9 | 54 | 82.5 | (78.3 to 86.7) | 50 | 79.4 | (74.2 to 84.5) | 82.6 | (78.6 to 86.7) | 78.5 | (73.3 to 83.6) | |
12 | 59 | 83.6 | (79.7 to 87.5) | 54 | 77.8 | (71.8 to 83.8) | 83.3 | (79.4 to 87.3) | 77.7 | (71.7 to 83.7) | |
EQ-5D index | 0 | 60 | 0.72 | (0.67 to 0.76) | 60 | 0.72 | (0.67 to 0.78) | 0.72 | (0.67 to 0.76) | 0.72 | (0.66 to 0.77) |
3 | 58 | 0.84 | (0.80 to 0.88) | 52 | 0.79 | (0.74 to 0.85) | 0.84 | (0.79 to 0.88) | 0.78 | (0.72 to 0.84) | |
6 | 57 | 0.86 | (0.80 to 0.91) | 51 | 0.80 | (0.73 to 0.87) | 0.85 | (0.79 to 0.90) | 0.79 | (0.73 to 0.86) | |
9 | 54 | 0.86 | (0.81 to 0.91) | 50 | 0.79 | (0.70 to 0.87) | 0.86 | (0.81 to 0.91) | 0.78 | (0.69 to 0.86) | |
12 | 59 | 0.88 | (0.84 to 0.91) | 54 | 0.79 | (0.72 to 0.86) | 0.87 | (0.84 to 0.91) | 0.80 | (0.73 to 0.87) | |
QALYs | 0–12 | 51 | 0.85 | (0.82 to 0.88) | 49 | 0.78 | (0.72 to 0.84) | 0.83 | (0.80 to 0.87) | 0.76 | (0.70 to 0.83) |
Completion rates for the three HRQoL measures were good: all randomised patients completed the questionnaires at baseline and 94% (113 out of 120) completed them at the 12-month assessment. All measures show a similar pattern of improvement between the baseline and 3-month assessment, followed by a plateau or small improvement up to the 12-month assessment. Figure 4 illustrates that the disease-specific AFEQT questionnaire detects a larger change from baseline to 3 months than the generic EQ-5D index.
FIGURE 4.
Scores over time for (a) the AFEQT questionnaire; and (b) the EQ-5D-5L index.


Figure 5 further illustrates these trends, alongside results for the EQ-5D VAS score and EHRA symptom scale (which shows the initial improvement in symptoms, which were maintained over the year of follow-up).
FIGURE 5.
Changes in symptoms and HRQoL scores over time. (a) AFEQT questionnaire overall score, no imputation (range 0–100, higher value indicates better quality of life); (b) EQ-5D index, UK ‘crosswalk’ values, no imputation (range –0.594 to 1, higher value indicates better quality of life); (c) EHRA symptom scale, no imputation (range 1–4, higher value indicates worse AF symptoms); and (d) EQ-5D VAS, no imputation (range 0–100, higher value indicates better quality of life).




Quality-adjusted life-years
Quality-adjusted life-years are the main outcome for the economic analysis, measured as the area under the mean EQ-5D index score curve over the 1-year follow-up period. The proportion of randomised patients with complete EQ-5D index data at all time points required for the QALY calculation (0, 3, 6, 9 and 12 months) was 83% (100/120).
Without imputation of missing data, estimated QALYs were 0.85 (95% CI 0.82 to 0.88) for CA and 0.78 (95% CI 0.72 to 0.84) for SA (see Table 9). With MI of missing EQ-5D index data, QALY estimates were slightly smaller in both treatment arms: 0.83 (95% CI 0.80 to 0.87) and 0.76 (95% CI 0.70 to 0.83) for CA and SA, respectively. We tested the robustness of results to changes to the random number seed and MI equation (see Validation checks).
Note that the differences between treatment arms in Table 9 are not adjusted for individuals’ baseline EQ-5D index values, which is important for the comparison of QALY differences between arms. 74 Adjusted estimates of the between-arm difference are reported as mean QALYs.
Health-care costs
Cost of the index admission
The mean LOS and costs of the index admission for the intervention procedure (CA or SA) are summarised in Table 10. The SA arm includes data for five patients who did not receive a procedure and another six patients who received CA. All patients in the CA arm received the intervention to which they were randomised.
Variable | No imputation for missing data | MI | ||||||||
---|---|---|---|---|---|---|---|---|---|---|
CA | SA | CA (N = 60) | SA (N = 60) | |||||||
n | Mean | (95% CI) | n | Mean | (95% CI) | Mean | (95% CI) | Mean | (95% CI) | |
Index admission | ||||||||||
Days (n) | 60 | 1.57 | (1.10 to 2.04) | 55 | 5.33 | (4.54 to 6.11) | 1.57 | (1.10 to 2.04) | 5.33 | (4.54 to 6.11) |
Cost (£) | 34 | 14,469 | (13,076 to 15,862) | 36 | 18,129 | (15,219 to 21,039) | 15,661 | (13,704 to 17,617) | 20,000 | (17,798 to 22,202) |
Other costs (£) | ||||||||||
Emergency | 54 | 79 | (24 to 134) | 49 | 93 | (41 to 145) | 75 | (25 to 125) | 104 | (51 to 156) |
Inpatient | 54 | 731 | (303 to 1160) | 49 | 1118 | (588 to 1647) | 750 | (332 to 1168) | 1,117 | (605 to 1629) |
Day case | 54 | 275 | (124 to 426) | 49 | 388 | (129 to 648) | 266 | (128 to 405) | 435 | (181 to 688) |
Outpatient | 40 | 308 | (213 to 402) | 44 | 380 | (259 to 500) | 290 | (212 to 367) | 387 | (278 to 495) |
Tests | 54 | 209 | (123 to 296) | 49 | 222 | (145 to 299) | 238 | (145 to 330) | 289 | (190 to 389) |
Primary care | 39 | 86 | (49 to 122) | 44 | 152 | (104 to 199) | 84 | (53 to 114) | 154 | (107 to 200) |
Medicines | 60 | 824 | (190 to 1458) | 60 | 703 | (373 to 1033) | 824 | (190 to 1458) | 735 | (380 to 1091) |
Total other | 39 | 2739 | (1524 to 3953) | 44 | 3129 | (2379 to 3879) | 2526 | (1681 to 3371) | 3221 | (2529 to 3913) |
Total costs | 20 | 17,377 | (14,928 to 19,827) | 25 | 23,539 | (21,841 to 25,236) | 18,186 | (16,017 to 20,356) | 23,221 | (20,975 to 25,466) |
Data on LOS for the index admission were available for 96% (115/120) of randomised patients. Based on the observed data, mean LOS was 1.57 (95% CI 1.10 to 2.04) days in the CA group and 5.33 (95% CI 4.54 to 6.11) days in the SA group. Estimates were similar with MI of missing data.
Data on the cost of the index admission were available from hospital HRG coding for 58% (70/120) of randomised patients. For these patients, the mean admission cost was £14,469 (95% CI £13,076 to £15,862) in the CA arm and £18,129 (95% CI £15,219 to £21,039) in the SA arm. The mean estimates were higher in both treatment arms with MI than in the complete-case analysis.
Post-discharge health-care use and costs
Estimates of costs for health services received over the 12-month post-procedure period are summarised in Table 10 and Figure 6. These estimates include costs for emergency, inpatient and day-case procedures, including cardioversion and CA for recurrent arrhythmia, as well as outpatient visits and tests, primary care services and medications. The social care costs reported were negligible (one patient reported being given a walking stick by the hospital), so they have not been included in total cost estimates.
FIGURE 6.
Cost estimates by (a) health-care category and (b) timing (excluding index admission).
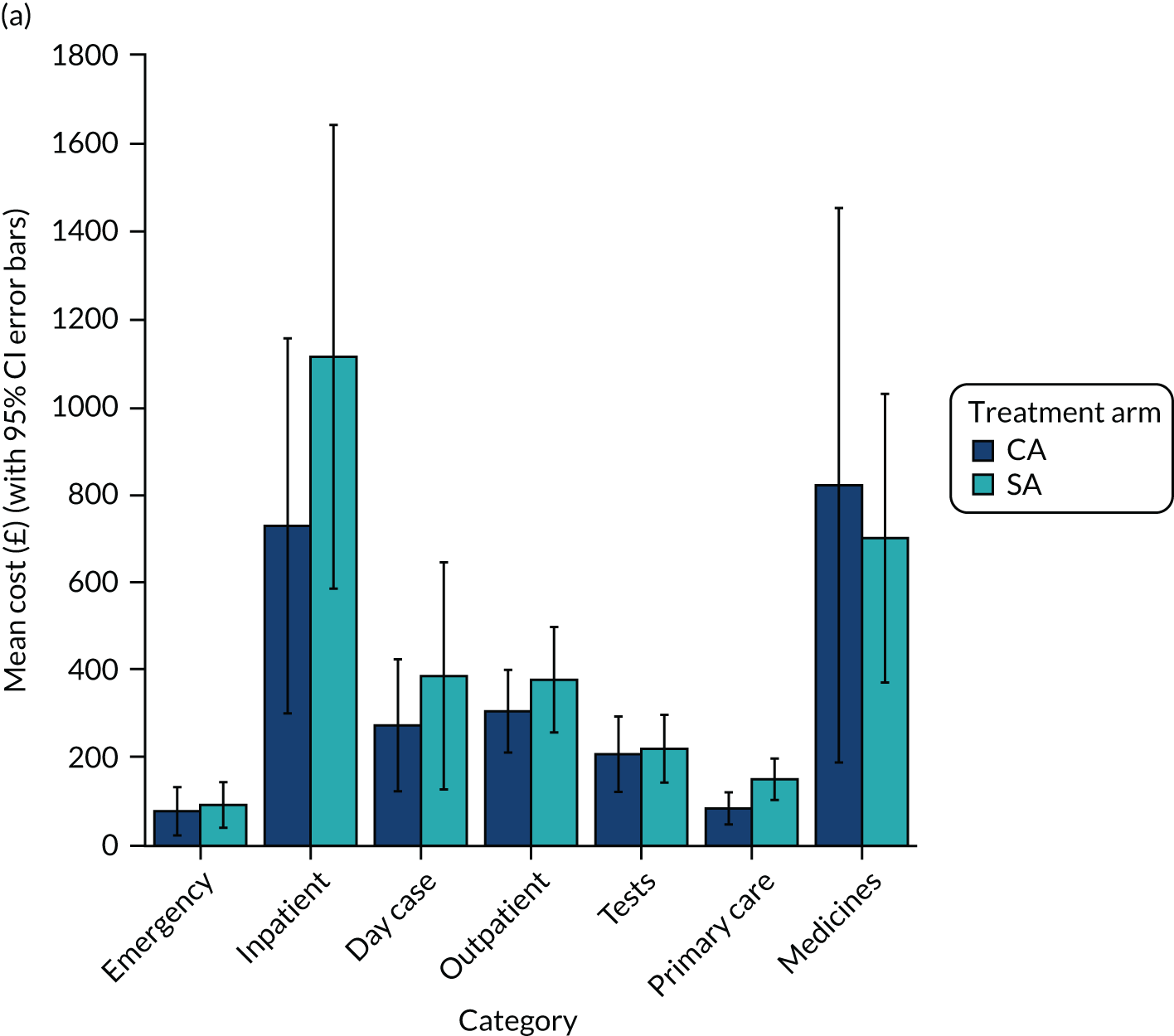

In total, other health-care costs over the year of follow-up were estimated at £2739 (95% CI £1524 to £3953) in the CA group and £3129 (95% CI £2379 to £3879) in the SA group. Estimates are similar with MI.
Total cost
The number of patients with complete-cost data for the index admission and all other health-care categories was small: only 37.5% (45/120) of randomised patients. It is unclear how well this minority reflects costs for the total population. (For further details, see Discussion and Validation checks.)
Incremental analysis
Estimates of mean differences between the treatment arms for selected health and economic outcomes are shown in Table 11. The outcomes reported are measures of symptoms and quality of life at 12 months: QALYs over 1-year follow-up; LOS and cost for the index admission and other services; and net benefits at cost-effectiveness thresholds of £20,000 per QALY gained and £30,000 per QALY gained. Table 11 shows incremental treatment effects on these variables for three alternative model specifications: a simple complete-case analysis with no covariates; an adjusted complete-case analysis, including only those covariates from a limited list that improved model fit (baseline variable value, age, sex, IMD, study site and LAD); and the adjusted model with MI of missing data values.
Variable | Unadjusted, no imputation | Adjusted, no imputation | Adjusted with MI | ||||||
---|---|---|---|---|---|---|---|---|---|
Mean | (95% CI) | p-value | Mean | (95% CI) | p-value | Mean | (95% CI) | p-value | |
Health outcomes | |||||||||
EHRA at 12 months | 0.834 | (0.08 to 1.59) | 0.03 | 0.894 | (0.11 to 1.68) | 0.03 | 0.914 | (0.13 to 1.69) | 0.02 |
AFEQT at 12 months | –7.30 | (–14.7 to 0.14) | 0.05 | –7.44 | (–14.3 to –0.62) | 0.03 | –6.58 | (–13.3 to 0.18) | 0.06 |
VAS at 12 months | –5.81 | (–12.8 to 1.14) | 0.10 | –5.77 | (–12.2 to 0.63) | 0.08 | –5.10 | (–11.5 to 1.31) | 0.12 |
Utility at 12 months | –0.083 | (–0.16 to –0.01) | 0.04 | –0.083 | (–0.15 to –0.02) | 0.01 | 0.076 | (–0.14 to –0.01) | 0.02 |
QALYs 0–12 months | –0.072 | (–0.14 to 0.00) | 0.04 | –0.066 | (–0.13 to –0.01) | 0.03 | –0.069 | (–0.13 to –0.01) | 0.02 |
Resources and costs | |||||||||
LOS (days) | 3.76 | (2.42 to 5.10) | < 0.001 | 3.87 | (2.79 to 4.96) | < 0.001 | 3.76 | (2.42 to 5.10) | < 0.001 |
Procedure (£) | 3660 | (664 to 6655) | 0.02 | 3660 | (664 to 6655) | 0.02 | 2673 | (4 to 5342) | 0.05 |
Other costs (£) | 390 | (–997 to 1778) | 0.58 | 562 | (–618 to 1742) | 0.35 | 940 | (–33 to 1914) | 0.06 |
Total cost (£) | 6162 | (3302 to 9021) | < 0.001 | 6162 | (3302 to 9021) | < 0.001 | 3399 | (517 to 6282) | 0.02 |
Net benefits (£) | |||||||||
At £20,000/QALY | –6164 | (–9782 to –2547) | < 0.001 | –6193 | (–9425 to –2961) | < 0.001 | –4801 | (–8124 to –1477) | 0.01 |
At £30,000/QALY | –6166 | (–10,427 to –1905) | 0.01 | –6624 | (–10,261 to –2986) | < 0.001 | –5495 | (–9139 to –1852) | < 0.001 |
The simple complete-case analysis indicates that, compared with CA, SA is associated with a small but statistically significant loss of 0.072 QALYs (95% CI 0 to 0.14) and an additional cost of £6162 (95% CI £3302 to £9021). This suggests that CA is ‘dominant’: it is less expensive and more effective than SA.
Only the baseline EQ-5D index score improved the fit of the QALY model. This gave a smaller estimate of the incremental QALY loss with SA: 0.066 (95% CI 0.01 to 0.13). None of the tested covariates improved the fit of the cost model.
As discussed above, less than half of the randomised patients had complete-cost data, and mean cost estimates differ with and without imputation of missing data (see Table 11). With MI and adjustment of QALYs for baseline EQ-5D index, SA is associated with an estimated QALY loss of 0.069 (95% CI 0.01 to 0.13) and an additional cost of £3399 (95% CI £517 to £6282).
We note that, although the absolute incremental QALY and cost estimates vary, the directions of effect are consistent and of a similar magnitude across the alternative model specifications that we tested. Additional model verification checks are described in Validation checks and Non-parametric bootstrap.
Non-parametric bootstrap
The above analysis estimates costs and QALYs independently. We also used non-parametric bootstrapping to produce a distribution of joint estimates of ICs and incremental QALYs. The analysis integrated MI of missing EQ-5D and cost data with a different MI data set per bootstrap iteration (using the same MI methods as in previous analyses). QALYs were adjusted for baseline EQ-5D index scores.
The results from 10,000 bootstrap/MI iterations are shown in Figure 7. This figure illustrates the negative correlation between ICs and IEs: iterations with a larger mean QALY loss are associated with a higher mean additional cost.
FIGURE 7.
Non-parametric bootstrap estimates of ICs and incremental QALYs. Mean differences: SA compared with CA.

The distribution of estimates, with most being in the upper-left quadrant of the cost-effectiveness plane, suggests a high probability (99.1%) that SA is more expensive and less effective than CA at a cost-effectiveness threshold of £20,000 per QALY gained. At this threshold, the estimated probability that SA is cost-effective is 0.04%.
Validation checks
The results were robust to alternative MI scenarios, as shown in Table 12. Different specifications of the MI prediction equation led to modest changes in the magnitude of cost and QALY differences; however, the overall conclusion that SA is dominated by CA does not change across scenarios.
Scenario | IEa (QALYs) | ICb (£) | INB at £20,000/QALY | INB at £30,000/QALY | |
---|---|---|---|---|---|
1 | Base case | –0.0689 | 3399 | –£4801 | –£5495 |
2 | MI random number seed: 14,967 | –0.0670 | 3364 | –£4719 | –£5396 |
3 | MI random number seed: 74,302 | –0.0671 | 3508 | –£4869 | –£5546 |
4 | MI imputations: 80 | –0.0689 | 3418 | –£4813 | –£5507 |
5 | MI PMM neighbours: 10 | –0.0648 | 3060 | –£4380 | –£5031 |
6 | MI equation: no covariates | –0.0709 | 2252 | –£3698 | –£4394 |
7 | MI equation: EQ-5D-5L index score covariate only | –0.0688 | 2513 | –£3918 | –£4588 |
8 | MI equation: separate per variable | –0.0675 | 3769 | –£4569 | –£4721 |
9 | Truncated OLS for EQ-5D-5L index score | –0.0754 | 3581 | –£5115 | –£5863 |
10 | Truncated OLS for costs | –0.0634 | 5967 | –£7244 | –£7886 |
Discussion
The economic evaluation was based on direct observations of health outcomes and costs over the trial period. We found consistent patterns of change over time for different outcome measures, including AF symptoms (EHRA checklist), AF-related quality of life (AFEQT questionnaire) and generic measures of quality of life (EQ-5D VAS and index score). Improvements in the first 3 months were sustained up to the end of follow-up at 12 months. There were also small, non-significant, but consistent, trends between the treatment arms, with slightly larger gains for patients randomised to CA than to SA. We believe that the improvements were due to the significant reduction in AF/AT burden following ablation, with subsequent symptom reduction. Over the year, CA was associated with a statistically significant gain of 0.069 QALYs (95% CI 0.01 to 0.13) and an NHS cost saving of £3399 (95% CI £517 to £26,282) per patient compared with SA. This translates to an INB of £4801 (95% CI £1477 to £8124) for CA compared with SA at a conservative cost-effectiveness threshold of £20,000 per QALY. The estimated probability that CA is less expensive and more effective than SA at this threshold is 99.1%. This conclusion would not change with modelling because extrapolation of costs and quality-of-life effects measured in the trial would increase the advantage of CA over SA, and there is no indication in the clinical results reported earlier that there are other longer-term benefits of SA due to extended freedom from arrhythmia or reduction of other risks. Consequently, we have not conducted the model-based analysis at this time.
The results of the economic evaluation were robust to alternative methods of estimation. Rates of follow-up and data completion were good for quality-of-life and cost questionnaires, although there is some uncertainty over the QALY and total cost estimates, which rely on data from several time points. Cost estimates for the index admission were based on individual hospital HRG coding, which is a strength as it reflects variations in LOS and additional procedures and tests for some individuals. However, it was available for only 58% of randomised patients from one trust, so may not be generalisable. Nevertheless, the conclusion that SA is more expensive than CA is likely to be robust. SA is clearly associated with a longer admission: a mean increase of 3.8 days (95% CI 2.4 to 5.1 days). We did not find a reduction in other NHS costs in the following year; in fact, cost estimates were £940 higher in the SA group than in the CA group (95% CI –£33 to £1914).
Chapter 6 Left atrial structure and function assessed by advanced echocardiography
Background
The LA modulates ventricular filling and contributes to cardiac output through its reservoir, conduit and contractile function. Left atrial reservoir function is the elastic ability of the walls of the atrium to stretch during LA filling. The left atrial conduit function is the passive recoil of the LA during early emptying of blood from the LA into the left ventricle (LV). The left atrial contractile function (booster) is the active emptying of blood from the LA to the LV, achieved by contraction of the LA. These functions can be impaired as a result of advancing age and health issues, such as obesity, diabetes, cardiovascular diseases, and renal, respiratory and endocrine disorders. 75–90 AF is another factor associated with poor left atrial function or left atrial remodelling manifested by LA enlargement. 91–94 Duration of AF is positively correlated with worsening of left atrial function,86,95–98 so early assessment and intervention may arrest progression of left atrial remodelling.
Sinus rhythm restoration therapy leads to reduction in the size of the LA and improvements in its function, the process known as reverse remodelling (RRM). It is thought that, in LSPAF patients, the advanced electroanatomical remodelling, along with metabolic and neurohormonal alterations, cause irreversible changes in the LA through fibrosis and post-ablation stunning, which often accompanies early recurrences of AF following ablation. 89,90,99,100
Timely assessment of the left atrial function helps determine the best treatment strategy, as well as the success of ablation87,101–104 or cardioversion. 105–109 Evaluating left atrial reservoir, conduit and contractile function can also be helpful in predicting left atrial electromechanical stunning, which can lead to thromboembolic events. 110–112
Left atrial function can be evaluated by measuring changes in left atrial volumes (maximum, minimum and volume at P wave) or atrial tissue displacement and deformation at the different ECG-derived stages of the cardiac cycle that are linked with mechanical motion related to left atrial relaxation and contraction. 109 Transthoracic echocardiography supports a comprehensive assessment of left atrial function by (1) deriving left atrial volumes by two-dimensional (2D) imaging, (2) measuring left atrial myocardial velocities by tissue Doppler imaging (TDI) and (3) assessing left atrial myocardial strain and strain rate by 2D speckle-tracking echocardiography. 87,109,113–116
Tissue Doppler imaging measures myocardial wall tissue velocities as opposed to blood flow velocities.
Using pulsed-wave TDI at different regions of interest, it is possible to assess both regional and global changes in left atrial function. During left atrial filling, the peak atrial myocardial velocity at a given region of interest in the left atrial wall is called the expansion index and is a measure of reservoir function. The peak left atrial myocardial velocity during the passive emptying phase is a measure of conduit function, and the peak left atrial tissue velocity during contraction is termed the booster function, a measure of contractile function. 109,117–119
In addition, pulsed-wave TDI velocities measured at two different regions of interest can be used to assess myocardial deformation, quantified as strain and strain rate. Strain refers to the change in myocardial length during a given time period in the cardiac cycle, whereas strain rate refers to the rate of change in myocardial length.
Measurement of left atrial myocardial tissue velocities has shown reduced function of the atria in atrial myopathies, diastolic impairment, atrioventricular valvular heart disease, atrial pressure overload and heart failure. 118–120 Myocardial tissue velocities of the LA have been shown to predict new-onset AF and AF recurrences following cardioversion and ablation. 121–124 In addition, TDI has been validated to calculate total atrial conduction time (TACT), which is related to the incidence of new-onset AF in post-SA patients and recurrence in patients known to have persistent AF. 123,124 TACT is defined as the duration from the onset of the P wave to the peak atrial contraction velocity at the lateral left atrial wall. 125,126 Atrial asynchrony, assessed by comparing regional differences in TACT, has been shown to be associated with low atrial voltages during CA and higher rates of AF recurrence. 122
A major limitation of TDI is that it is angle dependent, such that the sample tissue has to be parallel to the probe for accurate measurement of tissue velocities and strain assessment. 127 Two-dimensional speckle-tracking echocardiography has overcome these limitations by being independent of the direction of movement, and is not affected by volume changes and preload conditions. 128,129 Speckle tracking has allowed for strain and strain rate measurements of myocardial tissue to be analysed more accurately among the general population. 128,129 Speckle points of the heart are tagged and the anatomical change in movement is measured as a difference between two points on the myocardium. 128,129 Speckle tracking has limitations owing to factors such as the width of interest, frame rates of 2D acquisition (which need to be > 55 per second) and gating either the onset of the P wave or the R wave of the cardiac cycle. 130,131 These limitations can be improved with increased averaging of cardiac cycles and by reducing the width of interest that improves resolution of the myocardium. A recent meta-analysis by Pathan et al. 131 did not show any variations between P–P-wave or R–R-wave measurements of left atrial strain. Moreover, components of left atrial function are more reproducible with 2D strain, with fewer confounding factors than left atrial volume or TDI measurements. Strain values may also predict new-onset AF and AF recurrence before and after ablation. 96,107,132–137
A number of studies used left atrial volume, TDI and strain assessments to investigate changes in LA anatomy and function, but they were mostly performed in patients with earlier stages of AF (i.e. paroxysmal and persistent). 86,96,103,133,138,139 These studies also reported changes following specific treatments, cardioversion or ablation.
In this study, we present the results of left atrial function assessments in patients with LSPAF, pre and post ablation. We also investigate whether or not CA and SA affect the left atrial function in different ways, and report on the outcomes related to these treatments and the recurrence of AF.
The two ablative techniques used in the trial did not deliver identical lesion sets. Specifically, the CA lesion set included mitral isthmus and cavotricuspid line lesions, which were not performed in the SA arm. Ablation of ganglionic plexuses and mechanical isolation of the LAA were performed in the SA arm only. These lesions may affect left atrial RRM in different ways by acting on specific, regional left atrial wall functions (i.e. septal, lateral, anterior and inferior LA segment), which can be assessed using echocardiography.
Our comprehensive assessments of left atrial function therefore include 2D volume measurements, TDI velocities and 2D speckle-tracking strain and strain rate.
The main objectives of these exploratory analyses were to:
-
assess the effects of CA and SA on left atrial structure and function using TTE-derived 2D volumes, tissue Doppler velocities and speckle-tracking strain and strain rate imaging
-
compare left atrial structure and function in study participants who sustained SR compared with study participants with recurrent paroxysms of AF
-
assess the effects of ablation of the mitral isthmus line in CA and mechanical LAA occlusion in SA on regional left atrial function using TDI.
Methods
Population
Patients with LSPAF enrolled in the CASA-AF randomised controlled trial (RCT) underwent SA or CA (n = 115) and were followed up for 12 months. As part of the study assessments, echocardiographic measurements of the LA parameters were completed at baseline, and at 3 and 12 months after the ablation. Continuous heart rhythm monitoring was enabled by ILR (Reveal LINQ), as described in Implanted loop recorder.
Echocardiography
Transthoracic echocardiography was performed at baseline, at an average of 48 days (range 34–68.5 days) prior to ablation, and then at 3 and 12 months following ablation using Philips IE33 (Andover, MA, USA) and GE Vivid E9 and E95 machines (Chicago, IL, USA). All images were acquired using the standard minimum data set recommended by the British Society of Echocardiography. 140 In addition to the minimum data set, images of the LA were acquired to enable regional TDI measurements. The LAA is not clearly seen on TTE and, therefore, its volume was not calculated.
All measurements in follow-up were completed in patients who were in SR at that time point so that all aspects of left atrial function, in particular left atrial contractile function, could be evaluated. Images were obtained with loops of at least three cardiac cycles to ensure averaging of measurements, which was particularly important for pre-ablation scans when patients were in AF. Left atrial function was assessed by calculating reservoir, conduit and contractile function using echo modalities of left atrial volume, TDI and strain imaging as described below.
Left atrial volumes
Images were acquired over three cardiac cycles, with a minimum frame rate of 55 Hz. The width and depth of the acoustic window was set to allow visualisation of the whole LA to its base. The gain was adjusted to allow clear distinction between blood pool and myocardial tissues.
Left atrial volumes were calculated based on the biplane area–length method and measurements were performed manually as shown in Figure 8. Left atrial volume measurements included the left atrial maximal volume (LAmax), left atrial minimal volume (LAmin) and volume at the onset of the P wave (LA P wave). LAmax was defined as the volume of the LA just before mitral valve opening. LAmin was the volume at the beginning of mitral valve closure and electrically defined as the beginning of the QRS (Q wave, R wave, S wave) on the ECG. LA P wave was the volume at the onset of the P wave on the ECG. In patients with AF, only LAmax and LAmin were measured because of the absence of a P wave; in SR it was possible to measure all three components. Patients for whom there were inadequate views to take all three left atrial volume measurements (i.e. LAmax, LAmin and LA P wave) and for whom their LA endocardial outline could not be reliably determined were excluded from the study.
FIGURE 8.
Left atrial volume calculation using the biplane area–length method. A, area; L, length.


The echocardiographic measurements for each left atrial volume data set were as follows:
These volumetric measurements were then used to derive left atrial reservoir, conduit and contractile function using the equations below:
An illustration of the three components of left atrial function is given in Figure 9.
FIGURE 9.
Three components of the left atrial function using volume indices during SR.

Left atrial reservoir function represents left atrial expansion, the percentage increase in left atrial volume from its minimum volume to its maximum volume. Left atrial conduit function represents passive emptying of the LA, the percentage reduction in left atrial volume from its maximum volume to the volume just before P-wave onset (active atrial contraction). Left atrial contractile function represents active atrial contraction, the percentage reduction in volume from the onset of the P wave to the LA minimum volume (end of contraction).
Left atrial strain and strain rate by two-dimensional speckle-tracking echocardiography
Left atrial strain and strain rate were assessed on standard 2D images. The patient had ECG gated images taken with loops of cardiac cycles per acquisition. The images were acquired once the frame rate in two dimensions was at least 50 Hz and sector widths were reduced to focus on the area of interest. The images were read offline using TomTec (TomTec Imaging Systems, Unterschleissheim, Germany) software to measure atrial strain and strain rate. The image analysis in TomTec was conducted by one experienced operator to minimise variability. TomTec has a semiautomatic process of analysing strain, and it was important to ensure that the resolution and grey scale were of adequate quality to delineate the LA endocardial border. The semiautomatic process was manually adjusted where needed to ensure that all cardiac cycles were tracked appropriately along with the correct demarcation of end-systolic and end-diastolic periods. Once accepted, the strain and strain rate measurements were derived automatically from the software.
Left atrial strain (Figure 10a) and strain rate (Figure 10b) were assessed during the reservoir, conduit and contractile phases of left atrial function. Strain measurements reflected the peak percentage change in the length of the left atrial myocardium during the three phases of the cardiac cycle (SSR – reservoir strain; ESR – conduit strain; ASR – contractile strain), whereas strain rate was the peak change in length per second [SSR′ (two-dimensional left atrial reservoir strain rate); ESR′ (two-dimensional left atrial conduit strain rate); ASR′ (two-dimensional left atrial contractile strain rate)].
FIGURE 10.
Reservoir, conduit and contractile functions in a patient in SR. (a) Left atrial strain and (b) strain rate. a, Reservoir – baseline 0 to peak of LA curve; b, conduit function – peak left atrial strain to pre-atrial contraction; c, contractile function – peak of conduit to baseline 0; d, peak left atrial systolic strain rate; e, peak negative early diastolic strain rate; f, peak negative late diastolic strain rate. The orange boxes delineate the period in which the measurements were made for (a) strain and (b) strain rate.


Left atrial tissue Doppler imaging
Tissue Doppler images were acquired in the apical four-chamber and two-chamber views using a 3.5- to 5.5-MHz frequency probe with Nyquist limits of 10–20 cm per second velocities. The frame rate of the acquisition was maintained above 100 frames per second. The left atrial wall under interrogation was centred in the images to ensure that the wall was parallel to the probe. Once the images were of adequate quality, pulsed-wave TDI was obtained for at least two cardiac cycles to minimise variability in measurements. The sampling was taken from the atrial tissue just below the level of the mitral valve annulus so that annular velocities were not captured. The septal, lateral, anterior and inferior left atrial walls were examined and TDI velocities from each of these walls were then averaged to provide a global score for each parameter.
Figure 11 illustrates TDI velocity measurements. Left atrial SSR is the peak LA velocity during LA filling and therefore represents left atrial reservoir function; LA ESR is the peak LA velocity during passive filling and represents conduit function; LA ASR is the peak LA velocity during atrial contraction. Finally, we included S′ (mitral annular velocity during onset of ventricular systole) as a measure of the effect of mitral valve closure at the isovolumic left atrial contraction period just before LA diastole. The hypothesis is that if left atrial pressures are low, this may be reflected in a higher S′ created by mitral valve closure. Similarly, in instances of increased left atrial pressure the S′ should be reduced.
FIGURE 11.
Pulsed-wave tissue Doppler of the inferior atrial wall below the mitral valve annulus. Mitral annular velocity during onset of ventricular systole (S′), left atrial reservoir (LA SSR), left atrial conduit (LA ESR) and left atrial contractile function (LA ASR).

Finally, TACT was measured from the onset of the P wave on the ECG to the peak A wave (LA ASR) in the lateral wall of the LA.
The effect of LAA occlusion on left atrial function in patients who had SA was measured by comparing anterior wall function to other walls, considering that the clip might have a greater impact on LA anterior wall function.
Similarly, we evaluated the effect of LA mitral isthmus line ablation in patients who had CA by comparing the LA inferior and lateral wall functions.
Statistical analyses
The analyses presented in this chapter are exploratory in nature and are limited because of the small sample size.
Continuous variables with normal distribution are shown as means with SD and 95% CIs. Comparison of demographic and echocardiographic data between treatment groups was performed using Student’s t-test, analysis of variance for baseline data and analysis of covariance for follow-up data to adjust for baseline characteristics and estimates of effect size. Continuous variables not normally distributed are shown as median and IQRs, and significance testing was performed using the Mann–Whitney U-test. Categorical values are expressed as percentages and comparisons were made using a chi-squared test. A comparative analysis of data from patients who remained in SR throughout the study versus those who developed recurrences of AF was also performed in a similar fashion. Receiver operating characteristics (ROCs) curves were used to calculate the AUC of the variable associated with AF recurrence. Using the ROC analysis, the optimal cut-off values for the variables associated with AF recurrence were calculated using Youden’s index. A Cox regression model was used to calculate hazard ratios and 95% CIs for variables associated with AF recurrence. Kaplan–Meier curve analysis was used to show freedom from AF recurrence at the optimal cut-off threshold of the factor derived from ROC curve analysis. The analyses were performed with IBM SPSS Statistics version 25 (IBM Corporation, Armonk, NY, USA).
Results
Left atrial volumes and volume-derived function in catheter ablation and surgical ablation groups
The analyses in this section are based on TTE data from 59 patients, representing only 51% of the participants in the CASA-AF trial who underwent CA (n = 60) or SA (n = 55) as part of the study. The main reason for the reduced sample size in these analyses is the requirement for the patients to have good quality TTE scans from all three study time points.
Of 59 patients in these analyses, 33 had CA and 26 had SA. Demographics and clinical characteristics at baseline were similar in both treatment arms (see Appendix 3, Table 31) and did not differ from those of the rest of the trial population.
Comparisons of left atrial volumes and function in the two treatment arms
We observed significant reduction in volumes of the LA, LAmax and LAmin following ablation in both treatment arms.
Reduction in LAmax and LAmin in the CA arm was evident at 3 months and plateaued at 12 months (Table 13). Left atrial reservoir function and left atrial EF increased significantly at 3 months, and these changes were maintained at 12 months. Left atrial conduit function, LA P-wave volume, and contractile function did not change at follow-up. Compared with baseline values, there was a graded increase in LA sphericity index during follow-up, which reached statistical significance at 12 months.
Parameter and time point | Treatment arm, mean ± SD (95% CI) | p-value | |
---|---|---|---|
CA (n = 33) | SA (n = 26) | ||
LA sphericity index | |||
Baseline | 0.68 ± 0.09 (0.64 to 0.71) | 0.69 ± 0.08 (0.66 to 0.73) | 0.44 |
3 months | 0.71 ± 0.09 (0.68 to 0.75) | 0.72 ± 0.08 (0.69 to 0.75) | 0.94 |
12 months | 0.72 ± 0.10 (0.72 to 0.78) | 0.73 ± 0.07 (0.70 to 0.76) | 0.3 |
LAmax (ml/m2) | |||
Baseline | 51.4 ± 16.4 (45.6 to 57.2) | 49.6 ± 13.1 (44.3 to 54.8) | 0.64 |
3 months | 44.3 ± 14.8 (40.3 to 47.3) | 44.9 ± 10.8 (41.5 to 49.3) | 0.54 |
12 months | 44.6 ± 17.9 (40.4 to 47.4) | 47.2 ± 10 (43.7 to 51.6) | 0.16 |
LAmin (ml/m2) | |||
Baseline | 41.2 ± 15.6 (36.2 to 46.3) | 39.8 ± 13 (34.1 to 45.5) | 0.69 |
3 months | 30.9 ± 13.1 (27.6 to 33.4) | 31.7 ± 10.8 (29 to 35.5) | 0.43 |
12 months | 31.8 ± 16.6 (26.9 to 36.7) | 33.7 ± 9.9 (28.2 to 39.2) | 0.61 |
LA P wave (ml/m2) | |||
3 months | 36.9 ± 13.1 (32.8 to 41) | 37.7 ± 9.9 (33 to 42.3) | 0.79 |
12 months | 37.1 ± 17.5 (34 to 40.9) | 39.7 ± 9.8 (35.6 to 43.3) | 0.45 |
LA reservoir (%) | |||
Baseline | 27.2 ± 14.7 (22 to 32.4) | 27.5 ± 16.1 (20.9 to 34) | 0.95 |
3 months | 48.8 ± 25.2 (40.8 to 57) | 46.9 ± 22.3 (37.7 to 56) | 0.74 |
12 months | 49.5 ± 28.9 (40.6 to 58.4) | 44.2 ± 21.2 (34.1 to 54.2) | 0.43 |
Left atrial EF (%) | |||
Baseline | 20.4 ± 9.1 (17.2 to 23.6) | 20.4 ± 9.3 (16.7 to 24.2) | 0.99 |
3 months | 31.1 ± 10.5 (27.6 to 34.6) | 30.4 ± 10.5 (26.5 to 34.4) | 0.80 |
12 months | 30.8 ± 12.2 (26.8 to 34.9) | 29.1 ± 11 (24.6 to 33.7) | 0.58 |
LA conduit (%) | |||
3 months | 16.5 ± 8 (13.7 to 19.4) | 16.3 ± 7.6 (13.2 to 19.4) | 0.91 |
12 months | 18.4 ± 12.3 (14.5 to 22.4) | 16.2 ± 10 (11.7 to 20.7) | 0.46 |
Left atrial contraction (%) | |||
3 months | 17.3 ± 11 (13.4 to 21.2) | 17 ± 9.2 (13.2 to 20.7) | 0.89 |
12 months | 15.1 ± 10.1 (11.8 to 18.2) | 15.5 ± 7.8 (12 to 19) | 0.84 |
LA RRM (%) | |||
3 months | –10.9 ± 27.6 (–20.7 to –1.1) | –6 ± 23.8 (–15.6 to 3.7) | 0.47 |
12 months | –12.4 ± 22.4 (–18.6 to –4) | 0.8 ± 32 (–10 to 6.5) | 0.09 |
In the SA group, there were also significant reductions in LAmax and LAmin at 3 months compared with baseline values (see Table 13). However, at 12 months there was an increase in LAmax, towards baseline values. Consequently, we observed less RRM at 12 months than at 3 months. Left atrial reservoir function and left atrial EF increased significantly at 3 months and showed a plateau at 12 months. Left atrial conduit function, P-wave volume and contractile function measured at 3 months remained unchanged at 12 months. LA sphericity index increased at 12 months, possibly reflecting a reduction in anteroposterior diameter.
Comparisons of left atrial volumes and function between the two treatment groups are shown in Table 13.
Measurements of LA parameters at equivalent time points appear close in values, with less RRM in the SA group at 12 months.
Comparison of left atrial volumes and volume-derived function between sinus rhythm and recurrent atrial fibrillation groups following ablation
Heart rhythm in treated patients was assessed from the continuous monitoring by ILR. SR was maintained throughout follow-up in 32 out of 59 patients (54%). All patients were in SR at the time of the TTE scans.
We observed a reduction in left atrial volumes in all patients following ablation, irrespective of SR maintenance.
There was evidence of improvement in left atrial conduit and contractile functions and an increase in LA sphericity (Table 14). Baseline values of LAmax were significantly larger in the AF group than in the SR group. In general, there was a trend towards larger LAmax and LAmin and lower reservoir function, left atrial EF and conduit function in the AF group than in the SR group during follow-up (see Table 13). Indeed, LAmin and LA P-wave volume were significantly larger in the AF group in follow-up. Contractile function and the extent of RRM appeared similar in both groups.
Parameter and time point | Group, mean ± SD (95% CI) | p-value | |
---|---|---|---|
SR (n = 32) | AF (n = 27) | ||
LA sphericity index | |||
Baseline | 0.67 ± 0.09 (0.64 to 0.70) | 0.70 ± 0.08 (0.67 to 0.74) | 0.14 |
3 months | 0.70 ± 0.09 (0.67 to 0.73) | 0.732 ± 0.740 (0.70 to 0.76) | 0.27 |
12 months | 0.75 ± 0.10 (0.72 to 0.78) | 0.74 ± 0.07 (0.70 to 0.77) | 0.41 |
LAmax (ml/m2) | |||
Baseline | 47.1 ± 15.6 (41.5 to 52.7) | 54.7 ± 13.3 (49.5 to 60) | 0.05 |
3 months | 42 ± 13.8 (40.4 to 47.5) | 47.6 ± 11.8 (41.4 to 49.1) | 0.63 |
12 months | 42.8 ± 14.5 (41.4 to 49.1) | 49.3 ± 14.7 (42.2 to 50.6) | 0.68 |
LAmin (ml/m2) | |||
Baseline | 38.1 ± 15.3 (32.6 to 43.6) | 43.5 ± 12.9 (38.4 to 48.7) | 0.15 |
3 months | 28.5 ± 12.9 (27.1 to 32.9) | 34.6 ± 10.3 (29.6 to 36) | 0.20 |
12 months | 29.4 ± 13.5 (27.8 to 34.5) | 36.5 ± 13.7 (30.8 to 38.1) | 0.18 |
LA P wave (ml/m2) | |||
3 months | 34.3 ± 12.2 (29.9 to 38.7) | 40.7 ± 10.3 (36.6 to 44.7) | 0.04 |
12 months | 34.7 ± 14.2 (33.6 to 40.9) | 42.5 ± 14 (35.5 to 43) | 0.43 |
LA reservoir (%) | |||
Baseline | 26.5 ± 12.6 (22 to 31) | 28.3 ± 18.1 (21.2 to 35.5) | 0.65 |
3 months | 53.2 ± 25 (45.7 to 61.5) | 41.9 ± 21.1 (32.8 to 50) | 0.04 |
12 months | 52.5 ± 24.9 (44.1 to 61.7) | 40.7 ± 25.7 (30.8 to 49.9) | 0.06 |
Left atrial EF (%) | |||
Baseline | 20.2 ± 7.8 (17.4 to 23) | 20.6 ± 10.6 (16.5 to 24.8) | 0.85 |
3 months | 33 ± 10.8 (29.7 to 36.5) | 28.2 ± 9.4 (24.4 to 31.8) | 0.05 |
12 months | 32.6 ± 11.8 (28.7 to 36.7) | 27.1 ± 10.9 (22.7 to 31.4) | 0.06 |
LA conduit (%) | |||
3 months | 18 ± 8.9 (14.8 to 21.2) | 14.6 ± 5.8 (12.3 to 16.8) | 0.09 |
12 months | 20.1 ± 10.8 (16.2 to 24) | 14.2 ± 11.4 (9.7 to 18.7) | 0.03 |
Left atrial contraction (%) | |||
3 months | 18.3 ± 10.1 (14.6 to 21.9) | 15.9 ± 10.3 (11.8 to 19.9) | 0.37 |
12 months | 15.8 ± 7.6 (12.4 to 18.7) | 14.6 ± 10.7 (11.4 to 18.3) | 0.77 |
LA RRM (%) | |||
3 months | –6.6 ± 30.1 (–17.5 to 4.2) | –11.2 ± 20.1 (–19.2 to –3.3) | 0.49 |
12 months | –4.2 ± 33.8 (–13.2 to 2.1) | –9.5 ± 17.9 (–16.2 to 0.6) | 0.70 |
Left atrial volumes and function as predictors of atrial fibrillation recurrence
Cox regression analysis of baseline parameters did not identify a LA structural or functional variable as a predictor of AF recurrence (Table 15), but increasing body mass index (BMI) was associated with AF recurrence following ablation. However, the analyses of parameters measured at 3 months suggest that increasing LA P-wave volume is a predictor of AF recurrence (Table 16).
Parameter | Univariable analysis | ||
---|---|---|---|
Hazard ratio | 95% CI | p-value | |
Baseline | |||
LA sphericity index | 1.03 | 0.99 to 1.08 | 0.16 |
BMI (kg/m2) | 1.08 | 1.00 to 1.17 | 0.05 |
AF duration (days) | 1.00 | 0.99 to 1.01 | 0.68 |
Age (years) | 1.03 | 0.99 to 1.07 | 0.13 |
Sex (male) | 1.37 | 0.60 to 3.14 | 0.46 |
Hypertension | 1.07 | 0.50 to 2.29 | 0.86 |
Respiratory disease | 0.38 | 0.09 to 1.61 | 0.19 |
Diabetes mellitus | 1.13 | 0.55 to 2.33 | 0.73 |
CAD | 1.47 | 0.51 to 4.25 | 0.48 |
Thyroid disorders | 1.54 | 0.84 to 2.81 | 0.16 |
Stroke/TIA | 0.69 | 0.09 to 5.07 | 0.71 |
Renal disease | 1.53 | 0.46 to 5.11 | 0.49 |
Smoker | 0.91 | 0.55 to 1.50 | 0.71 |
Alcohol use | 1.01 | 0.76 to 1.35 | 0.93 |
LAmax (ml/m2) | 1.02 | 0.99 to 1.04 | 0.13 |
LAmin (ml/m2) | 1.01 | 0.99 to 1.04 | 0.28 |
Left atrial EF (%) | 1.01 | 0.96 to 1.06 | 0.71 |
LA reservoir (%) | 1.01 | 0.98 to 1.04 | 0.50 |
Parameter | Univariable analysis | ||
---|---|---|---|
Hazard ratio | 95% CI | p-value | |
3 months | |||
BMI (kg/m2) | 1.08 | 1.00 to 1.17 | 0.05 |
Age (years) | 1.03 | 0.99 to 1.07 | 0.13 |
LA sphericity index | 1.03 | 0.98 to 1.07 | 0.24 |
LAmax (ml/m2) | 1.02 | 0.99 to 1.04 | 0.15 |
LAmin (ml/m2) | 1.02 | 1.00 to 1.05 | 0.07 |
LA P wave (ml/m2) | 1.02 | 1.00 to 1.03 | 0.01 |
Left atrial EF (%) | 0.97 | 0.93 to 1.00 | 0.06 |
LA reservoir (%) | 0.98 | 0.97 to 1.00 | 0.06 |
LA conduit (%) | 0.96 | 0.92 to 1.01 | 0.13 |
Left atrial contraction (%) | 0.98 | 0.94 to 1.02 | 0.25 |
In Cox regression analyses of the individual variables collected at the 3-month follow-up, the LA P-wave volume appeared to be a statistically significant (p = 0.01) predictor of AF recurrence (see Table 16).
Receiver operating characteristic curve analysis of the LA P wave showed an AUC of 0.73 (95% CI 0.59 to 0.86; p = 0.003). The optimal LA P-wave volume cut-off value to predict AF recurrence was 35 ml/m2, conferring a sensitivity of 70.4% and specificity of 71.9%. In Kaplan–Meier survival analysis, 74% of patients with LA P-wave volume < 35 ml/m2 (measured at 3 months) were free from AF recurrence compared with 32% of patients with LA P-wave volume > 35 ml/m2 (hazard ratio 3.32, 95% CI 1.44 to 7.64; p = 0.005) (Figure 12).
FIGURE 12.
Receiver-operating curve analysis (a) evaluating LA P-wave volume at 3 months and its association with AF recurrence; and (b) Kaplan–Meier survival analysis showing freedom from AF recurrence using a LA P-wave volume at 3-month cut-off value of 35 ml/m2.
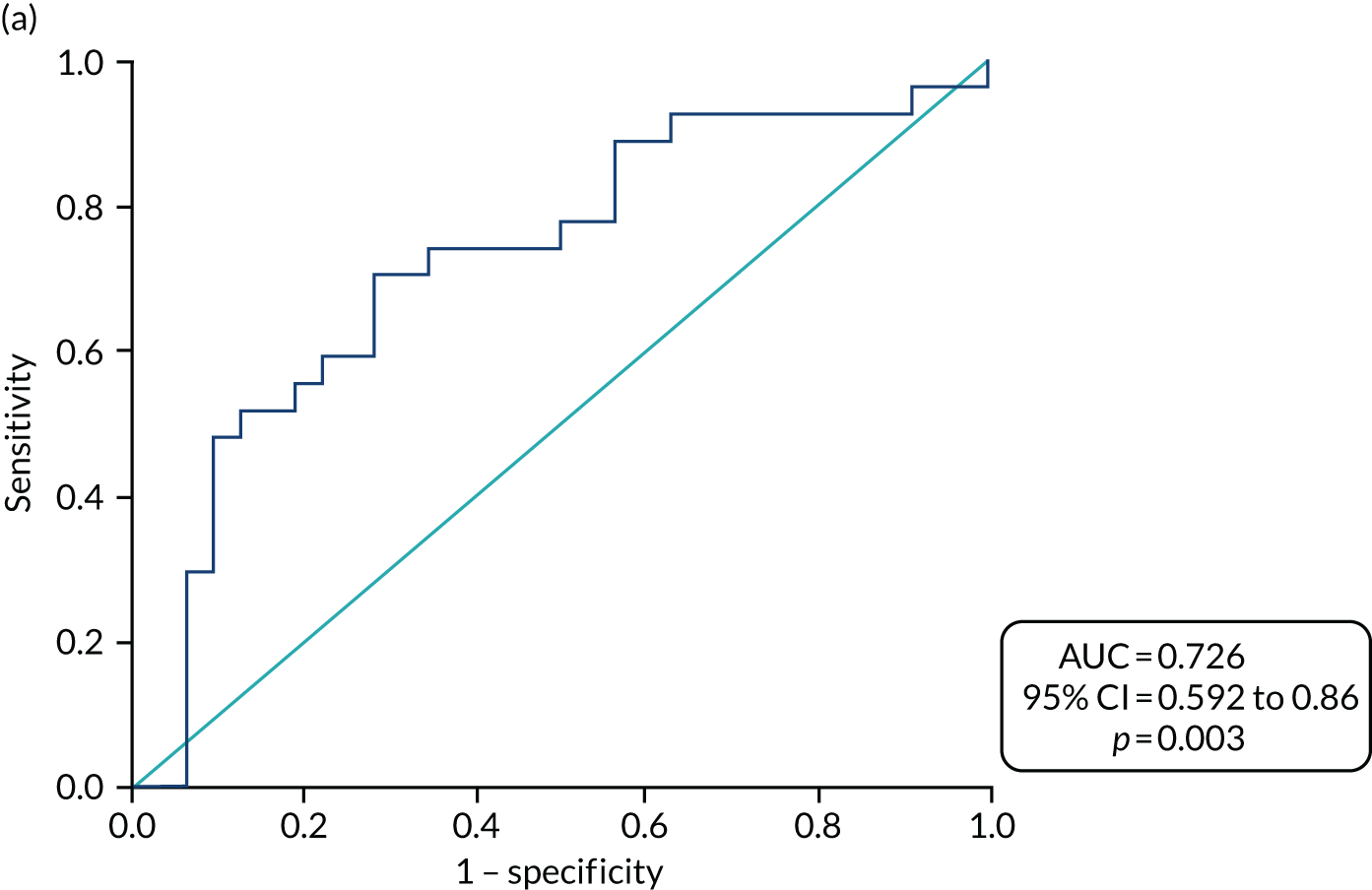

Left atrial function using two-dimensional speckle-tracking echocardiography for left atrial strain and strain rate
Of the 59 patients who had complete left atrial volumes data as above, five were excluded from the strain analysis because of inadequate tracking of left atrial myocardium throughout the cardiac cycle. The remaining 54 patients had good-quality, analysable TTE images at all three time points (i.e. baseline, and at 3 and 12 months). Four patients had cardioversion in the blanking period, but they were in SR at the time of the 3-month scan. Of the 54 patients, 29 had undergone CA as the index procedure. Baseline characteristics in both treatment arms were very similar (see Appendix 3, Table 32).
Comparisons of left atrial strain and strain rate in the two treatment arms
In the CA group, there was an increase in LA SSR and SSR′ from baseline (Table 17), indicating an increase in the magnitude and rate of LA myocardial lengthening during LA filling. LA ESR also increased in follow-up, suggesting significant LA myocardial shortening during passive emptying. However, since there was no change in ESR′, the rate of shortening did not change. LA ASR and ASR′ were similar at 3 and 12 months. An increase in the time to peak LA SSR was noted from baseline to follow-up; this may reflect a reduction in HR on return to SR.
LA parameter | Study time point | Treatment arm, mean ± SD (95% CI) | p-value | |
---|---|---|---|---|
CA (n = 29) | SA (n = 25) | |||
Left atrial strain time to peak (milliseconds) | Baseline | 347 ± 58 (331 to 373) | 350 ± 57 (332 to 385) | 0.69 |
3 months | 402 ± 50 (394 to 428) | 388 ± 42 (366 to 402) | 0.03 | |
12 months | 380 ± 60 (354 to 399) | 377 ± 59 (347 to 395) | 0.73 | |
LA SSR (%) | Baseline | 10.6 ± 4.5 (8.9 to 12.3) | 11.9 ± 3.9 (10.3 to 13.5) | 0.29 |
3 months | 20.6 ± 8 (18.4 to 23.6) | 18.5 ± 6.6 (15.3 to 20.8) | 0.13 | |
12 months | 20.3 ± 7.4 (18.1 to 22.9) | 16.5 ± 4.9 (13.7 to 18.8) | 0.02 | |
LA ESR (%) | Baseline | 5.7 ± 2.9 (4.6 to 6.8) | 6 ± 2.1 (5.1 to 6.8) | 0.7 |
3 months | 13.8 ± 5.8 (12.2 to 15.7) | 11 ± 4.7 (9 to 12.8 | 0.02 | |
12 months | 12.5 ± 5.6 (10.8 to 14.4) | 9.5 ± 3.9 (7.5 to 11.4) | 0.02 | |
SSR′ (1/second) | Baseline | 0.5 ± 0.2 (0.42 to 0.58) | 0.59 ± 0.2 (0.5 to 0.67) | 0.13 |
3 months | 0.83 ± 0.29 (0.76 to 0.95) | 0.78 ± 0.28 (0.66 to 0.86) | 0.16 | |
12 months | 0.8 ± 0.31 (0.70 to 0.92) | 0.65 ± 0.27 (0.51 to 0.75) | 0.03 | |
ESR′ (1/second) | Baseline | 0.82 ± 0.48 (0.64 to 1) | 0.82 ± 0.38 (0.67 to 0.98) | 0.99 |
3 months | 0.96 ± 0.58 (0.78 to 1.13) | 0.78 ± 0.34 (0.59 to 0.97) | 0.16 | |
12 months | 0.75 ± 0.38 (0.3 to 1.2) | 0.94 ± 1.8 (0.45 to 1.43) | 0.58 | |
LA ASR (%) | 3 months | 6.8 ± 3.3 (5.5 to 8.1) | 7.5 ± 3 (6.2 to 8.7) | 0.43 |
12 months | 7.8 ± 3.5 (6.8 to 9) | 7 ± 2.6 (5.7 to 8.1) | 0.22 | |
ASR′ (1/second) | 3 months | 0.64 ± 0.33 (0.51 to 0.76) | 0.64 ± 0.26 (0.53 to 0.75) | 0.95 |
12 months | 0.61 ± 0.39 (0.51 to 0.72) | 0.51 ± 0.27 (0.4 to 0.62) | 0.18 |
Similar observations were made in the SA group, apart from the ASR′, which was lower at 12 months than at 3 months. Between-group comparisons showed significantly higher values of LA SSR in the CA arm than in the SA arm (mean ± SD 20.3 ± 7.4% vs. 16.5 ± 4.9%; p = 0.03) at 12 months’ follow-up.
The LA ESR values were also significantly higher in the CA arm than in the SA arm at 3 months’ follow-up (mean ± SD 13.8 ± 5.8 vs. 11 ± 4.7, respectively) and at 12 months’ follow-up (mean ± SD 12.5 ± 5.6 vs. 9.5 ± 3.9, respectively) (p = 0.02). All other LA parameters measured at equivalent time points were similar, as shown in Table 17.
Comparison of left atrial strain and strain rate parameters between sinus rhythm and recurrent atrial fibrillation groups following ablation
Following ablation, 30 out of 54 (56%) patients remained in SR throughout follow-up, with similar proportions in each of the treatment arms.
The LA SSR, SSR′ and LA ESR were significantly higher at 3 months than at baseline in all patients after ablation, regardless of their AF recurrence status. ESR′ did not appear to change from baseline (Table 18).
Left atrial strain/strain rate parameter | Study time point | Group, mean ± SD (95% CI) | p-value | |
---|---|---|---|---|
AF (n = 24) | SR (n = 30) | |||
Left atrial strain time to peak (milliseconds) | Baseline | 358 ± 60 (332 to 384) | 353 ± 57 (331 to 374) | 0.76 |
3 months | 408 ± 50 (389 to 427) | 392 ± 41 (374 to 408) | 0.18 | |
12 months | 372 ± 60 (346 to 395) | 378 ± 56 (354 to 398) | 0.72 | |
LA SSR (%) | Baseline | 9.80 ± 3.70 (8.20 to 11.4) | 12.3 ± 4.3 (10.7 to 13.9) | 0.03 |
3 months | 16.40 ± 4.90 (14.20 to 19.90) | 22.2 ± 8.1 (19.2 to 24.2) | 0.02 | |
12 months | 17.02 ± 6.02 (14.80 to 20.30) | 19.6 ± 6.9 (16.9 to 21.8) | 0.34 | |
LA ESR (%) | Baseline | 5.10 ± 2.20 (4.10 to 6.00) | 6.40 ± 2.60 (5.50 to 7.40) | 0.05 |
3 months | 11.00 ± 4.40 (9.50 to 13.70) | 13.80 ± 5.90 (11.5 to 15.20) | 0.22 | |
12 months | 10.50 ± 0.05 (8.70 to 12.90) | 11.60 ± 5.20 (9.50 to 13.20) | 0.71 | |
SSR′ (1/second) | Baseline | 0.48 ± 0.14 (0.42 to 0.54) | 0.59 ± 0.24 (0.50 to 0.68) | 0.06 |
3 months | 0.73 ± 0.23 (0.65 to 0.85) | 0.88 ± 0.28 (0.77 to 0.95) | 0.13 | |
12 months | 0.65 ± 0.22 (0.54 to 0.78) | 0.79 ± 0.34 (0.67 to 0.89) | 0.16 | |
ESR′ (1/second) | Baseline | 0.73 ± 0.32 (0.59 to 0.87) | 0.90 ± 0.49 (0.71 to 1.08) | 0.16 |
3 months | 0.84 ± 0.60 (0.68 to 1.07) | 0.90 ± 0.39 (0.70 to 1.05) | 0.99 | |
12 months | 0.57 ± 0.25 (0.16 to 1.16) | 1.10 ± 1.70 (0.53 to 1.43) | 0.34 | |
LA ASR (%) | 3 months | 5.50 ± 2.10 (4.60 to 6.30) | 8.40 ± 3.30 (7.20 to 9.60) | 0.0003 |
12 months | 6.70 ± 2.20 (5.90 to 8.60) | 8.00 ± 3.70 (6.40 to 8.80) | 0.71 | |
ASR′ (1/second) | 3 months | 0.49 ± 0.23 (0.40 to 0.59) | 0.75 ± 0.30 (0.64 to 0.86) | 0.001 |
12 months | 0.44 ± 0.19 (0.40 to 0.64) | 0.67 ± 4.00 (0.49 to 0.71) | 0.39 |
Between-group comparisons showed that values of LA SSR and LA ESR at baseline were significantly higher in patients who maintained SR in follow-up than in those with AF recurrence. LA SSR, LA ASR and ASR′ values measured at 3 months’ follow-up were also significantly higher in patients who maintained SR during follow-up, as shown in Table 18.
Left atrial strain and strain rate as predictors of atrial fibrillation recurrence
The results of the univariable Cox regression analysis of the baseline demographic data and LA parameters measured at 3 months are shown in Table 19. Only LA SSR and SSR′ measured at baseline appear to have a negative association with AF recurrence (p = 0.05 and p = 0.03, respectively). Using data from 3 months’ follow-up, LA SSR, LA ASR and ASR′ seem to have a significant negative association with AF recurrence (p = 0.008, p = 0.001 and p = 0.002, respectively). As strain and strain rate measurements are collinear, strain measurements from the 3-month follow-up visit were used for ROC analysis and Kaplan–Meier survival plots.
Parameter | Univariable analysis | ||
---|---|---|---|
Hazard ratio | 95% CI | p-value | |
Baseline | |||
BMI (kg/m2) | 1.08 | 1.00 to 1.17 | 0.05 |
AF duration (days) | 1.00 | 1.00 to 1.00 | 0.78 |
Age (years) | 1.03 | 0.98 to 1.07 | 0.25 |
Sex (male) | 1.31 | 0.52 to 3.31 | 0.57 |
Hypertension | 1.32 | 0.58 to 2.98 | 0.51 |
Respiratory disease | 0.39 | 0.09 to 1.66 | 0.20 |
Diabetes mellitus | 1.15 | 0.56 to 2.38 | 0.70 |
CAD | 2.21 | 0.75 to 6.51 | 0.15 |
Thyroid disorders | 1.59 | 0.87 to 2.93 | 0.13 |
CVD | 0.64 | 0.09 to 4.72 | 0.66 |
Renal disease | 1.45 | 0.43 to 4.88 | 0.55 |
Smoker | 0.88 | 0.43 to 1.79 | 0.71 |
Alcohol use | 0.96 | 0.70 to 1.31 | 0.79 |
LA SSR (%) | 0.88 | 0.78 to 0.99 | 0.05 |
SSR′ (1/second) | 0.09 | 0.01 to 0.82 | 0.03 |
LA ESR (%) | 0.82 | 0.67 to 1.01 | 0.06 |
ESR′ (1/second) | 0.44 | 0.14 to 1.40 | 0.16 |
3 months | |||
LA SSR (%) | 0.90 | 0.84 to 0.97 | 0.008 |
SSR′ (1/second) | 0.17 | 0.03 to 1.01 | 0.051 |
LA ESR (%) | 0.92 | 0.84 to 1.01 | 0.09 |
ESR′ (1/second) | 0.77 | 0.30 to 1.96 | 0.59 |
LA ASR (%) | 0.77 | 0.66 to 0.91 | 0.001 |
ASR′ (1/second) | 0.06 | 0.01 to 0.36 | 0.002 |
The ROC curve analysis revealed 18.7% to be the optimal cut-off value for SSR at 3 months (sensitivity = 75% and specificity = 63%). A LA SSR at 3 months of > 18.7% was associated with 76% freedom from atrial arrhythmias, compared with 37.9% for SSR of < 18.7% (hazard ratio 3.18, 95% CI 1.25 to 8.05; p = 0.015) (Figure 13).
FIGURE 13.
(a) The ROC curve analysis of LA reservoir (LA SSR) at 3 months following ablation, showing that it is a strong predictor of SR maintenance; and (b) Kaplan–Meier survival curves illustrating freedom from AF recurrence at LA SSR values of less than or greater than 18.7%. Diagonal segments are produced by ties.

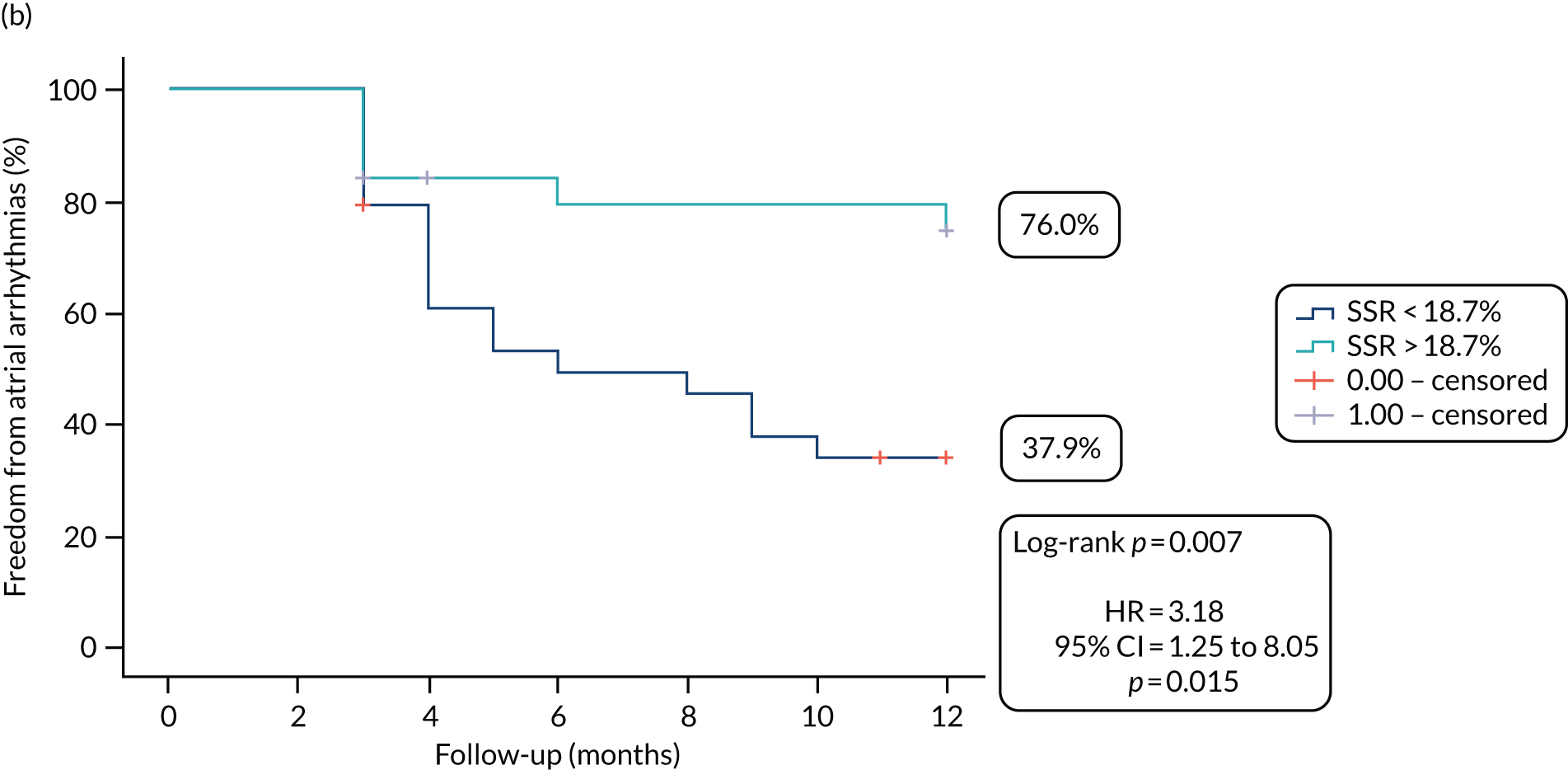
The ROC curve analysis revealed 7.5% to be the optimal cut-off value for ASR at 3 months (sensitivity = 88% and specificity = 60%). A LA ASR > 7.5% was associated with 86% freedom from atrial arrhythmias compared with 36% for ASR < 7.5% (hazard ratio 5.94, 95% CI 1.76 to 20.1; p = 0.004) (Figure 14).
FIGURE 14.
(a) The ROC curve analysis of left atrial contraction strain (LA ASR) at 3 months following ablation, showing that it is a strong predictor of SR maintenance; and (b) Kaplan–Meier survival curves illustrating freedom from AF recurrence at LA ASR values of less than or greater than 7.5%.


Tissue Doppler imaging data
A total of 52 patients (45%) had technically adequate TTE scans for analysis of TDI parameters in the CASA-AF trial cohort. Of these, 33 were common to the strain analysis and 19 were a different subset of patients. Among the 52 patients, 50% underwent CA. Baseline demographic characteristics and clinical data were similar in both groups (see Appendix 3, Table 33).
Comparisons of the left atrial tissue Doppler imaging parameters in the catheter ablation and surgical ablation groups
In the CA group, S′ and SSR were higher during follow-up than at baseline, whereas ESR was lower. There was a significant increase in ASR from 3 to 12 months, but no change in TACT or LV septal late atrial myocardial diastolic velocity (A′) during this time period.
In the SA group, S′ was lower during follow-up than at baseline. There was no significant change in SSR, but there was a reduction in ESR. ASR and TACT did not change from 3 months to 12 months. Finally, LV septal A′ at 12 months was lower than at 3 months.
As shown in Table 20, we found no evidence of a difference between treatment groups in left atrial TDI parameters at the three time points.
Study time point | Parameter | Treatment arm, mean ± SD (95% CI) | p-value | |
---|---|---|---|---|
CA (n = 26) | SA (n = 26) | |||
Global baseline | S′ (cm/second) | 7.2 ± 2.1 (6.3 to 8.1) | 8.1 ± 2 (7.3 to 8.9) | 0.13 |
SSR (cm/second) | 6.2 ± 1.3 (5.6 to 6.7) | 6.5 ± 1.3 (6 to 7) | 0.36 | |
ESR (cm/second) | 8.9 ± 2.2 (8 to 9.8) | 10.1 ± 2.9 (8.9 to 11.3) | 0.1 | |
Global month 3 | S′ (cm/second) | 6.7 ± 1.8 (6.3 to 7.6) | 6.6 ± 1.8 (5.8 to 7.1) | 0.32 |
SSR (cm/second) | 6.6 ± 1.8 (6.1 to 7.2) | 6.6 ± 1.3 (6 to 7.1) | 0.9 | |
ESR (cm/second) | 7.7 ± 1.2 (7.2 to 8.4) | 8.1 ± 2 (7.2 to 8.4) | 0.95 | |
ASR (cm/second) | 6.2 ± 1.9 (5.4 to 7) | 6.1 ± 1.6 (5.4 to 6.7) | 0.73 | |
TACT (milliseconds) | 156.2 ± 27.9 (138 to 174) | 168 ± 34 (151 to 185) | 0.33 | |
LV septal A′ (cm/second) | 6 ± 2 (5 to 7.1) | 7 ± 1.9 (6.1 to 8) | 0.14 | |
Global month 12 | S′ (cm/second) | 7.7 ± 2.2 (7.1 to 8.7) | 7.2 ± 2 (6.2 to 7.8) | 0.14 |
SSR (cm/second) | 7.3 ± 1.4 (6.7 to 8) | 7.2 ± 1.7 (6.5 to 7.8) | 0.67 | |
ESR (cm/second) | 7.8 ± 1.3 (7.2 to 8.5) | 8.1 ± 1.9 (7.4 to 8.6) | 0.8 | |
ASR (cm/second) | 6.8 ± 1.9 (6.1 to 7.6) | 5.9 ± 1.6 (5.2 to 6.6) | 0.06 | |
TACT (milliseconds) | 159.5 ± 29.4 (141.8 to 177.3) | 171.6 ± 22.2 (160.2 to 183.1) | 0.21 | |
LV septal A′ (cm/second) | 6.5 ± 1.5 (5.7 to 7.2) | 6.1 ± 1.9 (5.2 to 7.1) | 0.61 |
Longitudinal effects of catheter ablation and surgical ablation on regional left atrial wall motion and function
Individual ablation strategies may have different effects on regional left atrial wall motion and function that can evolve over time, so we compared changes in global and regional left atrial functions at baseline, and at 3 and 12 months post ablation.
The regional functions of the LA at baseline, as well as the function of the septal and inferior LA regions at 3 and 12 months, appear similar in CA and SA (see Appendix 3, Table 34). Small differences were observed in conduit and booster functions between the treatment arms in lateral and anterior left atrial walls at 12 months’ follow-up, but these did not translate into a change in the global left atrial function between the two groups (see Table 20). The reduced contractile function at the anterior and lateral wall in the SA group at 12 months might be a medium- to long-term effect of mechanical LAA exclusion using an epicardial compression device.
There was a noticeable increase in regional expansion following CA in most regions except in the lateral wall, with non-significant changes in this region after SA at 12 months’ follow-up.
Global conduit function was significantly reduced from baseline to 3 months and from baseline to 12 months in both the CA group and the SA group (more inferior and lateral for the CA group; more anterior and inferior for the SA group). More of the left atrial walls showed improved reservoir function in the CA group (inferior, septal, anterior) compared with none in the SA group.
Comparison of left atrial tissue Doppler imaging parameters in the sinus rhythm and atrial fibrillation recurrence groups
Of the 52 patients considered in these analyses, 17 remained in SR (33%) and 35 developed recurrent AF over the 12 months’ follow-up.
In the SR group, there were increases in SSR from baseline to 3 months and from 3 to 12 months. All other parameters remained unchanged (Table 21).
Study time point | Left atrial wall | Parameter | Group, mean ± SD (95% CI) | p-value | |
---|---|---|---|---|---|
AF (n = 35) | SR (n = 17) | ||||
Baseline | Global | S′ (cm/second) | 8 ± 2.2 (7.3 to 8.8) | 6.8 ± 1.3 (6.1 to 7.5) | 0.04 |
SSR (cm/second) | 6.4 ± 1.3 (5.9 to 6.9) | 6.2 ± 1.1 (5.6 to 6.8) | 0.66 | ||
ESR (cm/second) | 9.6 ± 2.7 (8.6 to 10.5) | 9.3 ± 2.6 (7.9 to 10.7) | 0.73 | ||
3 months | Global | S′ (cm/second) | 6.7 ± 1.9 (6 to 7.2) | 6.5 ± 1.5 (6 to 7.7) | 0.67 |
SSR (cm/second) | 6.4 ± 1.4 (5.9 to 6.8) | 7.1 ± 1.1 (6.4 to 7.8) | 0.08 | ||
ESR (cm/second) | 7.8 ± 1.8 (7.1 to 8.1) | 8 ± 1.2 (7.4 to 8.9) | 0.27 | ||
ASR (cm/second) | 5.5 ± 1.5 (5.0–6.1) | 7.4 ± 1.5 (6.6 to 8.2) | 0.00022 | ||
TACT (milliseconds) | 169.9 ± 32.7 (133.4 to 170.5) | 151.9 ± 27.6 (153.7 to 186.2) | 0.14 | ||
LV septal A′ (cm/second) | 5.9 ± 1.9 (5 to 6.7) | 7.8 ± 1.5 (6.8 to 8.7) | 0.006 | ||
12 months | Global | S′ (cm/second) | 7.5 ± 2.2 (6.6 to 8.1) | 7.3 ± 2.1 (6.6 to 8.7) | 0.60 |
SSR (cm/second) | 7 ± 1.6 (6.4 to 7.4) | 7.8 ± 1.2 (7.1 to 8.6) | 0.05 | ||
ESR (cm/second) | 7.9 ± 1.7 (7.3 to 8.4) | 8 ± 1.4 (7.3 to 8.9) | 0.59 | ||
ASR (cm/second) | 5.9 ± 1.5 (5.3 to 6.4) | 7.4 ± 1.9 (6.4 to 8.4) | 0.004 | ||
TACT (milliseconds) | 168.8 ± 29.3 (154.3 to 186.2) | 162 ± 20.3 (149.9 to 175.6) | 0.54 | ||
LV septal A′ (cm/second) | 5.6 ± 1.6 (4.9 to 6.3) | 7.4 ± 1.3 (6.7 to 8.2) | 0.001 |
In the AF recurrence group, ESR was reduced during follow-up. There were no significant differences in ASR, TACT and LV septal A′ from 3 to 12 months (see Table 21).
Comparison of SR and AF groups showed lower S′ in the SR group at baseline only (see Table 21). There was a gradual increase in SSR values during follow-up, which became significant at 12 months in the SR group. There were reductions in ESR from baseline to 12 months in both groups. Most notably, ASR and LV septal A′ were significantly higher at 3 months in the SR group than in the AF group, and these differences were maintained at 12 months. TACT was similar at all time points in both groups.
Booster function at the lateral and anterior left atrial walls appears significantly altered in follow-up for patients maintaining SR when compared with those with AF recurrence (see Appendix 3, Table 35).
Left atrial tissue Doppler imaging parameters as predictors of atrial fibrillation recurrence
As shown in Table 22, we did not identify any predictors of AF recurrence among the baseline parameters, but ASR measured at 3 months and LV septal A′ were negative-associated predictors of AF recurrence. LV septal A′ has collinearity with ASR, hence it was not used to calculate a cut-off value for use in Kaplan–Meier curves.
Parameter | Univariable analysis | ||
---|---|---|---|
Hazard ratio | 95% CI | p-value | |
Baseline | |||
BMI (kg/m2) | 0.98 | 0.91 to 1.06 | 0.67 |
AF duration (days) | 1.00 | 0.99 to 1.00 | 0.43 |
Age (years) | 1.02 | 0.98 to 1.07 | 0.25 |
Male | 1.20 | 0.56 to 2.56 | 0.64 |
Hypertension | 0.81 | 0.42 to 1.58 | 0.54 |
Respiratory disease | 0.66 | 0.23 to 1.88 | 0.44 |
Diabetes mellitus | 1.07 | 0.59 to 1.94 | 0.82 |
CAD | 2.30 | 1.03 to 5.16 | 0.04 |
Thyroid disorders | 1.22 | 0.68 to 2.21 | 0.51 |
CVD | 0.66 | 0.90 to 4.82 | 0.68 |
Renal disease | 0.57 | 0.17 to 1.92 | 0.36 |
Smoker | 1.06 | 0.72 to 1.56 | 0.77 |
Alcohol use | 1.19 | 0.89 to 1.60 | 0.23 |
S′ (cm/second) | 1.16 | 0.99 to 1.35 | 0.07 |
Reservoir (cm/second) | 1.16 | 0.88 to 1.52 | 0.29 |
Conduit (cm/second) | 1.01 | 0.88 to 1.15 | 0.92 |
3 months | |||
S′ (cm/second) | 1.03 | 0.86 to 1.22 | 0.77 |
Reservoir (cm/second) | 0.83 | 0.64 to 1.08 | 0.17 |
Conduit (cm/second) | 0.96 | 0.77 to 1.18 | 0.67 |
Contraction (cm/second) | 0.64 | 0.51 to 0.81 | 0.0002 |
LV septal A′ (cm/second) | 0.67 | 0.52 to 0.87 | 0.003 |
TACT (milliseconds) | 1.01 | 0.99 to 1.02 | 0.22 |
As illustrated in Figure 15, ROC curve analysis showed ASR at 3 months to have an AUC of 0.797 (p < 0.001) for the prediction of AF recurrence. At the optimal cut-off value of 6 cm/second, ASR was associated with 66% sensitivity and 81% specificity for the prediction of AF recurrence. Patients with ASR > 6 cm/second were more likely to remain in SR during follow-up than those with ASR < 6 cm/second (54% vs. 13%).
FIGURE 15.
(a) The ROC curve analysis of TDI ASR at 3 months with sensitivity and specificity for detecting AF recurrence; and (b) Kaplan–Meier survival analysis of TDI ASR.
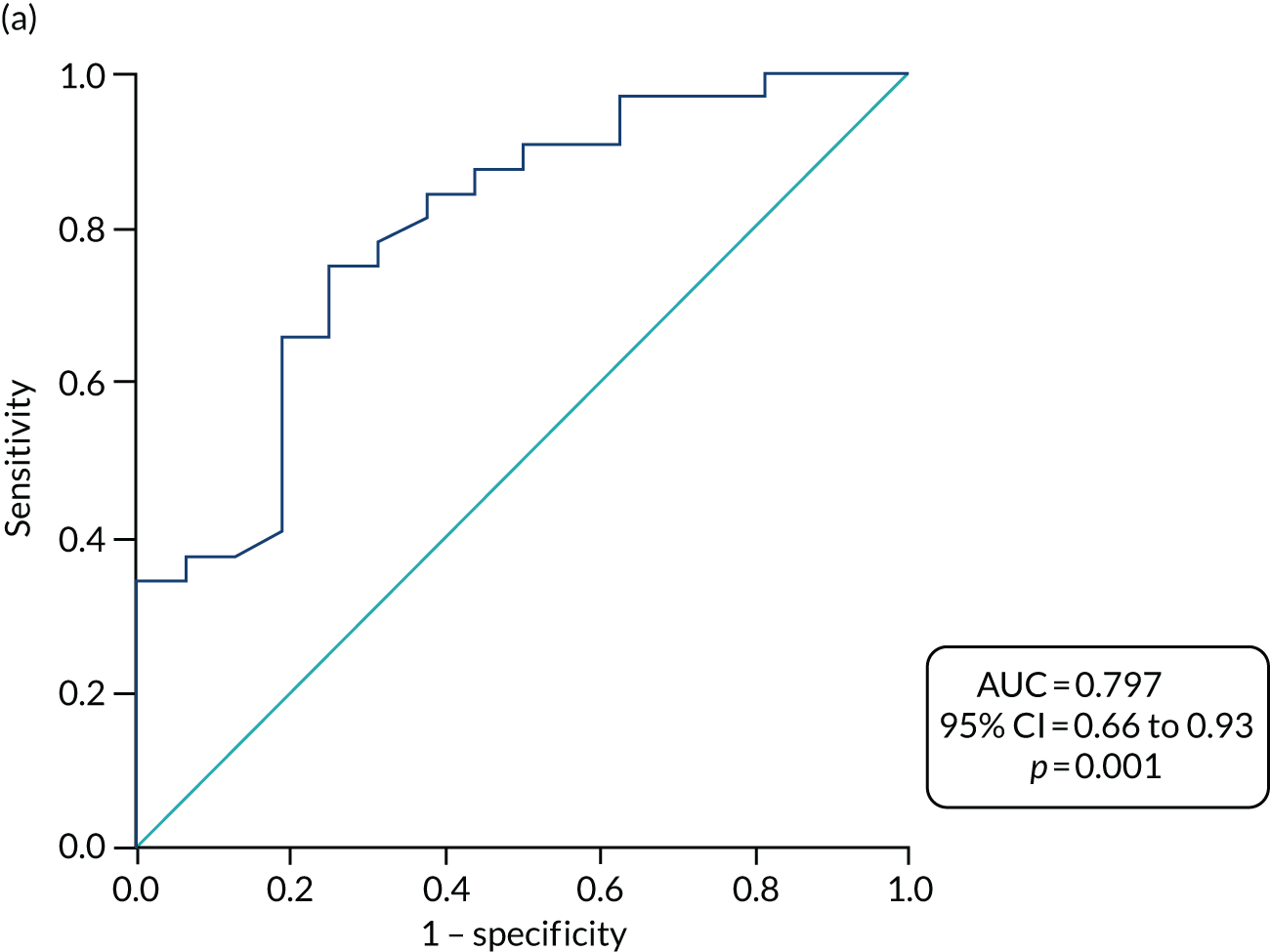

Discussion
Impairment of left atrial mechanical function may provide indirect information about the presence of fibrosis before or after ablation. It can be comprehensively assessed by multiple echocardiographic modalities, and this was undertaken in a number of earlier studies focusing on patients with paroxysmal AF and persistent AF. To our knowledge, this is the first randomised control study with a comprehensive assessment of left atrial function after CA and SA in patients with LSPAF.
The main findings of this substudy of the CASA-AF randomised trial are as follows:
-
A significant reduction in the left atrial volume and improvement in left atrial EF, left atrial reservoir function, TDI and speckle-tracking strain and strain rate were observed in both treatment arms during follow-up. A greater reduction of the LA maximum volume was observed in the CA group than in the SA group. There was no difference between the two groups in the left atrial volumes (maximum, minimum and P-wave volume) and left atrial TDI-based function assessments. However, strain reservoir and conduit functions were higher in the CA group at 12 months.
-
Left atrial volume was significantly reduced in patients who maintained SR after ablation during the 12 months’ follow-up, but volumetric assessment of left atrial function did not show significant difference between patients who maintained SR in follow-up and those who experienced AF recurrence. However, left atrial function assessed by TDI and speckle-tracking strain and strain rate was significantly better in patients who maintained SR.
-
The only predictors of AF recurrence in this study were left atrial volume at P wave, left atrial reservoir and contraction functions measured at 3 months’ follow-up.
-
LAA mechanical exclusion as part of SA could underlie the reduced left atrial contraction at the anterior and lateral walls in follow-up. Ablation of the mitral isthmus line as part of CA could be the reason for reduced left atrial reservoir function at the lateral wall in follow-up.
Ablation and left atrial volumes
In both treatment arms, the ablation was followed by a reduction in left atrial volumes and an increase in left atrial EF. We observed slightly less RRM in the SA group. There was also improvement in reservoir function as assessed by the volumes and strain data. Volumetrically assessed conduit function was similar in both groups after ablation, but conduit strain (LA ESR) was better in the CA group. This may indicate improved recoil and long-axis function during passive emptying in the CA group, but, for uncertain reasons, this may not translate to an increased volume of emptying. Notably, there was no improvement in conduit strain rate following ablation with either technique, suggesting that the degree of myocardial shortening and was the more important factor, not the rate of shortening. In the subgroup of patients who underwent TDI, conduit function based on myocardial velocities was reduced in both groups. This might reflect either a lesser contribution of conduit function from the left atrial walls just below the mitral annulus sampled by pulsed-wave TDI, or systematically poor sampling of the left atrial walls during the motion caused by passive emptying. LA speckle-tracking strain measurements tend to be more robust than pulsed-wave TDI as they take into account long-axis function from all walls and are not angle dependent or confounded by motion artefact. In both treatment groups, contractile function derived from left atrial volumes and strain/strain rate assessment was similar after ablation.
In summary, the impact of both ablation strategies was similar with respect to reducing left atrial volumes and improving reservoir function, with similar effects on contractile function. Conduit function by strain assessment was better in the CA group.
Atrial fibrillation recurrence and left atrial function
Analysis of the study population based on heart rhythm response following ablation showed that baseline LAmax was significantly larger in the AF recurrence group than in those who remained in SR throughout follow-up. Although the percentage reduction in LAmax and LAmin after ablation were similar in both groups, LAmin and LA P-wave volume were significantly larger in the AF group in follow-up. There were improvements in volume-derived reservoir function in both groups after ablation, but this was lower in the AF group than in the SR group. Strain and strain rate assessments showed improvement in reservoir function after ablation in both groups, but significantly smaller values in the AF group at baseline and 3 months. TDI also showed lower reservoir function in the AF group than in the SR group at 12 months. There were also subtle indications of better conduit function in the SR than in the AF recurrence group, with higher baseline conduit strain, higher conduit strain rate at 3 months and trends towards better volume-derived conduit function at 3 and 12 months. Although contractile function derived from left atrial volumes was similar in both groups, contractile strain and strain rate were clearly higher in the SR group following ablation. The TDI data also showed better left atrial contractile function in the SR group during follow-up.
Predictors of atrial fibrillation recurrence
It is important to acknowledge that the measurement of LAmax is a part of the minimum data set for performing transthoracic echocardiography in routine clinical practice, whereas assessment of left atrial strain remains a research tool. Baseline LAmax was found to be an independent predictor of AF recurrence in individual studies and in a recent meta-analysis, but we were not able to confirm this finding in our small data set. 100,141 Analysis of the data from the 3 months’ follow-up during SR showed that the LA P-wave volume (the left atrial volume just prior to active atrial contraction) was a predictor of AF recurrence with optimal cut-off value of 35 ml/m2. The optimal left atrial contractile strain (ASR) from 3 months’ follow-up for the prediction of AF recurrence was 7.5%. Left atrial contractile strain at 3 months had the highest AUC. Reservoir strain (LA SSR) measured 3 months after ablation was also associated with maintenance of SR, with an optimal cut-off value of 18.7%. These results are broadly in line with Moreno-Ruiz et al. ,97 who suggested global left atrial reservoir strain of < 10.75% as a predictor for AF recurrence following DCCV of patients with LSPAF.
A number of studies have assessed LA sphericity by computerised tomography (CT) and CMRI as predictors of AF recurrence with variable results. 142,143 A small study showed LA sphericity index by echocardiography to predict AF recurrence following DCCV. 144 Our findings did not show any predictive value of LA sphericity index for AF recurrence, in keeping with a recent study by Mulder et al. 145 that showed LA sphericity to be related to restraints in the thoracic cavity rather than AF remodelling.
The assessment of pulsed-wave myocardial tissue velocities by TDI has inherent limitations related to angle dependency and motion artefact. In addition, a global assessment of left atrial function using this technique is time-consuming and prone to variability, as multiple sites have to be sampled to derive an average value. Importantly, in our study, the subgroup of patients who underwent TDI were not the same as those who had the complete 2D volumes and strain data. Despite these limitations, it was interesting to note that left atrial contractile function represented by the S′ at 3 months was a strong predictor of AF recurrence. This finding was entirely consistent with the left atrial contractile strain data and the AUCs were similar. Longer duration of TACT derived from TDI has been shown to be related to the recurrence of AF following CA and cardiac surgery. The longer TACT may represent slow conduction in the tissue due to scar or large left atrial size,123,139,146–148 and local atrial wall segment conduction times have been used as a marker of AF recurrence following cardioversion. 149 In our study, there was no difference in TACT between CA and SA, likely because of similar lesion sets and similar changes in left atrial volumes and function between the two ablation techniques. Moreover, TACT did not predict AF recurrence following ablation.
Left atrial volumes increase with longer durations of AF and can be measured by TTE, CT or CMRI. 150 The left atrial volumes measured by TTE in our study were nearly twofold larger than those in a healthy population of a similar age. 151 Pre-procedural left atrial size is an important predictor of successful ablation, but we were not able to demonstrate this with our current analyses. 100,141,152,153 Our study also shows that atrial compliance, the ability of the LA to expand during the filling phase, was the more dominant factor in predicting heart rhythm response following ablation. Increased LA myocardial stiffness or rigidity may indicate greater fibrotic change and increased likelihood of AF recurrence. Passive recoil of the LA during the early emptying phase did not influence AF recurrence, perhaps because the pressure difference between the LA and LV during the early phase of emptying was the dominant factor in LA emptying without the need for a significant elastic recoil effect to be important. Notably, with the return of SR and mechanical atrial contraction following ablation, the size of the LA just prior to atrial contraction at 3 months was an important predictor of AF – the smaller the size, the lower the likelihood of AF recurrence. However, the extent of atrial contraction as measured by longitudinal myocardial shortening was the best predictor of AF recurrence as judged by the large AUC in ROC curve analysis. This finding is supported by the predictive value of left atrial TDI A-wave velocity, which had similarly large AUCs of 0.79 for the prediction of AF recurrence.
As the duration of AF increases, there tends to be more adverse LA remodelling, resulting in more scar formation, and greater LA stunning despite restoration of SR following ablation. 102,154,155 Using echocardiography, this LA substrate remodelling may be assessed most effectively by measuring strain and less so by evaluating myocardial tissue velocities using TDI156,157 or volume-derived measurements of left atrial function. Left atrial function as assessed by volumes and strain analysis in our study population was markedly reduced at baseline and improved following ablation. Despite these improvements with restoration of SR, 44% of the patients developed recurrences of AF in this substudy of the CASA-AF trial. The proportion of patients with recurrence of AF is smaller in comparison to the overall trial results, but this is most likely to be because of the exclusion of patients who were not in SR at the time of the scan.
The AF recurrence group had significant reductions in left atrial volumes and improvement in reservoir function from baseline to 3 months, which could be attributed to being predominantly in SR. However, the group with maintained SR throughout follow-up had better reservoir and contractile function assessed by strain and strain rate at 3 months than the AF recurrence group. Strain rate analysis also showed a significant improvement in left atrial conduit function at 3 months in the SR group. This is very important in understanding the possible reasons for AF recurrence, one of which could be LA fibrosis leading to a stiffer LA and a more advanced pathological substrate affecting atrial expansion, recoil and contractility. It is also important to note that LA strain values, although improved after ablation, remained substantially smaller than levels observed in a healthy population. 156 Pathan et al. 131 conducted a meta-analysis of strain values in 2542 healthy individuals from 40 studies and found that the mean left atrial reservoir strain was 39.4%, Left atrial conduit strain was 23% and left atrial contractile strain was 17.4%. In our study population of LSPAF, left atrial reservoir strain, conduit strain and contractile strain improved following ablation to only 18%, 11% and 7.5%, respectively.
As discussed earlier, left atrial function is determined by many factors, including duration of AF and associated cardiovascular risk factors. 86,158–160 The two rhythm groups in our study were well matched in this regard, with no significant differences in cardiovascular risk factors.
Atrial fibrillation ablation strategies have evolved over the last few decades, with more lesions sets added using a stepwise Bordeaux approach. 161 The impact of linear lesions and epicardial approaches on left atrial anatomy and function have not been extensively studied. Thomas et al. 162 assessed left atrial function in patients who had AF ablation (CA or SA) or cardioversion and compared their findings with measurements in healthy control patients. Patients who had linear lesions during ablation and patients who were cardioverted had larger left atrial size than the healthy control patients. Left atrial function was also reduced in patients with linear lesions compared with those who underwent cardioversion. 96 A small study by De Maat et al. 163 showed that thoracoscopic surgical occlusion of the LAA by a clip did not seem to have a detrimental effect on left atrial contractile function. Similarly, in our study, all SA patients underwent LAA exclusion using AtriClip without any detectable impact on global left atrial function despite a regional reduction in contraction in the anterolateral left atrial walls compared with the CA group. Similarly, all patients with mitral isthmus lesion had a reduction in lateral left atrial wall reservoir function without impact on global left atrial function compared with the SA group.
Limitations
The main limitation of this study is the reduced sample size, which was determined by the availability of good-quality TTE images for all relevant time points. A further limitation was the additional requirement that patients had to be in SR at the time of the scan to enable measurements of all components of left atrial function at different time points.
This had an impact on the proportion of patients in the SR/AF recurrence groups, which was different from the proportion for the primary outcome in the trial. However, the baseline characteristics of the patients within these subgroups were not different from the rest of the trial sample.
Good-quality images were particularly difficult to obtain for left atrial strain and tissue Doppler measurements because of breathing affecting the movement of the LA myocardial edge. The sonographers were trained during the early phase of trial recruitment, so some suboptimal quality scans in this period had to be excluded from the analysis.
Although left atrial volumes derived from 2D echocardiography tend to be systematically smaller than CMRI measurements, there is a high correlation between the two techniques,164 so the use of echo-derived measurements for serial assessment is justified, particularly as echocardiography is easily accessible, less time-consuming and inexpensive. 165 Three-dimensional echocardiography may have provided more accurate left atrial volume measurements, but the technique is technically challenging, requires very high-quality images and would almost certainly have led to further reductions in the number of patients available for these analyses.
Conclusion
The results of these exploratory analyses suggest that left atrial function in LSPAF improves after ablation by both CA and SA, as shown by reduced left atrial volumes, improved left atrial reservoir function and restored atrial contraction. Further studies are required to analyse the effect of isolated lesions on regional left atrial wall motion and over longer periods.
Chapter 7 Cardiac magnetic resonance imaging data analyses
Volumetric, structural and functional changes of the left atrium following ablation
Background
Left atrial dilatation is associated with AF, but whether it is the cause or the effect is still a controversial issue. Atrial remodelling in the presence of AF consists of biochemical, electrical, autonomic and structural changes with or without fibrosis. 166,167 Left atrial dilatation shows strong linear correlation with the duration of AF; therefore, in patients with LSPAF, a progressive electroanatomical remodelling is thought to lead to irreversible left atrial dilatation with commonly seen post-ablation stunning and recurrence of AF. 99,166,168 The chances of restoring the SR through ablation are inversely proportional to the size of the LA. 169–172 The remodelling process can be interrupted by the restoration of SR using AADs, cardioversion or ablation, which should be performed in the early stages of AF. Maintaining SR then leads to RRM, effectively a reduction in the left atrial volume, which was shown to be a predictor of clinical success following ablation. 173–175 Clinical decision-making regarding the appropriateness of an interventional AF strategy often rests on LA chamber size and duration of AF. 169–171,176
In the previous chapter, we analysed changes in left atrial volumes and function following CA and SA using echocardiography. Here, the objectives were to evaluate the same parameters using CMRI, which has superior spatial resolution. 172,174 We also present the results of right atrial size and function assessments, which is not possible to assess with the same degree of consistency using echocardiography. Changes in biatrial sizes and function will be correlated with the ablation modalities and AF recurrence in our cohort of difficult-to-treat patients with LSPAF. Using cardiac magnetic resonance images, we also evaluated changes in PV diameters following ablation, and defined > 50% stenosis or occlusion as serious procedure-related adverse events. 177,178
Methods
Cardiac magnetic resonance imaging protocol
A 1.5-T MRI system, with an 18-channel transmit/receive body coil and a 32-channel transmit/receive spine coil (Magnetom Avanto, Siemens Healthcare, Erlangen, Germany), was used to scan patients. Images of the atria were acquired in the two-chamber and four-chamber orientation using a breath-hold ECG-gated steady-state free precession cine sequence. Breath-hold ECG-gated steady-state free precession cine acquisitions (echo time = 1.2 milliseconds; repetition time = 2.8 milliseconds; spatial resolution = 1.5 mm × 1.5 mm × 8 mm; correct temporal resolution = 28 milliseconds) were obtained in two- and four-chamber orientations, followed by stacks of short-axis and transverse acquisitions covering the whole heart. ECG gating was retrospective for patients in SR and prospective for patients in AF to reduce HR variability artefacts.
Measuring atrial volumes and function with cardiac magnetic resonance imaging
The LAmax and LAmin were defined as left atrial volume just prior to the mitral valve opening and its closure, respectively. Right atrial maximal volume (RAmax) and right atrial minimal volume (RAmin) were defined as the right atrial volume just prior to the tricuspid valve opening and its closure, respectively. For left atrial volume calculation, the biplane area–length method was used, but the complex right atrial morphology, including right atrial appendage, was measured from a stack of contiguous transaxial cines encompassing the entire right atrium (RA) (as shown in Figures 16a and 16b, respectively). 179,180 Left atrial volumes at P wave were not calculated by CMR, and so conduit function and contractile function could not be assessed.
FIGURE 16.
(a) Illustration of methods for measurements of atrial volumes. Areas were measured in two-chamber (left panel) and four-chamber (right panel) views at the ventricular end-systole (LAmax) and end-diastole (LAmin), with the exclusion of the LAA and the ostia of the PVs. (b) Example of transaxial RA stack cines used to calculate right atrial volumes.

The RA endocardial borders were delineated in all slices in both ventricular end-systole (RAmax) and end-diastole (RAmin), with the inclusion of the atrial appendage and exclusion of the cava veins and the coronary sinus orifice.
From the LAmax measurements, we calculated the RRM, which is defined as the percentage reduction in left atrial volume following ablation from baseline to 6 months’ follow-up:
Atrial emptying fraction (left atrial EF and right atrial EF) was measured using the following equations:
Active and passive phasic functions of the atria were not considered in this study.
Absolute values of left atrial volume measurements using two imaging modalities are different, but we observed a high correlation (p = 0.0001) between dimensions of LAmax measured with echocardiography and magnetic resonance (correlation coefficient 0.82, 95% CI 0.647 to 0.993; beta coefficient 0.749).
Statistical analyses
The analyses presented in this chapter are descriptive and exploratory in nature and based on a small sample.
Continuous variables that followed normal distribution are presented as means with SDs. Student’s t-test and analysis of variance methods were used to assess differences between groups for baseline data. For 6-month continuous data, analysis of covariance method was used to estimate for effect size and adjust for baseline measurements. Continuous variables not normally distributed are presented as medians and IQRs, and between-group comparisons were made using the Mann–Whitney U-test. Categorical values are expressed as percentages and comparisons made using a chi-squared test. ROCs curves were used to calculate the AUC of the variable associated with AF recurrence. Using the ROC analysis, the optimal cut-off values for the variables associated with AF recurrence were calculated by using Youden’s index. A Cox regression model was used to calculate hazard ratios and 95% CIs for variables associated with AF recurrence. Kaplan–Meier curve analysis was used to show freedom from AF recurrence of the optimal cut-off threshold of the factor derived from ROC curve analysis. Statistical analysis was performed using IBM SPSS Statistics version 25 (IBM Corporation, Armonk, NY, USA).
Results
Patients
A total of 115 patients with LSPAF underwent CA or SA in the CASA-AF trial. Eleven patients did not undergo CMRI at baseline for the following reasons: two patients had a permanent pacemaker, three patients were claustrophobic, one patient had renal disease, and five patients did not undergo the procedure because of long waiting lists. Following ablation, a further eight patients did not attend their follow-up appointment, eight patients had additional CA prior to their 6-month follow-up CMR, two patients had a permanent pacemaker inserted because of bradycardia, two patients were in another hospital for management of another condition, and one patient died. We have therefore analysed data from 83 patients who had both baseline and 6-month CMRI scans, of whom 45 underwent CA and 38 underwent SA.
The baseline demographic data of the two treatment groups were similar apart from a higher prevalence of respiratory disease in the SA group than in the CA group (see Appendix 3, Table 36).
Atrial volumes, ejection fraction and reverse remodelling: comparisons between treatment arms (catheter ablation vs. surgical ablation)
Left atrial volumes significantly reduced in size 6 months after ablation in both treatment arms (Table 23). LAmax was reduced by a mean ± SD of 12.7 ± 13.4 ml/m2 in the CA arm (p ≤ 0.001) and 5.6 ± 11.5 ml/m2 in the SA arm (p = 0.005). LAmin was reduced by a mean ± SD of 18.4 ± 13.9 ml/m2 in the CA arm (p ≤ 0.001) and by 12.1 ± 11.1 ml/m2 in the SA arm (p ≤ 0.001).
Parameters | Time point | Treatment arm, mean ± SD (95% CI) | p-value | |
---|---|---|---|---|
CA (n = 45) | SA (n = 38) | |||
LAmax (ml/m2) | Baseline | 62.0 ± 13.4 (58.0 to 66.0) | 63.8 ± 17 (58.2 to 69.4) | 0.60 |
6 months | 49.3 ± 14 (46.4 to 53.3) | 58.2 ± 16.7 (53.8 to 61.3) | 0.003 | |
p-value | < 0.001 | < 0.01 | ||
LAmin (ml/m2) | Baseline | 51.8 ± 13.4 (47.7 to 55.9) | 52.7 ± 13.2 (48.4 to 57.1) | 0.75 |
6 months | 33.8 ± 13.3 (30.2 to 37.3) | 39.9 ± 14.6 (36.2 to 43.8) | 0.019 | |
p-value | < 0.001 | < 0.001 | ||
Left atrial EF (%) | Baseline | 17.4 ± 9.4 (14.6 to 20.2) | 16.8 ± 8.8 (13.9 to 19.7) | 0.78 |
6 months | 32.9 ± 9.9 (30.1 to 35.7) | 30.9 ± 9.5 (28.1 to 34.2) | 0.39 | |
p-value | < 0.001 | < 0.001 | ||
RAmax (ml/m2) | Baseline | 71.4 ± 17.9 (65.9 to 76.9) | 66 ± 16.2 (60.5 to 71.6) | 0.18 |
6 months | 58 ± 15.6 (53.2 to 60.7) | 57.6 ± 14.7 (55.6 to 63.7) | 0.33 | |
p-value | < 0.001 | < 0.001 | ||
RAmin (ml/m2) | Baseline | 60.9 ± 18.1 (55.3 to 66.5) | 59.3 ± 14.8 (54.3 to 64.4) | 0.69 |
6 months | 36.0 ± 12.7 (32.6 to 39.2) | 38.7 ± 12 (35.7 to 42.8) | 0.18 | |
p-value | < 0.001 | < 0.001 | ||
Right atrial EF (%) | Baseline | 15.2 ± 10.7 (11.9 to 18.5) | 10 ± 6.3 (7.8 to 12.1) | 0.013 |
6 months | 37.7 ± 12.4 (33.6 to 41.7) | 33.1 ± 8.4 (30.2 to 36.0) | 0.072 | |
p-value | < 0.001 | < 0.001 | ||
LA RRM (%) | –19.6 ± 20.6 (–25.8 to –13.4) | –7.6 ± 17.8 (–13.4 to –1.7) | 0.006 | |
RA RRM (%) | –15.9 ± 24.1 (–23.7 to –8.1) | –10.6 ± 16.6 (–16.5 to –4.8) | 0.30 |
Right atrial volumes were also significantly reduced following ablation. RAmax was reduced by a mean ± SD of 12.9 ± 16 ml/m2 in the CA ablation group (p ≤ 0.001) and by 8.2 ± 11.1 ml/m2 in the SA group (p ≤ 0.001). RAmin was reduced by a mean ± SD of 24.6 ± 16.2 ml/m2 in the CA arm (p ≤ 0.001) and by 20.9 ± 11.1 ml/m2 in the SA arm (p ≤ 0.001).
Emptying fractions of both atria improved significantly following ablation in both treatment arms and the increase was particularly marked for the RA. Right atrial EF was significantly poorer in the SA arm at baseline, but the significance was lost in the post-ablation period.
Between-group comparisons suggest that LAmax was significantly more reduced in the CA arm than in the SA arm (p = 0.011).
Significant reductions in right atrial volumes were observed in both treatment arms, but there was no difference in the extent of the reduction in RAmax or RAmin between treatment arms.
Left atrial EF was similar between treatment arms at baseline and at the 6-month follow-up. Right atrial EF was significantly better in the CA arm at baseline and remained better at follow-up in the CA arm than in the SA arm.
There was significantly more RRM in the LA in the CA group than in the thoracoscopic SA group (mean ± SD –19.6% ± 20.6% vs. –7.6% ± 17.8%; p = 0.006). RRM of the RA was not significantly different between the treatment arms.
Atrial volumes, ejection fraction and reverse remodelling: comparisons between sinus rhythm and atrial fibrillation recurrence
After ablation, patients were followed up for 12 months, and their rhythm was assessed from continuous monitoring using ILR. Of the 83 patients with CMRI data, SR was achieved throughout the follow-up period in 26 patients, whereas 57 patients developed recurrences of AF. A similar proportion of patients in each treatment arm had AF recurrence (68%).
Significant reductions in left and right atrial volumes were seen in patients who maintained SR throughout follow-up, and in those who experienced AF recurrence, as shown in Table 24.
Parameters | Time point | Group, mean ± SD (95% CI) | p-value | |
---|---|---|---|---|
AF (n = 57) | SR (n = 26) | |||
LAmax (ml/m2) | Baseline | 63.4 ± 16 (59.1 to 67.6) | 61.6 ± 13 (56.3 to 66.8) | 0.61 |
6 months | 53.9 ± 15.5 (50.3 to 56.8) | 52.1 ± 16.9 (48.1 to 57.7) | 0.83 | |
p-value | < 0.001 | < 0.001 | ||
LAmin (ml/m2) | Baseline | 53.5 ± 13.8 (49.8 to 57.2) | 49.4 ± 11.9 (44.7 to 54.2) | 0.20 |
6 months | 37.3 ± 13.5 (33.3 to 39.8) | 35.1 ± 15.7 (32 to 41.5) | 0.39 | |
p-value | < 0.001 | < 0.001 | ||
Left atrial EF (%) | Baseline | 16 ± 8.2 (13.8 to 18.2) | 19.5 ± 10.4 (15.3 to 23.7) | 0.11 |
6 months | 31.2 ± 10.1 (29.1 to 34.3) | 33.8 ± 8.6 (29.2 to 36.6) | 0.62 | |
p-value | < 0.001 | < 0.001 | ||
RAmax (ml/m2) | Baseline | 71.6 ± 15.6 (67.3 to 75.9) | 63.5 ± 19.6 (55.4 to 71.6) | 0.054 |
6 months | 60.8 ± 13.2 (56.7 to 63.3) | 51.7 ± 16.9 (49.7 to 59.2) | 0.064 | |
p-value | < 0.001 | < 0.01 | ||
RAmin (ml/m2) | Baseline | 62.1 ± 14.9 (58.0 to 66.2) | 56.1 ± 19.5 (48 to 64.1) | 0.13 |
6 months | 39 ± 12.4 (35.5 to 41.4) | 33.8 ± 11.9 (31.1 to 39.6) | 0.24 | |
p-value | < 0.001 | < 0.001 | ||
Right atrial EF (%) | Baseline | 13.1 ± 8.8 (10.6 to 15.5) | 12.3 ± 10.5 (8.0 to 16.7) | 0.75 |
6 months | 36.3 ± 11.1 (33.5 to 39.6) | 34.1 ± 10.5 (29.9 to 38.6 | 0.40 | |
p-value | < 0.001 | < 0.001 | ||
LA RRM (%) | –12.9 ± 21.9 (–19.1 to 7.4) | –15.9 ± 15.6 (–22.4 to –9.4) | 0.58 | |
RA RRM (%) | –14.2 ± 22.5 (–17.9 to –7.0) | –11.9 ± 18 (–26.3 to –4.8) | 0.56 |
The LAmax values at baseline (mean ± SD 61.6 ± 13 vs. 63.4 ± 16 ml/m2; p = 0.58) and 6 months after ablation (mean ± SD 52.1 ± 16.9 vs. 53.9 ± 15.5 ml/m2; p = 0.63) did not differ significantly between patients who maintained SR throughout follow-up and those who experienced AF recurrence. Also, LAmin values at baseline (mean ± SD 49.4 ± 11.9 vs. 53.5 ± 13.8 ml/m2; p = 0.18) and at 6 months’ follow-up (mean ± SD 35.1 ± 15.7 vs. 37.3 ± 13.5 ml/m2; p = 0.56) were similar in both groups.
The RAmax values were larger at baseline in patients with recurrent AF than in those who maintained SR, but this was not significant (mean ± SD 71.6 ± 15.6 vs. 63.5 ± 19.6 ml/m2; p = 0.08). RAmax measurements at follow-up were significantly smaller in patients who were in SR throughout follow-up than in those with recurrent AF (mean ± SD 51.7 ± 16.9 vs 60.8 ± 13.2 ml/m2; p = 0.026).
Cardiac magnetic resonance imaging measurements of the EF in both atria showed significant improvements following ablation, but the values did not differ between patients in SR and those with AF recurrence at both time points.
Predictors of atrial fibrillation recurrence
Cox regression analysis was performed to identify factors associated with AF recurrence (Table 25).
Parameter | Hazard ratio | 95% CI | p-value |
---|---|---|---|
Baseline | |||
Age (years) | 1.00 | 0.97 to 1.03 | 0.99 |
Sex (male) | 1.15 | 0.65 to 2.02 | 0.64 |
AF duration (days) | 1.00 | 0.999 to 1.001 | 0.94 |
BMI (kg/m2) | 1.01 | 0.96 to 1.07 | 0.67 |
Smoking | 1.04 | 0.76 to 1.42 | 0.81 |
Hypertension | 0.83 | 0.49 to 1.40 | 0.48 |
Diabetes | 1.25 | 0.84 to 1.86 | 0.27 |
Stroke | 0.65 | 0.09 to 4.66 | 0.66 |
Coronary artery disease | 2.24 | 1.12 to 4.50 | 0.02 |
Respiratory disease | 0.50 | 0.21 to 1.16 | 0.10 |
LAmax (ml/m2) | 1.01 | 0.99 to 1.02 | 0.70 |
LAmin (ml/m2) | 1.01 | 0.99 to 1.03 | 0.33 |
Left atrial EF (%) | 0.98 | 0.96 to 1.01 | 0.26 |
RAmax (ml/m2) | 1.01 | 0.99 to 1.03 | 0.07 |
RAmin (ml/m2) | 1.01 | 1.00 to 1.03 | 0.20 |
Right atrial EF (%) | 1.01 | 0.98 to 1.04 | 0.57 |
6 months | |||
LAmax (ml/m2) | 1.00 | 0.99 to 1.02 | 0.60 |
LAmin (ml/m2) | 1.01 | 0.99 to 1.02 | 0.49 |
Left atrial EF (%) | 0.98 | 0.96 to 1.01 | 0.20 |
RAmax (ml/m2) | 1.03 | 1.01 to 1.05 | 0.01 |
RAmin (ml/m2) | 1.02 | 1.01 to 1.05 | 0.04 |
Right atrial EF (%) | 1.01 | 0.98 to 1.03 | 0.78 |
Coronary artery disease at baseline appears to be an independent predictor of AF recurrence, although the analyses were based on a very small sample.
An increasing RAmax in data collected at 6 months’ follow-up appears to be predictive of AF recurrence (hazard ratio 1.03, 95% CI 1.01 to 1.05; p = 0.01).
Receiver operating characteristic curve analysis showed that RAmax (ml/m2) measured 6 months after ablation is strongly associated with AF recurrence (AUC 0.673, 95% CI 0.527 to 0.818; p = 0.017 for measurements at 6 months’ follow-up) (Figure 17).
FIGURE 17.
The RAmax values (ml/m2) measured at 6 months associated with AF recurrence.

At 6 months, a cut-off RAmax value of 49.9ml/m2 had a sensitivity of 81.6% and a specificity of 56.5%, resulting in a positive predictive value of 80% and a negative predictive value of 59.1% (see Figure 16).
Using Kaplan–Meier survival analysis (Figure 18), we show that 80% of the patients with a 6-month RAmax > 49.9 ml/m2 had AF recurrence within the follow-up period. AF was significantly less likely to return in patients who had a RAmax < 49.9 ml/m2 at 6 months’ follow-up (hazard ratio 2.67, 95% CI 1.29 to 5.53; p = 0.008, log-rank p = 0.003).
FIGURE 18.
Kaplan–Meier survival curves predicting AF recurrence with RAmax values (< 49.9 and > 49.9 ml/m2) measured at 6 months’ follow-up.

Pulmonary vein measurements
The PV measurements obtained from a subgroup of patients in CMRI analyses suggest that there are small reductions in PV diameter post ablation, ranging from 5% to 11%.
The reductions in size of all PVs in the CA group were significant, whereas in the SA group only the left inferior PV was significantly reduced in size following ablation, as shown in Table 26.
PV | Time point | Treatment arm, mean ± SD (95% CI) (mm) | p-value | |
---|---|---|---|---|
CA (n = 45) | SA (n = 38) | |||
RSPV | Baseline | 18.9 ± 3.1 (18.0 to 19.9) | 18.1 ± 2.6 (17.3 to 19) | 0.22 |
6 months | 17.5 ± 2.8 (16.7 to 18.4) | 17.2 ± 2.2 (16.5 to 17.9) | 0.56 | |
RIPV | Baseline | 18 ± 3 (17.1 to 18.9) | 16.8 ± 3.1 (15.8 to 17.8) | 0.08 |
6 months | 16.5 ± 2.9 (15.6 to 17.4) | 15.7 ± 2.9 (14.8 to 16.7) | 0.25 | |
LSPV | Baseline | 16 ± 2.9 (15.4 to 17.2) | 15.9 ± 2.8 (15.0 to 16.9) | 0.54 |
6 months | 14.9 ± 2.8 (14 to 15.7) | 14.9 ± 3.2 (13.7 to 16) | 0.99 | |
LIPV | Baseline | 15.8 ± 2.7 (14.7 to 16.8) | 15.8 ± 2.7 (14.9 to 16.7) | 0.98 |
6 months | 14.2 ± 3.6 (13.1 to 15.4) | 13.2 ± 2.4 (12.3 to 14) | 0.14 |
As described earlier, one patient in the SA arm had severe PV stenosis of the left inferior PV and occlusion of the left superior PV that required treatment. One patient in the CA arm had ≥ 50% stenosis of the left inferior PV that did not require treatment. As described in Chapter 4, the rates of moderate/severe stenosis were similar in both arms.
Pulmonary vein diameters at baseline and at the 6-month follow-up time point were similar when patients were compared in groups on the basis of AF recurrence, as shown in Table 27.
PV | Time point | Group, mean ± SD (95% CI) (mm) | p-value | |
---|---|---|---|---|
AF (n = 57) | SR (n = 26) | |||
RSPV | Baseline | 18.4 ± 3 (17.6 to 19.2) | 19 ± 2.7 (17.9 to 20.1) | 0.42 |
6 months | 17.5 ± 2.6 (16.8 to 18.2) | 17.2 ± 2.6 (16.1 to 18.2) | 0.60 | |
RIPV | Baseline | 17.3 ± 3 (16.5 to 18.1) | 17.8 ± 3.3 (16.5 to 19.2) | 0.49 |
6 months | 16.2 ± 3 (15.3 to 17.0) | 16.1 ± 2.8 (15.0 to 17.2) | 0.97 | |
LSPV | Baseline | 16.1 ± 3 (15.3 to 17.0) | 16.2 ± 2.5 (15.1 to 17.2) | 0.97 |
6 months | 14.8 ± 3.3 (13.9 to 15.7) | 15 ± 2.3 (14.0 to 15.9) | 0.84 | |
LIPV | Baseline | 15.9 ± 3.1 (15.0 to 16.7) | 15.5 ± 2.9 (14.3 to 16.7) | 0.64 |
6 months | 13.4 ± 3.1 (12.6 to 14.3) | 14.4 ± 3.3 (13.1 to 15.8) | 0.22 |
Discussion
The atria are complex anatomical structures responsible for ventricular late diastolic filling and improvement in ventricular EF. Assessment of atrial function by imaging modalities not only provides information about mechanical function but also indirectly provides information about fibrosis that might be present before or after ablation. Significant findings of this study are as follows:
-
Advanced LA remodelling associated with LSPAF is reversible following CA and SA.
-
Atrial RRM is significantly larger in the CA group than in the SA group, and was observed irrespective of arrhythmia outcome.
-
Significant improvements in left and right atrial EF are seen following ablation regardless of the type of procedure or maintenance of SR.
-
Larger RAmax measured 6 months after ablation is associated with AF recurrence during the 12-month follow-up period.
-
PV diameters appear to be reduced following either CA or SA, with reductions being more pronounced in the CA arm.
Atrial volumes
Left atrial volumes increase with longer duration of AF and can be measured by TTE, CT or CMR.
Left atrial volumes are usually underestimated by ECG when compared with CMR. 180 In this trial, we evaluated left atrial sizes using both modalities and are able to report high correlation between them.
The LAmax value used at baseline was the same as that used to predict AF recurrence in previous studies;100,141,152,153 however, our measurements based on CMRI data did not support the use of this value.
Our CMRI data show that RAmax at 6 months is strongly associated with AF recurrence and this finding is in agreement with earlier reports. 181,182 However, the data examining the function of the RA in patients with AF are sparse as clinical investigations prior to treatment do not usually involve CMR.
Reverse remodelling
LA RRM occurred in both treatment arms, but was more pronounced in the CA group.
LA RRM in CA and surgical reduction of LA have been shown to be related to improved SR maintenance post ablation,87–190 but our data do not support this. This is likely because of the complex AF substrate in LSPAF patients, which may require more than the demonstrable atrial RRM to support SR in the long term.
Furthermore, the changes in left atrial dimensions may be due to the extensive lesions sets used in the study that involved linear lesions, wide-area circumferential ablation or complex fractionated atrial electrography ablation. 191 It has been suggested that left atrial volume reduction could be simply a consequence of post-ablation contraction due to scarring in addition to restoration of SR, which may lead to improved atrial contraction. 174,192
Surgical ablation as used in our study was successful in restoring SR in a proportion of patients and improving left atrial EF. This is in contrast to Compier et al. ,193 whose patient cohort was a diverse spectrum of the AF population with concomitant ischaemic and valvular heart disease, which may underlie the loss of atrial contraction that they reported. In our patients, there was no clinical ischaemia and no valvular heart disease, and similar lesion sets were used by the two ablation techniques. Our findings suggest that the anatomical changes that occurred before or during LSPAF can be reversed with ablation, at least partially, although this does not necessarily provide long-lasting rhythm control.
It is important to highlight the reverse right atrial remodelling that occurred in our patients without direct ablation of the tissues in that area. This effect may be present in patients in the CA group as a result of scarring caused by the ablation of the cavotricuspid isthmus line performed in this group. Itakura et al. 192 recently demonstrated similar effects in patients with persistent AF who had PV isolation and cavotricuspid isthmus ablation. The authors reported reduction in right atrial volume, reduction in right atrial annulus diameter and reduction in severity of tricuspid regurgitation. 192
Pulmonary vein diameter
Wide-area circumferential ablation in the CA group and visually sparing PVs in the SA group should minimise the damage to the PVs during ablation. We observed very mild stenosis (5–11%) in all PV diameters; these changes appeared to be significant in the CA group. The left inferior PV is situated very close to the inferior ablation line on the posterior left atrial wall, and we had two patients in the study, one in each treatment arm, with stenosis of ≥ 50% in that vein. One of the patients in the SA arm had occlusion of the left upper PV and required treatment. Acquired PV stenosis is a known complication in both SA and CA, but is on the decrease. In the intention-to-treat analyses in this trial, the incidence of moderate and severe stenosis was 1.8% in the SA group and 1.7% in the CA group, which is low in comparison with incidence levels reported in published literature. 192
Limitations
The analyses presented in this chapter are subgroup exploratory analyses, which are very underpowered, affecting the reproducibility of the results as well as the reported effects/effect sizes.
All baseline data using CMRI were collected during AF and, hence, contractility of the atria could not be measured as a surrogate marker of fibrosis prior to ablation. Compared with echocardiography, left atrial volumes were not measured at the onset of P wave on ECG and, hence, conduit and contractile functions were not measured in CMRI analysis.
Using CMRI is technically challenging because of the thin walls of the atria and image quality can be reduced by HR interval variability. When possible, cine data were acquired with retrospective ECG gating to provide images throughout the entire cardiac cycle. However, if HR variability was sufficient to reduce image quality, this was switched to prospective gating. So-called arrhythmia rejection techniques (which exclude data acquired in HR intervals outside a user-defined window) were not used as they lead to long and unpredictable breath-hold durations and image quality is often reduced because of poor respiratory control. Real-time cine imaging (which allows imaging in a single cardiac cycle) was another option; however, the spatial and temporal resolution reduce substantially in these circumstances.
Conclusions
We have shown reductions in left atrial and right atrial volumes and larger left atrial EF and right atrial EF after both CA and SA, indicating that biatrial dilatation in symptomatic LSPAF is partially reversible, and interventional ablation therapies should be encouraged at the earliest opportunity in this group of patients. Greater reduction in left atrial volumes and therefore more RRM was noted in the CA group. However, the extent of LA and RA remodelling after ablation was not a major factor in preventing AF recurrence during follow-up.
Cardiac magnetic resonance evaluation and quantification of left atrial scar
Background
Atrial fibrillation is associated with structural, functional and electrical cardiac remodelling. 194 Structural remodelling of the LA is marked by increased fibrosis, which raises risks of stroke and heart failure and is associated with poorer outcomes following ablation. 99 Atrial fibrosis was traditionally considered a consequence of AF, but it has been shown that it also exists in patients in SR, particularly those with comorbidities such as hypertension and diabetes. Fibrosis may even precede and possibly contribute to the development of AF when there are predisposing conditions. 195 Fibrotic changes in the LA ultimately lead to its enlargement, which, in LSPAF patients, is thought to be irreversible. 196 Accurate identification and quantification of fibrosis would be useful as an adjunctive tool to stratify patients and identify those who might benefit the most from ablative interventions. In addition, it may be used to help guide ablation and reduce the requirements for repeat procedures. 4 Electroanatomical mapping, performed during an electrophysiological study, is the clinical method for the assessment of atrial scarring at present. 12 This is an invasive technique exposing the patients to ionising radiation, and localising scar tissue with this method is error prone. 197,198
An alternative, non-invasive technique for detecting myocardial scar tissue is delayed enhancement magnetic resonance imaging (DE-MRI). 199 This technique depends on differences in wash-in and wash-out contrast agent kinetics between healthy and scar tissues. Scar tissue is differentiated from the healthy myocardium by its enhanced signal intensity. Non-invasive evaluation of atrial tissue has been a significant advancement in interventional EP, with atrial 3D DE-MRI used to assess patient suitability for AF ablation by identifying potential non-responders200–206 and to define the most appropriate ablation approach. 202,203,207 Furthermore, DE-MRI can assess the distribution and quantity of scar in the LA and has been shown to predict ablation outcomes and also reconnection sites (gaps in ablation lines) responsible for AF recurrence post ablation. Histopathological studies in pigs have validated DE-MRI for the characterisation of AF ablation-induced wall injury. 208
Marrouche and colleagues at the University of Utah demonstrated that the burden of atrial fibrosis can predict the success of AF ablation. 201,203,209–215 However, their technique and results have been very difficult to replicate by other centres around the world. 216–218 Furthermore, all the MRI data used in these papers were exclusively acquired in SR. Low-voltage areas, instead of delayed enhancement, have been suggested to be areas of increased arrhythmogenesis and targets for ablation strategies. 219
Segmentation of atrial scar from DE-MRI images is often very challenging because of poor image quality. This is a result of HR variability, residual respiratory motion-related artefacts, low signal–to–noise ratio and contrast agent wash-out during the long acquisition.
Accuracy of the scar delineation is often impaired by enhancement from nearby structures (e.g. valves and aortic wall) and blood flow. In addition, distinguishing scar tissue in a thin-walled LA is difficult even for skilled MRI experts.
A Grand Challenge (© grand-challenge.org; URL: https://grand-challenge.org) for evaluation and benchmarking of various atrial scar segmentation methods has shown promising results, although most techniques rely on manual segmentation of the left atrial wall and PV and assumed fixed thickness of the left atrial wall. 196,220,221 Manual segmentation is labour intensive and prone to human error, and it exhibits intraobserver and interobserver variation, which affects its reproducibility in multicentre and multiscanner studies. 196,221,222
Our clinical interest is in patients with LSPAF who are difficult to electrically cardiovert and maintain in SR long enough to conduct a cardiac MRI with prospective ECG gating. Our goal in this substudy was to use the technique of acquiring magnetic resonance images in patients who are in AF at the time and to then develop the methodology for automatic segmentation and quantification of the scar. We then planned to use this technique to evaluate the scar prior to and following ablation, and to compare the results in groups based on the ablation treatment and on the heart rhythm in follow-up. Here, we describe the multiview two-task segmentation (MVTT) technique, which is effective and efficient at automated LA and PV segmentation combined with quantification of left atrial scar. 223
Methods
Data acquisition
Cardiac MR data were acquired on a Siemens Magnetom Avanto 1.5-T scanner (Siemens Medical Systems, Erlangen, Germany). Transverse navigator-gated 3D DE-MRI201 was performed using an inversion-prepared segmented gradient echo sequence [TE/TR (echo time/repetition time) 2.2/5.2 milliseconds] 15 minutes after gadolinium administration (gadobutrol, Gadovist 0.1 mmol/kg body weight; Bayer Schering Pharma AG, Berlin, Germany). 224–226 The inversion time was set to null the signal from normal myocardium and varied on a beat-by-beat basis (depending on the patient’s respiratory rate interval) to maintain nulling. 227 Detailed scanning parameters have been reported previously. 222 The navigator-restore pulse was delayed by 100 milliseconds to reduce artefactual signal in the right PVs. 228 The nominal acquisition duration was 204–32 cardiac cycles assuming 100% respiratory efficiency. 228
Coronal navigator-gated 3D balanced steady state-free precession (TE/TR 1/2.3 milliseconds) roadmap data were acquired prior to contrast agent administration. Signal loss from blood arriving in the LA and PV was minimised by using the shortest possible TE/TR. 229 This was achieved by using non-selective radiofrequency excitation. 230
Segmentation
Deep-learning-based methods
Although conventional machine learning and pattern recognition-based methods, previously described by our group,223,231 can achieve promising results for LA chamber and PV segmentation with accurate atrial scar localisation, the requirement of additional roadmap data may be a hurdle for a reproducible study and not all centres have roadmap data available. 223 Recently, we proposed a deep-learning-based method to tackle this limitation. 231
Medical imaging analysis is an extremely important research area that combines advanced multidisciplinary topics. Recently, deep learning-based methods showed very promising results in medical image computing, mainly owing to the fast accumulation of medical data and graphics processing unit-powered computing resources. Deep learning is a new ‘artificial intelligence’ trend that uses a multilayer perceptron network that contains multiple hidden layers and is therefore called a deep-learning structure. 232 According to Shen et al. ,233 in terms of input types, we can consider deep models as typical multilayer hierarchical neural networks, which take non-structured vector formatted values as input or convolutional neural networks (CNNs) that take structured 2D or 3D values as input. Owing to the abundant information embedded in the neighbourhood voxels, especially for the structural medical images that normally represent anatomical characteristics of the imaged organs, CNNs have been widely used in medical image analysis.
In this study, we have developed a combined MVTT deep-learning paradigm with recursive attention that can work directly on 3D late gadolinium enhancement (LGE) cardiac magnetic resonance images to segment the LA (and proximal PVs) and delineate the scar on the same data set.
Our deep-learning method imitates the inspection method of radiologists who go through 2D axial slices to locate associated details, while still utilising complementary orthogonal views details. Thus, we divide the volume of 3D LGE CMRI into contiguous 2D slices and conduct 2D slice segmentation. This has two main advantages: (1) it improves abundant training data and (2) it provides greater memory capacity in 2D convolution. Our MVTT method consists of three major subnetworks (i.e. a multiview learning network, a dilated residual network and a dilated attention network), which automatically and concurrently execute LA and proximal PV and left atrial scars segmentations.
The MVTT system primarily consists of a multiview learning network and a dilated concentration network. The multiview learning network uses a sequential learning subnetwork to know the association between 2D axial slices. At the same time, in the sagittal and coronal views, two dilated residual subnetworks learn the complementary details. Then, we combine the two forms of complementary details into the axial slice features to get fused multiview features to accomplish the LA anatomy segmentation. Since LA wounds are very small, the dilated attention network learns from the picture of an attention map to force our network to concentrate on certain tiny regions and suppress background noise effect. The LA anatomy and left atrial scars share the multiview functionality in our MVTT to manage the two segmentation functions, which can therefore reduce the issue of error accumulation.
Each data set was scrutinised before MVTT was applied. Acquired images were scored by a senior cardiac MRI physicist on a Likert-type scale [i.e. 0 (non-diagnostic), 1 (poor), 2 (fair), 3 (good) and 4 (very good)] depending on the level of signal–to–noise ratio, quality of normal myocardial nulling and the existence of navigator beam and ghost artefacts. The data sets used had to have a Likert scale score > 2.
The quantity of scar is expressed as the percentage of surface area of the LA. The accuracy of the automatic segmentation and scar measurement was established by comparison with the manual ground truth model of scar quantification. We found that automatic segmentation measurements were highly correlated with manual measurements having a mean (± SD) Dice score of 0.82 (± 0.05) (correlation coefficient 0.902; p < 0.00001).
Statistical analysis
Descriptive statistics are used in this chapter, which is exploratory in nature.
Statistical analysis was performed using IBM SPSS Statistics version 25. Continuous variables that followed normal distribution are presented as means and SDs, and Student’s t-test was used to assess differences between groups. A paired-sample t-test was used to assess differences within groups from baseline to 6 months. Non-normally distributed continuous variables are presented as medians and IQRs, and between-group comparisons were undertaken using the Mann–Whitney U-test. Categorical variables were compared using a chi-squared test. Hazard ratios were calculated for baseline demographic variables using univariable Cox regression analysis to observe association with AF recurrence.
Results
Of 97 patients who were enrolled in the CASA-AF RCT at the Royal Brompton and Harefield NHS Foundation Trust, 17 did not undergo MRI: 13 patients at baseline and four at follow-up. The reasons were withdrawal prior to treatment (n = 3), claustrophobia (n = 2), no available slot for MRI (n = 5), advanced renal disease or another condition precluding MRI (n = 6) and death (n = 1). We have excluded MRI studies of seven patients who had an additional ablation in follow-up to ensure that comparisons of scarring between ablative modalities are not compromised.
Of the remaining 73 patients, 27 did not have MRI scans of sufficient quality for analyses (22 at baseline and five at the follow-up visit), so they too were excluded (30%).
Forty-six patients in this study had CMRI at baseline and at 6 months following ablation, and their images were of an appropriate standard for MVVT and scar assessment, as shown in Figure 19.
FIGURE 19.
Scar distribution (green rendering) based on 3D DE-MRI scan, pre and post ablation (not the same patient). (a) Pre ablation, posterior view; (b) pre ablation, anterior view; (c) post ablation, posterior view; and (d) post ablation, anterior view.

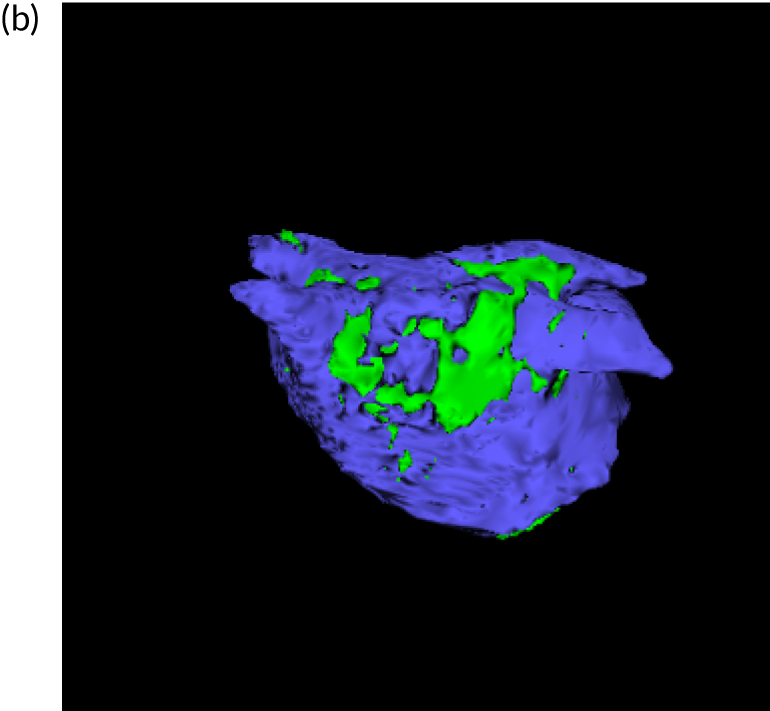
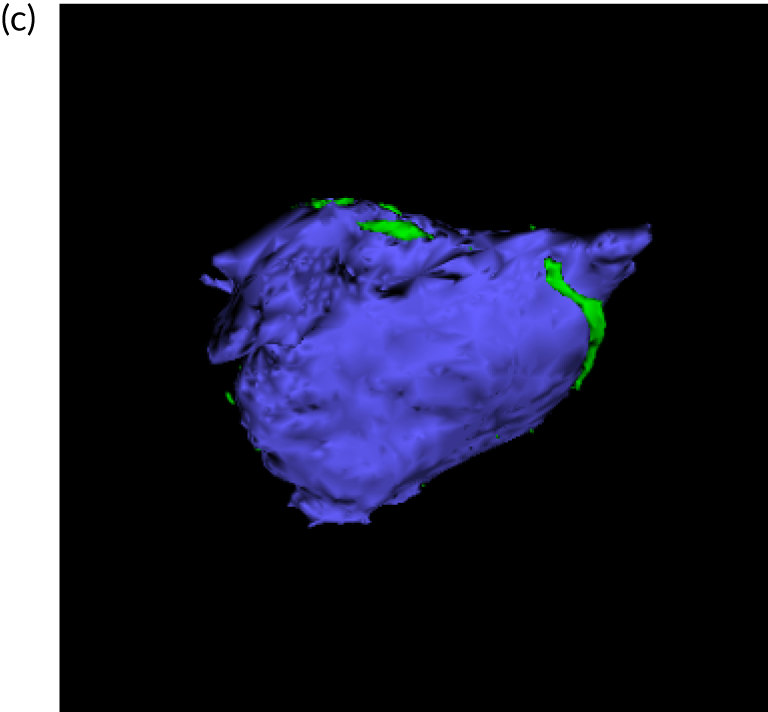

The patients considered in these analyses had a mean (± SD) age of 62.4 (± 8.6) years and 36 were male (78.3%); 27 were randomised to CA and 19 to thoracoscopic SA. Their baseline characteristics were the same as those of the remainder of the trial participants (see Table 2).
The median time from baseline MRI to treatment was 48 days (range 34–68.5 days).
Left atrial scar measurements using MVTT are presented in Table 28.
Measurement of LA scar | Treatment arm, mean ± SD (95% CI) | p-value | Group in follow-up, mean ± SD (95% CI) | p-value | ||
---|---|---|---|---|---|---|
CA (n = 27) | SA (n = 19) | SR (n = 16) | AF (n = 30) | |||
At baseline | 9.8 ± 4.9 (7.9 to 11.8) | 13.4 ± 5.8 (10.6 to 16.2) | 0.028 | 13 ± 5.2 (10.3 to 15.8) | 10.4 ± 5.6 (8.3 to 12.5) | 0.13 |
At 6 months | 29.4 ± 8.7 (26.5 to 34.2) | 29.7 ± 11.9 (23.7 to 32.9) | 0.44 | 31.1 ± 10 (25.2 to 35.1) | 28.6 ± 10.1 (25.6 to 32.7) | 0.74 |
Δ scar | 19.5 ± 8.9 (16.0 to 23.1) | 16.3 ± 10.8 (11.1 to 21.5) | 0.27 | 18.2 ± 8.6 (13.4 to 22.9) | 17.5 ± 10.5 (14.4 to 22.1) | 0.98 |
Mean (± SD) pre-ablation left atrial scar in patients randomised to CA was 9.8 (± 4.9)% and in those allocated to SA it was 13.4 (± 5.8)%. The difference in size between the two treatment groups appears to be significant, as the p-value was 0.034.
Post-ablation CMRI was performed, on average, a mean (± SD) of 173 (± 45) days after ablation. The scar area increase (Δ scar) was significant in both treatment arms following ablation: mean ± SD 19.5 ± 8.9% in the CA arm and 16.3 ± 10.8% in the SA arm (p < 0.0001 for both groups).
The proportion of scar in the LA measured at 6 months’ follow-up did not appear to differ significantly between treatment groups (p = 0.92).
Thirty patients had AF recurrence during the follow-up period. Scar measurements at baseline were similar in patients with AF recurrence and in those who maintained SR throughout follow-up (mean ± SD 10.4 ± 5.6% vs. 13 ± 5.2%; p = 0.12).
At 6 months’ follow-up, there was a significant increase in the proportion of scar in patients who maintained SR (mean ± SD Δ scar 18.2 ± 8.6%; p < 0.0001) and in those who experienced AF recurrence (mean ± SD Δ scar 17.5 ± 10.5%; p < 0.0001). The size of the increase was similar in both groups (p = 0.8).
Univariable analysis
We used demographic and clinical variables in this subgroup of patients in univariable analyses to identify a possible predictor of AF recurrence. In addition, we used left atrial scar measurements (MVTT and manual) at baseline and at 6 months to establish the correlation between fibrosis and AF recurrence. None of the variables in the univariable Cox regression analysis model appeared to achieve a significance that meritted further testing (Table 29).
Parameter | Univariable analysis | ||
---|---|---|---|
Hazard ratio | 95% CI | p-value | |
Baseline | |||
Age | 0.99 | 0.95 to 1.04 | 0.77 |
Sex (male) | 0.77 | 0.32 to 1.90 | 0.77 |
AF duration | 1.00 | 0.99 to 1.00 | 0.57 |
BMI | 1.01 | 0.94 to 1.08 | 0.65 |
Smoking | 1.73 | 0.71 to 4.2 | 0.23 |
Hypertension | 0.94 | 0.45 to 1.94 | 0.86 |
Diabetes | 1.12 | 0.62 to 2.03 | 0.71 |
Stroke | 0.70 | 0.10 to 5.11 | 0.72 |
Coronary artery disease | 0.67 | 0.23 to 1.92 | 0.45 |
Respiratory disease | 0.53 | 0.16 to 1.76 | 0.30 |
Left atrial scar MVTT | 0.96 | 0.90 to 1.03 | 0.25 |
Left atrial scar GT | 0.96 | 0.90 to 1.03 | 0.27 |
6 months | |||
Left atrial scar MVTT | 0.98 | 0.95 to 1.02 | 0.41 |
Left atrial scar GT | 0.99 | 0.95 to 1.03 | 0.65 |
Discussion
We have developed a novel, fully automatic segmentation method to detect atrial scarring in LGE-MRI images acquired uniquely during AF, and have validated it against manual ground truth segmentation carried out by experienced imaging cardiologists. 223,231 This methodology was applied in this study to quantify left atrial scars prior to and after ablation in patients with LSPAF.
The main finding of this exploratory study is that the left atrial scar is significantly increased following both ablation strategies, but we did not find it predictive of AF recurrence.
One of the key issues in left atrial scar segmentation is distinguishing the LA and the scar from the high enhancement regions, which we successfully accomplished. 223 In addition, we scanned patients in AF by adopting MRI acquisition strategies, including beat-to-beat changes in inversion time and navigator artefact reduction schemes. Despite these improvements, almost 30% of the acquired data sets in our study were not of the required standard, largely owing to the suboptimal HR control in our patients, which affected our sample size.
The multiatlas LA auto-segmentation in our study was strongly correlated with manual ground truth delineation, as shown by a consistent Dice score of > 90. 223 The development of deep-learning methods renders the analysis of scar less time-consuming and the methodology probably represents the future of image-related analyses. However, scar identification and assessment can change within the same data set with different learning methods, so accuracy and reproducibility still need to be resolved. 232–234
In our study, the amount of fibrosis identified at baseline with automatic segmentation was larger in patients in the SA group, but this may be due to a type II error as our sample size was defined by the quality of the acquired images.
Quantification of scar at baseline was < 20% of the total LA surface in our patients, so these patients would be classified as being in stage 1 and 2 of fibrosis as defined by the investigators of the DECAAF (Delayed Enhancement MRI Determinant of Successful Radiofrequency Catheter Ablation of Atrial Fibrillation) study. 201 In the DECAAF study, 92 patients had persistent or permanent AF, and pre-ablation scar measurements for 61% of patients were in stage 1 (< 10%) and 2 (> 10% and < 20%). These measurements were associated with only 15% of AF recurrence in follow-up.
Following ablation, the proportion of scar in the LA of our patients increased to around 30%, which is largely in line with electroanatomical mapping of scar and low-voltage post ablation in patients with chronic AF. 227 This is surprising as scar estimates with electroanatomical mapping during CA are significantly larger than those obtained with LGE-MRI. 213
A number of studies reported discrepancies in scar quantification using different methodologies and, at this point, we still lack a gold standard. 218,234,235
Quantification of scar is highly influenced by the CMRI acquisition sequences and post-processing methods, which then leads to non-generalisable results. 217,235 The imaging technologies, as well as electroanatomical mapping, most likely need further histological validation before being universally adopted to identify and quantify scar so that it can be used in clinical decision-making.
All our patients had an ILR implanted at the end of the ablation procedure, so the artefact from the ILR can be seen in the intrathoracic space in about one-third of the patients. It is possible that this artefact interfered with automatic LA segmentation, although the published literature suggests otherwise, and our own ground truth measurements were highly correlated with values from automatic segmentation. 221,235,236
Conclusions
In this study, we made significant progress by acquiring DE-MRI data sets in AF and developing a fully automated segmentation tool for LA and PV geometry and left atrial scar delineation. This tool has allowed us to assess the left atrial scar burden pre and post ablation, and showed that both ablative techniques increase the scar in the LA. However, the size of the scar does not appear to be predictive of AF recurrence, although this result has to be treated with caution owing to the small sample size. Further work is required to refine our segmentation and left atrial scar quantification techniques.
Chapter 8 Patient and public involvement
Our study was primarily focused on assessing the effectiveness of two different ablation techniques to establish normal heart rhythm and alleviate patients’ symptoms related to AF. The Royal Brompton and Harefield NHS Foundation Trust Patient Advisory Group (PAG) was heavily involved in the study design, from advising on the suitability of the research question, the potential benefits to AF sufferers and the practical elements of study design at the time of the grant application. This group also informed the recruitment targets, hospital visit schedule and the overall level of involvement that would be appropriate for a service user during the trial.
The study benefited enormously from patients’ input to the design of the study materials, including the PIS, the consent form and the health economic questionnaire. The materials were revised to make them easier to read, and the patients helped us decide on the appropriate level of detail to provide regarding study treatments and the risks associated with them.
The PAG was instrumental in helping us meet our recruitment target by providing appropriate information throughout all stages of the study.
Our trust has close links with the charitable organisation, the Arrhythmia Alliance, which helped us develop our study materials and increase wider public awareness of the study. Its chief executive officer is a lay member of our Trial Steering Group. The patient group at the Liverpool Heart and Chest Hospital, one of the trial sites, was also involved with evaluating our PIS and consent form before the study was given its research and development approval.
The feedback from the PAG was provided through group discussions either in person or by e-mail. Our TSC included two lay members who were regularly informed of study progress and participated in the meetings twice a year. In addition, our lay TSC members helped us design closer monitoring procedures for the trial participants once they were discharged from the hospital following treatment for AF. We were encouraged to telephone patients once per week for a month and found that this was a welcome reassurance rather than a burden.
Our monthly recruitment target was ambitious, and some study participants volunteered to be contacted by eligible patients to relay their experience with our procedures and help with recruitment.
The feedback from the lay members of the TSC and PAG helped with the conduct of the study and our recruitment success, including a very low attrition rate in the trial (5%).
Patients with AF are interested in understanding the complexities of their condition and are enthusiastic to help advance the knowledge and treatment of this disease. Our study participants were involved in discussions about the merits and rationale for continuing participant follow-up in the future, and helped us develop another funding application. They were enthusiastic about participating in the new study based on the following:
-
They considered this research important and believe that other patients would be keen to participate.
-
They were happy to share their experience of trial participation with others.
-
They considered that hospital visits during study participation are beneficial to them in other respects.
-
They had no concerns regarding emotional, safety or ethics issues with the new study.
In discussion with our lay TSC members and the Royal Brompton PAG, we have decided to write to each study participant to disseminate the results of the study. The contents of the letter were agreed with the Royal Brompton PAG, as will other materials we plan to publish online to disseminate the study results.
Throughout the study, our research was represented at numerous events, such as the Imperial Festival and Royal Brompton and Harefield Research open days, to promote the study and inform the public of our research activities. These annual events were used to inspire school children into scientific careers, encourage the public to participate in research and educate patients about interventional treatments.
Chapter 9 Discussion
Principal findings
The CASA-AF RCT is the first multicentre RCT with continuous cardiac monitoring comparing thoracoscopic SA and CA strategies as a first-line procedure in LSPAF patients. The main finding was that the rates of arrhythmia recurrence and procedure-related SAEs during the 12 months’ follow-up occurred in a similar proportion of patients in both treatment arms. Despite two decades of CA use in the treatment of AF, outcomes in persistent and long-standing persistent AF remain suboptimal. 237 The underlying substrate in LSPAF is complex and characterised by the interplay of cellular hypertrophy and fibroblastic activation and proliferation, resulting in atrial fibrosis, which in turn leads to anisotropic conduction properties and electrical dissociation. Electroanatomical remodelling is the ideal harbinger of perpetuating AF, and is the reason why these patients are so difficult to treat. At present, the European Society of Cardiology guidelines237 recommend consideration of thoracoscopic SA for symptomatic AF when CA has failed (IIaB) or for symptomatic drug refractory persistent AF or LSPAF (IIaC).
Early thoracoscopic SA investigations showed promise in terms of arrhythmia-free outcomes,238,239 and this was subsequently borne out by two out of three RCTs. In the first of these trials, the FAST study,33 124 patients had either SA or CA. The SA group had a significantly higher level of AF-free survival at 12 months without AADs than the CA group (65.6% vs. 36.5%, respectively; p = 0.0022), although the adverse event rate was considerably higher in the SA group than in the CA group (34% vs. 16%, respectively). Two-thirds of patients had an unsuccessful CA before participating in the trial and 67% had paroxysmal AF. Limitations of the trial included significant heterogeneity in lesion sets with inconsistent verification of conduction block and the use of intermittent 7-day ambulatory ECG monitoring to identify AF recurrence. The second RCT, by Pokushalov et al. ,240 enrolled 64 patients, again with a mixed caseload of paroxysmal and persistent AF and failed initial CA. At 12 months, SA was superior to CA in terms of AF-free survival (81% vs. 47%, respectively; p = 0.004). AF recurrence in this study was identified by continuous cardiac monitoring using an ILR. The third randomised study, by Adiyaman et al. ,241 was a smaller non-inferiority trial with 52 (74%) predominantly paroxysmal patients, again, using continuous cardiac monitoring over 2 years’ follow-up. CA was not inferior to SA in terms of freedom from arrhythmia at 2 years (56% vs. 29%, respectively; log-rank p = 0.059) and was not associated with major complications, unlike SA, which had a 20% major complication rate.
In keeping with the majority of these trials, in our own pilot non-randomised study, 73% of participants with LSPAF were free from AF/AT 12 months after SA, compared with 32% of participants after CA using intermittent 7-day ambulatory ECG monitoring in follow-up. 242 This current study was subsequently designed to determine whether SA was clinically better in restoring normal heart rhythm, and to further evaluate the effects of both procedures on patients’ quality of life and health economic outcomes in a multicentre RCT. All our patients were diagnosed with de novo LSPAF and had their first invasive treatment in large-volume centres using continuous cardiac monitoring to measure rhythm outcomes. We found no difference in the proportion of patients who were arrhythmia free at the end of the follow-up between the two treatments. Although this finding contradicts the results of our pilot study, there are a few possible explanations for the difference in results.
First, the use of continuous cardiac monitoring is far more sensitive for detecting AF recurrence than intermittent monitoring methods, especially in patients without any symptoms. 243 Thus, intermittent monitoring significantly overestimates the success rate of ablation procedures,55,242,244,245 and this is likely to be the main reason for the difference in our outcomes compared with other studies.
Second, in our trial, the recruited patients all had true de novo LSPAF with a mean time from persistent AF diagnosis to ablation of just less than 2 years, and an overall AF history of approximately 3 years. It is well known that LSPAF is resistant to different treatment approaches, and our results are in line with those experiences. In keeping with previous reports, in this population, a single CA procedure achieves AF/AT-free status without AADs in approximately 30% of cases. 26,32,242 However, the SA results we report are in contrast to previous studies, except that of Adiyaman et al. ,241 which may be due to the different characteristics of the study populations. The key difference is that previous studies included a large proportion of patients who had unsuccessful prior CA procedures, hence the SA procedure was, in essence, a reverse-staged hybrid case (CA then SA), which extended or completed previous lesion sets. Therefore, the efficacy of SA in these studies is in fact the efficacy of two ablative procedures done in succession.
Third, although the SA operators in our study were expert cardiothoracic surgeons skilled in thoracoscopic techniques, their experience in treating AF thoracoscopically was still relatively limited in comparison with the larger volume of experience of the electrophysiologists delivering CA treatment. It is therefore likely that specific procedural expertise may have played a role in the final outcomes.
Finally, the evolution of CA techniques, including the use of contact force-sensing catheters, high-density multielectrode mapping and lesion predication algorithms, has led to incremental benefits in the durability of lesion sets and a reduction in the potential benefit of direct application of the radiofrequency to the myocardial tissues as performed in SA. 13,246–248
Clinical outcomes
The clinical improvement, as measured by ≥ 75% reduction in time spent in AF/AT with just one procedure and without AADs, was seen in about 70% of study participants, similar in both treatment arms. Given that these patients were highly symptomatic (i.e. had a EHRA symptom score ≥ 3) and had been in continuous AF for 2 years before the procedure, this reduction in AF burden provides significant clinical benefits, as can be seen from the results of the health economic analyses.
To aid clinical management of patients with LSPAF, we suggest that the AF burden reduction is used as a guide for outcomes, because this is more reflective of the clinical improvement that patients derive post ablation. We also advocate the use of continuous cardiac monitoring for accurate assessment of the burden of arrhythmia both before, if possible, and after ablation.
Safety outcomes
Despite one death occurring in the SA arm, we found no difference in the rate of SAEs (major complications) occurring within 30 days of the ablation between arms: 10% in the CA arm and 13% in the SA arm. Rates of SAEs over the entire 12-month follow-up period were also not significantly different between the treatment arms, as 8 out of 60 (13.3%) people in the CA arm and 10 out of 55 (18.2%) people in the SA arm experienced a serious procedure-related complication.
In comparison with other trials,33,240,241 our procedure-related complication rates within 30 days are low, which reflects the considerable experience of the operators involved in the trial.
Atrial anatomy and function outcomes
Using both standard and advanced echocardiography techniques, we have shown that in LSPAF the left atrial function does improve after either CA or SA. We see RRM in terms of reduced left atrial volumes and also improvement in left atrial function, with improved left atrial reservoir function and restored atrial contraction. The degree of RRM seems to be greater in the CA group than in the SA group, as evidenced by better left atrial strain reservoir and conduit functions at 12 months. Furthermore, in those who maintain SR, multiple parameters (TDI and speckle-tracking strain and strain rate) confirm an improvement in left atrial function.
From the MRI perspective, it is important to highlight that, in LSPAF patients, RRM of both the LA and RA does occur after CA or SA. The degree of remodelling was greater in the CA group than in the SA group, but we were unable to show a difference in the degree of RRM between patients who maintained SR and those with AF recurrence. The right atrial size in our cohort was predictive of success. Mechanistically, we demonstrated that it is possible to acquire good-quality images during AF, which we used to develop a fully automatic segmentation tool for left atrial scar quantification. The automated tool was validated against manual ground truth segmentation and showed that left atrial scar increases post ablation, but baseline left atrial scar was not predictive of procedural success. These anatomical and mechanistic findings in LSPAF are encouraging and support our clinical outcomes by showing that RRM is possible in LSPAF, with significant rates of clinical success as determined by AF burden reduction and improvement in quality of life. However, additional work is required to further delineate thresholds for treatment using left atrial size and refine the methodology for left atrial scar quantification to assess its clinical use as a prognostic tool.
Using both echo imaging and MRI, we have shown that the adverse remodelling associated with LSPAF is in fact reversible, and that left atrial function can improve significantly with restoration of SR. Imaging markers can also provide valuable predictors of success. Therefore, depending on local resources, imaging should be considered as an integral part of the decision-making process when considering interventional strategies for LSPAF, as well as to assess the post-ablation impact on atrial structure and function.
Quality of life and health economics
The economic evaluation was based on direct observations of health outcomes and costs over the trial period. We found consistent improvements over time for different outcome measures, including AF symptoms (EHRA symptom score), AF-related quality of life (AFEQT) and generic measures of quality of life (EQ-5D VAS and index score). Improvements in the first 3 months were sustained up to the end of follow-up at 12 months. Slightly larger improvements were measured in patients randomised to CA than to SA. Over the year, CA was associated with a statistically significant gain of 0.069 QALYs and an NHS cost-saving of £3534 per patient compared with SA. This translates to an INB of £4918 for CA compared with SA at a conservative cost-effectiveness threshold of £20,000 per QALY. The estimated probability that CA is less expensive and more effective than SA is 98.9%. Clinical results from the trial suggest that this result would not change with extrapolation, as SA was not associated with prolonged freedom from arrhythmia or reduction of other risks.
Limitations
There are several limitations of this study. First, the sample size does not allow for comparisons of differences in efficacy < 30%, which was deemed clinically obtainable and relevant. Second, the study treatments were performed in four highly specialised centres in England, which may have an impact on generalisability. Compared with CA, thoracoscopic AF ablation is not yet an established or widely available procedure, and as such it is performed by teams whose clinical experience is not matched to those that deliver CA. Finally, differences in structural and functional cardiac changes between treatment arms, where they exist, are observational only and based on a considerably reduced number of data points because of incomplete imaging data for all patients.
Chapter 10 Conclusion
The most important finding from this multicentre RCT was that no evidence was found to support the original hypothesis that SA is clinically superior to CA as the first-line ablation strategy for LSPAF patients.
Both treatments appear to offer significant clinical and symptomatic improvements with modest major complication rates.
Surgical ablation, however, is a more invasive and more expensive procedure, and post-ablation quality-of-life measures are poorer with SA than with CA. On this basis, we consider CA to be the first-line treatment of choice for patients with de novo LSPAF.
Acknowledgements
Data Monitoring Committee members
Malcolm Walker (chairperson), Gareth Ambler and Ranjit Deshpande.
Trial Steering Committee members
William D Toff (chairperson), Tim Betts, Sylvia Clarke, Guy Haywood, Steven Hunter and Trudie Lobban.
Adverse events independent review committee
Malcolm Dalrymple-Hay, Ross Hunter, Chris Pepper and Paul Ridley.
Implanted loop recorder data quality control at Barts Health NHS Trust
Hollie Granville and Sophia John.
CASA-AF investigators
Royal Brompton and Harefield NHS Foundation Trust
Toufan Bahrami, Joanne Benton, Vennela Boyalla, Ruth Chester, Eliane Cunliffe, Lucy Edmondson, Matthew Gill, Vicky Griffiths, Shouvik Haldar, Rebecca Harman, Catherine Huggett, Wajid Hussain, David G Jones, Jenny Keegan, Habib R Khan, Rajdeep Khattar, Kevin Kirby, Ines Kralj-Hans, Karen Lascelles, Ramasamy Manivarmane, Raad Mohiaddin, Iulia Munteanu, Eva Nyktari, Karen O’Brien, Tess Phyl, Shelley Rahman-Halley, Tsveta Rahneva, Cheryl Riley, Paula Rogers, Anitha Sathishkumar, Katherine Smith, Rick Wage, Cathy West, Joyce Wong, Aaisha Opel, Zhong Chen, Riyaz Kaba, Yakup Yakupoglu and Guang Yang.
Liverpool Heart and Chest Hospital
Audra Audraite, Sandra Belchambers, Timothy Fairbairn, Dhiraj Gupta, Susan Hughes, Neeraj Mediratta, Maureen Morgan, Christina Ronayne, Rob Shaw, Paulinus Simkus and Clive Taylerson.
Brighton and Sussex University Hospitals NHS Trust
Chloe Bruce, Jonathan Hyde, Michael Lewis, James McCready, Andrew McGregor and Catherine Thomson.
Contributions of authors
Shouvik Haldar (https://orcid.org/0000-0001-8129-8520) (Consultant Cardiologist) and Tom Wong (https://orcid.org/0000-0002-6484-4961) (Consultant Cardiologist) conceived the study and obtained the funding, with advice from Darrel Francis (https://orcid.org/0000-0002-3410-0814) (Professor of Cardiology) at the application stage.
Habib R Khan (https://orcid.org/0000-0002-4828-5768) (Clinical Research Fellow), Vennela Boyalla (https://orcid.org/0000-0002-0285-171X) (Clinical Research Fellow) and Ines Kralj-Hans (https://orcid.org/0000-0001-7401-6281) (Clinical Trial Manager) made substantial contributions to the conduct of the trial and the acquisition of data presented in the manuscript.
Shouvik Haldar, Habib R Khan, Vennela Boyalla and Ines Kralj-Hans prepared the drafts of the manuscript.
Simon Jones (https://orcid.org/0000-0002-8267-5647) (Research Professor) conducted the statistical analyses presented in Chapters 3 and 4.
Joanne Lord (https://orcid.org/0000-0003-1086-1624) (Professorial Fellow) and Oluchukwu Onyimadu (https://orcid.org/0000-0002-1724-3485) (Senior Research Assistant) conducted the health economic analyses.
Habib R Khan conducted the analyses presented in Chapters 6 and 7 to fulfil the requirements of his Doctor of Philosophy degree.
Anitha Sathishkumar (https://orcid.org/0000-0001-9818-9259) (Chief Cardiac Physiologist) evaluated the heart rhythm data from all study participants.
Toufan Bahrami (https://orcid.org/0000-0002-1402-8771) (Cardiothoracic Surgeon), Anthony De Souza (https://orcid.org/0000-0002-6244-821X) (Cardiothoracic Surgeon), Neeraj Mediratta (https://orcid.org/0000-0003-3134-4116) (Cardiothoracic Surgeon), Jonathan Hyde (https://orcid.org/0000-0002-0699-7281) (Cardiothoracic Surgeon) and Michael Lewis (https://orcid.org/0000-0003-2466-4867) (Cardiothoracic Surgeon) conducted thoracoscopic SAs in study participants.
Jonathan Clague (Consultant Cardiologist), Dr Wajid Hussain (https://orcid.org/0000-0003-4104-1821) (Consultant Cardiologist), Julian Jarman (https://orcid.org/0000-0002-8274-5676) (Consultant Cardiologist), David G Jones (https://orcid.org/0000-0003-3921-4906) (Consultant Cardiologist), Tushar Salukhe (https://orcid.org/0000-0002-3505-9905) (Consultant Cardiologist), Vias Markides (https://orcid.org/0000-0002-9385-1298) (Consultant Cardiologist), James McCready (https://orcid.org/0000-0002-5430-7871) (Consultant Cardiologist) and Dhiraj Gupta (https://orcid.org/0000-0002-3490-090X) (Consultant Cardiologist) screened and referred patients for treatment and conducted CAs in study participants.
Raad Mohiaddin (https://orcid.org/0000-0002-1768-7457) (Professor Of Cardiovascular Imaging) and Rajdeep Khattar (https://orcid.org/0000-0001-5992-2315) (Consultant Cardiologist in Adult Echocardiography) provided guidance related to CMRI and echocardiographic data acquisition, analysis and interpretation.
Caroline Murphy (https://orcid.org/0000-0001-7547-8998) (Operational Director) and Joanna Kelly (https://orcid.org/0000-0002-4389-5284) (Strategic Data Management Lead) provided advice on the development of the trial database and data capture forms, as well as support in the management and conduct of the trial.
Zhong Chen (https://orcid.org/0000-0001-7201-7491) (Consultant Cardiologist) and William D Toff (https://orcid.org/0000-0001-5631-4496) (Associate Professor in Cardiology) provided input regarding quality assessment of the heart rhythm data and ascertainment of adverse events in the study.
All authors read and revised the manuscript for important intellectual content and agreed on the final version submitted.
Publications
Khan HR, Kralj-Hans I, Haldar S, Bahrami T, Clague J, De Souza A, et al. Catheter ablation versus thoracoscopic surgical ablation in long standing persistent atrial fibrillation (CASA-AF): study protocol for a randomised controlled trial. Trials 2018;19:117.
Haldar S, Khan HR, Boyalla V, Kralj-Hans I, Jones S, Lord J, et al. Catheter ablation vs. thoracoscopic surgical ablation in long-standing persistent atrial fibrillation: CASA-AF randomized controlled trial. Europ Heart J 2020;41:4471–80.
Data-sharing statement
Data sharing requests should be directed to the corresponding author. Access to available anonymised, aggregated data may be granted following review.
Patient data
This work uses data provided by patients and collected by the NHS as part of their care and support. Using patient data is vital to improve health and care for everyone. There is huge potential to make better use of information from people’s patient records, to understand more about disease, develop new treatments, monitor safety, and plan NHS services. Patient data should be kept safe and secure, to protect everyone’s privacy, and it’s important that there are safeguards to make sure that it is stored and used responsibly. Everyone should be able to find out about how patient data are used. #datasaveslives You can find out more about the background to this citation here: https://understandingpatientdata.org.uk/data-citation.
Disclaimers
This report presents independent research. The views and opinions expressed by authors in this publication are those of the authors and do not necessarily reflect those of the NHS, the NIHR, the MRC, NETSCC, the EME programme or the Department of Health and Social Care. If there are verbatim quotations included in this publication the views and opinions expressed by the interviewees are those of the interviewees and do not necessarily reflect those of the authors, those of the NHS, the NIHR, NETSCC, the EME programme or the Department of Health and Social Care.
References
- Miyasaka Y, Barnes ME, Gersh BJ, Cha SS, Bailey KR, Abhayaratna WP, et al. Secular trends in incidence of atrial fibrillation in Olmsted County, Minnesota, 1980 to 2000, and implications on the projections for future prevalence. Circulation 2006;114:119-25. https://doi.org/10.1161/CIRCULATIONAHA.105.595140.
- Lim WY, Khan F. Management of atrial fibrillation: recommendations from NICE. Br J Hosp Med 2015;76:C108-12. https://doi.org/10.12968/hmed.2015.76.7.C108.
- Chugh SS, Havmoeller R, Narayanan K, Singh D, Rienstra M, Benjamin EJ, et al. Worldwide epidemiology of atrial fibrillation: a Global Burden of Disease 2010 Study. Circulation 2014;129:837-47. https://doi.org/10.1161/CIRCULATIONAHA.113.005119.
- Camm AJ, Lip GYH, De Caterina R, Savelieva I, Atar D, Hohnloser SH, et al. 2012 focused update of the ESC Guidelines for the management of atrial fibrillation: an update of the 2010 ESC Guidelines for the management of atrial fibrillation. Eur Heart J 2012;14:1385-413.
- Zakeri R, Van Wagoner DR, Calkins H, Wong T, Ross HM, Heist EK, et al. The burden of proof: the current state of atrial fibrillation prevention and treatment trials. Heart Rhythm 2017;14:763-82. https://doi.org/10.1016/j.hrthm.2017.01.032.
- Dorian P, Jung W, Newman D, Paquette M, Wood K, Ayers GM, et al. The impairment of health-related quality of life in patients with intermittent atrial fibrillation: implications for the assessment of investigational therapy. J Am Coll Cardiol 2000;36:1303-9. https://doi.org/10.1016/S0735-1097(00)00886-X.
- Benjamin EJ, Wolf PA, D’Agostino RB, Silbershatz H, Kannel WB, Levy D. Impact of atrial fibrillation on the risk of death: the Framingham Heart Study. Circulation 1998;98:946-52. https://doi.org/10.1161/01.cir.98.10.946.
- Camm AJ, Kirchhof P, Lip GY, Schotten U, Savelieva I, Ernst S, et al. Guidelines for the management of atrial fibrillation: the Task Force for the Management of Atrial Fibrillation of the European Society of Cardiology (ESC). Eur Heart J 2010;31:2369-429. https://doi.org/10.1093/eurheartj/ehq278.
- Senoo K, Lau YC, Lip GY. Updated NICE guideline: management of atrial fibrillation (2014). Expert Rev Cardiovasc Ther 2014;12:1037-40. https://doi.org/10.1586/14779072.2014.943189.
- Stewart S, Murphy NF, Murphy N, Walker A, McGuire A, McMurray JJ. Cost of an emerging epidemic: an economic analysis of atrial fibrillation in the UK. Heart 2004;90:286-92. https://doi.org/10.1136/hrt.2002.008748.
- Calkins H, Brugada J, Packer DL, Cappato R, Chen SA, Crijns HJ, et al. HRS/EHRA/ECAS expert consensus statement on catheter and surgical ablation of atrial fibrillation: recommendations for personnel, policy, procedures and follow-up. A report of the Heart Rhythm Society (HRS) Task Force on Catheter and Surgical Ablation of Atrial Fibrillation developed in partnership with the EHRA and the European Cardiac Arrhythmia Society (ECAS); in collaboration with the American College of Cardiology (ACC), American Heart Association (AHA), and the Society of Thoracic Surgeons (STS). Endorsed and approved by the governing bodies of the American College of Cardiology, the American Heart Association, the European Cardiac Arrhythmia Society, the European Heart Rhythm Association, the Society of Thoracic Surgeons, and the Heart Rhythm Society. Europace 2007;9:335-79. https://doi.org/10.1093/europace/eum120.
- Calkins H, Kuck KH, Cappato R, Brugada J, Camm AJ, Chen SA, et al. 2012 HRS/EHRA/ECAS Expert Consensus Statement on Catheter and Surgical Ablation of Atrial Fibrillation: recommendations for patient selection, procedural techniques, patient management and follow-up, definitions, endpoints, and research trial design. Europace 2012;14:528-606. https://doi.org/10.1093/europace/eus027.
- Haldar S, Jarman JW, Panikker S, Jones DG, Salukhe T, Gupta D, et al. Contact force sensing technology identifies sites of inadequate contact and reduces acute pulmonary vein reconnection: a prospective case control study. Int J Cardiol 2013;168:1160-6. https://doi.org/10.1016/j.ijcard.2012.11.072.
- Krittayaphong R, Raungrattanaamporn O, Bhuripanyo K, Sriratanasathavorn C, Pooranawattanakul S, Punlee K, et al. A randomized clinical trial of the efficacy of radiofrequency catheter ablation and amiodarone in the treatment of symptomatic atrial fibrillation. J Med Assoc Thai 2003;86:S8-16.
- Wazni OM, Marrouche NF, Martin DO, Verma A, Bhargava M, Saliba W, et al. Radiofrequency ablation vs antiarrhythmic drugs as first-line treatment of symptomatic atrial fibrillation: a randomized trial. JAMA 2005;293:2634-40. https://doi.org/10.1001/jama.293.21.2634.
- Wilber DJ, Pappone C, Neuzil P, De Paola A, Marchlinski F, Natale A, et al. Comparison of antiarrhythmic drug therapy and radiofrequency catheter ablation in patients with paroxysmal atrial fibrillation: a randomized controlled trial. JAMA 2010;303:333-40. https://doi.org/10.1001/jama.2009.2029.
- Pappone C, Radinovic A, Manguso F, Vicedomini G, Ciconte G, Sacchi S, et al. Atrial fibrillation progression and management: a 5-year prospective follow-up study. Heart Rhythm 2008;5:1501-7. https://doi.org/10.1016/j.hrthm.2008.08.011.
- Stabile G, Iuliano A, Agresta A, La Rocca V, D’Ascia S, De Simone A. Antiarrhythmic therapy following ablation of atrial fibrillation. Expert Rev Cardiovasc Ther 2013;11:837-42. https://doi.org/10.1586/14779072.2013.811982.
- Oral H, Knight BP, Tada H, Ozaydin M, Chugh A, Hassan S, et al. Pulmonary vein isolation for paroxysmal and persistent atrial fibrillation. Circulation 2002;105:1077-81. https://doi.org/10.1161/hc0902.104712.
- Haïssaguerre M, Shah DC, Jaïs P, Hocini M, Yamane T, Deisenhofer I, et al. Mapping-guided ablation of pulmonary veins to cure atrial fibrillation. Am J Cardiol 2000;86:9K-19K. https://doi.org/10.1016/S0002-9149(00)01186-3.
- Haïssaguerre M, Jaïs P, Shah DC, Garrigue S, Takahashi A, Lavergne T, et al. Electrophysiological end point for catheter ablation of atrial fibrillation initiated from multiple pulmonary venous foci. Circulation 2000;101:1409-17. https://doi.org/10.1161/01.cir.101.12.1409.
- Haïssaguerre M, Jaïs P, Shah DC, Arentz T, Kalusche D, Takahashi A, et al. Catheter ablation of chronic atrial fibrillation targeting the reinitiating triggers. J Cardiovasc Electrophysiol 2000;11:2-10. https://doi.org/10.1111/j.1540-8167.2000.tb00727.x.
- Verma A, Jiang CY, Betts TR, Chen J, Deisenhofer I, Mantovan R, et al. Approaches to catheter ablation for persistent atrial fibrillation. N Engl J Med 2015;372:1812-22. https://doi.org/10.1056/NEJMoa1408288.
- Mont L, Bisbal F, Hernández-Madrid A, Pérez-Castellano N, Viñolas X, Arenal A, et al. Catheter ablation vs. antiarrhythmic drug treatment of persistent atrial fibrillation: a multicentre, randomized, controlled trial (SARA study). Eur Heart J 2014;35:501-7. https://doi.org/10.1093/eurheartj/eht457.
- Jadidi AS, Lehrmann H, Keyl C, Sorrel J, Markstein V, Minners J, et al. Ablation of persistent atrial fibrillation targeting low-voltage areas with selective activation characteristics. Circ Arrhythm Electrophysiol 2016;9. https://doi.org/10.1161/CIRCEP.115.002962.
- Scherr D, Khairy P, Miyazaki S, Aurillac-Lavignolle V, Pascale P, Wilton SB, et al. Five-year outcome of catheter ablation of persistent atrial fibrillation using termination of atrial fibrillation as a procedural endpoint. Circ Arrhythm Electrophysiol 2015;8:18-24. https://doi.org/10.1161/CIRCEP.114.001943.
- Rivard L, Hocini M, Rostock T, Cauchemez B, Forclaz A, Jadidi AS, et al. Improved outcome following restoration of sinus rhythm prior to catheter ablation of persistent atrial fibrillation: a comparative multicenter study. Heart Rhythm 2012;9:1025-30. https://doi.org/10.1016/j.hrthm.2012.02.016.
- Ardashev AV, Zheliakov EG, Dupliakov DV, Konev AV, Rybachenko MS, Glukhova VL, et al. Long-term results of radiofrequency catheter ablation of long-lasting persistent atrial fibrillation: five years of follow-up. Kardiologiia 2013;53:4-11.
- Haïssaguerre M, Sanders P, Hocini M, Takahashi Y, Rotter M, Sacher F, et al. Catheter ablation of long-lasting persistent atrial fibrillation: critical structures for termination. J Cardiovasc Electrophysiol 2005;16:1125-37. https://doi.org/10.1111/j.1540-8167.2005.00307.x.
- Haldar SK, Jones DG, Bahrami T, De Souza A, Panikker S, Butcher C, et al. Catheter ablation vs. electrophysiologically guided thoracoscopic surgical ablation in long-standing persistent atrial fibrillation: the CASA-AF Study. Heart Rhythm 2017;14:1596-603. https://doi.org/10.1016/j.hrthm.2017.08.024.
- Jarman JWE, Panikker S, Das M, Wynn G, Ullah W, Kontogeorgis A, et al. Relationship between contact force sensing technology and medium-term outcome of atrial fibrillation ablation: a multicenter study of 600 patients: contact force sensing af ablation outcomes. J Cardiovasc Electrophysiol 2015;26:378-84. https://doi.org/10.1111/jce.12606.
- Fiala M, Bulková V, Škňouřil L, Nevřalová R, Toman O, Januška J, et al. Sinus rhythm restoration and arrhythmia noninducibility are major predictors of arrhythmia-free outcome after ablation for long-standing persistent atrial fibrillation: a prospective study. Heart Rhythm 2015;12:687-98. https://doi.org/10.1016/j.hrthm.2015.01.004.
- Boersma LV, Castella M, van Boven W, Berruezo A, Yilmaz A, Nadal M, et al. Atrial fibrillation catheter ablation versus surgical ablation treatment (FAST): a 2-center randomized clinical trial. Circulation 2012;125:23-30. https://doi.org/10.1161/CIRCULATIONAHA.111.074047.
- Sabashnikov A, Weymann A, Haldar S, Soliman RF, Fatullayev J, Jones D, et al. Position of totally thoracoscopic surgical ablation in the treatment of atrial fibrillation: an alternative method of conduction testing. Med Sci Monit Basic Res 2015;21:76-80. https://doi.org/10.12659/MSMBR.894239.
- Sirak JH, Schwartzman D. Interim results of the 5-box thoracoscopic maze procedure. Ann Thorac Surg 2012;94:1880-4. https://doi.org/10.1016/j.athoracsur.2012.06.010.
- Edgerton JR, Jackman WM, Mahoney C, Mack MJ. Totally thorascopic surgical ablation of persistent AF and long-standing persistent atrial fibrillation using the ‘Dallas’ lesion set. Heart Rhythm 2009;6:64-70. https://doi.org/10.1016/j.hrthm.2009.09.011.
- Chan AW, Tetzlaff JM, Altman DG, Laupacis A, Gøtzsche PC, Krle A-Jerić K, et al. SPIRIT 2013 Statement: defining standard protocol items for clinical trials. Rev Panam Salud Publica 2015;38:506-14.
- Agha RA, Altman DG, Rosin D. The SPIRIT 2013 statement – defining standard protocol items for trials. Int J Surg 2015;13:288-91. https://doi.org/10.1016/j.ijsu.2014.12.007.
- Khan HR, Kralj-Hans I, Haldar S, Bahrami T, Clague J, De Souza A, et al. Catheter ablation versus thoracoscopic surgical ablation in long standing persistent atrial fibrillation (CASA-AF): study protocol for a randomised controlled trial. Trials 2018;19. https://doi.org/10.1186/s13063-018-2487-9.
- Spertus J, Dorian P, Bubien R, Lewis S, Godejohn D, Reynolds MR, et al. Development and validation of the Atrial Fibrillation Effect on QualiTy-of-Life (AFEQT) questionnaire in patients with atrial fibrillation. Circ Arrhythm Electrophysiol 2011;4:15-2. https://doi.org/10.1161/CIRCEP.110.958033.
- Kirchhof P, Auricchio A, Bax J, Crijns H, Camm J, Diener HC, et al. Outcome parameters for trials in atrial fibrillation: recommendations from a consensus conference organized by the German Atrial Fibrillation Competence NETwork and the European Heart Rhythm Association. Europace 2007;9:1006-23. https://doi.org/10.1093/europace/eum191.
- Heidbuchel H, Verhamme P, Alings M, Antz M, Diener HC, Hacke W, et al. Updated European Heart Rhythm Association Practical Guide on the use of non-vitamin K antagonist anticoagulants in patients with non-valvular atrial fibrillation. Europace 2015;17:1467-507. https://doi.org/10.1093/europace/euv309.
- Keeling D, Baglin T, Tait C, Watson H, Perry D, Baglin C, et al. Guidelines on oral anticoagulation with warfarin – fourth edition: guideline. Br J Haematol 2011;154:311-24. https://doi.org/10.1111/j.1365-2141.2011.08753.x.
- Verma A, Cairns JA, Mitchell LB, Macle L, Stiell IG, Gladstone D, et al. 2014 focused update of the Canadian Cardiovascular Society Guidelines for the management of atrial fibrillation. Can J Cardiol 2014;30:1114-30. https://doi.org/10.1016/j.cjca.2014.08.001.
- Santarpia G, Curcio A, Sibilio G, Indolfi C. Clinical significance of non-vitamin K antagonist oral anticoagulants in the management of atrial fibrillation. Circ J 2015;79:914-23. https://doi.org/10.1253/circj.CJ-15-0319.
- Yilmaz A, Geuzebroek GS, Van Putte BP, Boersma LV, Sonker U, De Bakker JM, et al. Completely thoracoscopic pulmonary vein isolation with ganglionic plexus ablation and left atrial appendage amputation for treatment of atrial fibrillation. Eur J Cardiothorac Surg 2010;38:356-60. https://doi.org/10.1016/j.ejcts.2010.01.058.
- Krul SP, Driessen AH, Wilde AA, de Bakker JM, de Mol BA, de Groot JR. Thoracoscopic treatment of atrial fibrillation. Ned Tijdschr Geneeskd 2012;156.
- Gelsomino S, Van Breugel HN, Pison L, Parise O, Crijns HJ, Wellens F, et al. Hybrid thoracoscopic and transvenous catheter ablation of atrial fibrillation. Eur J Cardiothorac Surg 2014;45:401-7. https://doi.org/10.1093/ejcts/ezt385.
- de Groot JR, Driessen AH, Van Boven WJ, Krul SP, Linnenbank AC, Jackman WM, et al. Epicardial confirmation of conduction block during thoracoscopic surgery for atrial fibrillation – a hybrid surgical-electrophysiological approach. Minim Invasive Ther Allied Technol 2012;21:293-301. https://doi.org/10.3109/13645706.2011.615329.
- Betts TR, Leo M, Panikker S, Kanagaratnam P, Koa-Wing M, Davies DW, et al. Percutaneous left atrial appendage occlusion using different technologies in the United Kingdom: a multicenter registry. Catheter Cardiovasc Interv 2017;89:484-92. https://doi.org/10.1002/ccd.26782.
- Panikker S, Jarman JW, Virmani R, Kutys R, Haldar S, Lim E, et al. Left atrial appendage electrical isolation and concomitant device occlusion to treat persistent atrial fibrillation: a first-in-human safety, feasibility, and efficacy study. Circ Arrhythm Electrophysiol 2016;9. https://doi.org/10.1161/CIRCEP.115.003710.
- Panikker S, Virmani R, Sakakura K, Kolodgie F, Francis DP, Markides V, et al. Left atrial appendage electrical isolation and concomitant device occlusion: a safety and feasibility study with histologic characterization. Heart Rhythm 2015;12:202-10. https://doi.org/10.1016/j.hrthm.2014.09.010.
- Panikker S, Lord J, Jarman JW, Armstrong S, Jones DG, Haldar S, et al. Outcomes and costs of left atrial appendage closure from randomized controlled trial and real-world experience relative to oral anticoagulation. Eur Heart J 2016;37:3470-82. https://doi.org/10.1093/eurheartj/ehw048.
- Jones DG, Haldar SK, Jarman JW, Johar S, Hussain W, Markides V, et al. Impact of stepwise ablation on the biatrial substrate in patients with persistent atrial fibrillation and heart failure. Circ Arrhythm Electrophysiol 2013;6:761-8. https://doi.org/10.1161/CIRCEP.113.000390.
- Sanna T, Diener HC, Passman RS, Di Lazzaro V, Bernstein RA, Morillo CA, et al. Cryptogenic stroke and underlying atrial fibrillation. N Engl J Med 2014;370:2478-86. https://doi.org/10.1056/NEJMoa1313600.
- Maines M, Zorzi A, Tomasi G, Angheben C, Catanzariti D, Piffer L, et al. Clinical impact, safety, and accuracy of the remotely monitored implantable loop recorder Medtronic Reveal LINQTM. Europace 2018;20:1050-7. https://doi.org/10.1093/europace/eux187.
- Sanders P, Pürerfellner H, Pokushalov E, Sarkar S, Di Bacco M, Maus B, et al. Performance of a new atrial fibrillation detection algorithm in a miniaturized insertable cardiac monitor: results from the Reveal LINQ Usability Study. Heart Rhythm 2016;13:1425-30. https://doi.org/10.1016/j.hrthm.2016.03.005.
- Giancaterino S, Lupercio F, Nishimura M, Hsu JC. Current and future use of insertable cardiac monitors. JACC Clin Electrophysiol 2018;4:1383-96. https://doi.org/10.1016/j.jacep.2018.06.001.
- Lee R, Mittal S. Utility and limitations of long-term monitoring of atrial fibrillation using an implantable loop recorder. Heart Rhythm 2018;15:287-95. https://doi.org/10.1016/j.hrthm.2017.09.009.
- Aragon T. Epidemiology Tools n.d. https://Cran.r-Project.Org/Web/Packages/Epitools/Epitools.Pdf.
- van Buuren S, Groothuis-Oudshoorn K. mice: multivariate imputation by chained equations in R. J Stat Softw 2011;45. https://doi.org/10.18637/jss.v045.i03.
- Donoghoe MW, Marschner IC. logbin: an R package for relative risk regression using the log-binomial model. J Stat Softw 2018;86. https://doi.org/10.18637/jss.v086.i09.
- Husereau D, Drummond M, Petrou S, Carswell C, Moher D, Greenberg D, et al. Consolidated Health Economic Evaluation Reporting Standards (CHEERS) statement. Value Health 2013;16:e1-5. https://doi.org/10.1016/j.jval.2013.02.010.
- National Institute for Health and Care Excellence (NICE) . Position Statement on Use of the EQ-5D-5L Value Set for England 2019 n.d. www.nice.org.uk/about/what-we-do/our-programmes/nice-guidance/technology-appraisal-guidance/eq-5d-5l (accessed March 2020).
- Dolan P, Gudex C, Kind P, Williams A. A Social Tariff for EuroQol: Results from a UK General Population Survey. York: Centre for Health Economics, University of York; 1995.
- van Hout B, Janssen MF, Feng YS, Kohlmann T, Busschbach J, Golicki D, et al. Interim scoring for the EQ-5D-5L: mapping the EQ-5D-5L to EQ-5D-3L value sets. Value Health 2012;15:708-15. https://doi.org/10.1016/j.jval.2012.02.008.
- National Institute for Health and Care Excellence (NICE) . Guide to the Methods of Technology Appraisal 2013 2013. www.nice.org.uk/process/pmg9/resources/guide-to-the-methods-of-technology-appraisal-2013-pdf-2007975843781 (accessed March 2020).
- NHS Improvement . National Schedule of NHS Costs n.d. www.england.nhs.uk/national-cost-collection/ (accessed March 2020).
- Curtis LA, Burns A. Unit Costs of Health and Social Care 2019. Canterbury: Personal Social Services Research Unit, University of Kent; 2019.
- Monthly Index of Medical Specialities (MIMS) . Monthly Index of Medical Specialities (MIMS) Online 2020 n.d. www.mims.co.uk/ (accessed March 2020).
- Burton A, Billingham LJ, Bryan S. Cost-effectiveness in clinical trials: using multiple imputation to deal with incomplete cost data. Clin Trials 2007;4:154-61. https://doi.org/10.1177/1740774507076914.
- Manca A, Palmer S. Handling missing data in patient-level cost-effectiveness analysis alongside randomised clinical trials. Appl Health Econ Health Policy 2005;4:65-7. https://doi.org/10.2165/00148365-200504020-00001.
- MacNeil Vroomen J, Eekhout I, Dijkgraaf MG, van Hout H, de Rooij SE, Heymand MW, et al. Multiple imputation strategies for zero-inflated cost data in economic evaluations: which method works best?. Eur J Health Econ 2016;17:939-50. https://doi.org/10.1007/s10198-015-0734-5.
- Manca A, Hawkins N, Sculpher MJ. Estimating mean QALYs in trial-based cost-effectiveness analysis: the importance of controlling for baseline utility. Health Econ 2005;14:487-96. https://doi.org/10.1002/hec.944.
- Qureshi W, Soliman EZ, Solomon SD, Alonso A, Arking DE, Shah A, et al. Risk factors for atrial fibrillation in patients with normal versus dilated left atrium (from the Atherosclerosis Risk in Communities Study). Am J Cardiol 2014;114:1368-72. https://doi.org/10.1016/j.amjcard.2014.07.073.
- Katoh Y, Nakazato Y. Can we predict electroanatomical remodelling of left atrium in patients with non-valvular atrial fibrillation by transforming growth factor-β and tissue inhibitor of metalloproteinase-1?. Circ J 2011;75:536-7. https://doi.org/10.1253/circj.CJ-11-0071.
- Grieco D, Palamà Z, Borrelli A, De Ruvo E, Sciarra L, Scarà A, et al. Diabetes mellitus and atrial remodelling in patients with paroxysmal atrial fibrillation: role of electroanatomical mapping and catheter ablation. Diab Vasc Dis Res 2018;15:185-95. https://doi.org/10.1177/1479164117752492.
- Bonapace S, Valbusa F, Bertolini L, Zenari L, Canali G, Molon G, et al. Early impairment in left ventricular longitudinal systolic function is associated with an increased risk of incident atrial fibrillation in patients with type 2 diabetes. J Diabetes Complications 2017;31:413-18. https://doi.org/10.1016/j.jdiacomp.2016.10.032.
- Diouf I, Magliano DJ, Carrington MJ, Stewart S, Shaw JE. Prevalence, incidence, risk factors and treatment of atrial fibrillation in Australia: the Australian Diabetes, Obesity and Lifestyle (AusDiab) longitudinal, population cohort study. Int J Cardiol 2016;205:127-32. https://doi.org/10.1016/j.ijcard.2015.12.013.
- Asghar O, Alam U, Hayat SA, Aghamohammadzadeh R, Heagerty AM, Malik RA. Obesity, diabetes and atrial fibrillation; epidemiology, mechanisms and interventions. Curr Cardiol Rev 2012;8:253-64. https://doi.org/10.2174/157340312803760749.
- Chao TF, Suenari K, Chang SL, Lin YJ, Lo LW, Hu YF, et al. Atrial substrate properties and outcome of catheter ablation in patients with paroxysmal atrial fibrillation associated with diabetes mellitus or impaired fasting glucose. Am J Cardiol 2010;106:1615-20. https://doi.org/10.1016/j.amjcard.2010.07.038.
- Nichols GA, Reinier K, Chugh SS. Independent contribution of diabetes to increased prevalence and incidence of atrial fibrillation. Diabetes Care 2009;32:1851-6. https://doi.org/10.2337/dc09-0939.
- Rietbrock S, Heeley E, Plumb J, van Staa T. Chronic atrial fibrillation: incidence, prevalence, and prediction of stroke using the Congestive heart failure, Hypertension, Age >75, Diabetes mellitus, and prior Stroke or transient ischemic attack (CHADS2) risk stratification scheme. Am Heart J 2008;156:57-64. https://doi.org/10.1016/j.ahj.2008.03.010.
- Liu T, Li G. Inflammation: another potential mechanism between diabetes mellitus and atrial fibrillation. Am J Cardiol 2008;101:1681-2. https://doi.org/10.1016/j.amjcard.2008.04.006.
- Tanaka N, Takigiku K, Takahashi K, Kuraoka A, Matsui K, Iwashima S, et al. Assessment of the developmental change in the left atrial volume using real time three-dimensional echocardiography. Echocardiography 2015;32:1131-9. https://doi.org/10.1111/echo.12829.
- Ma XX, Boldt LH, Zhang YL, Zhu MR, Hu B, Parwani A, et al. Clinical relevance of left atrial strain to predict recurrence of atrial fibrillation after catheter ablation: a meta-analysis. Echocardiography 2016;33:724-33. https://doi.org/10.1111/echo.13184.
- Sarvari SI, Haugaa KH, Stokke TM, Ansari HZ, Leren IS, Hegbom F, et al. Strain echocardiographic assessment of left atrial function predicts recurrence of atrial fibrillation. Eur Heart J Cardiovasc Imaging 2016;17:660-7. https://doi.org/10.1093/ehjci/jev185.
- Hesse B, Schuele SU, Thamilasaran M, Thomas J, Rodriguez L. A rapid method to quantify left atrial contractile function: Doppler tissue imaging of the mitral annulus during atrial systole. Eur J Echocardiogr 2004;5:86-92. https://doi.org/10.1016/s1525-2167(03)00046-5.
- Calkins H, Hindricks G, Cappato R, Kim YH, Saad EB, Aguinaga L, et al. 2017 HRS/EHRA/ECAS/APHRS/SOLAECE expert consensus statement on catheter and surgical ablation of atrial fibrillation. Europace 2018;20:e1-e160. https://doi.org/10.1093/europace/eux274.
- Calkins H, Hindricks G, Cappato R, Kim YH, Saad EB, Aguinaga L, et al. 2017 HRS/EHRA/ECAS/APHRS/SOLAECE expert consensus statement on catheter and surgical ablation of atrial fibrillation. Heart Rhythm 2017;14:e275-e444.
- Casaclang-Verzosa G, Gersh BJ, Tsang TSM. Structural and functional remodeling of the left atrium. J Am Coll Cardiol 2008;51:1-11. https://doi.org/10.1016/j.jacc.2007.09.026.
- Mori M, Kanzaki H, Amaki M, Ohara T, Hasegawa T, Takahama H, et al. Impact of reduced left atrial functions on diagnosis of paroxysmal atrial fibrillation: results from analysis of time-left atrial volume curve determined by two-dimensional speckle tracking. J Cardiol 2011;57:89-94. https://doi.org/10.1016/j.jjcc.2010.08.006.
- Habibi M, Lima JA, Khurram IM, Zimmerman SL, Zipunnikov V, Fukumoto K, et al. Association of left atrial function and left atrial enhancement in patients with atrial fibrillation: cardiac magnetic resonance study. Circ Cardiovasc Imaging 2015;8. https://doi.org/10.1161/CIRCIMAGING.114.002769.
- Gupta DK, Shah AM, Giugliano RP, Ruff CT, Antman EM, Grip LT, et al. Left atrial structure and function in atrial fibrillation: ENGAGE AF-TIMI 48. Eur Heart J 2014;35:1457-65. https://doi.org/10.1093/eurheartj/eht500.
- Donal E, Ollivier R, Veillard D, Hamonic S, Pavin D, Daubert JC, et al. Left atrial function assessed by trans-thoracic echocardiography in patients treated by ablation for a lone paroxysmal atrial fibrillation. Eur J Echocardiogr 2010;11:845-52. https://doi.org/10.1093/ejechocard/jeq074.
- Ma XX, Zhang YL, Hu B, Zhu MR, Jiang WJ, Wang M, et al. The usefulness of global left atrial strain for predicting atrial fibrillation recurrence after catheter ablation in patients with persistent and paroxysmal atrial fibrillation. Arch Cardiovasc Dis 2017;110:447-55. https://doi.org/10.1016/j.acvd.2016.11.005.
- Moreno-Ruiz LA, Madrid-Miller A, Martínez-Flores JE, González-Hermosillo JA, Arenas-Fonseca J, Zamorano-Velázquez N, et al. Left atrial longitudinal strain by speckle tracking as independent predictor of recurrence after electrical cardioversion in persistent and long standing persistent non-valvular atrial fibrillation. Int J Cardiovasc Imaging 2019;35:1587-96. https://doi.org/10.1007/s10554-019-01597-7.
- Parwani AS, Morris DA, Blaschke F, Huemer M, Pieske B, Haverkamp W, et al. Left atrial strain predicts recurrence of atrial arrhythmias after catheter ablation of persistent atrial fibrillation. Open Heart 2017;4. https://doi.org/10.1136/openhrt-2016-000572.
- Thomas L, Abhayaratna WP. Left atrial reverse remodeling. JACC Cardiovasc Imaging 2017;10:65-77. https://doi.org/10.1016/j.jcmg.2016.11.003.
- Njoku A, Kannabhiran M, Arora R, Reddy P, Gopinathannair R, Lakkireddy D, et al. Left atrial volume predicts atrial fibrillation recurrence after radiofrequency ablation: a meta-analysis. Europace 2018;20:33-42. https://doi.org/10.1093/europace/eux013.
- Prabhu S, McLellan AJ, Walters TE, Sharma M, Voskoboinik A, Kistler PM. Atrial structure and function and its implications for current and emerging treatments for atrial fibrillation. Prog Cardiovasc Dis 2015;58:152-67. https://doi.org/10.1016/j.pcad.2015.08.004.
- Nührich JM, Geisler AC, Steven D, Hoffmann BA, Schäffer B, Lund G, et al. Active atrial function and atrial scar burden after multiple catheter ablations of persistent atrial fibrillation. Pacing Clin Electrophysiol 2017;40:175-82. https://doi.org/10.1111/pace.13004.
- Mochizuki A, Yuda S, Fujito T, Kawamukai M, Muranaka A, Nagahara D, et al. Left atrial strain assessed by three-dimensional speckle tracking echocardiography predicts atrial fibrillation recurrence after catheter ablation in patients with paroxysmal atrial fibrillation. J Echocardiogr 2017;15:79-87. https://doi.org/10.1007/s12574-017-0329-5.
- Tsai WC, Lee CH, Lin CC, Liu YW, Huang YY, Li WT, et al. Association of left atrial strain and strain rate assessed by speckle tracking echocardiography with paroxysmal atrial fibrillation. Echocardiography 2009;26:1188-94. https://doi.org/10.1111/j.1540-8175.2009.00954.x.
- Costa C, González-Alujas T, Valente F, Aranda C, Rodríguez-Palomares J, Gutierrez L, et al. Left atrial strain: a new predictor of thrombotic risk and successful electrical cardioversion. Echo Res Pract 2016;3:45-52. https://doi.org/10.1530/ERP-16-0009.
- Shaikh AY, Maan A, Khan UA, Aurigemma GP, Hill JC, Kane JL, et al. Speckle echocardiographic left atrial strain and stiffness index as predictors of maintenance of sinus rhythm after cardioversion for atrial fibrillation: a prospective study. Cardiovasc Ultrasound 2012;10. https://doi.org/10.1186/1476-7120-10-48.
- Wang T, Wang M, Fung JW, Yip GW, Zhang Y, Ho PP, et al. Atrial strain rate echocardiography can predict success or failure of cardioversion for atrial fibrillation: a combined transthoracic tissue Doppler and transoesophageal imaging study. Int J Cardiol 2007;114:202-9. https://doi.org/10.1016/j.ijcard.2006.01.051.
- Thomas L, McKay T, Byth K, Marwick TH. Abnormalities of left atrial function after cardioversion: an atrial strain rate study. Heart 2007;93:89-95. https://doi.org/10.1136/hrt.2006.088609.
- Hoit BD. Left atrial size and function. J Am Coll Cardiol 2014;63:493-505. https://doi.org/10.1016/j.jacc.2013.10.055.
- Boyd A, Schiller N, Ross D, Thomas L. Segmental atrial contraction in patients restored to sinus rhythm after cardioversion for chronic atrial fibrillation: a colour Doppler tissue imaging study. Eur J Echocardiogr 2008;9:12-7. https://doi.org/10.1016/j.euje.2006.11.004.
- Kerut EK, McKinnie J, Reilly JP, Hanawalt C, Everson CT. Doppler evidence of persistent left atrial mechanical standstill with normal P-waves by electrocardiogram after biatrial CryoMaze procedure for atrial fibrillation: Doppler of isolated left atrial standstill. Echocardiography 2011;28:809-11. https://doi.org/10.1111/j.1540-8175.2011.01435.x.
- Buber J, Luria D, Sternik L, Raanani E, Feinberg MS, Goldenberg I, et al. Left atrial contractile function following a successful modified Maze procedure at surgery and the risk for subsequent thromboembolic stroke. J Am Coll Cardiol 2011;58:1614-21. https://doi.org/10.1016/j.jacc.2011.05.051.
- Agner BF, Kühl JT, Linde JJ, Kofoed KF, Åkeson P, Rasmussen BV, et al. Assessment of left atrial volume and function in patients with permanent atrial fibrillation: comparison of cardiac magnetic resonance imaging, 320-slice multi-detector computed tomography, and transthoracic echocardiography. Eur Heart J Cardiovasc Imaging 2014;15:532-40. https://doi.org/10.1093/ehjci/jet239.
- Otani K, Nakazono A, Salgo IS, Lang RM, Takeuchi M. Three-dimensional echocardiographic assessment of left heart chamber size and function with fully automated quantification software in patients with atrial fibrillation. J Am Soc Echocardiogr 2016;29:955-65. https://doi.org/10.1016/j.echo.2016.06.010.
- Shang Z, Su D, Cong T, Sun Y, Liu Y, Chen N, et al. Assessment of left atrial mechanical function and synchrony in paroxysmal atrial fibrillation with two-dimensional speckle tracking echocardiography. Echocardiography 2017;34:176-83. https://doi.org/10.1111/echo.13434.
- Wałek P, Sielski J, Starzyk K, Gorczyca I, Roskal-Wałek J, Wożakowska-Kapłon B. Echocardiographic assessment of left atrial morphology and function to predict maintenance of sinus rhythm after electrical cardioversion in patients with non-valvular persistent atrial fibrillation and normal function or mild dysfunction of left ventricle. Cardiol J 2020;27:246-53. https://doi.org/10.5603/CJ.a2019.0068.
- Imanishi J, Tanaka H, Sawa T, Motoji Y, Miyoshi T, Mochizuki Y, et al. Left atrial booster-pump function as a predictive parameter for new-onset postoperative atrial fibrillation in patients with severe aortic stenosis. Int J Cardiovasc Imaging 2014;30:295-304. https://doi.org/10.1007/s10554-013-0346-z.
- Nappo R, Degiovanni A, Bolzani V, Sartori C, Di Giovine G, Cerini P, et al. Quantitative assessment of atrial conduit function: a new index of diastolic dysfunction. Clin Res Cardiol 2016;105:17-28. https://doi.org/10.1007/s00392-015-0882-8.
- Abhayaratna WP, Fatema K, Barnes ME, Seward JB, Gersh BJ, Bailey KR, et al. Left atrial reservoir function as a potent marker for first atrial fibrillation or flutter in persons ≥ 65 years of age. Am J Cardiol 2008;101:1626-9. https://doi.org/10.1016/j.amjcard.2008.01.051.
- Dons M, BieringSørensen T, Jensen JS, Fritz-Hansen T, Bech J, de Knegt MC, et al. Systolic and diastolic function by tissue doppler imaging predicts mortality in patients with atrial fibrillation. J Atr Fibrillation 2015;8. https://doi.org/10.4022/jafib.1241.
- Hsiao SH, Lin KL, Chiou KR. Comparison of left atrial volume parameters in detecting left ventricular diastolic dysfunction versus tissue Doppler recordings. Am J Cardiol 2012;109:748-55. https://doi.org/10.1016/j.amjcard.2011.10.040.
- Löbe S, Knopp H, Le TV, Nedios S, Seewöster T, Bode K, et al. Left atrial asynchrony measured by pulsed-wave tissue doppler is associated with abnormal atrial voltage and recurrences of atrial fibrillation after catheter ablation. JACC Clin Electrophysiol 2018;4:1640-1. https://doi.org/10.1016/j.jacep.2018.08.017.
- Salah A, Yang H, Tang L, Li X, Liu Q, Zhou S. Left atrial dyssynchrony time measured by tissue Doppler imaging to predict atrial fibrillation recurrences after pulmonary vein isolation. Anatol J Cardiol 2015;15:115-22. https://doi.org/10.5152/akd.2014.5217.
- Müller P, Schiedat F, Bialek A, Bösche L, Ewers A, Kara K, et al. Total atrial conduction time assessed by tissue doppler imaging (PA-TDI Interval) to predict early recurrence of persistent atrial fibrillation after successful electrical cardioversion. J Cardiovasc Electrophysiol 2014;25:161-7. https://doi.org/10.1111/jce.12306.
- Özlü MF, Erdem K, Kırış G, Parlar Aİ, Demirhan A, Ayhan SS, et al. Predictive value of total atrial conduction time measured with tissue Doppler imaging for postoperative atrial fibrillation after coronary artery bypass surgery. J Interv Card Electrophysiol 2013;37:27-33. https://doi.org/10.1007/s10840-012-9756-4.
- Müller P, Hars C, Schiedat F, Bösche LI, Gotzmann M, Strauch J. Correlation between total atrial conduction time estimated via tissue Doppler imaging (PA-TDI Interval), structural atrial remodeling and new-onset of atrial fibrillation after cardiac surgery: the role of total atrial conduction time in patients undergoing cardiac surgery. J Cardiovasc Electrophysiol 2013;24:626-31. https://doi.org/10.1111/jce.12084.
- De Boeck BW, Meine M, Leenders GE, Teske AJ, van Wessel H, Kirkels JH, et al. Practical and conceptual limitations of tissue Doppler imaging to predict reverse remodelling in cardiac resynchronisation therapy. Eur J Heart Fail 2008;10:281-90. https://doi.org/10.1016/j.ejheart.2008.02.003.
- Skaarup KG, Christensen H, Høst N, Mahmoud MM, Ovesen C, Olsen FJ, et al. Usefulness of left ventricular speckle tracking echocardiography and novel measures of left atrial structure and function in diagnosing paroxysmal atrial fibrillation in ischemic stroke and transient ischemic attack patients. Int J Cardiovasc Imaging 2017;33:1921-9. https://doi.org/10.1007/s10554-017-1204-1.
- Mares RG, Nistor DO, Golu MV. Usefulness of left atrial speckle-tracking echocardiography in patients with atrial fibrillation. Anatol J Cardiol 2017;18:377-8. https://doi.org/10.14744/AnatolJCardiol.2017.8070.
- Rimbaş RC, Mihăilă S, Vinereanu D. Sources of variation in assessing left atrial functions by 2D speckle-tracking echocardiography. Heart Vessels 2016;31:370-81. https://doi.org/10.1007/s00380-014-0602-8.
- Pathan F, D’Elia N, Nolan MT, Marwick TH, Negishi K. Normal ranges of left atrial strain by speckle-tracking echocardiography: a systematic review and meta-analysis. J Am Soc Echocardiogr 2017;30:59-70.e8. https://doi.org/10.1016/j.echo.2016.09.007.
- Inaba Y, Yuda S, Kobayashi N, Hashimoto A, Uno K, Nakata T, et al. Strain rate imaging for noninvasive functional quantification of the left atrium: comparative studies in controls and patients with atrial fibrillation. J Am Soc Echocardiogr 2005;18:729-36. https://doi.org/10.1016/j.echo.2004.12.005.
- Hwang HJ, Choi EY, Rhee SJ, Joung B, Lee BH, Lee SH, et al. Left atrial strain as predictor of successful outcomes in catheter ablation for atrial fibrillation: a two-dimensional myocardial imaging study. J Interv Card Electrophysiol 2009;26:127-32. https://doi.org/10.1007/s10840-009-9410-y.
- Her AY, Kim JY, Kim YH, Choi EY, Min PK, Yoon YW, et al. Left atrial strain assessed by speckle tracking imaging is related to new-onset atrial fibrillation after coronary artery bypass grafting. Can J Cardiol 2013;29:377-83. https://doi.org/10.1016/j.cjca.2012.06.006.
- Candan O, Ozdemir N, Aung SM, Dogan C, Karabay CY, Gecmen C, et al. Left atrial longitudinal strain parameters predict postoperative persistent atrial fibrillation following mitral valve surgery: a speckle tracking echocardiography study. Echocardiography 2013;30:1061-8. https://doi.org/10.1111/echo.12222.
- Candan O, Ozdemir N, Aung SM, Hatipoglu S, Karabay CY, Guler A, et al. Atrial longitudinal strain parameters predict left atrial reverse remodeling after mitral valve surgery: a speckle tracking echocardiography study. Int J Cardiovasc Imaging 2014;30:1049-56. https://doi.org/10.1007/s10554-014-0433-9.
- Peters DC, Duncan JS, Grunseich K, Marieb MA, Cornfeld D, Sinusas AJ, et al. CMR-verified lower LA strain in the presence of regional atrial fibrosis in atrial fibrillation. JACC Cardiovasc Imaging 2017;10:207-8. https://doi.org/10.1016/j.jcmg.2016.01.015.
- Paraskevaidis IA, Vartela V, Tsiapras D, Iliodromitis EK, Parissis J, Farmakis D, et al. Tissue Doppler imaging analysis at pre-cardioversion time predicts recurrent atrial fibrillation: a 12-month follow-up study. J Cardiovasc Electrophysiol 2006;17:1005-10. https://doi.org/10.1111/j.1540-8167.2006.00566.x.
- den Uijl DW, Gawrysiak M, Tops LF, Trines SA, Zeppenfeld K, Schalij MJ, et al. Prognostic value of total atrial conduction time estimated with tissue Doppler imaging to predict the recurrence of atrial fibrillation after radiofrequency catheter ablation. Europace 2011;13:1533-40. https://doi.org/10.1093/europace/eur186.
- Wharton G, Steeds R, Allen J, Phillips H, Jones R, Kanagala P, et al. A minimum dataset for a standard adult transthoracic echocardiogram: a guideline protocol from the British Society of Echocardiography. Echo Res Pract 2015;2:G9-G24. https://doi.org/10.1530/ERP-14-0079.
- Takarada K, Ströker E, de Asmundis C, Sieira J, Abugattas JP, Coutiño HE, et al. Second-generation cryoballoon ablation for atrial fibrillation – a detailed analysis of the impact of left atrial volume index on clinical outcome. Circ J 2018;83:84-90. https://doi.org/10.1253/circj.CJ-18-0650.
- Bisbal F, Guiu E, Calvo N, Marin D, Berruezo A, Arbelo E, et al. Left atrial sphericity: a new method to assess atrial remodeling. Impact on the outcome of atrial fibrillation ablation: left atrial sphericity predicts af ablation outcome. J Cardiovasc Electrophysiol 2013;24:752-9. https://doi.org/10.1111/jce.12116.
- Moon J, Lee H-J, Yu J, Pak HN, Ha JW, Lee MH, et al. Prognostic implication of left atrial sphericity in atrial fibrillation patients undergoing radiofrequency catheter ablation. Pacing Clin Electrophysiol 2017;40:713-20. https://doi.org/10.1111/pace.13088.
- Osmanagic A, Möller S, Osmanagic A, Sheta HM, Vinther KH, Egstrup K. Left atrial sphericity index predicts early recurrence of atrial fibrillation after direct-current cardioversion: an echocardiographic study. Clin Cardiol 2016;39:406-12. https://doi.org/10.1002/clc.22545.
- Mulder MJ, Kemme MJB, Visser CL, Hopman LHGA, van Diemen PA, van de Ven PM, et al. Left atrial sphericity as a marker of atrial remodeling: comparison of atrial fibrillation patients and controls. Int J Cardiol 2020;304:69-74. https://doi.org/10.1016/j.ijcard.2020.01.042.
- Yuasa T, Imoto Y. Usefulness of tissue doppler imaging-derived atrial conduction time for prediction of atrial fibrillation. Circ J 2016;80:58-9. https://doi.org/10.1253/circj.CJ-15-1256.
- Hori Y, Nakahara S, Anjo N, Nakagawa A, Nishiyama N, Yamada K, et al. Investigation of the atrial conduction time measured by tissue Doppler imaging at the left atrial appendage and the actual electrical conduction time: consideration of left atrial remodeling in atrial fibrillation patients. J Interv Card Electrophysiol 2017;48:89-97. https://doi.org/10.1007/s10840-016-0185-7.
- Takahashi S, Katayama K, Watanabe M, Kodama H, Taguchi T, Kurosaki T, et al. Preoperative tissue Doppler imaging-derived atrial conduction time predicts postoperative atrial fibrillation in patients undergoing mitral valve surgery for mitral valve regurgitation. Circ J 2016;80:101-9. https://doi.org/10.1253/circj.CJ-15-0796.
- Omi W, Nagai H, Takamura M, Okura S, Okajima M, Furusho H, et al. Doppler tissue analysis of atrial electromechanical coupling in paroxysmal atrial fibrillation. J Am Soc Echocardiogr 2005;18:39-44. https://doi.org/10.1016/j.echo.2004.08.029.
- Tops LF, van der Wall EE, Schalij MJ, Bax JJ. Multi-modality imaging to assess left atrial size, anatomy and function. Heart 2007;93:1461-70. https://doi.org/10.1136/hrt.2007.116467.
- Henriksen E, Selmeryd J, Leppert J, Hedberg P. Echocardiographic assessment of maximum and minimum left atrial volumes: a population-based study of middle-aged and older subjects without apparent cardiovascular disease. Int J Cardiovasc Imaging 2015;31:57-64. https://doi.org/10.1007/s10554-014-0533-6.
- Kohári M, Zado E, Marchlinski FE, Callans DJ, Han Y. Left atrial volume best predicts recurrence after catheter ablation in patients with persistent and longstanding persistent atrial fibrillation. Pacing Clin Electrophysiol 2014;37:422-9. https://doi.org/10.1111/pace.12279.
- Shin SH, Park MY, Oh WJ, Hong SJ, Pak HN, Song WH, et al. Left atrial volume is a predictor of atrial fibrillation recurrence after catheter ablation. J Am Soc Echocardiogr 2008;21:697-702. https://doi.org/10.1016/j.echo.2007.10.022.
- Yoshida N, Okamoto M, Nanba K, Yoshizumi M. Transthoracic tissue Doppler assessment of left atrial appendage contraction and relaxation: their changes with aging: left atrial appendage wall velocity. Echocardiography 2010;27:839-46. https://doi.org/10.1111/j.1540-8175.2010.01157.x.
- Xiong B, Li D, Wang J, Gyawali L, Jing J, Su L. The effect of catheter ablation on left atrial size and function for patients with atrial fibrillation: an updated meta-analysis. PLOS ONE 2015;10. https://doi.org/10.1371/journal.pone.0129274.
- Limantoro I, de Vos CB, Delhaas T, Marcos E, Blaauw Y, Weijs B, et al. Tissue velocity imaging of the left atrium predicts response to flecainide in patients with acute atrial fibrillation. Heart Rhythm 2014;11:478-84. https://doi.org/10.1016/j.hrthm.2013.12.010.
- Limantoro I, de Vos CB, Delhaas T, Weijs B, Blaauw Y, Schotten U, et al. Clinical correlates of echocardiographic tissue velocity imaging abnormalities of the left atrial wall during atrial fibrillation. Europace 2014;16:1546-53. https://doi.org/10.1093/europace/euu047.
- Su HM, Lin TH, Hsu PC, Lee WH, Chu CY, Lee CS, et al. Global left ventricular longitudinal systolic strain as a major predictor of cardiovascular events in patients with atrial fibrillation. Heart 2013;99:1588-96. https://doi.org/10.1136/heartjnl-2013-304561.
- Wang Z, Tan H, Zhong M, Jiang G, Zhang Y, Zhang W. Strain rate imaging for noninvasive functional quantification of the left atrium in hypertensive patients with paroxysmal atrial fibrillation. Cardiology 2008;109:15-24. https://doi.org/10.1159/000105322.
- Cho GY, Jo SH, Kim MK, Kim HS, Park WJ, Choi YJ, et al. Left atrial dyssynchrony assessed by strain imaging in predicting future development of atrial fibrillation in patients with heart failure. Int J Cardiol 2009;134:336-41. https://doi.org/10.1016/j.ijcard.2008.08.019.
- O’Neill MD, Wright M, Knecht S, Jaïs P, Hocini M, Takahashi Y, et al. Long-term follow-up of persistent atrial fibrillation ablation using termination as a procedural endpoint. Eur Heart J 2009;30:1105-12. https://doi.org/10.1093/eurheartj/ehp063.
- Thomas L, Thomas SP, Hoy M, Boyd A, Schiller NB, Ross DL. Comparison of left atrial volume and function after linear ablation and after cardioversion for chronic atrial fibrillation. Am J Cardiol 2004;93:165-70. https://doi.org/10.1016/j.amjcard.2003.09.033.
- De Maat GE, Benussi S, Hummel YM, Krul S, Pozzoli A, Driessen AHG, et al. Surgical left atrial appendage exclusion does not impair left atrial contraction function: a pilot study. BioMed Res Int 2015;2015. https://doi.org/10.1155/2015/318901.
- Madueme PC, Mazur W, Hor KN, Germann JT, Jefferies JL, Taylor MD. Comparison of area–length method by echocardiography versus full-volume quantification by cardiac magnetic resonance imaging for the assessment of left atrial volumes in children, adolescents, and young adults. Pediatr Cardiol 2014;35:645-51. https://doi.org/10.1007/s00246-013-0833-y.
- Rabbat MG, Wilber D, Thomas K, Malick O, Bashir A, Agrawal A, et al. Left atrial volume assessment in atrial fibrillation using multimodality imaging: a comparison of echocardiography, invasive three-dimensional CARTO and cardiac magnetic resonance imaging. Int J Cardiovasc Imaging 2015;31:1011-18. https://doi.org/10.1007/s10554-015-0641-y.
- Winkle RA, Mead RH, Engel G, Patrawala RA. Relation of early termination of persistent atrial fibrillation by cardioversion or drugs to ablation outcomes. Am J Cardiol 2011;108:374-9. https://doi.org/10.1016/j.amjcard.2011.03.055.
- Zarse M, Deharo JC, Mast F, Allessie MA. Importance of right and left atrial dilation and linear ablation for perpetuation of sustained atrial fibrillation. J Cardiovasc Electrophysiol 2002;13:164-71. https://doi.org/10.1046/j.1540-8167.2002.00164.x.
- Zakrzewska-Koperska J, Derejko P, Walczak F, Urbanek P, Bodalski R, Orczykowski M, et al. Early stage of left atrium remodelling predicts better outcome in long-term follow-up of atrial fibrillation ablation. Kardiol Pol 2014;72:925-33. https://doi.org/10.5603/KP.a2014.0112.
- Pecha S, Hakmi S, Subbotina I, Willems S, Reichenspurner H, Wagner FM. Concomitant surgical ablation for atrial fibrillation (AF) in patients with significant atrial dilation > 55 mm. Worth the effort?. J Cardiothorac Surg 2015;10. https://doi.org/10.1186/s13019-015-0337-3.
- Berruezo A, Tamborero D, Mont L, Benito B, Tolosana JM, Sitges M, et al. Pre-procedural predictors of atrial fibrillation recurrence after circumferential pulmonary vein ablation. Eur Heart J 2007;28:836-41. https://doi.org/10.1093/eurheartj/ehm027.
- Arya A, Hindricks G, Sommer P, Huo Y, Bollmann A, Gaspar T, et al. Long-term results and the predictors of outcome of catheter ablation of atrial fibrillation using steerable sheath catheter navigation after single procedure in 674 patients. Europace 2010;12:173-80. https://doi.org/10.1093/europace/eup331.
- Yang CH, Chou CC, Hung KC, Wen MS, Chang PC, Wo HT, et al. Comparisons of the underlying mechanisms of left atrial remodeling after repeat circumferential pulmonary vein isolation with or without additional left atrial linear ablation in patients with recurrent atrial fibrillation. Int J Cardiol 2017;228:449-55. https://doi.org/10.1016/j.ijcard.2016.11.020.
- La Meir M, Gelsomino S, Lucà F, Pison L, Rao CM, Wellens F, et al. Improvement of left atrial function and left atrial reverse remodeling after minimally invasive radiofrequency ablation evaluated by 2-dimensional speckle tracking echocardiography. J Thorac Cardiovasc Surg 2013;146:72-7. https://doi.org/10.1016/j.jtcvs.2012.05.068.
- Müller H, Noble S, Keller PF, Sigaud P, Gentil P, Lerch R, et al. Biatrial anatomical reverse remodelling after radiofrequency catheter ablation for atrial fibrillation: evidence from real-time three-dimensional echocardiography. Europace 2008;10:1073-8. https://doi.org/10.1093/europace/eun187.
- Santarpino G, Rubino AS, Onorati F, Curcio A, Torella D, Tucci L, et al. Atrial fibrillation ablation induces reverse remodelling and impacts cardiac function. Minerva Cardioangiol 2011;59:17-29.
- Fornengo C, Antolini M, Frea S, Gallo C, Grosso Marra W, Morello M, et al. Prediction of atrial fibrillation recurrence after cardioversion in patients with left-atrial dilation. Eur Heart J Cardiovasc Imaging 2015;16:335-41. https://doi.org/10.1093/ehjci/jeu193.
- Schoene K, Arya A, Jahnke C, Paetsch I, Nedios S, Hilbert S, et al. Acquired pulmonary vein stenosis after radiofrequency ablation for atrial fibrillation: single-center experience in catheter interventional treatment. JACC Cardiovasc Interv 2018;11:1626-32. https://doi.org/10.1016/j.jcin.2018.05.016.
- Schoene K, Sommer P, Arya A, Kostelka M, Mohr FW, Misfeld M, et al. Complex cases of acquired pulmonary vein stenosis after radiofrequency ablation: is surgical repair an option?. EP Eur 2019;21:73-9. https://doi.org/10.1093/europace/euy017.
- Lang RM, Badano LP, Mor-Avi V, Afilalo J, Armstrong A, Ernande L, et al. Recommendations for cardiac chamber quantification by echocardiography in adults: an update from the American Society of Echocardiography and the European Association of Cardiovascular Imaging. Eur Heart J Cardiovasc Imaging 2015;16:233-70. https://doi.org/10.1093/ehjci/jev014.
- Sievers B, Kirchberg S, Addo M, Bakan A, Brandts B, Trappe HJ. Assessment of left atrial volumes in sinus rhythm and atrial fibrillation using the biplane area–length method and cardiovascular magnetic resonance imaging with TrueFISP. J Cardiovasc Magn Reson 2004;6:855-63. https://doi.org/10.1081/jcmr-200036170.
- Luong C, Thompson DJ, Bennett M, Gin K, Jue J, Barnes ME, et al. Right atrial volume is superior to left atrial volume for prediction of atrial fibrillation recurrence after direct current cardioversion. Can J Cardiol 2015;31:29-35. https://doi.org/10.1016/j.cjca.2014.10.009.
- Sasaki T, Nakamura K, Naito S, Minami K, Koyama K, Yamashita E, et al. The right to left atrial volume ratio predicts outcomes after circumferential pulmonary vein isolation of longstanding persistent atrial fibrillation: RA/LA volume ratio in AF ablation. Pacing Clin Electrophysiol 2016;39:1181-90. https://doi.org/10.1111/pace.12953.
- Cuspidi C, Negri F, Sala C, Valerio C, Mancia G. Association of left atrial enlargement with left ventricular hypertrophy and diastolic dysfunction: a tissue Doppler study in echocardiographic practice. Blood Press 2012;21:24-30. https://doi.org/10.3109/08037051.2011.618262.
- Weijs B, de Vos CB, Limantoro I, Cheriex EC, Tieleman RG, Crijns HJ. The presence of an atrial electromechanical delay in idiopathic atrial fibrillation as determined by tissue Doppler imaging. Int J Cardiol 2012;156:121-2. https://doi.org/10.1016/j.ijcard.2012.01.024.
- Erdem FH, Erdem A, Özlü F, Ozturk S, Ayhan SS, Çağlar SO, et al. Electrophysiological validation of total atrial conduction time measurement by tissue doppler echocardiography according to age and sex in healthy adults. J Arrhythm 2016;32:127-32. https://doi.org/10.1016/j.joa.2015.11.006.
- Safir-Mardanloo A, Khorsand Askari M, Lotfi Tokaldany M, Ashrafi MM, Sadeghian H. Normal values for tissue velocity and strain rate imaging parameters of left and right atrial myocardium in normal subjects. Echocardiography 2019;36:521-7. https://doi.org/10.1111/echo.14261.
- Kim JH, Jang WS, Kim JB, Lee SJ. Impact of volume reduction in giant left atrium during surgical ablation of atrial fibrillation. J Thorac Dis 2019;11:84-92. https://doi.org/10.21037/jtd.2018.12.118.
- Oka T, Inoue K, Tanaka K, Ninomiya Y, Hirao Y, Tanaka N, et al. Left atrial reverse remodeling after catheter ablation of nonparoxysmal atrial fibrillation in patients with heart failure with reduced ejection fraction. Am J Cardiol 2018;122:89-96. https://doi.org/10.1016/j.amjcard.2018.03.026.
- Tops LF, Delgado V, Bertini M, Marsan NA, Den Uijl DW, Trines SA, et al. Left atrial strain predicts reverse remodeling after catheter ablation for atrial fibrillation. J Am Coll Cardiol 2011;57:324-31. https://doi.org/10.1016/j.jacc.2010.05.063.
- Kuppahally SS, Akoum N, Badger TJ, Burgon NS, Haslam T, Kholmovski E, et al. Echocardiographic left atrial reverse remodeling after catheter ablation of atrial fibrillation is predicted by preablation delayed enhancement of left atrium by magnetic resonance imaging. Am Heart J 2010;160:877-84. https://doi.org/10.1016/j.ahj.2010.07.003.
- Phung T-KN, Moyer CB, Norton PT, Ferguson JD, Holmes JW. Effect of ablation pattern on mechanical function in the atrium. Pacing Clin Electrophysiol 2017;40:648-54. https://doi.org/10.1111/pace.13086.
- Itakura K, Hidaka T, Nakano Y, Utsunomiya H, Kinoshita M, Susawa H, et al. Successful catheter ablation of persistent atrial fibrillation is associated with improvement in functional tricuspid regurgitation and right heart reverse remodeling. Heart Vessels 2020;35:842-51. https://doi.org/10.1007/s00380-019-01546-3.
- Compier MG, Tops LF, Braun J, Zeppenfeld K, Klautz RJ, Schalij MJ, et al. Limited left atrial surgical ablation effectively treats atrial fibrillation but decreases left atrial function. Europace 2017;19:560-7. https://doi.org/10.1093/europace/euw106.
- Allessie M, Ausma J, Schotten U. Electrical, contractile and structural remodeling during atrial fibrillation. Cardiovasc Res 2002;54:230-46. https://doi.org/10.1016/S0008-6363(02)00258-4.
- Tandon K, Tirschwell D, Longstreth WT, Smith B, Akoum N. Embolic stroke of undetermined source correlates to atrial fibrosis without atrial fibrillation. Neurology 2019;93:e381-7. https://doi.org/10.1212/WNL.0000000000007827.
- Karim R, Housden RJ, Balasubramaniam M, Chen Z, Perry D, Uddin A, et al. Evaluation of current algorithms for segmentation of scar tissue from late gadolinium enhancement cardiovascular magnetic resonance of the left atrium: an open-access grand challenge. J Cardiovasc Magn Reson 2013;15. https://doi.org/10.1186/1532-429X-15-105.
- Zhong H, Lacomis JM, Schwartzman D. On the accuracy of CartoMerge for guiding posterior left atrial ablation in man. Heart Rhythm 2007;4:595-602. https://doi.org/10.1016/j.hrthm.2007.01.033.
- Schmidt EJ, Mallozzi RP, Thiagalingam A, Holmvang G, d’Avila A, Guhde R, et al. Electroanatomic mapping and radiofrequency ablation of porcine left atria and atrioventricular nodes using magnetic resonance catheter tracking. Circ Arrhythm Electrophysiol 2009;2:695-704. https://doi.org/10.1161/CIRCEP.109.882472.
- Simonetti OP, Kim RJ, Fieno DS, Hillenbrand HB, Wu E, Bundy JM, et al. An improved MR imaging technique for the visualization of myocardial infarction. Radiology 2001;218:215-23. https://doi.org/10.1148/radiology.218.1.r01ja50215.
- Oakes RS, Badger TJ, Kholmovski EG, Akoum N, Burgon NS, Fish EN, et al. Detection and quantification of left atrial structural remodeling with delayed-enhancement magnetic resonance imaging in patients with atrial fibrillation. Circulation 2009;119:1758-67. https://doi.org/10.1161/CIRCULATIONAHA.108.811877.
- Marrouche NF, Wilber D, Hindricks G, Jais P, Akoum N, Marchlinski F, et al. Association of atrial tissue fibrosis identified by delayed enhancement MRI and atrial fibrillation catheter ablation: the DECAAF study. JAMA 2014;311:498-506. https://doi.org/10.1001/jama.2014.3.
- Vergara GR, Marrouche NF. Tailored management of atrial fibrillation using a LGE-MRI based model: from the clinic to the electrophysiology laboratory. J Cardiovasc Electrophysiol 2011;22:481-7. https://doi.org/10.1111/j.1540-8167.2010.01941.x.
- Akoum N, Daccarett M, McGann C, Segerson N, Vergara G, Kuppahally S, et al. Atrial fibrosis helps select the appropriate patient and strategy in catheter ablation of atrial fibrillation: a DE-MRI guided approach. J Cardiovasc Electrophysiol 2011;22:16-22. https://doi.org/10.1111/j.1540-8167.2010.01876.x.
- Malcolme-Lawes LC, Juli C, Karim R, Bai W, Quest R, Lim PB, et al. Automated analysis of atrial late gadolinium enhancement imaging that correlates with endocardial voltage and clinical outcomes: a 2-center study. Heart Rhythm 2013;10:1184-91. https://doi.org/10.1016/j.hrthm.2013.04.030.
- Ravanelli D, dal Piaz EC, Centonze M, Casagranda G, Marini M, Del Greco M, et al. A novel skeleton based quantification and 3-D volumetric visualization of left atrium fibrosis using late gadolinium enhancement magnetic resonance imaging. IEEE Trans Med Imaging 2014;33:566-76. https://doi.org/10.1109/TMI.2013.2290324.
- McGann C, Akoum N, Patel A, Kholmovski E, Revelo P, Damal K, et al. Atrial fibrillation ablation outcome is predicted by left atrial remodeling on MRI. Circ Arrhythm Electrophysiol 2014;7:23-30. https://doi.org/10.1161/CIRCEP.113.000689.
- Jadidi AS, Cochet H, Shah AJ, Kim SJ, Duncan E, Miyazaki S, et al. Inverse relationship between fractionated electrograms and atrial fibrosis in persistent atrial fibrillation: combined magnetic resonance imaging and high-density mapping. J Am Coll Cardiol 2013;62:802-12. https://doi.org/10.1016/j.jacc.2013.03.081.
- Harrison JL, Jensen HK, Peel SA, Chiribiri A, Grøndal AK, Bloch LØ, et al. Cardiac magnetic resonance and electroanatomical mapping of acute and chronic atrial ablation injury: a histological validation study. Eur Heart J 2014;35:1486-95. https://doi.org/10.1093/eurheartj/eht560.
- Akoum N, Wilber D, Hindricks G, Jais P, Cates J, Marchlinski F, et al. MRI assessment of ablation-induced scarring in atrial fibrillation: analysis from the DECAAF Study. J Cardiovasc Electrophysiol 2015;26:473-80. https://doi.org/10.1111/jce.12650.
- Akoum N, McGann C, Vergara G, Badger T, Ranjan R, Mahnkopf C, et al. Atrial fibrosis quantified using late gadolinium enhancement MRI is associated with sinus node dysfunction requiring pacemaker implant. J Cardiovasc Electrophysiol 2012;23:44-50. https://doi.org/10.1111/j.1540-8167.2011.02140.x.
- Daccarett M, Badger TJ, Akoum N, Burgon NS, Mahnkopf C, Vergara G, et al. Association of left atrial fibrosis detected by delayed-enhancement magnetic resonance imaging and the risk of stroke in patients with atrial fibrillation. J Am Coll Cardiol 2011;57:831-8. https://doi.org/10.1016/j.jacc.2010.09.049.
- Akoum N, Fernandez G, Wilson B, Mcgann C, Kholmovski E, Marrouche N. Association of atrial fibrosis quantified using LGE-MRI with atrial appendage thrombus and spontaneous contrast on transesophageal echocardiography in patients with atrial fibrillation. J Cardiovasc Electrophysiol 2013;24:1104-9. https://doi.org/10.1111/jce.12199.
- Parmar BR, Jarrett TR, Burgon NS, Kholmovski EG, Akoum NW, Hu N, et al. Comparison of left atrial area marked ablated in electroanatomical maps with scar in MRI: electroanatomic map overestimates the ablated area. J Cardiovasc Electrophysiol 2014;25:457-63. https://doi.org/10.1111/jce.12357.
- Kheirkhahan M, Baher A, Goldooz M, Kholmovski EG, Morris AK, Csecs I, et al. Left atrial fibrosis progression detected by LGE-MRI after ablation of atrial fibrillation. Pacing Clin Electrophysiol 2020;43:402-11. https://doi.org/10.1111/pace.13866.
- Higuchi K, Akkaya M, Akoum N, Marrouche NF. Cardiac MRI assessment of atrial fibrosis in atrial fibrillation: implications for diagnosis and therapy. Heart 2014;100:590-6. https://doi.org/10.1136/heartjnl-2013-303884.
- Appelbaum E, Manning WJ. Left atrial fibrosis by late gadolinium enhancement cardiovascular magnetic resonance predicts recurrence of atrial fibrillation after pulmonary vein isolation: do you see what I see?. Circ Arrhythm Electrophysiol 2014;7:2-4. https://doi.org/10.1161/CIRCEP.114.001354.
- Almeida AG. Searching for the key to foreseeing left atrial fibrosis in atrial fibrillation: could this be simple and reliable with MRI?. Radiology 2019;292:583-4. https://doi.org/10.1148/radiol.2019191221.
- Hunter RJ, Jones DA, Boubertakh R, Malcolme-Lawes LC, Kanagaratnam P, Juli CF, et al. Diagnostic accuracy of cardiac magnetic resonance imaging in the detection and characterization of left atrial catheter ablation lesions: a multicenter experience. J Cardiovasc Electrophysiol 2013;24:396-403. https://doi.org/10.1111/jce.12063.
- Chen J, Arentz T, Cochet H, Müller-Edenborn B, Kim S, Moreno-Weidmann Z, et al. Extent and spatial distribution of left atrial arrhythmogenic sites, late gadolinium enhancement at magnetic resonance imaging, and low-voltage areas in patients with persistent atrial fibrillation: comparison of imaging vs. electrical parameters of fibrosis and arrhythmogenesis. Europace 2019;21:1484-93. https://doi.org/10.1093/europace/euz159.
- Tobon-Gomez C, Geers AJ, Peters J, Weese J, Pinto K, Karim R, et al. Benchmark for algorithms segmenting the left atrium from 3D CT and MRI datasets. IEEE Trans Med Imaging 2015;34:1460-73. https://doi.org/10.1109/TMI.2015.2398818.
- Karim R, Blake LE, Inoue J, Tao Q, Jia S, Housden RJ, et al. Algorithms for left atrial wall segmentation and thickness – evaluation on an open-source CT and MRI image database. Med Image Anal 2018;50:36-53. https://doi.org/10.1016/j.media.2018.08.004.
- Giannakidis A, Nyktari E, Keegan J, Pierce I, Suman Horduna I, Haldar S, et al. Rapid automatic segmentation of abnormal tissue in late gadolinium enhancement cardiovascular magnetic resonance images for improved management of long-standing persistent atrial fibrillation. Biomed Eng Online 2015;14. https://doi.org/10.1186/s12938-015-0083-8.
- Yang G, Zhuang X, Khan H, Haldar S, Nyktari E, Li L, et al. Fully automatic segmentation and objective assessment of atrial scars for long-standing persistent atrial fibrillation patients using late gadolinium-enhanced MRI. Med Phys 2018;45:1562-76. https://doi.org/10.1002/mp.12832.
- Peters DC, Wylie JV, Hauser TH, Nezafat R, Han Y, Woo JJ, et al. Recurrence of atrial fibrillation correlates with the extent of post-procedural late gadolinium enhancement: a pilot study. JACC Cardiovasc Imaging 2009;2:308-16. https://doi.org/10.1016/j.jcmg.2008.10.016.
- Peters DC, Wylie JV, Hauser TH, Kissinger KV, Botnar RM, Essebag V, et al. Detection of pulmonary vein and left atrial scar after catheter ablation with three-dimensional navigator-gated delayed enhancement MR imaging: initial experience 1. Radiology 2007;243:690-5. https://doi.org/10.1148/radiol.2433060417.
- Keegan J, Jhooti P, Babu-Narayan SV, Drivas P, Ernst S, Firmin DN. Improved respiratory efficiency of 3D late gadolinium enhancement imaging using the continuously adaptive windowing strategy (CLAWS): improved respiratory efficiency of 3D LGE imaging with CLAWS. Magn Reson Med 2014;71:1064-74. https://doi.org/10.1002/mrm.24758.
- Takahashi Y, O’Neill MD, Hocini M, Reant P, Jonsson A, Jaïs P, et al. Effects of stepwise ablation of chronic atrial fibrillation on atrial electrical and mechanical properties. J Am Coll Cardiol 2007;49:1306-14. https://doi.org/10.1016/j.jacc.2006.11.033.
- Keegan J, Drivas P, Firmin DN. Navigator artifact reduction in three-dimensional late gadolinium enhancement imaging of the atria: navigator artifact reduction in 3D LGE imaging. Magn Reson Med 2014;72:779-85. https://doi.org/10.1002/mrm.24967.
- Moghari MH, Peters DC, Smink J, Goepfert L, Kissinger KV, Goddu B, et al. Pulmonary vein inflow artifact reduction for free-breathing left atrium late gadolinium enhancement. Magn Reson Med 2011;66:180-6. https://doi.org/10.1002/mrm.22769.
- Krishnam MS, Tomasian A, Deshpande V, Tran L, Laub G, Finn JP, et al. Noncontrast 3D steady-state free-precession magnetic resonance angiography of the whole chest using nonselective radiofrequency excitation over a large field of view: comparison with single-phase 3D contrast-enhanced magnetic resonance angiography. Invest Radiol 2008;43:411-20. https://doi.org/10.1097/RLI.0b013e3181690179.
- Yang G, Chen J, Gao Z, Zhang H, Ni H, Angelini E, et al. Multiview sequential learning and dilated residual learning for a fully automatic delineation of the left atrium and pulmonary veins from late gadolinium-enhanced cardiac MRI images. Annu Int Conf IEEE Eng Med Biol Soc 2018:1123-7. https://doi.org/10.1109/EMBC.2018.8512550.
- Information Resources Management Association . Deep Learning and Neural Networks: Concepts, Methodologies, Tools, and Applications 2020. https://doi.org/10.4018/978-1-7998-0414-7.
- Shen D, Wu G, Suk HI. Deep learning in medical image analysis. Annu Rev Biomed Eng 2017;19:221-48. https://doi.org/10.1146/annurev-bioeng-071516-044442.
- Moccia S, Banali R, Martini C, Muscogiuri G, Pontone G, Pepi M, et al. Development and testing of a deep learning-based strategy for scar segmentation on CMR-LGE images. MAGMA 2019;32:187-95. https://doi.org/10.1007/s10334-018-0718-4.
- Pontecorboli G, Figueras i, Ventura RM, Carlosena A, Benito A, Prat-Gonzales S, et al. Use of delayed-enhancement magnetic resonance imaging for fibrosis detection in the atria: a review. Europace 2017;19:180-9. https://doi.org/10.1093/europace/euw053.
- Wiles BM, Illingworth CA, Couzins ML, Roberts PR, Harden SP. A tertiary centre experience of thoracic CT and cardiac MRI scanning in the presence of a reveal LINQ insertable cardiac monitoring system: a case series review of artefact, patient safety and data preservation. Br J Radiol 2018;91. https://doi.org/10.1259/bjr.20170615.
- Kirchhof P, Benussi S, Kotecha D, Ahlsson A, Atar D, Casadei B, et al. 2016 ESC Guidelines for the management of atrial fibrillation developed in collaboration with EACTS. Eur Heart J 2016;37:2893-962. https://doi.org/10.1093/eurheartj/ehw210.
- Sirak J, Jones D, Sun B, Sai-Sudhakar C, Crestanello J, Firstenberg M. Toward a definitive, totally thoracoscopic procedure for atrial fibrillation. Ann Thorac Surg 2008;86:1960-4. https://doi.org/10.1016/j.athoracsur.2008.07.066.
- Krul SP, Driessen AH, van Boven WJ, Linnenbank AC, Geuzebroek GS, Jackman WM, et al. Thoracoscopic video-assisted pulmonary vein antrum isolation, ganglionated plexus ablation, and periprocedural confirmation of ablation lesions: first results of a hybrid surgical-electrophysiological approach for atrial fibrillation. Circ Arrhythm Electrophysiol 2011;4:262-70. https://doi.org/10.1161/CIRCEP.111.961862.
- Pokushalov E, Romanov A, Elesin D, Bogachev-Prokophiev A, Losik D, Bairamova S, et al. Catheter versus surgical ablation of atrial fibrillation after a failed initial pulmonary vein isolation procedure: a randomized controlled trial. J Cardiovasc Electrophysiol 2013;24:1338-43. https://doi.org/10.1111/jce.12245.
- Adiyaman A, Buist TJ, Beukema RJ, Smit JJJ, Delnoy PPHM, Hemels MEW, et al. Randomized controlled trial of surgical versus catheter ablation for paroxysmal and early persistent atrial fibrillation. Circ Arrhythm Electrophysiol 2018;11. https://doi.org/10.1161/CIRCEP.118.006182.
- Wechselberger S, Kronborg M, Huo Y, Piorkowski J, Neudeck S, Päßler E, et al. Continuous monitoring after atrial fibrillation ablation: the LINQ AF study. Europace 2018;20:f312-f320. https://doi.org/10.1093/europace/euy038.
- Balabanski T, Brugada J, Arbelo E, Laroche C, Maggioni A, Blomström-Lundqvist C, et al. Impact of monitoring on detection of arrhythmia recurrences in the ESC-EHRA EORP atrial fibrillation ablation long-term registry. Europace 2019;21:1802-8. https://doi.org/10.1093/europace/euz216.
- Hanke T, Charitos EI, Stierle U, Karluss A, Kraatz E, Graf B, et al. Twenty-four-hour holter monitor follow-up does not provide accurate heart rhythm status after surgical atrial fibrillation ablation therapy: up to 12 months experience with a novel permanently implantable heart rhythm monitor device. Circulation 2009;120:S177-84. https://doi.org/10.1161/CIRCULATIONAHA.108.838474.
- Martinek M, Aichinger J, Nesser HJ, Ziegler PD, Purerfellner H. New insights into long-term follow-up of atrial fibrillation ablation: full disclosure by an implantable pacemaker device. J Cardiovasc Electrophysiol 2007;18:818-23. https://doi.org/10.1111/j.1540-8167.2007.00883.x.
- Ariyarathna N, Kumar S, Thomas SP, Stevenson WG, Michaud GF. Role of contact force sensing in catheter ablation of cardiac arrhythmias: evolution or history repeating itself?. JACC Clin Electrophysiol 2018;4:707-23. https://doi.org/10.1016/j.jacep.2018.03.014.
- Segerson NM, Lynch B, Mozes J, Marks MM, Noonan DK, Gordon D, et al. High-density mapping and ablation of concealed low-voltage activity within pulmonary vein antra results in improved freedom from atrial fibrillation compared to pulmonary vein isolation alone. Heart Rhythm 2018;15:1158-64. https://doi.org/10.1016/j.hrthm.2018.04.035.
- Hussein A, Das M, Riva S, Morgan M, Ronayne C, Sahni A, et al. Use of ablation index-guided ablation results in high rates of durable pulmonary vein isolation and freedom from arrhythmia in persistent atrial fibrillation patients: the PRAISE study results. Circ Arrhythm Electrophysiol 2018;11. https://doi.org/10.1161/CIRCEP.118.006576.
Appendix 1 Schedule of enrolment
Tests/data collection | Baseline | Treatment allocation | Index ablation | Blanking period: months after index ablation | |||||||||||
---|---|---|---|---|---|---|---|---|---|---|---|---|---|---|---|
1 | 2 | 3 | 4 | 5 | 6 | 7 | 8 | 9 | 10 | 11 | 12 | ||||
Post-operative surveillance | First follow-up | Second follow-up | Third follow-up | Fourth follow-up | |||||||||||
Registration (demographics) | ✗ | ||||||||||||||
Eligibility | ✗ | ||||||||||||||
Medical history (significant conditions not related to LSPAF) | ✗ | ||||||||||||||
Cardiac risk history (medical conditions associated with AF) | ✗ | ||||||||||||||
Cardiac risk scoring (CHA2DS2-VASc, HAS-BLED) | ✗ | ✗ | |||||||||||||
Concomitant medication | ✗ | ✗ | ✗ | ✗ | ✗ | ✗ | |||||||||
Current anticoagulant therapy | ✗ | ✗ | ✗ | ✗ | ✗ | ✗ | |||||||||
Arrhythmia characteristics (diagnosis date, prior history of cardioversion, other arrhythmias, other ablations) | ✗ | ||||||||||||||
EHRA AF classification | ✗ | ✗ | ✗ | ✗ | ✗ | ||||||||||
Clinical examination (blood pressure, HR, weight) | ✗ | ✗ | ✗ | ✗ | ✗ | ||||||||||
Haematological and biochemical assessments | ✗ | ✗ | ✗ | ✗ | |||||||||||
12-lead ECG | ✗ | ✗ | ✗ | ✗ | ✗ | ||||||||||
TTE | ✗ | ✗ | ✗ | ||||||||||||
Cardiac magnetic resonance | ✗ | ✗ | |||||||||||||
EQ-5D-5L questionnaire | ✗ | ✗ | ✗ | ✗ | ✗ | ||||||||||
AFEQT questionnaire | ✗ | ✗ | ✗ | ✗ | ✗ | ||||||||||
Allocate to treatment | ✗ | ||||||||||||||
Health economics questionnaire | ✗ | ✗ | ✗ | ✗ | |||||||||||
Adverse events | ✗ | ✗ | ✗ | ✗ | ✗ | ✗ | |||||||||
ILR interrogation (primary outcome) | ✗ | ✗ | ✗ | ✗ | ✗ | ✗ | ✗ | ✗ | ✗ | ✗ | ✗ | ✗ | |||
TOE | ✗ | ||||||||||||||
CA | ✗ | ||||||||||||||
SA | ✗ | ||||||||||||||
Discharge | ✗ | ||||||||||||||
DCCV (if needed) | ✗ | ✗ | ✗ | ✗ | ✗ | ✗ | |||||||||
Clinical CA (if needed) | ✗ | ✗ | ✗ | ✗ |
Appendix 2 Supplementary sensitivity analyses of the heart rhythm outcomes
Outcome | Complete case in modified intention to treat | ||||||||||
---|---|---|---|---|---|---|---|---|---|---|---|
Treatment group, raw data, n/N (%) | OR | RR | LR model (adjusted for minimisation variables) | ||||||||
SAa | CA | OR | 95% CI | p-value | RR | 95% CI | p-value (χ2) | OR | 95% CI | p-value | |
Free from AF/AT after a single procedure without AADs | 14/54 (26) | 17/60 (28) | 1.13 | 0.46 to 2.83 | 0.84 | 1.09 | 0.60 to 2.00 | 0.77 | 1.25 | 0.53 to 3.04 | 0.62 |
≥ 75% reduction in AF/AT burden after one procedure without AADs | 36/54 (67) | 46/60 (77) | 1.64 | 0.67 to 4.08 | 0.30 | 1.50 | 0.91 to 1.45 | 0.24 | 2.11 | 0.89 to 5.22 | 0.11 |
≥ 75% reduction in AF/AT burden after one procedure with AADs | 39/54 (72) | 49/60 (82) | 1.71 | 0.71 to 4.15 | 0.23 | 1.13 | 0.92 to 1.39 | 0.23 | 1.72 | 0.65 to 4.67 | 0.27 |
Outcome | Treatment arm, n/N (%) | MI for missing data | ||||||
---|---|---|---|---|---|---|---|---|
OR | RR | |||||||
SAa | CA | OR | 95% CI | p-value | RR | 95% CI | p-value (χ2) | |
Free from AF/AT after a single procedure without AADs | 60 | 60 | 1.10 | 0.75 to 1.49 | 0.80 | 1.08 | 0.80 to 1.32 | 0.80 |
≥ 75% reduction in AF/AT burden after one procedure without AADs | 60 | 60 | 1.56 | 0.69 to 3.56 | 0.29 | 1.14 | 0.87 to 1.03 | 0.29 |
≥ 75% reduction in AF/AT burden after one procedure with AADs | 60 | 60 | 1.64 | 0.78 to 2.46 | 0.28 | 0.99 | 0.93 to 1.20 | 0.28 |
Outcome | Treatment arm, n/N (%) | Per-protocol analysisa | ||||||
---|---|---|---|---|---|---|---|---|
OR | RR | |||||||
SAb | CA | OR | 95% CI | p-value | RR | 95% CI | p-value (χ2) | |
Free from AF/AT after a single procedure without AADs | 11/39 (28) | 17/53 (32) | 1.20 | 0.45 to 3.32 | 0.82 | 1.14 | 0.60 to 2.15 | 0.69 |
≥ 75% reduction in AF/AT burden after one procedure | 33/39 (85) | 46/53 (87) | 1.19 | 0.30 to 4.58 | 0.77 | 1.03 | 0.81 to 1.22 | 0.77 |
Appendix 3 Supplementary tables for echocardiographic and magnetic resonance analyses chapters
Baseline characteristic | Treatment arm | |
---|---|---|
CA (N = 33) | SA (N = 26) | |
Age (years), mean ± SD | 61 ± 11 | 65.2 ± 8.4 |
Sex (male), n (%) | 25 (76) | 20 (77) |
AF duration (days), mean (95% CI) | 575 (417.5 to 858.5) | 754.5 (416.5 to 905) |
BMI (kg/m2), mean ± SD | 31.4 ± 5.8 | 29.7 ± 4.1 |
LV EF (%), mean ± SD | 55.8 ± 7.2 | 57 ± 7.7 |
Smoking, n (%) | 3 (9) | 1 (4) |
Hypertension, n (%) | 17 (52) | 14 (54) |
Diabetes, n (%) | 3 (9) | 1 (4) |
Alcohol use, n (%) | 19 (58) | 12 (46) |
Stroke/TIA, n (%) | 0 (0) | 3 (12) |
Thyroid disorders, n (%) | 2 (6) | 2 (8) |
Coronary artery disease, n (%) | 4 (12) | 3 (11.5) |
Renal disease, n (%) | 4 (12) | 1 (4) |
Respiratory disease, n (%) | 3 (9) | 6 (23) |
Medication, n (%) | ||
Beta-blocker | 29 (88) | 22 (85) |
Digoxin | 10 (30) | 3 (12) |
Calcium channel blocker | 4 (12) | 4 (15) |
ACE/ARB | 16 (49) | 11 (42) |
Diuretic | 8 (24) | 9 (35) |
AADs | 4 (12) | 3 (12) |
Statin | 13 (39) | 14 (54) |
Baseline characteristic | Treatment arm | |
---|---|---|
CA (N = 29) | SA (N = 25) | |
Age (years), mean ± SD | 60 ± 10.2 | 65 ± 8.5 |
Sex (male), n (%) | 24 (82.8) | 19 (76) |
BMI (kg/m2), mean ± SD | 31.6 ± 6 | 29.6 ± 4.1 |
Smoking, n (%) | 1 (3.4) | 1 (4.2) |
Hypertension, n (%) | 16 (55.2) | 13 (52) |
Diabetes, n (%) | 3 (10.3) | 1 (4.2) |
Stroke, n (%) | 0 (0) | 3 (12) |
Coronary artery disease, n (%) | 4 (13.8) | 2 (8) |
Renal disease, n (%) | 4 (13.8) | 1 (4.2) |
Respiratory disease, n (%) | 3 (10.3) | 6 (24) |
Thyroid disorders, n (%) | 2 (6.9) | 2 (8) |
Alcohol use, n (%) | 17 (58.6) | 12 (48) |
Medication, n (%) | ||
Beta-blocker | 26 (90) | 21 (84) |
Digoxin | 7 (24.1) | 3 (12) |
Calcium channel blocker | 3 (10.3) | 4 (16) |
ACE/ARB | 15 (51.7) | 10 (40) |
Diuretic | 7 (24.1) | 9 (36) |
AADs | 4 (13.8) | 3 (12) |
Statin | 11 (37.9) | 13 (52) |
Baseline characteristic | Treatment arm | |
---|---|---|
CA (N = 26) | SA (N = 26) | |
Age (years), mean ±SD | 64 ± 8.9 | 67.4 ± 8.3 |
Sex (male), n (%) | 20 (76.9%) | 20 (76.9%) |
BMI (kg/m2), mean ± SD | 30.8 ± 4.6 | 29.5 ± 4.6 |
Smoking, n (%) | 2 (7.7) | 0 (0) |
Hypertension, n (%) | 10 (38.5) | 17 (65.4) |
Diabetes, n (%) | 2 (7.7) | 2 (7.7) |
Stroke, n (%) | 0 (0) | 2 (7.7) |
Coronary artery disease, n (%) | 5 (19.2) | 4 (15.4) |
Renal disease, n (%) | 3 (11.5) | 1 (3.8) |
Respiratory disease, n (%) | 4 (15.4) | 4 (15.4) |
Thyroid disorders, n (%) | 1 (3.8) | 3 (11.5) |
Alcohol use, n (%) | 15 (57.7) | 12 (46.2) |
Medication, n (%) | ||
Beta-blocker | 21 (80.8) | 21 (80.8) |
Digoxin | 9 (34.6) | 3 (11.5) |
Calcium channel blocker | 3 (11.5) | 3 (11.5) |
ACE/ARB | 12 (46.2) | 13 (50) |
Diuretic | 6 (23) | 11 (42.3) |
AADs | 1 (3.8) | 1 (3.8) |
Statin | 16 (61.5) | 14 (53.9) |
Location | Parameter | Time point | Treatment arm, mean ± SD | p-value | |
---|---|---|---|---|---|
CA (n = 26) | SA (n = 26) | ||||
Septum | S′ (cm/second) | Baseline | 6.6 ± 2.7 | 7.8 ± 2.2 | 0.08 |
3 months | 6.8 ± 2 | 7.1 ± 2.3 | 0.57 | ||
12 months | 8.2 ± 2.9 | 8.2 ± 2.7 | 0.96 | ||
Reservoir (%) | Baseline | 5.3 ± 1.5 | 5.6 ± 1.4 | 0.56 | |
3 months | 6 ± 1.3 | 5.7 ± 1.8 | 0.52 | ||
12 months | 6.5 ± 2.1 | 6.3 ± 2.3 | 0.67 | ||
Conduit (%) | Baseline | 7.4 ± 2.2 | 7.6 ± 2.6 | 0.76 | |
3 months | 6.9 ± 2.1 | 6.3 ± 2.1 | 0.3 | ||
12 months | 6.3 ± 2.3 | 6.5 ± 2.2 | 0.75 | ||
Booster (%) | Baseline | – | – | ||
3 months | 6.1 ± 1.9 | 6 ± 1.9 | 0.86 | ||
12 months | 6.5 ± 2.1 | 6 ± 1.9 | 0.37 | ||
Lateral | S′ (cm/second) | Baseline | 6.9 ± 2.7 | 7.9 ± 2.8 | 0.21 |
3 months | 6 ± 2.1 | 5.9 ± 1.9 | 0.8 | ||
12 months | 6.7 ± 2.6 | 6.3 ± 2.7 | 0.65 | ||
Reservoir (%) | Baseline | 6.7 ± 1.8 | 7.1 ± 2.2 | 0.53 | |
3 months | 7.1 ± 1.6 | 7.1 ± 2 | 0.97 | ||
12 months | 7.5 ± 2.1 | 7.9 ± 2.1 | 0.56 | ||
Conduit (%) | Baseline | 10.6 ± 2.6 | 11.2 ± 4.3 | 0.55 | |
3 months | 8.5 ± 1.3 | 9.9 ± 3.8 | 0.07 | ||
12 months | 8.7 ± 1.5 | 10.3 ± 3.2 | 0.03 | ||
Booster (%) | Baseline | – | – | ||
3 months | 5.7 ± 2.4 | 5.2 ± 1.7 | 0.45 | ||
12 months | 6.2 ± 2.8 | 4.8 ± 1.7 | 0.05 | ||
Anterior | S′ (cm/second) | Baseline | 7.1 ± 2 | 8.2 ± 2.5 | 0.09 |
3 months | 6.7 ± 2.3 | 5.7 ± 2.2 | 0.11 | ||
12 months | 7.5 ± 2.9 | 6.1 ± 2.3 | 0.05 | ||
Reservoir (%) | Baseline | 6.6 ± 2 | 7.1 ± 1.8 | 0.40 | |
3 months | 6.8 ± 2.1 | 7.3 ± 1.5 | 0.33 | ||
12 months | 7.7 ± 1.6 | 7.4 ± 2 | 0.52 | ||
Conduit (%) | Baseline | 10.7 ± 2.8 | 12.2 ± 4.4 | 0.16 | |
3 months | 8.4 ± 2.7 | 8.7 ± 2.5 | 0.65 | ||
12 months | 8.7 ± 1.7 | 8.6 ± 2.3 | 0.84 | ||
Booster (%) | Baseline | – | – | ||
3 months | 6.4 ± 2.5 | 5.8 ± 2.2 | 0.41 | ||
12 months | 7.1 ± 2.4 | 5.5 ± 2.3 | 0.02 | ||
Inferior | S′ (cm/second) | Baseline | 8.1 ± 3.2 | 8.4 ± 2.9 | 0.67 |
3 months | 7.3 ± 2.6 | 7.6 ± 2.7 | 0.63 | ||
12 months | 8.3 ± 2.9 | 8.3 ± 2.1 | 0.92 | ||
Reservoir (%) | Baseline | 5.9 ± 1.4 | 6.4 ± 1.6 | 0.31 | |
3 months | 6.5 ± 1.8 | 6.5 ± 1.8 | 1 | ||
12 months | 7.4 ± 1.9 | 7.3 ± 2.1 | 0.82 | ||
Conduit (%) | Baseline | 8.3 ± 2.2 | 9.5 ± 3.2 | 0.11 | |
3 months | 6.8 ± 1.7 | 7.3 ± 2.3 | 0.44 | ||
12 months | 7.3 ± 2.1 | 7.1 ± 2.2 | 0.81 | ||
Booster (%) | Baseline | – | – | ||
3 months | 6.8 ± 2.1 | 7.2 ± 2.2 | 0.49 | ||
12 months | 7.4 ± 1.9 | 7.1 ± 2.7 | 0.66 |
Location | Parameter | Time point | Treatment arm, mean ± SD | p-value | |
---|---|---|---|---|---|
AF (n = 35) | SR (n = 17) | ||||
Septum | S′ (cm/second) | Baseline | 7.4 ± 2.8 | 6.8 ± 1.7 | 0.35 |
3 months | 7 ± 2.2 | 7 ± 2.1 | 0.95 | ||
12 months | 8.3 ± 2.9 | 8 ± 2.6 | 0.78 | ||
Reservoir (%) | Baseline | 5.5 ± 1.6 | 5.4 ± 1.1 | 0.79 | |
3 months | 5.7 ± 1.7 | 6.2 ± 1.3 | 0.24 | ||
12 months | 6.1 ± 1.9 | 7 ± 2 | 0.14 | ||
Conduit (%) | Baseline | 7.3 ± 2.5 | 7.9 ± 2.1 | 0.36 | |
3 months | 6.7 ± 2.2 | 6.4 ± 1.8 | 0.66 | ||
12 months | 6.4 ± 2.1 | 6.5 ± 2.6 | 0.82 | ||
Booster (%) | Baseline | – | – | ||
3 months | 5.6 ± 1.9 | 6.9 ± 1.7 | 0.07 | ||
12 months | 5.9 ± 1.8 | 7.2 ± 2.3 | 0.1 | ||
Lateral | S′ (cm/second) | Baseline | 7.6 ± 2.5 | 6.8 ± 3.2 | 0.34 |
3 months | 6 ± 2.1 | 5.9 ± 1.9 | 0.93 | ||
12 months | 6.7 ± 2.9 | 6.2 ± 2.1 | 0.53 | ||
Reservoir (%) | Baseline | 7 ± 2.2 | 6.8 ± 1.6 | 0.79 | |
3 months | 6.8 ± 1.8 | 7.7 ± 1.5 | 0.07 | ||
12 months | 7.6 ± 2.2 | 8 ± 1.8 | 0.41 | ||
Conduit (%) | Baseline | 11.1 ± 3.7 | 10.4 ± 3.1 | 0.5 | |
3 months | 8.9 ± 2.8 | 9.9 ± 3 | 0.26 | ||
12 months | 9.6 ± 2.5 | 9.5 ± 3 | 0.89 | ||
Booster (%) | Baseline | – | – | ||
3 months | 4.6 ± 1.6 | 7.1 ± 2.1 | 0.0002 | ||
12 months | 5.1 ± 1.8 | 6.9 ± 2.9 | 0.08 | ||
Anterior | S′ (cm/second) | Baseline | 8.2 ± 2.3 | 6.4 ± 1.7 | 0.003 |
3 months | 6.6 ± 2.4 | 5.5 ± 1.9 | 0.08 | ||
12 months | 6.8 ± 2.8 | 6.9 ± 2.6 | 0.86 | ||
Reservoir (%) | Baseline | 7.1 ± 2 | 6.4 ± 1.6 | 0.19 | |
3 months | 6.9 ± 2 | 7.5 ± 1.5 | 0.28 | ||
12 months | 7.3 ± 1.8 | 8.1 ± 1.7 | 0.19 | ||
Conduit (%) | Baseline | 12 ± 3.6 | 10.2 ± 3.8 | 0.13 | |
3 months | 8.5 ± 2.8 | 8.9 ± 2.1 | 0.75 | ||
12 months | 8.6 ± 2.1 | 8.9 ± 2 | 0.78 | ||
Booster (%) | Baseline | – | – | – | |
3 months | 4.6 ± 1.6 | 7.8 ± 2.3 | 0.001 | ||
12 months | 5.1 ± 1.8 | 7.8 ± 2.5 | 0.004 | ||
Inferior | S′ (cm/second) | Baseline | 8.8 ± 3.1 | 7.1 ± 2.4 | 0.04 |
3 months | 7.5 ± 2.8 | 7.6 ± 2.4 | 0.97 | ||
12 months | 8.4 ± 2.5 | 8.2 ± 2.7 | 0.77 | ||
Reservoir (%) | Baseline | 6.1 ± 1.6 | 6.3 ± 1.4 | 0.67 | |
3 months | 6.3 ± 1.8 | 6.9 ± 1.6 | 0.21 | ||
12 months | 6.9 ± 2.1 | 8.2 ± 1.2 | 0.008 | ||
Conduit (%) | Baseline | 9 ± 2.4 | 8.7 ± 3.5 | 0.72 | |
3 months | 7 ± 2.2 | 7.1 ± 1.3 | 0.83 | ||
12 months | 7 ± 2.1 | 7.4 ± 1.9 | 0.91 | ||
Booster (%) | Baseline | – | – | – | |
3 months | 6.5 ± 2.2 | 7.8 ± 2 | 0.06 | ||
12 months | 6.9 ± 2.4 | 8.2 ± 2 | 0.07 |
Baseline characteristic | Treatment arm | |
---|---|---|
CA (n = 45) | SA (n = 38) | |
Age (years), mean ± SD | 62.3 ± 10.1 | 63.1 ± 9.8 |
Sex (male), n (%) | 33 (73.3) | 28 (73.6) |
AF duration (days), mean (95% CI) | 575 (429 to 848) | 686 (495 to 1053) |
BMI (kg/m2), mean ± SD | 30.7 ± 4.9 | 29.6 ± 4.1 |
Smoking, n (%) | 3 (6.7) | 4 (10.5) |
Hypertension, n (%) | 21 (46.7) | 20 (52.6) |
Diabetes, n (%) | 4 (8.9) | 4 (10.5) |
Stroke, n (%) | 0 (0) | 2 (5.3) |
Coronary artery disease, n (%) | 7 (15.6) | 4 (10.5) |
Respiratory disease, n (%) | 4 (8.9) | 10 (26.3) |
Medication, n (%) | ||
Beta-blocker | 38 (84.4) | 29 (76.3) |
Digoxin | 14 (31.1) | 7 (18.4) |
Calcium channel blocker | 4 (8.9) | 7 (18.4) |
ACE/ARB | 23 (51.1) | 18 (47.4) |
Diuretic | 12 (26.7) | 12 (31.6) |
AADs | 5 (11.1) | 6 (15.8) |
Glossary
- CHA2DS2-VASc
- A tool to estimate risk of stroke in atrial fibrillation.
- HAS-BLED
- A tool to estimate the risk of major bleeding for patients on anticoagulation to assess risk–benefit in atrial fibrillation care.
List of abbreviations
- 2D
- two-dimensional
- 3D
- three-dimensional
- A′
- late atrial myocardial diastolic velocity
- AAD
- antiarrhythmic drug
- AF
- atrial fibrillation
- AFEQT
- Atrial Fibrillation Effect on QualiTy-of-life
- ASR′
- two-dimensional left atrial contractile strain rate
- AT
- atrial tachycardia
- AUC
- area under the curve
- BMI
- body mass index
- CA
- catheter ablation
- CASA-AF
- Catheter Ablation versus thoracoscopic Surgical Ablation in long-standing persistent Atrial Fibrillation
- CI
- confidence interval
- CMRI
- cardiac magnetic resonance imaging
- CT
- computerised tomography
- DCCV
- direct-current cardioversion
- DE-MRI
- delayed enhancement magnetic resonance imaging
- DMC
- Data Monitoring Committee
- ECG
- electrocardiography
- EF
- ejection fraction
- EHRA
- European Heart Rhythm Association
- EP
- electrophysiology
- EQ-5D
- EuroQol-5 Dimensions
- EQ-5D-5L
- EuroQol-5 Dimensions, five-level version
- ESR′
- two-dimensional left atrial conduit strain rate
- GLM
- generalised linear model
- GP
- ganglionic plexus
- HR
- heart rate
- HRG
- Healthcare Resource Group
- HRQoL
- health-related quality of life
- IC
- incremental cost
- IE
- incremental effect
- ILR
- implanted loop recorder
- IMD
- Index of Multiple Deprivation
- INB
- incremental net benefit
- IQR
- interquartile range
- LA
- left atrium
- LAA
- left atrial appendage
- LAD
- left atrial diameter
- LAmax
- left atrial maximal volume
- LAmin
- left atrial minimal volume
- LGE
- late gadolinium enhancement
- LOS
- length of stay
- LSPAF
- long-standing persistent atrial fibrillation
- LV
- left ventricle
- MI
- multiple imputation
- MICE
- multivariate imputation via chained equations
- MRI
- magnetic resonance imaging
- MVTT
- multiview two-task segmentation
- NICE
- National Institute for Health and Care Excellence
- NOAC
- novel oral anticoagulant
- OLS
- ordinary least squares
- OR
- odds ratio
- PAG
- Patient Advisory Group
- PIS
- participant information sheet
- PMM
- predictive mean matching
- PV
- pulmonary vein
- PVI
- pulmonary vein isolation
- QALY
- quality-adjusted life-year
- RA
- right atrium
- RAmax
- right atrial maximal volume
- RAmin
- right atrial minimal volume
- RCT
- randomised control trial
- ROC
- receiver operating characteristic
- RR
- relative risk
- RRM
- reverse remodelling
- S′
- mitral annular velocity during onset of ventricular systole
- SA
- surgical ablation
- SAE
- serious adverse event
- SD
- standard deviation
- SPIRIT
- Standard Protocol Items: Recommendations for Interventional Trials
- SR
- sinus rhythm
- SSR′
- two-dimensional left atrial reservoir strain rate
- TACT
- total atrial conduction time
- TDI
- tissue Doppler imaging
- TE
- echo time
- TOE
- transoesophageal echocardiography
- TR
- repetition time
- TSC
- Trial Steering Committee
- TTE
- transthoracic echocardiogram
- VAS
- visual analogue scale