Notes
Article history
The research reported in this issue of the journal was funded by the EME programme as award number 12/66/35. The contractual start date was in August 2015. The draft manuscript began editorial review in October 2023 and was accepted for publication in December 2023. The authors have been wholly responsible for all data collection, analysis and interpretation, and for writing up their work. The EME editors and production house have tried to ensure the accuracy of the authors’ manuscript and would like to thank the reviewers for their constructive comments on the draft document. However, they do not accept liability for damages or losses arising from material published in this article.
Permissions
Copyright statement
Copyright © 2024 Cehajic-Kapetanovic et al. This work was produced by Cehajic-Kapetanovic et al. under the terms of a commissioning contract issued by the Secretary of State for Health and Social Care. This is an Open Access publication distributed under the terms of the Creative Commons Attribution CC BY 4.0 licence, which permits unrestricted use, distribution, reproduction and adaptation in any medium and for any purpose provided that it is properly attributed. See: https://creativecommons.org/licenses/by/4.0/. For attribution the title, original author(s), the publication source – NIHR Journals Library, and the DOI of the publication must be cited.
2024 Cehajic-Kapetanovic et al.
Chapter 1 Introduction
Background
Choroideremia is an inherited retinal disease, affecting approximately 1 in 50,000 people, which presents with the same symptoms as a rod–cone dystrophy – beginning in childhood with nyctalopia, followed by gradual loss of peripheral vision and progressive constriction of visual fields, and ultimately resulting in loss of central vision in late adulthood once the degeneration affects the fovea. Choroideremia is caused by null mutations in the CHM gene located on the X-chromosome, and thus has an X-linked recessive mode of inheritance. Males are therefore severely affected by this disease, whereas female carriers present with a range of phenotype severities dependant on the extent and timing of X-chromosome inactivation. 1–3
As the central cone photoreceptors are generally preserved until the late stages of choroideremia, due to the centripetal nature of the degeneration, patients usually retain good visual acuity until degeneration encroaches on the fovea. 4–7 Fundus examinations of patients with choroideremia reveal characteristic centripetal retinal degeneration (Figure 1).
FIGURE 1.
Retinal degeneration in a moderately advanced choroideremia patient. (a) Fundus photograph showing the characteristic centripetal retinal degeneration and (b) fundus autofluorescence image showing the autofluorescent areas of the surviving retinal pigment epithelium and remaining functional retina.
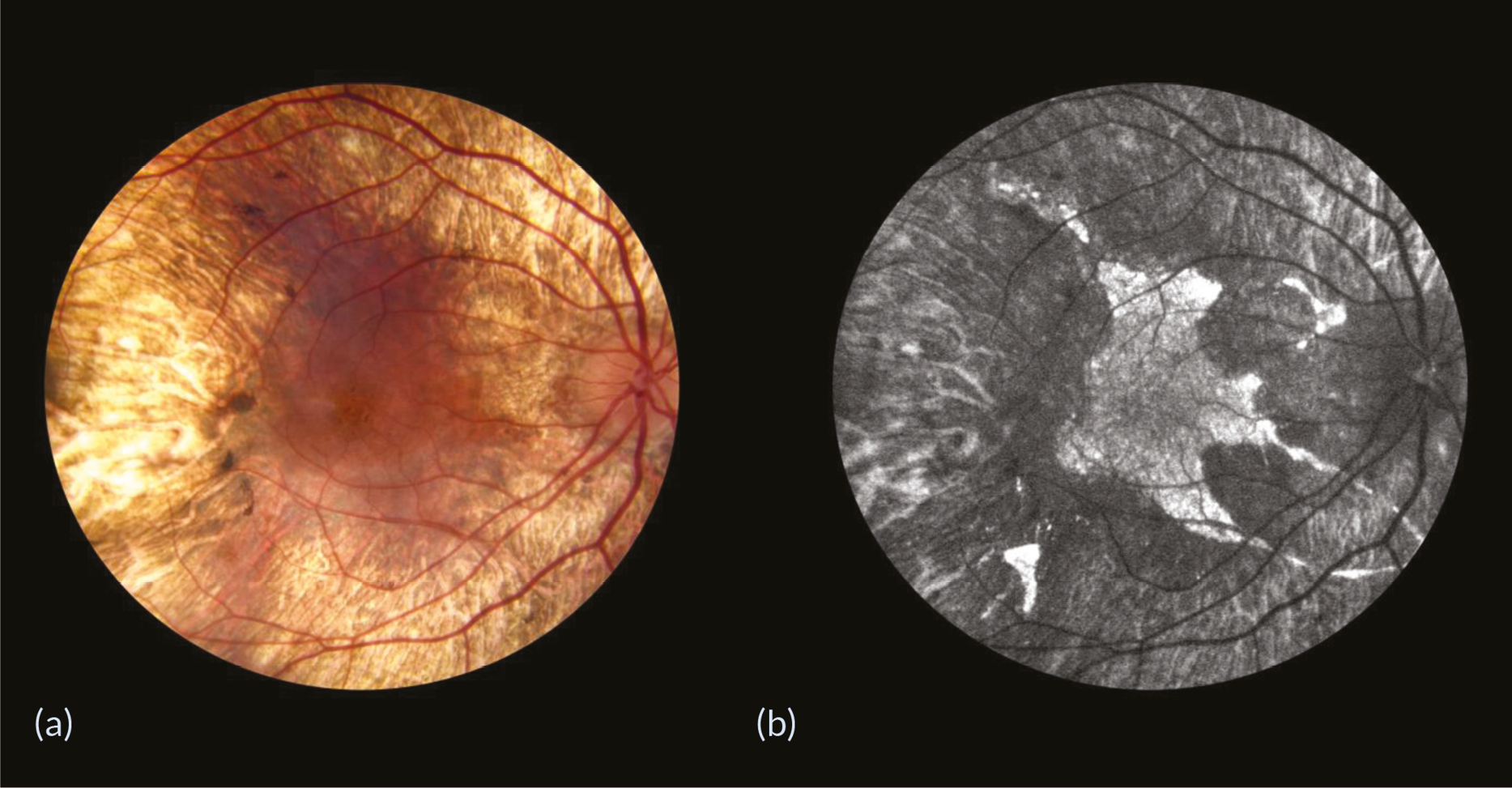
The CHM gene encodes Rab escort protein 1 (REP1), which facilitates intracellular vesicular trafficking. Null mutations in the CHM gene cause a deficiency of REP1 protein, leading to degeneration of the retinal pigment epithelium (RPE), followed by secondary degeneration of photoreceptors and the choroid. 8,9 REP1 mediates the addition of prenyl groups (prenylation) to Rab GTPases, which are needed to anchor the Rabs to the membranes of intracellular organelles and vesicles to enable intracellular trafficking between the retina and RPE. 10 In choroideremia, the absence of functional REP1 causes impaired Rab membrane association. This subsequently leads to impaired intracellular trafficking that disrupts cell homeostasis and causes accumulation of intracellular deposits in the RPE, resulting in retinal degeneration. 11–13 Degeneration primarily occurs in the RPE causing characteristic pigment clumping, before secondary degeneration of photoreceptors and the choroid. 13–15 The disease presents as a rod–cone degeneration, since rods are more vulnerable to RPE degeneration due to their reliance on RPE visual cycle retinal isomerisation. Cones may survive longer because their oxygen requirements from the residual choroidal circulation are less and Mueller cells may compensate for RPE cell deficiency and partly sustain the cone visual cycle. 13,14
Rationale
Gene therapy, a procedure whereby a disease is treated at the genetic level by intracellular delivery of a therapeutic transgene, is an appealing strategy for treating choroideremia. The slow rate of degeneration means that there is a long therapeutic window before cell death occurs. In addition, virtually all cases reported so far are functionally null mutations and are often predicted to result in the severe truncation or absence of endogenous REP1 protein. 13,16 This is useful because the product of the therapeutic gene will not compete with a large pool of mutant protein. This would limit dominant negative effects and, in theory, would mean that only a small amount of functional REP1 protein would be needed to arrest degeneration. 17
Furthermore, the eye is an attractive site for gene therapy for several reasons. Surgical access is relatively straightforward and gene therapy products can be administered directly to cells in the outer retina (photoreceptors and RPE) by subretinal injection. Potential adverse events can be detected and monitored directly via ocular examination. Therapeutic outcomes can be measured directly using non-invasive assessments of visual function and retinal anatomy, with an untreated contralateral eye available as a control. The risk of systemic immune reactions is reduced by the anatomical compartmentalisation of the eye and the immunological privilege provided by the blood–retina barrier. Moreover, the small tissue volume of the subretinal space means that the therapeutic dose required to treat the retina is several thousand times lower in comparison with doses required for treatment of other organs, further reducing the risk of systemic adverse reactions.
Most gene therapies developed thus far as potential treatments for inherited retinal diseases have utilised recombinant adeno-associated virus (AAV) particles as vectors to deliver therapeutic transgenes to target cells in the retina. 18 Different AAV serotypes have slight differences in their capsid proteins and consequently in their cell selectivity. AAV serotype 2 (AAV2) has shown high affinity for photoreceptors and RPE cells. 19 Therapeutic administration is effected by injection of a suspension of AAV2 vector particles into the subretinal space, which are subsequently taken up by photoreceptors and RPE cells. Voretigene neparvovec (Luxturna®), an AAV2 vector-based gene therapy for treatment of RPE65-associated inherited retinal disease manufactured by Hoffmann-La Roche AG (Basel, Switzerland), became the first retinal gene therapy to be approved by the US Food and Drug Administration (FDA). 20 Following subsequent approval by the European Medicines Agency (EMA),21 Luxturna is now available as an NHS treatment. 22
This gene therapy for choroideremia uses an AAV2 vector containing the coding sequence for human REP1 protein, regulated by a ubiquitous cytomegalovirus-enhanced chicken β-actin promoter and a woodchuck hepatitis virus post-transcriptional regulatory element (WPRE). The vector, designated as AAV2-REP1, was first tested in human subjects in a phase I/II safety and dose escalation study (NCT01461213) at Oxford (UK). A cohort of six participants was initially treated with a dose of up to 1 × 1010 vector particles administered subretinally in one eye, followed by a cohort of eight participants treated with a higher dose of up to 1 × 1011 vector particles. Initial results were promising with gains in best corrected visual acuity (BCVA) of 11 and 21 letters recorded in the treated eyes of two participants at 6 months post treatment. 17 Two serious adverse events (SAEs) occurred during the clinical trial, comprising a case of retinal thinning due to surgical complications and a case of postoperative inflammation, but otherwise the study data confirmed the safety and possible efficacy of the AAV2-REP1 vector for treatment for choroideremia. 23
An additional 18 choroideremia patients were subsequently treated with a dose of up to 1 × 1011 particles of the same AAV2-REP1 vector administered subretinally in one eye – 6 participants in a phase I/II study (NCT02077361) at Edmonton (Canada), 6 participants in a phase II study (NCT02553135) at Miami (USA) and 6 participants in a phase II study (NCT02671539) at Tübingen (Germany). These studies showed that the AAV2-REP1 vector was generally well tolerated, with a subset of participants achieving clinically significant improvements in BCVA. 24–26 One SAE involving a case of postoperative inflammation was reported in the Edmonton study, resulting in a loss of BCVA (nine letters) in the treated eye of the relevant participant, but it was also reported that surgery had been difficult in the case of this participant, for whom subretinal air and haemorrhage had complicated the subretinal administration of the AAV2-REP1 vector. There were no cases of postoperative inflammation reported in the 12 choroideremia patients treated in Tübingen and Miami. In the Tübingen study, a pre-existing degenerative macular hole opened up in one of the participants during surgery but subsequently closed spontaneously. In the Miami study, two participants developed an atrophic retinal hole in a non-functioning macular area.
In view of these satisfactory clinical outcomes, which indicated that gene therapy for choroideremia using the AAV2-REP1 vector demonstrated a good safety profile and sustained gains in BCVA in a proportion of trial participants,27 the REGENERATE (REP1 gene replacement therapy) phase II study (NCT02407678) was initiated to assess the efficacy of gene therapy (dose of up to 1 × 1011 particles of the AAV2-REP1 vector administered subretinally in one eye) in 30 choroideremia patients at an earlier stage of the disease and therefore still possessing substantial areas of surviving retinal tissue, thereby facilitating the measurement of area changes in order to assess the effect of gene therapy on retinal deterioration.
Another biotechnology company, Spark Therapeutics (Philadelphia, PA, USA), now owned by Hoffmann-La Roche AG (Basel, Switzerland), has initiated a phase I/II safety and dose escalation study (NCT02341807) of a similar AAV2 vector construct which does not contain WPRE. 28 Fifteen choroideremia patients were recruited, of whom 5 participants were treated with a dose of up to 5 × 1010 vector genomes administered subretinally in one eye, and 10 participants were treated with a higher dose of up to 1 × 1011 vector particles. BCVA returned to within 15 letters of baseline in all but 2 patients (of whom 1 developed acute foveal thinning and the other developed a macular hole). No incidents of intraocular inflammation were reported.
Aim and objectives
The aim of the REGENERATE study was to investigate the efficacy and safety of a single subretinal injection of AAV2-REP1 in participants with a confirmed diagnosis of choroideremia over an assessment period of 24 months post treatment.
Primary objective
The primary safety-related outcome was change from baseline in BCVA in treated eyes at 24 months post treatment, with prospective efficacy evaluated by comparative change from baseline in BCVA in treated and untreated contralateral (control) eyes.
Secondary objectives
-
Comparative change from baseline in central visual fields and mean retinal sensitivity (determined by mesopic microperimetry) in treated and control eyes at 24 months post treatment
-
Comparative change from baseline in the area of surviving RPE (determined by fundus autofluorescence) in treated and control eyes at 24 months post treatment
-
Comparative change from baseline in other measures of visual function and retinal anatomy in treated and control eyes at 24 months post treatment
-
Change from baseline in safety-related immunological and physiological indicators
Chapter 2 Methods
Study design
The REGENERATE study is an open-label Phase II clinical trial investigating the efficacy and safety of AAV2-REP1 vector-mediated gene therapy for treatment of choroideremia.
In contrast to other interventional studies, the REGENERATE study recruited choroideremia patients at an earlier stage of the disease and therefore still possessing substantial areas of surviving retinal tissue. The intention behind this study design was to facilitate the measurement of changes in area of surviving RPE (determined by fundus autofluorescence) in order to assess the effect of gene therapy on retinal deterioration.
Whereas in changes in BCVA and other measures of visual function in the treated eye could be compared against baseline values, assessment of the anatomical rate of degeneration ideally required the untreated contralateral eye to be used as an internal control. This condition required, in turn, the inclusion of participants having a fairly symmetrical disease. In consequence, randomisation of treatment of one eye or the other was also required to avoid selection bias.
Equality, diversity and inclusion
According to the 2021 Census, the total population of England and Wales was 59.6 million, broadly composed of the following ethnic groups: 81.7% white (48.7 million), 9.3% Asian (5.5 million) and 9.0% black/mixed/other (5.4 million). As choroideremia is a rare disease affecting approximately 1 in 50,000 people, it was therefore anticipated that the pool of prospective participants for the REGENERATE study consisted of approximately 1000 white, 100 Asian and 100 black/mixed/other choroideremia patients.
In view of the significant challenges in achieving the recruitment target of the REGENERATE study considering the limited pool of choroideremia patients meeting the inclusion criteria, it was not possible to arrange special provisions for recruitment of ethnically diverse trial participants representative of the overall demographic profile of the country.
Patient and public involvement
Choroideremia patients were invited to help with writing patient information sheets, including their feedback on suitable fonts and layouts for sight-impaired persons. The medical charity Fight for Sight was also approached for help in public communication of the aims and objectives of the REGENERATE trial and how choroideremia patients could get involved.
Fight for Sight also co-ordinated a fundraising campaign with the assistance of the Tommy Salisbury Choroideremia Fund, which raised funds for the purchase of an ophthalmic operating microscope with an integrated optical coherence tomography (OCT) scanner for use in the REGENERATE study. OCT operating microscopes have an integrated scanner providing a head-up display of a cross-section of the retina in real time for the surgeon, thereby permitting subretinal delivery of the vector to be achieved more safely and accurately.
Sample size
As this was an exploratory study to assess a new end point (comparative change from baseline in the area of surviving RPE in the treated and control eyes, determined by fundus autofluorescence), there was no predetermined power calculation to determine the number of trial participants. Instead, data from this trial will be used for future power calculations. A sample size of 30 participants in the REGENERATE study was deemed sufficiently statistically powered to show a signal in the primary end point of efficacy (comparative change from baseline in BCVA in the treated and control eyes) based on power calculations from the original phase I/II study (NCT01461213).
Study set-up
The REGENERATE study was listed on the U.S. National Library of Medicine ClinicalTrials.gov database on 3 April 2015: National Clinical Trial identifier (NCT02407678). The study was also registered in the International Standard Randomised Controlled Trial Number (ISRCTN) registry on 18 April 2016: reference ISRCTN15602229. Clinical trial authorisation was given by the Medicines and Healthcare products Regulatory Agency on 11 November 2015: European Union Drug Regulating Authorities Clinical Trials database number 2015-001383-18. Recruitment commenced on 16 August 2016 and closed on 23 May 2019.
Independent oversight of the REGENERATE study was provided by the Trial Steering Committee that received recommendations from the independent Data and Safety Monitoring Committee. (The members of the trial oversight committees are listed in Appendix 2.)
Study setting
The REGENERATE study was conducted at two NHS eye hospitals, namely, the Oxford Eye Hospital and Moorfields Eye Hospital. These sites were selected based on their expertise and prior experience in conducting clinical trials of retinal gene therapies and their access to specialist clinics for patients with inherited retinal diseases.
Protocol
The REGENERATE study protocol is available at https://fundingawards.nihr.ac.uk/award/12/66/35. The REGENERATE study commenced recruitment on protocol version 2.0, dated 19 October 2015. A number of updates and minor corrections were made to the protocol during the course of the study:
-
Version 3.0, dated 14 November 2016, included a change of principal investigator at the Moorfields Eye Hospital site, clarifications of the randomisation and informed consent procedures, an amendment to the interval between screening and surgery, amendments to the schedule of assessments (full ophthalmic examination, viral shedding, immunology and colour vision) and a clarification of the procedures for recording and managing study data.
-
Version 4.0, dated 4 January 2017, included amendments to the schedule of assessments (vital signs, blood chemistry, immunology, fundus photography and refraction/BCVA).
-
Version 5.0, dated 15 March 2017, included an amendment to the inclusion criterion for BCVA such that candidates must have BCVA better than or equal to 6/60 (20/200; LogMAR 1.0).
-
Version 6.0, dated 4 October 2017, included amendments extending the oral prednisolone course and permitting administration of 0.2 ml of the AAV2-REP1 vector.
-
Version 7.0, dated 8 July 2020, included updates to the safety reporting section to include parameters for determining the clinical significance of changes in BCVA.
Participants
Eligibility for recruitment
All participants were male with a clinical phenotype of choroideremia and a confirmed diagnosis. (As choroideremia is an X-linked recessive disease, males are severely affected, whereas female carriers present with a range of phenotype severities dependent on the extent of X-chromosome inactivation.)
Confirmation of a diagnosis of choroideremia preceded the invitation of a candidate to participation in the study and to the initial screening visit. Confirmation of diagnosis included:
-
provision of demographic, medical and ocular history;
-
a characteristic clinical phenotype of choroideremia apparent from full ophthalmic examination, retinal imaging and visual function tests;
-
genetically confirmed diagnosis of choroideremia.
Deoxyribonucleic acid sequencing of candidates’ CHM genes was undertaken by specialist genetics laboratories.
Note that the study protocol made provision for recruitment of candidates without a confirmed mutation in the REP1 coding sequence (i.e. the exonic regions of the CHM gene encoding REP1 protein), but who had the clinical phenotype typical of choroideremia confirmed by a minimum of three independent retinal specialists, and additionally met the following three criteria:
-
Family history consistent with X-linked inheritance. Since choroideremia has a distinct clinical appearance compared with other types of retinal degeneration and an X-linked inheritance, the appropriate family history would provide strong evidence that a candidate had choroideremia even though a mutation in the CHM gene could not be detected. (Note that absence of functional REP1 protein would still need to be confirmed as detailed in the following criterion.)
-
Absence of functional REP1 protein. Despite being unable to find a mutation in (the exons of) the CHM gene, an absence of functional REP1 protein in a candidate could be caused by other factors such as a mutation within an intron affecting splicing of messenger ribonucleic acid expressed by the CHM gene, or a mutation in the CHM promoter region. Since the REP1 protein is expressed ubiquitously, deficiency of the CHM gene product could be confirmed by screening peripheral blood for REP1 protein by a western blot, and absence of REP1 function could be assessed through a prenylation assay. The absence of functional REP1 protein would provide a molecular confirmation of the clinical diagnosis of choroideremia.
-
Presence of a normal RPE65 gene. The RPE65 gene codes for RPE-specific 65 kDa protein. Mutations in RPE65 have been associated with Leber congenital amaurosis type 2 and retinitis pigmentosa. Early in the course of the disease, choroideremia may share similar features with RPE65-associated disease, such as nyctalopia and circumferential loss of RPE. However, differences emerge in time as the disease progresses.
Inclusion criteria
Inclusion criteria comprised:
-
candidate willing and able to give informed consent for participation in the study;
-
male aged 18 years or above;
-
genetic or molecular confirmed diagnosis of choroideremia;
-
active disease visible clinically within the macula region;
-
BCVA better than or equal to 6/60 (20/200; LogMAR 1.0) in the study eye.
Exclusion criteria
Exclusion criteria comprised:
-
any female, or male, aged below 18 years;
-
an additional cause for sight loss (e.g. amblyopia);
-
any other significant ocular and non-ocular disease or disorder which, in the opinion of the investigator, might put candidates at risk through participation in the study;
-
inability to take systemic prednisolone for a period of 45 days;
-
unwillingness to use barrier contraception methods for a period of 3 months following administration of gene therapy;
-
participation in another research study involving an investigational product in the preceding 12 weeks.
Allocation for treatment
Participants in the REGENERATE study were assigned to one or both of the following cohorts:
-
Cohort 1 included all participants and compared changes in BCVA and other measures of visual function in the treated eye against baseline values.
-
Cohort 2 included the subset of participants having symmetrical disease for whom selection of the treated eye was randomised and compared the rate of anatomical degeneration in the treated eye and the untreated contralateral (control) eye.
In participants having asymmetrical disease (and therefore not included in Cohort 2), the decision about which eye to treat was made on clinical grounds and the worse-affected eye was chosen.
Note that randomisation was not used for assigning treatment (vs. placebo/standard care as in randomised controlled trials) to participants included in Cohort 2, but solely for selection of the eye to be treated in these participants for whom the progress of retinal degeneration was relatively symmetrical between the two eyes, defined as
-
a difference in BCVA of no more than one line of letters, as measured on an Early Treatment Diabetic Retinopathy Study (ETDRS) chart; and
-
no more than 25% difference in the area of surviving RPE, as measured by fundus autofluorescence.
Block randomisation was achieved by creating a numbered list on a Microsoft Excel® (Microsoft Corporation, Redmond, WA, USA) spreadsheet and using the RANDBETWEEN function to generate a value of zero or one in a random fashion, which was assigned to each number on the list. A value of zero indicated that the right eye was to be treated and a value of one indicated that the left eye was to be treated. Participants in Cohort 2 were then assigned an appropriate number and corresponding randomised value.
Intervention
Adeno-associated virus serotype 2 vector encoding Rab escort protein 1 vector suspension (1 × 1012 vector particles per ml) was supplied by Nightstar Therapeutics (London, UK), now part of Biogen Inc. (Cambridge, MA, USA). Up to 0.1 ml of AAV2-REP1 vector suspension, corresponding to a dose of up to 1 × 1011 vector particles, was administered to the treated eye by subretinal injection. The dose varied slightly dependent on the amount of residual retina. Hence, in more advanced patients, 0.03–0.05 ml might have been sufficient to detach the target area of retina completely. The concentration of vector remained the same.
The surgical technique for subretinal administration of the AAV2-REP1 vector suspension has been described previously. 17 All surgeries took place at the participating hospital sites using the standard BIOM® (binocular indirect ophthalmic microscope) operating system. 29 Following a standard vitrectomy to remove the vitreous gel (Figure 2a), the retina was detached with 0.1–0.5 ml of balanced salt solution injected through a subretinal cannula connected to a vitreous injection set. A dose of up to 1 × 1011 vector particles was then injected into the subretinal fluid through the same entry site (Figure 2b).
FIGURE 2.
Surgical procedure for subretinal injection of AAV2-REP1 vector. (a) A standard vitrectomy through an operating system to remove the vitreous gel is followed by (b) injection of vector suspension into the subretinal space.
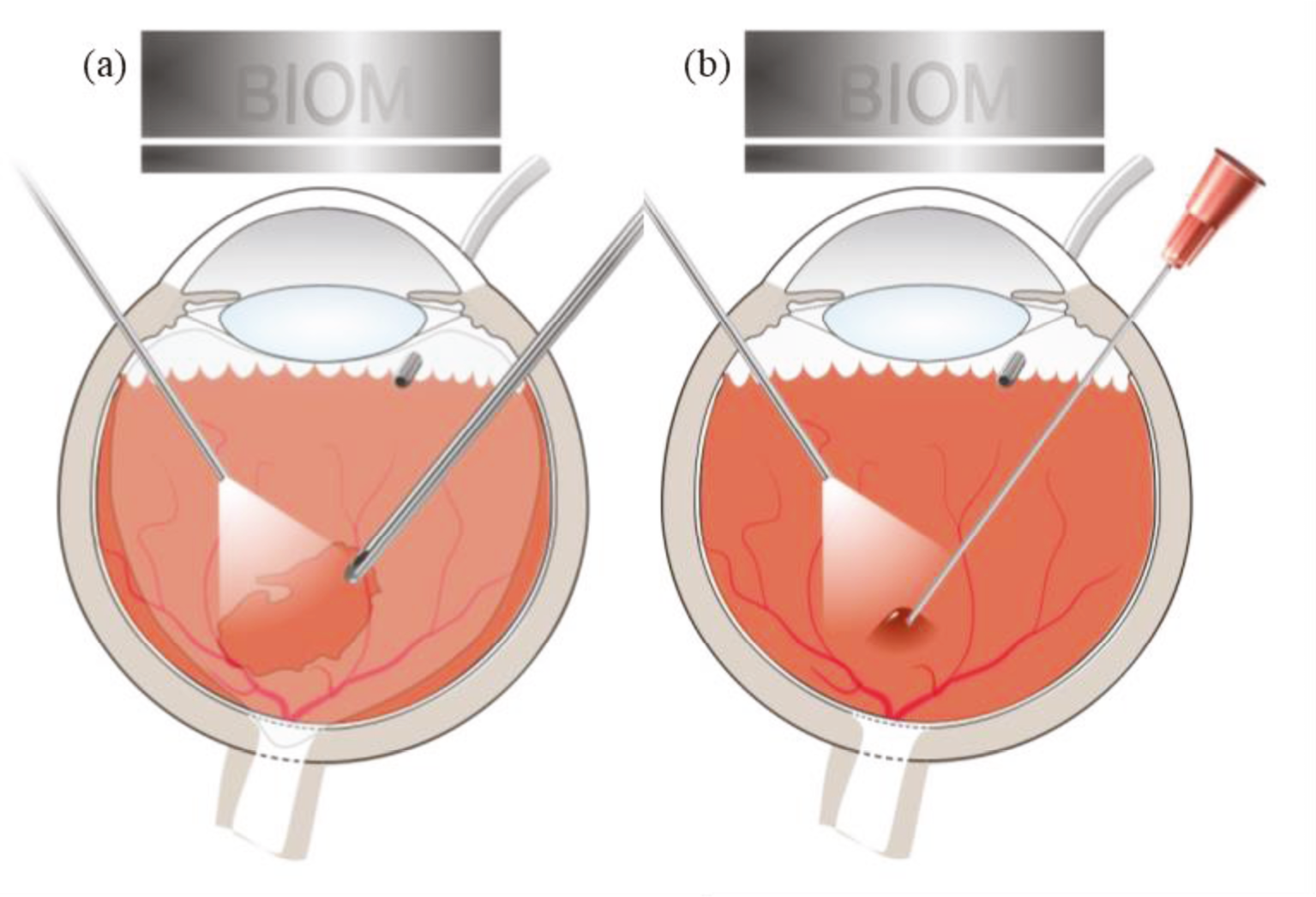
Control eyes received no intervention.
Assessments
Data collection and follow-up schedule
The schedule of assessments is shown in Table 1. Functional assessments included BCVA, contrast sensitivity, dark adaptometry and central visual field mapping (using microperimetry), while anatomical assessments included imaging techniques such as OCT and fundus autofluorescence. Safety tests included assessments of ocular and general physical condition, as well as standard blood tests of common physiological markers. Biological samples were also analysed to monitor vector shedding and immunogenicity.
Visit 1 | Visit 2 | Visit 3 | Visit 4 | Visit 5 | Visit 6 | Visit 7 | Visit 8 | Visit 9 | Visit 10 | Visit 11 | Early termination visit b | Unscheduled visit c | |
---|---|---|---|---|---|---|---|---|---|---|---|---|---|
Screeninga | Day 0 | Day 1 | Day 7, ± 3d | Month 1, ± 7d | Month 3, ± 7d | Month 6, ± 21d | Month 9, ± 21d | Month 12, ± 21d | Month 18, ± 28d | Month 24, ± 28d | |||
Informed consent | X | ||||||||||||
Demographic, medical and ocular history | X | ||||||||||||
Full ophthalmic examinationd | X | X | X | X | X | X | X | X | X | X | X | X | |
Prednisolone/omeprazole administratione | Administered daily from day −2 to day 42 | ||||||||||||
Randomisation of treated eye (if relevantf) | X | ||||||||||||
Administration of AAV2-REP1g | X | ||||||||||||
Vital signsh | X | X | X | X | |||||||||
Blood chemistryi | X | X | X | ||||||||||
Viral sheddingj | X | X | X | X | X | ||||||||
Immunologyk | X | X | X | X | |||||||||
Contrast sensitivity | X | X | X | X | X | ||||||||
Colour vision | X | X | X | X | X | ||||||||
Fundus photography | X | X | X | X | X | ||||||||
Refractive error + BCVA | X | X | X | X | X | X | X | X | X | X | X | ||
Optical coherence tomography + fundus autofluorescence | X | X | X | X | X | X | X | X | X | X | X | X | |
Microperimetryl | X | X | X | X | X | X | X | X | X | X | X | X | |
Perimetrym | X | X | X | ||||||||||
Angiographyn | X | X | X | ||||||||||
Adaptive optics imagingo | X | X | |||||||||||
Dark adaptation curve + full-field stimulus threshold + pattern electroretinography | X | X | |||||||||||
Monitoring of adverse events | X | X | X | X | X | X | X | X | X | X | X | X | X |
Concomitant medications | X | X | X | X | X | X | X | X | X | X | X | X | X |
Participant demographics and other baseline characteristics
Baseline characteristics details, such as age and genetic diagnosis, were retrieved from the patient and their hospital medical records.
Masking of assessors
In order to minimise investigator bias, visual assessments of all participants (whether or not they were included in Cohort 2) were conducted by masked assessors throughout the entire course of the study. Participants were advised not to disclose which eye had been treated to the relevant clinicians.
Assessments of functional outcomes
Refraction
Each participant underwent a refraction at each study visit, prior to BCVA testing, to obtain the most up-to-date spectacle correction using ETDRS chart R. 30 Full aperture lenses were used with a trial frame. The right eye was refracted first, prior to the left; the eye not being tested was covered by an occluder in the trial frame.
Best corrected visual acuity
The ETDRS chart was set at 4 m. With only the ETDRS letter chart light box was switched on (160 cd/m2), all other room luminance was either turned off or blocked out as per standard ETDRS testing procedures. 30 Following refraction, the BCVA was obtained for the right eye using the ETDRS chart 1. As participants read down chart 1 of the ETDRS chart, the letters were marked according to whether they were read correctly; the total number of letters seen correctly were counted, in accordance with ETDRS testing procedures. 30 Once BCVA testing was completed on the right eye, the occluders were swapped and the same procedure was repeated for the left eye, using the ETDRS chart 2.
Contrast sensitivity
Contrast sensitivity is the ability to discriminate an object from its background. Reduced contrast sensitivity can have a significant impact on mobility and ability to see to complete everyday tasks. Contrast sensitivity visual function was assessed using the Pelli–Robson chart. The test was performed prior to pupil dilation with the latest distance refraction correction.
The Pelli–Robson chart involves letters of reducing contrast, there are eight rows, each row has six letters, and the contrast decreases by a factor 1/√2 after every triplet. The chart was retro-illuminated using the ETDRS illuminator cabinet and placed at eye level, 1 m in front of the patient. Tested monocularly, right eye then the left eye, participants read down the chart as far as possible until they can no longer resolve any letters. The last triplet where 2/3 letters were read correctly was used as the log contrast sensitivity score.
Microperimetry
Microperimetry, also known as fundus-controlled perimetry, is a robust method for assessing global macular sensitivity. The test combines computerised central perimetry assessment with a scanning laser ophthalmoscope and real-time eye-tracking technology. This reduces errors that result from poor fixation and enable longitudinal assessment of specific retinal locations. Microperimetry has been shown to be a valid and repeatable assessment of central retinal sensitivity in patients with choroideremia. 4 Although high test–retest variability has been reported at degeneration borders and asymmetric central degeneration may skew mean sensitivity values, volumetric measures have been recommended for more reliable robust analyses. 31,32
Microperimetry assessment was undertaken using the MAIA microperimeter (CenterVue, Padova, Italy). Each participant underwent 20 minutes of dark adaptation (< 1 lux) prior to assessment. No pupil dilation was required. The 10-2 testing grid centred on the fovea was used. The 4-2 bracketing test strategy was used, with the Goldmann size III stimulus (duration 200 ms) presented against a mesopic background luminance of 1.27 cd/m2. The test covered a dynamic sensitivity range of 36 db. The right eye was tested first, then the left eye. Participants were encouraged throughout the test to ensure optimum attention and reliable results. If the participants had more than 15% fixation errors (positive responses to 10 dB stimuli presented in the blind spot), the test was repeated.
Pattern electroretinography
Pattern electroretinography (ERG) measures a retinal evoked response to a reversing black and white checkerboard using the Espion Visual Electrophysiology System (Diagnosys LLC, Littleton, MA, USA). It enables an objective functional assessment of the macular and retinal ganglion cell function. Pattern ERG was performed at baseline, month 12 and month 24, on each eye. The test was carried out in accordance with the published International Society for Clinical Electrophysiology of Vision (ISCEV) standards. 33 The test was performed prior to dilation with optimal spectacle refractive correction under normal room lighting; direct bright lights and glare were avoided. Testing was completed binocularly. Additional monocular testing was only performed if the participant had strabismus or evident eccentric fixation to help aid fixation.
Gold foil corneal electrodes were carefully positioned over the lower eyelids so that the electrode was in contact with the corneoscleral junction in the midline. Surface electrodes were applied after routine skin preparation and had an impedance of 5 kΩ or less between any pair of electrodes. The checkerboard stimulus comprising 50-minute checks in a 15 × 12-degree field (width and height, respectively) was generated using the cathode-ray tube screen. The luminance of the white squares was 100 cd/m2; contrast will be ≥ 98%. Stimulus reversal rate was 4.4 reversals/second. Additional testing to a 30 × 24-degree field was performed by reducing the distance to the screen by half and adjusting the check size accordingly. Analysis time was 150 ms; the filter bandwidth was 1–100 Hz (−3 dB). Line frequency notch filtering was not used, although artefact rejection was used to eliminate spuriously large signals generated from blinks and eye movements. The ‘interrupted stimulus’ technique was used to minimise small eye movements and blinking artefacts not detected with the automated artefact reject window. To ensure reliable waveform without excess noise several sweeps were averaged and repeated. The pattern ERG N35, P50 and N95 components were marked as per the ISCEV standards. 33
Colour vision
Colour vision was assessed using the Farnsworth–Munsell 100 hue test. The test was carried out monocularly (right eye then left eye) and prior to pupil dilation and clinical examination. The Farnsworth–Munsell 100 hue test was developed to determine colour discrimination. The test was conducted under a bright light source (≥ 270 lux), with a spectral distribution stimulating International Commission on Illumination (CIE) standard illuminance C condition, at 50 cm. Participants were required to arrange the tests coloured caps in a progressive colour change order. The sequence of the caps was then recorded and compared with the true sequence; the size of sequence difference at each cap was summed to generate an error score.
Perimetry
Kinetic perimetry using either the Goldmann or Octopus 900 machine was performed to assess overall retinal function within participants’ 180-degree visual field to produce a map indicating the extent of peripheral vision function. Patients with choroideremia typically have their discrete central island of vision, accompanied by one or more peripheral islands – optimally detected with perimetry. To assess this, testing was performed prior to any pupil dilation, in a darkened room. Each eye was tested monocularly (right eye then the left eye); the non-tested eye was occluded. Appropriate refractive correction was used. Both the III4e and V4e stimuli were used.
Dark adaptometry
Dark adaptometry is an automated test that enables the measurement of dark adaptation (the ability of the eye to change or recover its low light sensitivity after being exposed to bright light), producing a dark adaptation curve/timeline. The process is initially cone-dominated since cones take less time to recover sensitivity to lower light levels, before transitioning to rod-dominated retinal function. The test was performed monocularly (right eye then left eye) following pupil dilation using the Espion system. The room light was switched off and efforts made to minimise light leakage from electronic sources. The light adaptation included a 5-minute bleaching period. The participants were then presented with a series of single lights of varying intensity presented at the back of the light bowl. Participants indicated using the button box whether the light was seen. Throughout testing to minimise fatigue, a series of rest periods were included. The test lasted a minimum of 25 minutes. The end point is determined when the sensitivity curve reaches its plateaux; this was determined by five consecutive points of equal sensitivity. The test was stopped once this was determined.
Full-field stimulus threshold testing
Full-field stimulus threshold (FST) testing assesses global retinal sensitivity to different colours following dark adaptation allowing for maximum retinal sensitivity assessment. It was measured using the Espion system using a standardised FST testing protocol. It was performed monocularly (right eye and then left eye), following pupil dilation. Lights of different intensities were presented in the Espion Colourdome; participants then indicated whether they saw the light flash or not. This continued until a threshold measurement was obtained. White light was tested first, followed by the red, green and blue colour options. A 3-minute dark adaptation recovery time was allowed between tests repeats. Each colour test was repeated twice for each eye. The highest sensitivity threshold achieved (in decibels) for each colour, per eye, was recorded.
Assessments of anatomical outcomes
Optical coherence tomography
Macular spectral-domain OCT (30 degree) scans were taken using the Heidelberg Spectralis® (HRA + OCT) confocal scanning laser ophthalmoscope (Heidelberg Engineering GmbH, Heidelberg, Germany) at each visit on each eye following pupil dilation (with 1% tropicamide and 2.5% phenylephrine) to enable assessment of macular health. OCT facilitates visualisation of the retinal layers and RPE to enable accurate monitoring of the disease and integrity of the central remaining visual island observed in choroideremia patients.
Fundus autofluorescence
Fundus autofluorescence images were taken also using the Heidelberg Spectralis (HRA + OCT) confocal scanning laser ophthalmoscope (Heidelberg Engineering GmbH, Heidelberg, Germany) with an excitation filter of 488 nm. The fundus autofluorescence images were recorded with automatic real time (ART) mode 100 or the highest possible ART number excluding motion artefact using the 30- and 55-degree lenses following pupil dilation. The Heidelberg Eye Explorer (HEYEX) software was used to view the images and identify the autofluorescent central ‘island’ of residual functional retina, surrounded by areas of degeneration. The HEYEX software area tool was used to trace the area of residual autofluorescence to enable monitoring of progression. 34
Adaptive optics imaging
Adaptive optics imaging enables direct visualisation of individual cone photoreceptors, the cone photoreceptor mosaic, retinal pigment epithelial cells and retinal white blood cells. 35 An adaptive optics scanning laser ophthalmoscope (rtx1™; Imagine Eyes, Orsay, France) was used to obtain photoreceptor images. The device uses a 4-degree by 4-degree imaging field of view (1.2 × 1.2 mm at the retina) and a non-coherent flood-illuminated design, with a focus range of 600 μm. A low-noise camera with a pixel resolution of 1.6 μm and a 9.5 fps frame rate was used to obtain high-resolution cone and cone mosaic images. Adaptive optics images were obtained at baseline and month 24 only, following pupil dilation.
Safety assessments
Full ophthalmic examination
A full ophthalmic exam was undertaken at each visit; this included a medical and ocular history, a pupil examination, anterior and posterior segment examination using slit-lamp biomicroscopy and intraocular pressure measurement using Goldmann contact tonometry.
Vital signs
An assessment of the participants’ physical condition including resting pulse rate and blood pressure was performed at screening, day 1 after surgery and month 1.
Indocyanine green angiography and fundus fluorescein angiography
Participants were injected first with 0.5% indocyanine green. High-speed 55-degree images capturing the whole disc and macular view were taken using the Spectralis® HRA system (Heidelberg Engineering GmbH, Heidelberg, Germany). The images were taken at set intervals following the imaging procedure. After 1 minute, the procedure was repeated with 10% or 20% fluorescein solution to obtain the fundus fluorescein angiography images.
Biological samples
Venepuncture blood samples were collected from each participant at predetermined time points in accordance with local clinical procedures. The Vacutainer® system (BD: Becton, Dickinson and Company, Franklin Lakes, NJ, USA) was used with as large as possible gauge hypodermic needle (i.e. 21G) to prevent haemolysis.
-
Some samples were used to assess blood biochemistry and haematological composition.
-
Some samples were processed and cryopreserved so that they could be later used to assess shedding of the gene therapy vector.
-
Other samples were processed to collect specific blood fractions and cells for use in immunological tests. These samples included serum for enzyme-linked immunosorbent assays and peripheral blood mononuclear cells for enzyme-linked immunosorbent spot assays; these were cryopreserved until needed.
Vector shedding
Vector shedding is the dissemination of the virus vector following gene therapy through secretions or excreta of the trial participant. Tears, urine and saliva samples as well as blood and its related products were collected for vector shedding assessment at specific time points, as per the schedule outlined in Table 1. The assessment used polymerase chain reaction assays to assess presence and levels of the virus vector. This enabled understanding of any potential risk associated with transmission to third parties and the environment.
Safety reporting
Reporting procedures
All adverse events and SAEs, observed by the investigator or reported by the participant within the 24-month assessment period following administration of gene therapy, were recorded.
Categorisation of decreases in visual acuity as adverse events
The ETDRS chart, used to measure BCVA in the REGENERATE study and preceding interventional studies evaluating AAV2-REP1-mediated gene therapy for choroideremia, is a logarithm of the minimum angle of resolution (logMAR) chart.
Each line of five letters on the ETDRS chart is equivalent to 0.1 logMAR. This is the log10 value of the change in minutes of arc resolution. A drop in BCVA of 0.3 logMAR (3 lines/15 letters) represents a doubling of the visual angle, as log102 equals 0.30 (rounded to 2 significant figures).
Bland–Altman test–retest variability studies have shown that a 0.2 logMAR (2 lines/10 letters) or greater is required to be deemed as clinically significant and reliably distinguished from no clinically meaningful change. 36,37 Furthermore, as a rule of thumb, in patients with severe visual impairment, two to three lines’ change is required to be deemed clinically significant. 38 The FDA stipulates that a gain (or loss) must be at least 3 lines/15 letters to be clinically meaningful since this represents a halving (or doubling) of the letter size visual angle. 39 Any decreases in vision meeting this criterion were reported as SAEs, with the exception of decreases in BCVA caused by cataract.
Statistical methods
Cohort 1 comprised the 30 participants who were enrolled in this study, of whom 28 participants had symmetrical disease and were therefore also assigned to Cohort 2. The statistical analyses were therefore performed on the total cohort comparing visual function and anatomical degeneration in treated versus control eyes.
Summary statistics of each assessed variable was performed for treated eyes versus control eyes. Data involving a comparison of an assessed variable between the treated and untreated eyes (of each participant) were estimated as the difference between the eyes (with a 95% confidence interval), and simple analysis of change from baseline (paired t-test) was performed at 24 months post treatment. As most participants (93%) had symmetric disease, analysis of covariance was deemed unnecessary.
Chapter 3 Results
Recruitment
Recruitment commenced on 16 August 2016 and closed on 23 May 2019. The recruitment target of 30 participants was achieved, of whom 28 participants had symmetrical disease and could therefore be included in Cohort 2 (for whom selection of the treated eye was randomised).
The progress of actual recruitment versus initial target recruitment is outlined in Figure 3. An initial delay in recruitment was caused by a temporary interruption in the supply of the AAV2-REP1 vector. As choroideremia is a rare disease, additional delays in recruitment caused challenges in finding choroideremia patients meeting the inclusion criteria.
FIGURE 3.
Initial target recruitment and actual recruitment of trial participants.

Participant flow and baseline characteristics
A total of 30 patients met the inclusion criteria and were recruited into the trial, of whom 12 were treated at the Oxford Eye Hospital and 18 at the Moorfields Eye Hospital. The Consolidated Standards of Reporting Trials flow diagram (Figure 4) shows the participant flow through the REGENERATE trial. Cohort 1 comprised the 30 participants who were enrolled in this study. Cohort 2 comprised the 28 participants who had symmetrical disease and for whom selection of the treated eye was randomised. Table 2 shows the participants’ subject identifiers and their CHM gene variants. Participant demographics are shown in Table 3. All participants were male, 29 were white and mean age was 32.1 years [standard deviation (SD) 8.79].
Participant | CHM gene variant |
---|---|
201 | c.940 + 3del A |
202 | c.98_96delCCGG |
203 | c.877C > T |
204 | c.877C > T |
205 | c.808C > T |
206 | c.808C > T |
207 | c.49 + 2dupT |
208 | c.808C > T |
209 | c.1770 + 1G > C |
211 | c.1214_121insC |
212 | Exon 4–18 deletion |
213 | c.930_931InsA p. |
601 | c.1584_1587del |
602 | Complete deletion |
603 | Exon 1–15 deletion |
604 | c.1099_1100ins TACC |
605 | c.759delA |
606 | c.315–1536 A > G |
607 | c.1079delA |
608 | c.116 + 1G > A |
609 | c.116 + 1G > A |
610 | c.492_493delGA |
611 | c.492_493delGA |
612 | c.1245_1246del ins 14 |
613 | Exon 1–11 deletion |
614 | c.116 + 1G > A |
615 | c.819 + 1G > A |
616 | Deletion exon 3–intron 4 (c.117–?_314+?del) |
617 | Exon 1–15 deletion |
619 | c.799C > T |
620 | Exons 12 and 13 deletion |
Total participants (n = 30) | |
---|---|
Age, yearsa | |
Mean (SD) | 32.1 (8.79) |
Sex | |
Male, n (%) | 30 (100) |
Race, n (%) | |
Asian | 1 (3.3) |
White | 29 (96.7) |
Cohort, n (%) | |
1, 2 | 28 (93.3) |
1 | 2 (6.7) |
Study eye, n (%) | |
Right | 15 (50.0) |
Left | 15 (50.0) |
Surgical site, n (%) | |
Oxford Eye Hospital, UK | 12 (40.0) |
Moorfields Eye Hospital, London, UK | 18 (60.0) |
FIGURE 4.
Participant flow through the REGENERATE trial.

Clinical outcomes
Primary efficacy measure
Comparative change from baseline in best corrected visual acuity in treated and control eyes at 24 months post treatment
The primary efficacy-related end point (comparative change from baseline in BCVA, measured in ETDRS letters, in treated and control eyes at 24 months post treatment) was not statistically different between treated eyes [−2.63 letters, standard error of the mean (SEM) 2.76] and control eyes (+2.67 letters, SEM 0.768) in all 30 participants, including 4 participants with ocular SAEs (p = 0.08) (Figure 5a). The difference reduced to 0.84 letters (SEM 0.996) in the treated group and remained similar in the control group at + 2.5 letters (SEM 1.85) when BCVA was analysed in 26 participants with no ocular SAEs (p = 0.53) (Figure 5b).
FIGURE 5.
Comparative change from baseline in BCVA in treated and control eyes in all 30 participants, including 4 participants with ocular SAEs (a) and 26 participants with no ocular SAEs (b). The tick marks on the y-axis are standard errors of the mean, and the data are normally distributed. (As defined by GraphPad Prism software: ns = not significant, p > 0.05; *p < 0.05; **p < 0.005; ***p < 0.0005; ****p < 0.0001.)

Analysis excluding patients who suffered an ocular SAE is standard policy in pivotal studies as the SAEs are used separately to assess risks of the potential treatment effect. This is the approach taken by the regulatory authorities for pivotal studies since a complication, such as loss of vision, will most likely be an outlier for statistical analyses. Furthermore, by treating the ocular SAEs separately, there is more transparency on a small number of participants having a complication which might otherwise be lost amongst a large number of participants with favourable outcomes.
Key secondary efficacy measures
Comparative change from baseline in mean microperimetry, total microperimetry and central microperimetry (four central points) in treated and control eyes at 24 months post treatment
Mean total microperimetry declined from baseline in treated eyes (−3.67 dB, SEM 0.585) and control eyes (−1.11, SEM 0.189) with statistically significant difference between the groups at 24 months post treatment (p = 0.0004) when analysing all 30 participants, including 4 participants with ocular SAEs (Figure 6a). A smaller decline in the treated group was observed when four participants with ocular SAEs were excluded (treated = −2.83 dB, SEM 0.359; control = −1.22 dB, SEM 0.192; p < 0.0001) (Figure 6b). Mean central microperimetry (average of four central points tested) declined in treated eyes (−2.93 dB, SEM 1.33) and control eyes (0.05 dB, SEM 0.584) at 24 months (p = 0.04) (Figure 6c). The decline in the treated group was less when four participants with ocular SAEs were excluded from the analysis (treated group mean = −1.08 dB, SEM 0.986; control group = −0.02 dB, SEM 0.671), and the difference between the groups at 24 months was no longer statistically significant (p = 0.3) (Figure 6d).
FIGURE 6.
Comparative change from baseline in mean microperimetry in treated and control eyes: total microperimetry in all 30 subjects, including 4 participants with ocular SAEs (a), total microperimetry in 26 participants with no ocular SAEs (b), central microperimetry in all 30 participants, including 4 participants with ocular SAEs (c) and central microperimetry in 26 participants with no ocular SAEs (d). The tick marks on the y-axis are standard errors of the mean, and the data are normally distributed. (As defined by GraphPad Prism software: ns = not significant, p > 0.05; *p < 0.05; **p < 0.005; ***p < 0.0005; ****p < 0.0001.)

Comparative change from baseline in total area of autofluorescence in treated and control eyes at 24 months post treatment
Total area of autofluorescence declined from baseline in treated eyes (−5.18 mm2, SEM 0.797) and control eyes (−2.47 mm2, SEM 0.374) with statistically significant difference between the groups at 24 months post treatment (p = 0.0004) (Figure 7a). The difference reduced to −4.31 mm2 (SEM 0.578) in the treated group and remained similar in the control group at −2.22 mm2 (SEM 0.295) when the analysis at 24 months was performed excluding four participants with ocular SAEs (p = 0.0003) (Figure 7b).
FIGURE 7.
Comparative change from baseline in total area of autofluorescence in treated and control eyes in all 30 participants, including 4 participants with ocular SAEs (a) and 26 participants with no ocular SAEs (b). The tick marks on the y-axis are standard errors of the mean, and the data are normally distributed. (As defined by GraphPad Prism software: ns = not significant, p > 0.05; *p < 0.05; **p < 0.005; ***p < 0.0005; ****p < 0.0001.)

Safety
Of the 30 participants recruited in the REGENERATE trial, 26 participants (87%) completed the study without any long-standing or unresolved adverse events. No participants discontinued from the study, and no systemic adverse events were reported that were plausibly associated with the study. Most adverse events were treatable, controlled and resolved without sequelae (Figure 8). In total, 128 adverse events (Table 4) were reported during the study:
Number of affected participants (% of affected participants) | Number of adverse events (% of the total) | |
---|---|---|
Ocular adverse events | 30 | 100 |
Inflammatory events | 8 (27%) | 15 (15%) |
Anterior uveitis | 3 | 4 |
Postoperative inflammation | 4 | 4 |
Vitritis | 1 | 2 |
Choroiditis | 1 | 1 |
Panuveitis | 2 | 4 |
Anterior segment events | 22 (73%) | 33 (33%) |
Chalazion | 1 | 1 |
Dry eye | 7 | 7 |
Conjunctival granuloma (cyst) | 2 | 2 |
Eye irritation from suture | 1 | 1 |
Conjunctivitis | 4 | 4 |
Preservative toxicity | 1 | 1 |
Subconjunctival haemorrhage | 8 | 8 |
Punctate epithelial keratopathy | 2 | 2 |
Corneal abrasion | 2 | 2 |
Corneal ulcer | 1 | 1 |
Corneal oedema | 1 | 1 |
Blepharokeratitis | 1 | 1 |
Cataract | 2 | 2 |
Intraocular pressure-related events | 17 (57%) | 21 (21%) |
Hypotony | 11 | 11 |
Ocular hypertension | 7 | 9 |
Hypotonous papillopathy | 1 | 1 |
Retinal events | 11 (37%) | 12 (12%) |
Cystoid macular oedema | 5 | 5 |
Reduced autofluorescence | 5 | 5 |
Choroidal folds | 1 | 1 |
Retinal blot haemorrhage | 1 | 1 |
Vision changes | 11 (37%) | 19 (19%) |
Reduced visual acuity or visual impairment | 4 | 6a |
Reduced low luminance visual acuity | 1 | 1 |
Reduced microperimetry | 1 | 2 |
Visual field defect | 1 | 1 |
Reduced or altered colour perception | 2 | 2 |
Vitreous floaters | 2 | 3 |
Binocular diplopia | 2 | 2 |
Metamorphopsia | 1 | 1 |
Flashing lights | 1 | 1 |
Non-ocular adverse events | 24 | 28 |
Eyelid folliculitis | 1 | 1 |
Blepharitis allergic | 1 | 1 |
Muscle spasms/pain | 2 | 2 |
Skin infection | 3 | 3 |
Herpes zoster | 1 | 1 |
Syncope | 1 | 1 |
Colonoscopy | 1 | 1 |
Cholelithotomy/cholecystitis | 2 | 2a |
Motion sickness | 1 | 1 |
Nasopharyngitis | 2 | 4 |
Dermatitis atopic | 1 | 1 |
Infectious mononucleosis | 1 | 1 |
Seasonal allergy | 1 | 1 |
Inflammatory marker increased | 2 | 2 |
Headache | 1 | 1 |
Gastroenteritis | 1 | 1 |
Acne | 1 | 3 |
Tooth infection | 1 | 1 |
FIGURE 8.
Best corrected visual acuity, central retinal sensitivity (microperimetry) and autofluorescence pattern in a participant (subject 201) with no SAEs over the 24-month assessment period. The patient had no concerns and reported increased colour depth perception at 24 months. (AF = autofluorescence.)
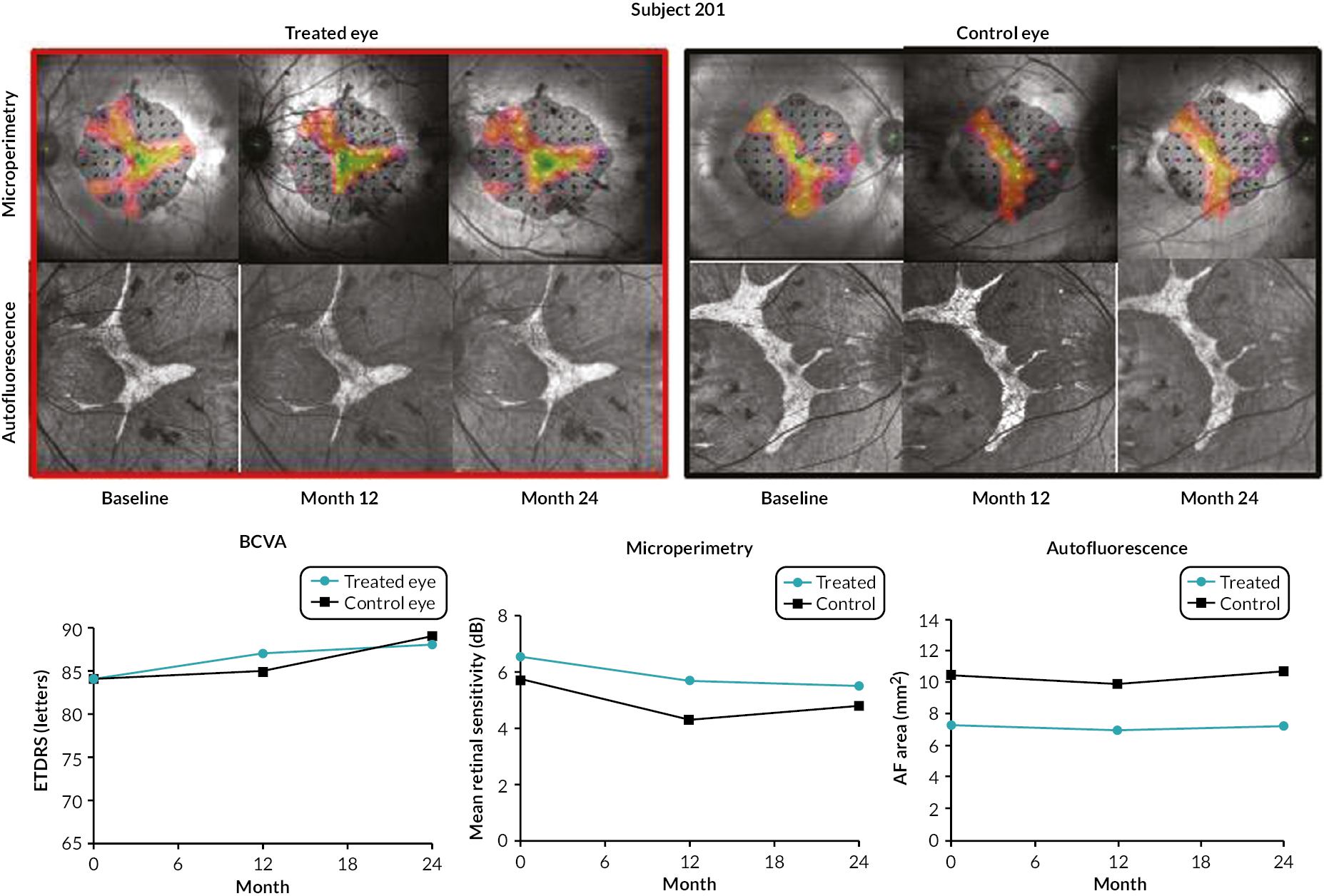
-
Twenty-eight adverse events were classified as non-ocular and identified by the reporting clinician as not associated with the surgical procedure or AAV2-REP1 vector. These included two participants treated at the Oxford Eye Hospital (subjects 203 and 209), who each experienced a non-ocular SAE involving gallstones that required hospitalisation for cholecystitis or cholelithotomy.
-
One hundred adverse events were classified as ocular, of which:
-
thirty-eight adverse events were not plausibly associated with the surgical procedure or AAV2-REP1 vector.
-
sixty-two adverse events, including six SAEs, were associated with the surgical procedure or AAV2-REP1 vector.
-
Six ocular SAEs related to the surgical procedure or AAV2-REP1 vector were reported in four participants treated at Moorfields Eye Hospital:
-
A suspected unexpected serious adverse reaction (SUSAR) was reported for subject 602 (Figure 9a) for a profound loss of BCVA in the treated eye caused by sustained postoperative inflammation related to the AAV2-REP1 vector that was not responsive to immunosuppression.
-
A SUSAR was reported for subject 603 (Figure 9b) for a clinically significant decrease in BCVA in the treated eye caused by recurring postoperative inflammation related to the AAV2-REP1 vector.
-
Three SAEs were reported for subject 617 (Figure 9c) for visual impairment involving loss of autofluorescence and reduced central retinal sensitivity in the treated eye, consequent to choroidal inflammation associated with the AAV2-REP1 vector. BCVA remained stable.
-
An SAE was reported for subject 620 (Figure 9d) for a clinically significant decrease in BCVA in the treated eye following surgery.
FIGURE 9.
Best corrected visual acuity, central retinal sensitivity (microperimetry) and autofluorescence pattern in four participants (subjects 602, 603, 617 and 620) with the six ocular SAEs over the 24-month assessment period. (a) Subject 602 experienced a profound loss of BCVA in the treated eye caused by sustained postoperative inflammation related to the AAV2-REP1 vector that was not responsive to immunosuppression; (b) subject 603 experienced a clinically significant decrease in BCVA in the treated eye caused by recurring postoperative inflammation related to the AAV2-REP1 vector; (c) subject 617 experienced visual impairment involving loss of autofluorescence and reduced central retinal sensitivity in the treated eye, consequent to choroidal inflammation associated with the AAV2-REP1 vector. BCVA remained stable; and (d) subject 620 experienced a clinically significant decrease in BCVA in the treated eye following surgery. (continued)
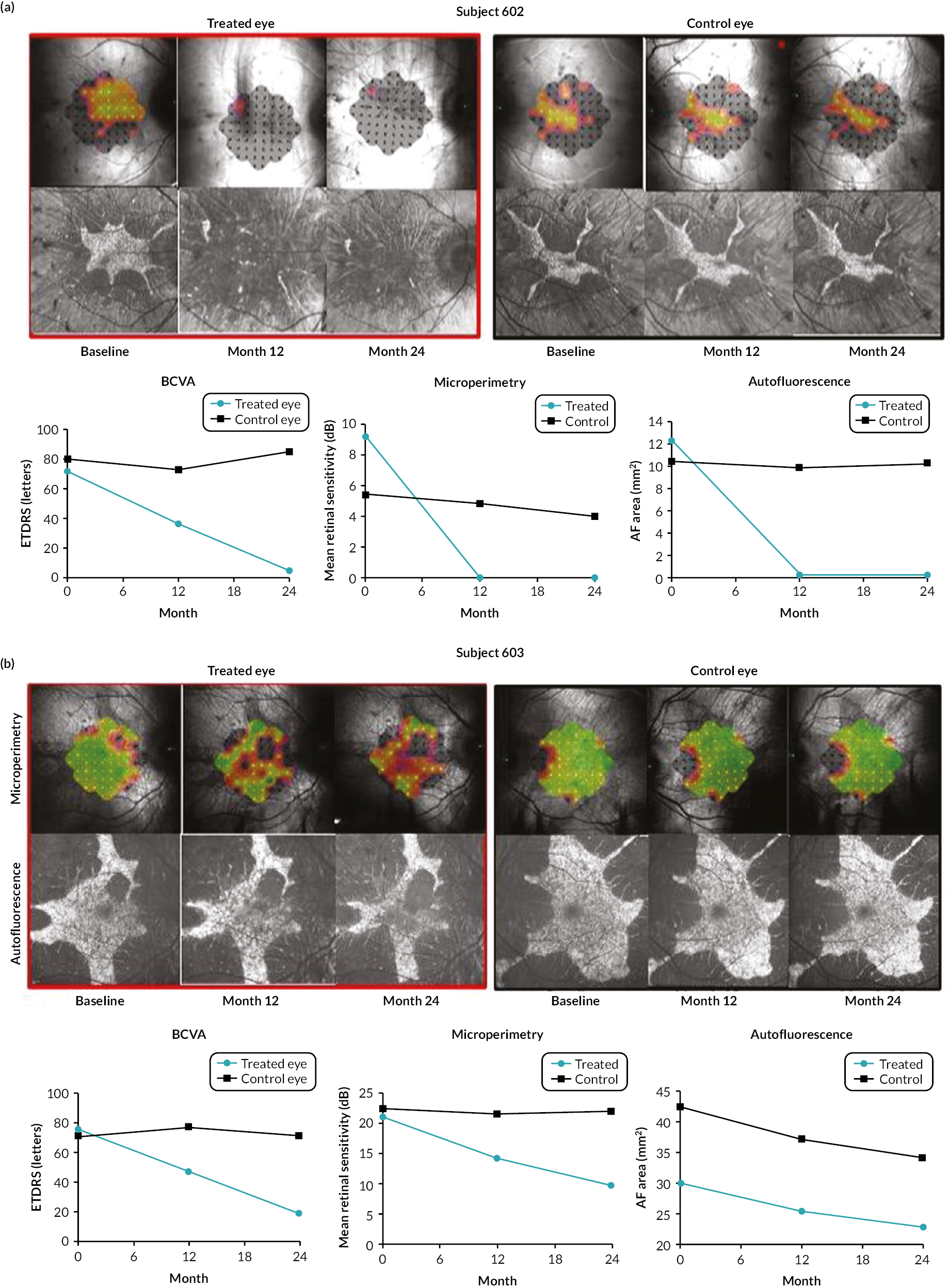

Table 4 details ocular and non-ocular adverse events reported during the study.
Chapter 4 Discussion
Main findings of the study
There has been no signal of possible efficacy of the intervention (in terms of comparative change from baseline in BCVA between treated and control eyes) over the 24-month assessment period.
Overall, BCVA remained relatively stable in treated eyes over the 24-month assessment period. Therefore, in terms of the primary safety-related end point (change from baseline in BCVA in treated eyes at 24 months post treatment), the safety profile of the REGENERATE trial was comparable to other choroideremia gene therapy studies that evaluated the same AAV2-REP1 vector.
Retinal degeneration in choroideremia leads to thin and fragile retinae, making surgical procedures very challenging. Moreover, the RPE at the edges of surviving islands of retinal tissue is very disrupted and may be more susceptible to surgical trauma. A statistically significant deterioration in visual fields was observed in treated eyes compared to control eyes, possibly caused by surgery-induced damage in some treated eyes as manifested by increased loss of autofluorescence and retinal sensitivity at the edges of surviving islands of retinal tissue. The treated eyes underwent retinal detachment prior to injection of the vector suspension into the subretinal space, and this procedure itself can lead to some degree of reduction in central retinal sensitivity and autofluorescence. However, the statistical significance of the comparative reduction in visual fields in treated and control eyes was lost when central retinal sensitivity and area of autofluorescence were analysed in uncomplicated cases. In eyes with no complications, retinal degeneration continued centripetally in both treated and control eyes.
Most adverse events were treatable, controlled and resolved without sequelae. Six SAEs were reported in the treated eyes of four participants: one surgery-related and two inflammation-related SAEs involving clinically significant decreases in BCVA and three SAEs in one participant involving reduction in central retinal sensitivity but with BCVA remaining stable.
Limitations of the study
No evidence of possible efficacy of the intervention was observed, as a meaningful difference in comparative change from baseline in BCVA in treated and control eyes was not discernible over the 24-month assessment period. As choroideremia is a very slow degeneration, BCVA in control eyes did not decline significantly during the assessment period.
Future investigations
Future investigations will include a long-term assessment of BCVA, central retinal sensitivity and area of autofluorescence in REGENERATE trial participants in an observational study (SOLSTICE: NCT03584165). Possible approaches to further the investigation of gene therapy for choroideremia include the optimisation of inclusion criteria for subjects still retaining a healthy central retina which may be more amenable to rescue by gene therapy.
Chapter 5 Conclusion
There has been no signal of possible efficacy of the intervention (in terms of comparative change from baseline in BCVA between treated and control eyes) over the 24-month assessment period.
Overall, BCVA remained relatively stable in treated eyes over the 24-month assessment period. Therefore, in terms of the primary safety-related end point (change from baseline in BCVA in treated eyes at 24 months post treatment), the safety profile of the REGENERATE trial was comparable to other choroideremia gene therapy studies that evaluated the same AAV2-REP1 vector. Of the 30 participants recruited in the REGENERATE trial, 26 participants (87%) completed the study without any long-standing or unresolved adverse events. No participants discontinued from the study, and no systemic adverse events were reported that were plausibly associated with the study. Most adverse events were treatable, controlled and resolved without sequelae. However, ocular SAEs were reported for the following four participants treated at the Moorfields Eye Hospital:
-
Subject 602 experienced a profound loss of BCVA in the treated eye caused by sustained postoperative inflammation related to the AAV2-REP1 vector that was not responsive to immunosuppression.
-
Subject 603 experienced a clinically significant decrease in BCVA in the treated eye caused by recurring postoperative inflammation related to the AAV2-REP1 vector.
-
Subject 617 experienced visual impairment involving loss of autofluorescence and reduced central retinal sensitivity in the treated eye, consequent to choroidal inflammation associated with the AAV2-REP1 vector. BCVA remained stable.
-
Subject 620 experienced a clinically significant decrease in BCVA in the treated eye following surgery.
Retinal degeneration in choroideremia leads to thin and fragile retinae, making surgical procedures very challenging. Moreover, the RPE at the edges of surviving islands of retinal tissue is very disrupted and may be more susceptible to surgical trauma. A statistically significant deterioration in visual fields was observed in treated eyes compared to control eyes, possibly caused by surgery-induced damage in some treated eyes as manifested by increased loss of autofluorescence and retinal sensitivity at the edges of surviving islands of retinal tissue. The treated eyes underwent retinal detachment prior to injection of the vector suspension into the subretinal space, and this procedure itself can lead to some degree of reduction in central retinal sensitivity and area of autofluorescence. However, the statistical significance of the comparative reduction in visual fields in treated and control eyes was lost when central retinal sensitivity and area of autofluorescence were analysed in uncomplicated cases. In eyes with no complications, retinal degeneration continued centripetally in both treated and control eyes.
Due to the centripetal nature of retinal degeneration in choroideremia, RPE in the peripheral ‘mottled zone’ of fundus autofluorescence may be at a more advanced level of degeneration and therefore more difficult to rescue. 40 Possible approaches to further the investigation of gene therapy for choroideremia include the optimisation of inclusion criteria for subjects still retaining a healthy central retina, characterised by the presence of a ‘smooth zone’ of fundus autofluorescence that represents an area of healthy RPE tissue which may be more amenable to rescue by gene therapy. 41
Although this study has not presented evidence that reduction in visual fields caused by the intervention would be justified by the possible rescue of BCVA, a more definitive assessment may be provided by long-term monitoring of REGENERATE trial participants in an observational study allowing for up to 60 months of follow-up of treated subjects (SOLSTICE: NCT03584165). This study is sponsored by Nightstar Therapeutics (London, UK), a biotechnology company founded by the University of Oxford to progress clinical evaluation of gene therapies for choroideremia and other inherited retinal diseases.
Nightstar Therapeutics, now part of Biogen Inc. (Cambridge, MA, USA), has also sponsored a natural history study (NIGHT: NCT03359551) investigating the progression of choroideremia, a Phase II clinical trial (GEMINI: NCT03507686) investigating the safety of bilateral administration of AAV2-REP1 in choroideremia patients and a Phase III clinical trial (STAR: NCT03496012) investigating the safety and efficacy of unilateral administration of AAV2-REP1 in choroideremia patients randomised to receive a high dose (1 × 1011 vector genomes; n = 69) or low dose (1 × 1010 vector genomes; n = 34) of the gene therapy versus a non-treated control group (n = 66).
The results of the STAR Phase III clinical trial have recently been reported. 42 In the primary end-point analysis, 3 of 65 participants (5%) in the high-dose group, 1 of 34 (3%) participants in the low-dose group and 0 of 62 (0%) participants in the control group had ≥ 15-letter ETDRS improvement from baseline BCVA at 12 months (high dose, p = 0.245 vs. control; low dose, p = 0.354 vs. control). As the primary end point was not met, key secondary end points were not tested for significance. In a key secondary end point, 9 of 65 (14%), 6 of 35 (18%) and 1 of 62 (2%) participants in the high-dose, low-dose and control groups, respectively, experienced ≥ 10-letter ETDRS improvement from baseline BCVA at 12 months. A ≥ 10-letter ETDRS improvement from baseline in BCVA has been considered clinically relevant from the patient’s perspective according to scientific advice given at a workshop by the EMA. 43
Note that the choroideremia patients selected for the STAR trial had far more advanced disease than those in the REGENERATE trial due to the low BCVA entry requirement. While gain in BCVA may be a useful end point in patients who have started to lose it, BCVA cannot improve from baseline levels when patients (such as those recruited in the REGENERATE trial) are still able to read the maximum number of letters on an eye chart. Optimisation of clinical end points to evaluate potential efficacy of gene therapy in early-stage choroideremia patients is therefore imperative.
Potential opportunities to enhance future gene therapy studies for choroideremia include optimisation of surgical techniques and selection of participants still retaining a healthy central retina which may be more amenable to rescue by gene therapy.
Additional information
CRediT contribution statement
Jasmina Cehajic-Kapetanovic (https://orcid.org/0000-0002-9956-6412): Data curation (lead), Formal analysis (lead), Investigation (lead), Visualisation (lead), Writing – reviewing and editing (supporting). Marco P Bellini (https://orcid.org/0000-0002-1104-3968): Project administration (lead), Writing – original draft (lead), Writing – reviewing and editing (lead). Laura J Taylor (https://orcid.org/0000-0001-7072-0853): Data curation (supporting), Investigation (supporting), visualisation (supporting), Writing – original draft (supporting). Imran H Yusuf (https://orcid.org/0000-0002-0424-5852): Data curation (supporting), Formal analysis (supporting), Investigation (supporting). Taha Soomro (https://orcid.org/0000-0003-2756-3674): Data curation (supporting), Formal analysis (supporting), Investigation (supporting). Lyndon da Cruz (https://orcid.org/0000-0002-7695-6354): Supervision (equal), Writing – reviewing and editing (supporting). Robert E MacLaren (https://orcid.org/0000-0002-3096-4682): Conceptualisation (lead), Funding acquisition (lead), supervision (equal), Writing – reviewing and editing (supporting). Andrew Stockman: Data curation (supporting), Investigation (supporting). Kanmin Xue: Data curation (supporting), Investigation (supporting). Odysseas Georgiadis: Data curation (supporting), Investigation (supporting). Edward Bloch: Data curation (supporting), Investigation (supporting). Blanca C Flores-Sánchez: Data curation (supporting), Investigation (supporting). Adam M Dubis: Data curation (supporting), Investigation (supporting). Anthony G Robson: Data curation (supporting), Investigation (supporting). Alun R Barnard: Data curation (supporting), Investigation (supporting). Jasleen K Jolly: Data curation (supporting), Investigation (supporting). Andrew R Webster: Funding acquisition (supporting).
Acknowledgements
The authors would like to thank Sr Anna Rudenko and the other members of the Eye Research Group Oxford clinical trials unit at the Oxford Eye Hospital and Mr Panayiotis Panayiotou and the other members of the clinical team at the Moorfields Eye Hospital for their invaluable assistance in co-ordinating the REGENERATE trial at the Oxford Eye Hospital and Moorfields Eye Hospital, respectively. The authors would also like to thank the members of the REGENERATE Study Group (see Appendix 1), Trial Steering Committee (see Appendix 2) and Data and Safety Monitoring Committee (see Appendix 2) for their important contributions.
The study was sponsored by the University of Oxford and supported by the UK Clinical Research Network, NIHR Oxford Biomedical Research Centre (BRC) and NIHR Moorfields BRC.
Patient data statement
This work uses data provided by patients and collected by the NHS as part of their care and support. Using patient data is vital to improve health and care for everyone. There is huge potential to make better use of information from people’s patient records, to understand more about disease, develop new treatments, monitor safety and plan NHS services. Patient data should be kept safe and secure to protect everyone’s privacy, and it is important that there are safeguards to make sure that they are stored and used responsibly. Everyone should be able to find out about how patient data are used. #datasaveslives You can find out more about the background to this citation here: https://understandingpatientdata.org.uk/data-citation.
Data-sharing statement
All data requests should be submitted to the corresponding author for consideration. Access to anonymised data may be granted following review.
Ethics statement
An application for ethical approval was submitted to the Gene Therapy Advisory Committee (GTAC), the United Kingdom’s national Research Ethics Committee (REC) overseeing clinical trials of gene therapies. Ethics approval was granted by the London – West London and GTAC REC on 16 October 2015: reference 15/LO/1379.
Information governance statement
The University of Oxford is committed to handling all personal information in accordance with the Data Protection Act 2018 and the General Data Protection Regulation (GDPR). This is specified in the University’s data protection policy (https://compliance.admin.ox.ac.uk/data-protection-policy) and in the REGENERATE study protocol. The University of Oxford, as sponsor of the REGENERATE study, is the data controller. If you agreed to participate in the REGENERATE study, information regarding the use of your personal data for research purposes by the University of Oxford is available at https://compliance.web.ox.ac.uk/research-data. Information about your individual rights under the GDPR and the contact details of the University’s Information Compliance Team is available at https://compliance.admin.ox.ac.uk/individual-rights.
Disclosure of interests
Full disclosure of interests: Completed ICMJE forms for all authors, including all related interests, are available in the toolkit on the NIHR Journals Library report publication page at https://doi.org/10.3310/WARA5730.
Primary conflicts of interest: Robert E MacLaren reports financial support from the NIHR via Senior Investigator Award NIHR201338 during the conduct of the study; grants, personal fees and non-financial support from Nightstar Therapeutics (London, UK), now part of Biogen Inc. (Cambridge, MA, USA), during the conduct of the study; grants, personal fees and advisory board honoraria from Gyroscope Therapeutics (London, UK), now part of Novartis International AG (Basel, Switzerland), outside the submitted work; and is a named inventor on international patent WO2012114090 (gene therapy for choroideremia), US patent US20160206704 (subretinal administration of retinal gene therapy) and international patent WO2017153774 (assay for Rab escort protein 1).
Disclaimers
This article presents independent research. The views and opinions expressed by authors in this publication are those of the authors and do not necessarily reflect those of the NHS, the NIHR, the MRC, the EME programme or the Department of Health and Social Care. If there are verbatim quotations included in this publication the views and opinions expressed by the interviewees are those of the interviewees and do not necessarily reflect those of the authors, those of the NHS, the NIHR, the EME programme or the Department of Health and Social Care.
References
- Ortiz-Ramirez GY, Villanueva-Mendoza C, Zenteno Ruiz JC, Reyes M, Cortés-González V. Autofluorescence in female carriers with choroideremia: a familial case with a novel mutation in the CHM gene. Ophthalmic Genet 2020;41:625-8. https://doi.org/10.1080/13816810.2020.1810283.
- Bonilha VL, Trzupek KM, Li Y, Francis PJ, Hollyfield JG, Rayborn ME, et al. Choroideremia: analysis of the retina from a female symptomatic carrier. Ophthalmic Genet 2008;29:99-110. https://doi.org/10.1080/13816810802206499.
- Fahim AT, Daiger SP. The role of X-chromosome inactivation in retinal development and disease. Adv Exp Med Biol 2016;854:325-31. https://doi.org/10.1007/978-3-319-17121-0_43.
- Jolly JK, Xue K, Edwards TL, Groppe M, MacLaren RE. Characterizing the natural history of visual function in choroideremia using microperimetry and multimodal retinal imaging. Invest Ophthalmol Vis Sci 2017;58:5575-83. https://doi.org/10.1167/iovs.17-22486.
- Shen LL, Ahluwalia A, Sun M, Young BK, Grossetta Nardini HK, Del Priore LV. Long-term natural history of visual acuity in eyes with choroideremia: a systematic review and meta-analysis of data from 1004 individual eyes. Br J Ophthalmol 2021;105:271-8. https://doi.org/10.1136/bjophthalmol-2020-316028.
- Hariri AH, Velaga SB, Girach A, Ip MS, Le PV, Lam BL, et al. Natural History of the Progression of Choroideremia (NIGHT) Study Group . Measurement and reproducibility of preserved ellipsoid zone area and preserved retinal pigment epithelium area in eyes with choroideremia. Am J Ophthalmol 2017;179:110-7. https://doi.org/10.1016/j.ajo.2017.05.002.
- Hariri AH, Ip MS, Girach A, Lam BL, Fischer MD, Sankila EM, et al. for Natural History of the Progression of Choroideremia (NIGHT) Study Group . Macular spatial distribution of preserved autofluorescence in patients with choroideremia. Br J Ophthalmol 2019;103:933-7. https://doi.org/10.1136/bjophthalmol-2018-312620.
- Cremers FP, van de Pol DJ, van Kerkhoff LP, Wieringa B, Ropers HH. Cloning of a gene that is rearranged in patients with choroideraemia. Nature 1990;347:674-7. https://doi.org/10.1038/347674a0.
- Seabra MC, Brown MS, Goldstein JL. Retinal degeneration in choroideremia: deficiency of rab geranylgeranyl transferase. Science 1993;259:377-81. https://doi.org/10.1126/science.8380507.
- Zhang FL, Casey PJ. Protein prenylation: molecular mechanisms and functional consequences. Annu Rev Biochem 1996;65:241-69. https://doi.org/10.1146/annurev.bi.65.070196.001325.
- Patrício MI, Barnard AR, Xue K, MacLaren RE. Choroideremia: molecular mechanisms and development of AAV gene therapy. Expert Opin Biol Ther 2018;18:807-20. https://doi.org/10.1080/14712598.2018.1484448.
- Seabra MC, Ho YK, Anant JS. Deficient geranylgeranylation of Ram/Rab27 in choroideremia. J Biol Chem 1995;270:24420-7. https://doi.org/10.1074/jbc.270.41.24420.
- Cehajic Kapetanovic J, Barnard AR, MacLaren RE. Molecular therapies for choroideremia. Genes (Basel) 2019;10. https://doi.org/10.3390/genes10100738.
- Sun LW, Johnson RD, Williams V, Summerfelt P, Dubra A, Weinberg DV, et al. Multimodal imaging of photoreceptor structure in choroideremia. PLOS ONE 2016;11. https://doi.org/10.1371/journal.pone.0167526.
- Tolmachova T, Anders R, Abrink M, Bugeon L, Dallman MJ, Futter CE, et al. Independent degeneration of photoreceptors and retinal pigment epithelium in conditional knockout mouse models of choroideremia. J Clin Invest 2006;116:386-94. https://doi.org/10.1172/JCI26617.
- van den Hurk JA, Schwartz M, van Bokhoven H, van de Pol TJ, Bogerd L, Pinckers AJ, et al. Molecular basis of choroideremia (CHM): mutations involving the Rab escort protein-1 (REP-1) gene. Hum Mutat 1997;9:110-7. https://doi.org/10.1002/(SICI)1098-1004(1997)9:2<110::AID-HUMU2>3.0.CO;2-D.
- MacLaren RE, Groppe M, Barnard AR, Cottriall CL, Tolmachova T, Seymour L, et al. Retinal gene therapy in patients with choroideremia: initial findings from a phase 1/2 clinical trial. Lancet 2014;383:1129-37. https://doi.org/10.1016/S0140-6736(13)62117-0.
- Bennett J, Maguire AM, Cideciyan AV, Schnell M, Glover E, Anand V, et al. Stable transgene expression in rod photoreceptors after recombinant adeno-associated virus-mediated gene transfer to monkey retina. Proc Natl Acad Sci U S A 1999;96:9920-5. https://doi.org/10.1073/pnas.96.17.9920.
- Vandenberghe LH, Auricchio A. Novel adeno-associated viral vectors for retinal gene therapy. Gene Ther 2012;19:162-8. https://doi.org/10.1038/gt.2011.151.
- U.S. Food and Drug Administration . Luxturna n.d. www.fda.gov/vaccines-blood-biologics/cellular-gene-therapy-products/luxturna (accessed November 2023).
- European Medicines Agency . Luxturna n.d. www.ema.europa.eu/en/medicines/human/EPAR/luxturna (accessed November 2023).
- National Institute for Health and Care Excellence . Voretigene Neparvovec for Treating Inherited Retinal Dystrophies Caused by RPE65 Gene Mutations n.d. www.nice.org.uk/guidance/hst11 (accessed October 2023).
- Xue K, Jolly JK, Barnard AR, Rudenko A, Salvetti AP, Patrício MI, et al. Beneficial effects on vision in patients undergoing retinal gene therapy for choroideremia. Nat Med 2018;24:1507-12. https://doi.org/10.1038/s41591-018-0185-5.
- Dimopoulos IS, Hoang SC, Radziwon A, Binczyk NM, Seabra MC, MacLaren RE, et al. Two-year results after AAV2-mediated gene therapy for choroideremia: the Alberta experience. Am J Ophthalmol 2018;193:130-42. https://doi.org/10.1016/j.ajo.2018.06.011.
- Fischer MD, Ochakovski GA, Beier B, Seitz IP, Vaheb Y, Kortuem C, et al. Efficacy and safety of retinal gene therapy using adeno-associated virus vector for patients with choroideremia: a randomized clinical trial. JAMA Ophthalmol 2019;137:1247-54. https://doi.org/10.1001/jamaophthalmol.2019.3278.
- Lam BL, Davis JL, Gregori NZ, MacLaren RE, Girach A, Verriotto JD, et al. Choroideremia gene therapy phase 2 clinical trial: 24-month results. Am J Ophthalmol 2019;197:65-73. https://doi.org/10.1016/j.ajo.2018.09.012.
- Xue K, MacLaren RE. Ocular gene therapy for choroideremia: clinical trials and future perspectives. Expert Rev Ophthalmol 2018;13:129-38. https://doi.org/10.1080/17469899.2018.1475232.
- Aleman TS, Huckfeldt RM, Serrano LW, Pearson DJ, Vergilio GK, McCague S, et al. Adeno-associated virus serotype 2–hCHM subretinal delivery to the macula in choroideremia: two-year interim results of an ongoing phase I/II gene therapy trial. Ophthalmology 2022;129:1177-91. https://doi.org/10.1016/j.ophtha.2022.06.006.
- Spitznas M. A binocular indirect ophthalmomicroscope (BIOM) for non-contact wide-angle vitreous surgery. Graefes Arch Clin Exp Ophthalmol 1987;225:13-5. https://doi.org/10.1007/BF02155797.
- Ferris FL, Kassoff A, Bresnick GH, Bailey I. New visual acuity charts for clinical research. Am J Ophthalmol 1982;94:91-6. https://doi.org/10.1016/0002-9394(82)90197-0.
- Dimopoulos TS, Tseng C, MacDonald IM. Microperimetry as an outcome measure in choroideremia trials: reproducibility and beyond. Invest Ophthalmol Vis Sci 2016;57:4151-61. https://doi.org/10.1167/iovs.16-19338.
- Josan AS, Buckley TMW, Wood LJ, Jolly JK, Cehajic-Kapetanovic J, MacLaren RE. Microperimetry hill of vision and volumetric measures of retinal sensitivity. Transl Vis Sci Technol 2021;10. https://doi.org/10.1167/tvst.10.7.12.
- Bach M, Brigell MG, Hawlina M, Holder GE, Johnson MA, McCulloch DL, et al. ISCEV standard for clinical pattern electroretinography (PERG): 2012 update. Doc Ophthalmol 2013;126:1-7. https://doi.org/10.1007/s10633-012-9353-y.
- Jolly JK, Edwards TL, Moules J, Groppe M, Downes SM, MacLaren RE. A qualitative and quantitative assessment of fundus autofluorescence patterns in patients with choroideremia. Invest Ophthalmol Vis Sci 2016;57:4498-503. https://doi.org/10.1167/iovs.15-18362.
- Akyol E, Hagag AM, Sivaprasad S, Lotery AJ. Adaptive optics: principles and applications in ophthalmology. Eye (Lond) 2021;35:244-64. https://doi.org/10.1038/s41433-020-01286-z.
- Rosser DA, Cousens SN, Murdoch IE, Fitzke FW, Laidlaw DA. How sensitive to clinical change are ETDRS logMAR visual acuity measurements?. Invest Ophthalmol Vis Sci 2003;44:3278-81. https://doi.org/10.1167/iovs.02-1100.
- Bland JM, Altman DG. Statistical methods for assessing agreement between two methods of clinical measurement. Lancet 1986;327:307-10. https://doi.org/10.1016/S0140-6736(86)90837-8.
- Kiser AK, Mladenovich D, Eshraghi F, Bourdeau D, Dagnelie G. Reliability and consistency of visual acuity and contrast sensitivity measures in advanced eye disease. Optom Vis Sci 2005;82:946-54. https://doi.org/10.1097/01.opx.0000187863.12609.7b.
- U.S. Food and Drug Administration . Human Gene Therapy for Retinal Disorders: Guidance for Industry n.d. www.fda.gov/media/124641/download (accessed November 2023).
- Stevanovic M, Cehajic Kapetanovic J, Jolly JK, MacLaren RE. A distinct retinal pigment epithelial cell autofluorescence pattern in choroideremia predicts early involvement of overlying photoreceptors. Acta Ophthalmol 2020;98:e322-7. https://doi.org/10.1111/aos.14281.
- Poli FE, Yusuf IH, Jolly JK, Taylor LJ, Adeyoju D, Josan AS, et al. Correlation between fundus autofluorescence pattern and retinal function on microperimetry in choroideremia. Transl Vis Sci Technol 2023;12. https://doi.org/10.1167/tvst.12.9.24.
- MacLaren RE, Fischer MD, Gow JA, Lam BL, Sankila EK, Girach A, et al. Subretinal timrepigene emparvovec in adult men with choroideremia: a randomized phase 3 trial. Nat Med 2023;29:2464-72. https://doi.org/10.1038/s41591-023-02520-3.
- European Medicines Agency . EU Regulatory Workshop – Ophthalmology – Summary and Report n.d. www.ema.europa.eu/en/documents/report/european-union-regulatory-workshop-ophthalmology-summary-report_en.pdf (accessed November 2023).
Appendix 1 Members of the REGENERATE Study Group
-
Alun R Barnard, Nuffield Department of Clinical Neurosciences, University of Oxford, Oxford, UK. https://orcid.org/0000-0002-0662-1073
-
Edward Bloch, Moorfields Eye Hospital, London, UK. https://orcid.org/0000-0002-0799-3258
-
Adam M Dubis, University College London (UCL) Institute of Ophthalmology, London, UK. https://orcid.org/0000-0002-5108-2179
-
Blanca C Flores-Sánchez, Moorfields Eye Hospital, London, UK. https://orcid.org/0000-0001-6730-5413
-
Odysseas Georgiadis, Moorfields Eye Hospital, London, UK. https://orcid.org/0000-0002-6764-0163
-
Jasleen K Jolly, Nuffield Department of Clinical Neurosciences, University of Oxford, Oxford, UK. https://orcid.org/0000-0001-9878-4621
-
Anthony G Robson, Moorfields Eye Hospital, London, UK. https://orcid.org/0000-0002-8391-6123
-
Andrew Stockman, University College London (UCL) Institute of Ophthalmology, London, UK. https://orcid.org/0000-0001-9807-6289
-
Andrew R Webster, Moorfields Eye Hospital, London, UK. http://orcid.org/0000-0001-6915-9560
-
Kanmin Xue, Nuffield Department of Clinical Neurosciences, University of Oxford, Oxford, UK. https://orcid.org/0000-0003-0065-9131
Appendix 2 Members of the trial oversight committees
Trial Steering Committee
-
Chairperson: Robert Henderson, Consultant Ophthalmic Surgeon, Great Ormond St Children’s Hospital, London, UK.
-
Independent member: Tim Jackson, Consultant Ophthalmic Surgeon, King’s College Hospital, London, UK.
-
Independent member: Edward Lee, Consultant Ophthalmic Surgeon, Epsom and St Helier Hospital, Carshalton, UK.
-
Non-independent member: Adnan Tufail, Consultant Ophthalmic Surgeon, Moorfields Eye Hospital, London, UK.
Data and Safety Monitoring Committee
-
Chairperson: Tony Moore, Professor of Ophthalmology, University of California San Francisco, San Francisco, USA.
-
Member: David Wong, Professor of Ophthalmology, University of Liverpool, Liverpool, UK.
-
Member: Miles Stanford, Professor of Clinical Ophthalmology, King’s College London, London, UK.
-
Member and statistician: Kashfia Chowdhury, Senior Research Associate, University College London, London, UK.
List of abbreviations
- AAV
- adeno-associated virus
- AAV2
- adeno-associated virus serotype 2
- ART
- automatic real time
- BCVA
- best corrected visual acuity
- BRC
- Biomedical Research Centre
- CIE
- International Commission on Illumination (Commission Internationale de l’Eclairage)
- EMA
- European Medicines Agency
- ERG
- electroretinography
- ETDRS
- Early Treatment Diabetic Retinopathy Study
- FDA
- US Food and Drug Administration
- FST
- full-field stimulus threshold
- GDPR
- General Data Protection Regulation
- HEYEX
- Heidelberg Eye Explorer
- ISCEV
- International Society for Clinical Electrophysiology of Vision
- ISRCTN
- International Standard Randomised Controlled Trial Number
- logMAR
- logarithm of the minimum angle of resolution
- NIHR
- National Institute for Health and Care Research
- OCT
- optical coherence tomography
- REC
- Research Ethics Committee
- REP1
- Rab escort protein 1
- RPE
- retinal pigment epithelium
- SAE
- serious adverse event
- SEM
- standard error of the mean
- SUSAR
- suspected unexpected serious adverse reaction
- WPRE
- woodchuck hepatitis virus post-transcriptional regulatory element