Notes
Article history
The research reported in this issue of the journal was commissioned by the HTA programme as project number 09/106/01. The contractual start date was in June 2010. The draft report began editorial review in July 2011 and was accepted for publication in January 2012. As the funder, by devising a commissioning brief, the HTA programme specified the research question and study design.The authors have been wholly responsible for all data collection, analysis and interpretation, and for writing up their work. The HTA editors and publisher have tried to ensure the accuracy of the authors’ report and would like to thank the referees for their constructive comments on the draft document. However, they do not accept liability for damages or losses arising from material published in this report.
Declared competing interests of authors
none
Permissions
Copyright statement
© Queen’s Printer and Controller of HMSO 2012. This work was produced by Burch et al. under the terms of a commissioning contract issued by the Secretary of State for Health. This issue may be freely reproduced for the purposes of private research and study and extracts (or indeed, the full report) may be included in professional journals provided that suitable acknowledgement is made and the reproduction is not associated with any form of advertising. Applications for commercial reproduction should be addressed to NETSCC. This journal is a member of and subscribes to the principles of the Committee on Publication Ethics (COPE) (http://www.publicationethics.org/). This journal may be freely reproduced for the purposes of private research and study and may be included in professional journals provided that suitable acknowledgement is made and the reproduction is not associated with any form of advertising. Applications for commercial reproduction should be addressed to: NETSCC, Health Technology Assessment, Alpha House, University of Southampton Science Park, Southampton SO16 7NS, UK.
2012 Queen’s Printer and Controller of HMSO
Chapter 1 Background
Description of health problem
Epilepsy
Epilepsy is the most common serious neurological condition,1 with a prevalence of between 0.5% and 1% in developed countries, and a cumulative incidence of up to 3% by the age of 75 years. 2,3 A review of epidemiological data from European countries reported prevalence as ranging from 0.32% to 0.78%, depending on the country and population studied. 4 The only UK study included in that review4 reported a prevalence of 0.43% in children aged 6–14 years in England. 5 With approximately 456,000 people in the UK having a diagnosis of epilepsy,6–8 overall prevalence can be estimated at around 0.8%. Most patients achieve good seizure control, but up to 30% continue to have seizures despite ongoing treatment with one or more antiepileptic drugs (AEDs). 9
Classification of epilepsies
Epilepsies are a large heterogeneous group of disorders, including those that are genetically determined (idiopathic, tending to present in childhood and adolescence) as well as those that are symptomatic of a brain injury (e.g. stroke or head injury). The epilepsies are classified into syndromes according to seizure types, aetiology, age at onset, electroencephalographic changes and magnetic resonance imaging (MRI) findings. One of the major classifications is between localisation-related epilepsies (affecting a limited area of the brain, i.e. focal or partial), which result in focal seizures and can be simple (no associated impairment of consciousness) or complex (impairment of consciousness), and secondary generalised seizures (resulting in distortion of the electrical activity of the whole, or a large part of, the brain). These epilepsies are subclassified according to the site of the focus (frontal, temporal, parietal, occipital lobes) and then further divided according to aetiology:
-
Symptomatic epilepsy Where there is evidence of a lesion or damage to the brain (e.g. mesial temporal lobe sclerosis, cortical dysplasia, tumour, vascular malformation, haemorrhage, infarct, infection and trauma).
-
Cryptogenic epilepsy Where there are symptoms to suggest brain damage, but there is no evidence of a lesion or damage to the brain.
-
Idiopathic epilepsy Assumed genetic aetiology.
Psychogenic seizures are non-epileptic seizures, although patients can be misdiagnosed initially as having epilepsy. Patients with such seizures can generally be identified using video-EEG monitoring, as they do not have the electrical discharges on EEG that are characteristic of epilepsy.
Epilepsy pathophysiology
An epileptic focus may be located anywhere in the cerebral cortex, and there are a number of potential aetiologies. Approximately 20% of patients who are evaluated for intractable epilepsy are thought to have non-epileptic psychogenic seizures. 10 From an epilepsy surgery perspective, epilepsy tends to be grouped into two categories: temporal and extratemporal. Temporal lobe epilepsy (TLE) is the most prevalent focal (partial) epilepsy, and is the epilepsy that is most frequently treated surgically. 1 The focus is most often located in the amygdalohippocampal region of the mesial (medial) temporal lobe,1 with mesial temporal lobe sclerosis (MTS) being the most common form of TLE for which surgery is undertaken, and is thought to be associated with hippocampal neuronal loss [hippocampal sclerosis (HS)] and gliosis,1 although the cause is still uncertain. Lateral temporal onset can occur, but this is less common. 1 The most common type of extra-TLE is frontal lobe epilepsy. Causes of epilepsy other than MTS include focal cortical dysplasia (congenital abnormality where neurons fail to develop normally), tuberous sclerosis (non-malignant growths caused by an inherited genetic mutation), and brain injury (caused by illness, for example cerebral infarction, alcohol or drug misuse, and trauma), while a large proportion remains cryptogenic.
Surgery for epilepsy
Of the 20–30% of people who continue to have seizures despite drug treatment, the majority have symptomatic or cryptogenic localisation-related epilepsy. A review conducted in 2001 reported response rates in patients with refractory localisation related epilepsy to adjunctive therapies (adjusted for placebo response rates) of between 12% and 29% for antiepileptic drugs (drugs evaluated were gabapentin, lamotrigine, levetiracetam, oxcarbazepine, tiagabine, topiramate, zonisamide) and 12% for adjunctive vagal nerve stimulation. 11 For patients who do not respond to adjunctive therapy, surgical resection of the epileptic focus may be considered, and can result in the patient becoming seizure free (SF). The main aim of surgery is to remove the seizure focus, leaving the patient free from seizures without causing other disability. Most epilepsy surgery programmes focus on temporal lobe surgery (temporal lobectomy); excision of the temporal lobe has a higher rate of success and a lower chance of causing harm than resection of extratemporal lobe foci. Temporal lobectomy has been estimated to result in the long-term cure of TLE (free from seizures and the discontinuation of AEDs) in 25–30% of patients undergoing the procedure, with a further 25–30% becoming SF with continued AED treatment. 1 Surgical procedures other than lobectomy are sometimes performed, for example hemispherectomy, corpus callosotomy, multiple subpial transaction and vagal nerve stimulation. The first randomised controlled trial (RCT) evaluating epilepsy surgery randomised 80 patients with drug-refractory TLE to either surgery or continued AED treatment. At 12 months, 58% of the surgical group and 8% of the AED group were free of complex focal seizures, giving a number needed to treat of 2 [95% confidence interval (CI) 1.3 to 3]. 12
Burden of the disease
According to Hospital Episode Statistics (HES), in 2009–10 epilepsy was responsible for 54,428 consultant episodes and 42,385 admissions (35,515 emergency, 4192 waiting list and 2678 day cases), which accounted for 153,035 bed-days. 7 In 2009–10, 344 operations were conducted where major excision of brain tissue was the main operation, and 3890 where excision of a lesion of brain tissue was the main operation; it is unclear how many of these procedures were conducted on patients with epilepsy. 7 The 1999 Department of Health-commissioned report from the Clinical Standards Advisory Group (CSAG) on services for patients with epilepsy estimated that between 5% and 10% of patients with refractory focal epilepsy might benefit from epilepsy surgery. 3 Using this estimate, the CSAG calculated that between 5000 and 10,000 UK patients might benefit from epilepsy surgery, with between 750 and 1500 cases being added to this cohort each year. A study using the UK National General Practice Study of Epilepsy estimated the number of patients with newly diagnosed epilepsy who may eventually require surgery, based on an estimate of 30,000 incident cases in the UK, to be around 1.5% (95% CI 0.5% to 2.5%) or 450 patients a year. 13
In a 1998 study,9 the direct cost of epilepsy was estimated at £1568 per patient per annum. When seizure frequency was taken into account, the total cost of care for patients having more than one seizure per month was eight times that of patients who were SF (£3508 vs £443). Drug costs accounted for 23% of the overall cost, 43% of which was spent on the then new AEDs vigabatrin (Sabril®, Sanofi-Aventis) and lamotrigine (Lamicatl®, GlaxoSmithKline UK), which were prescribed for only 6% of patients. Since this study, several drugs, used either as monotherapy and/or adjunctive therapy, have obtained licences in the UK, including levetiracetam (2000) (Keppra®, USB Pharma Ltd), oxcarbazepine (2000) (Triteptal®, Novartis Pharmaceuticals UK Ltd), pregabalin (2004) (Lyrica®, Pfizer Ltd), zonisamide (2005) (Zobegran®, Eisai Ltd), rufinamide (2007) (Inovelon®, Eisai Ltd), lacosamide (2008) (Vimpat®, UCB Pharma Ltd)14,15 and, most recently, retigabine (2011) (Trobalt®, GlaxoSmithKline). A 2007 review estimated the cost of epilepsy in European countries at 2004 pricing levels. 4 The total cost (direct and indirect) ranged from 2000 euros (Estonia) to 11,500 euros (Switzerland); approximately 18% was direct health-care costs. 4 The total cost in the UK was estimated at approximately 8250 euros. 4
Current service provision
The work-up for epilepsy surgery aims to isolate the seizure focus and identify the underlying aetiology. 16 In the UK, all patients being considered for surgery will have had a detailed history taken, a clinical examination, psychometric testing (testing of memory and IQ for evidence of focal cognitive dysfunction), routine interictal and ictal EEG with surface (scalp) electrodes and routine MRI [T1, T2, with or without fluid-attenuated inversion recovery (FLAIR)]. The Wada test [whereby a short-acting barbiturate (sodium amytal) is injected into one carotid artery to anaesthetise part of one hemisphere, following which memory and language are tested in the opposite hemisphere] may also be conducted.
After these investigations, several potential courses of action may become available:
-
A seizure focus, features of mesial temporal sclerosis, or a lesion such as a tumour, vascular malformation, developmental malformation or post-trauma/infection malformation may be apparent. In these cases, surgery can proceed or, where surgery is not possible, further evaluation can be halted and an alternative strategy to best control seizures decided.
-
If there is a clear indication as to the site of the seizure focus the person may be admitted to hospital for continuous intracranial (invasive) EEG (iEEG) monitoring with electrodes inserted into, or across, the surface of the brain. During this monitoring, patients usually have their AED medication reduced to increase the likelihood of seizures; this is associated with a small risk of status epilepticus and death.
-
If there is no clear indication as to the site of the seizure focus, and it is considered unlikely that the patient will benefit from surgery, a decision might be made not to continue with the presurgical evaluation.
-
If none of the above apply A decision may be made to proceed with further, non-invasive, investigations, such as specialised imaging scans or magnetoencephalography (MEG).
Neuroimaging can provide information about structural and/or functional abnormalities. Neuroimaging technologies can be categorised according to their purpose:
-
Technologies identifying where important functions, such as language and memory, are located, so that they can be avoided during surgery. For example, functional MRI (fMRI). Although these are important in the work-up for surgery, the sole use of imaging technologies being used to identify the location of potential foci is being considered in this review. fMRI was included as a search term as this technology seems to have been used by some to assist in the identification of the seizure focus when combined with EEG. 17–21
-
Technologies that identify structural abnormalities and space-occupying lesions, such as tumours. For example, routine [T1, T2, with or without FLAIR; used in the UK prior to invasive EEG (iEEG) techniques] and volumetric MRI, quantitative T2 measurements and magnetisation transfer imaging (MTI).
-
Technologies that measure physiological activities, such as blood flow and metabolism. Changes in these functional activities can provide information about the site of seizure onset. For example, single-photon emission computed tomography (SPECT), positron emission tomography (PET), and magnetic resonance spectroscopy (MRS).
-
Technologies that provide information on both structure and function, such as diffusion tensor imaging (DTI) or diffusion MRI.
Currently, non-invasive tests are used primarily to guide the placement of the electrodes for iEEG. The main iEEG options are:
-
Depth electrodes Wires are inserted into the brain via a small hole drilled into the skull.
-
Subdural strips A strip of electrodes is placed over an area of the cerebral cortex, usually inserted via a burr hole.
-
Subdural mats A craniotomy is performed and a grid of electrodes placed over an area of the cerebral cortex.
-
Foramen ovale electrodes Wires are passed through the foramen ovale to lie under the mesial temporal lobe. These are rarely used in current practice but, given that a number of studies are expected to be restricted to patients with TLE, they are included in the list of suitable comparator tests to maximise the available data in this patient population.
However, the placement of the electrodes during an iEEG is crucial; a single electrode has a field of view of only several millimetres, and the electrodes need to include the site of the focus to successfully provide an accurate location. 22 In addition, invasive methods of EEG monitoring are associated with a small risk of infection and haemorrhage,23 and they cannot be repeated easily because of local scarring after an initial evaluation. 22 There are ethical considerations in using such a test in all patients. 24
Therefore, if sufficient confidence in the accuracy of non-invasive technologies to inform the decision to go to surgery, and the subsequent site of surgery in those who do proceed, can be achieved, they may have the potential to replace iEEG, at least in some patients.
Description of the technologies under assessment
This review evaluated a range of non-invasive technologies used to locate the epileptic focus.
Single-photon emission computed tomography
Single-photon emission computed tomography uses a radiolabelled compound that binds preferentially to certain areas of the brain, depending on the compound’s properties. The most commonly used methods during epilepsy surgery work up are technetium-99-labelled compounds [hexamethylpropylenamine oxime (HMPAO)] and ethyl cysteinate dimer (ECD). Once the tracer has been given there is a maximum 6-hour window in which to do the scan. Scans can be conducted interictally (not during a seizure), but more reliable information about the site of seizure onset is provided by injecting the radiolabelled compound at the start of a seizure (ictal) or just after it (post-ictal). Scans show an area of increased uptake at the site of seizure activity. Patients require simultaneous video-EEG monitoring and the presence of a member of staff who can give the radiolabelled compound as soon as the seizure starts.
Subtraction ictal single-photon emission computed tomography coregistered with magnetic resonance imaging
Subtraction ictal SPECT coregistered with MRI (SISCOM) subtracts the results of ictal and interictal SPECT images, and overlays the result on an MRI image.
Positron emission tomography
Positron emission tomography uses radiolabelled tracers; 15O-labelled water is used to assess blood flow (interictal only), [18F]-fluorodeoxy-d-glucose (18F-FDG) to assess glucose metabolism (ictal and post-ictal) and flumazenil to visualise the distribution of ionotropic gamma-aminobutyric acid A (GABAA) receptors in the brain. PET provides better spatial resolution than SPECT. FDG-PET is thought to provide more reliable results and provide better spatial resolution, and is thus more commonly used in selection for surgery.
Volumetric magnetic resonance imaging
Stereological techniques are used to estimate the volume of brain structures, most commonly the hippocampus, amygdala and temporal lobe. 25,26 Differences in volume, usually a reduction when compared with normative data, indicate focal pathology and, potentially, the site of the onset of seizures. Patients may have normal volumes, a unilateral abnormality or bilateral abnormalities that need to be taken into account when evaluating this technology. In addition, volumetric information is usually interpreted in conjunction with quantitative T2 data.
Magnetic resonance spectroscopy
Magnetic resonance spectroscopy is a magnetic resonance technology that is used interictally to measure the relative concentration of certain molecules in an attempt to find focal abnormalities that are consistent with the seizure focus. Proton spectroscopy can provide information about N-acetylaspartate (NAA), creatine lactate and choline-containing compounds, whereas phosphorus-31 spectroscopy provides information about phosphorus-containing compounds, such as adenosine triphosphate.
High-density scalp electroencephalography
High-density scalp electroencephalography uses surface electrodes as in routine EEG, but differs from routine EEG by using more electrode contacts (up to 256, compared with 21 usually used in standard EEG) and sophisticated data analysis strategies. 27
Magnetoencephalography
Magnetoencephalography measures the magnetic fields produced by electrical activity in the brain using sensitive devices such as superconducting quantum interference devices (SQUIDs). Advantages of MEG have been reported as high spatiotemporal resolution, insensitivity to conductivity differences (including skull defects and lesions), complementary sensitivity to EEG, high signal–noise ratio in superficial areas, focus localisation and functional mapping. 22 Disadvantages have been stated as metal implant artefact, cost, insensitivity to radial sources, less sensitivity to deep sources (gradiometers) and limited long-term monitoring feasibility, i.e. low likelihood of ictal recordings. 22
Magnetic source imaging
Magnetic source imaging (MSI) is MEG coregistered with MRI. The two sets of data are combined by measuring the location of a common set of points of reference; these are marked during MRI with lipid markers, and with electrified coils of wire that give off magnetic fields during MEG.
Diffusion tensor imaging
Diffusion tensor imaging is a magnetic resonance technique that quantitatively measures the magnitude and directionality of diffusion in a three-dimensional space.
Cost of the technologies under assessment
The cost of these diagnostic technologies can be high, for example £218 for MRI of one area with contrast (RA02Z),28 £215 for SPECT (RA37Z, nuclear medicine category 3),28 and £700–1000 for PET. 29,30 However, a recent study has shown that if seizure control is achieved after surgery, epilepsy-related costs are reduced in the 2 years after surgery from approximately US$2068 to US$2094 (in patients with persisting seizures whether they undergo surgery or not) to US$582 in SF patients. 16 Therefore, the cost of surgery, and the surgical work-up, may be offset by savings post surgery in those who attain a SF status.
Prior research
The commissioning brief for this health technology assessment (HTA) was to evaluate the diagnostic accuracy, clinical utility and cost-effectiveness of SPECT, MEG, PET and new forms of enhanced MR imaging. On clinical advice, we added high-density electroencephalography (HD-EEG) to the list of technologies of interest. The evaluation of any diagnostic technology can be summarised using the following framework suggested by Fryback and Thornbury;31 although a number of frameworks have been developed,32 this is the most comprehensive:
-
Level 1: Technical efficacy A preclinical phase developing the technical specifications of the test, such as image quality of a new imaging technology.
-
Level 2: Diagnostic accuracy efficacy The determination of diagnostic outcomes such as sensitivity, specificity and predictive values by using the test in patients with and without the disease/disorder.
-
Level 3: Diagnostic thinking efficacy The impact of the results of a diagnostic test on the treatment decisions made by clinicians.
-
Level 4: Therapeutic efficacy The frequency that the test influences clinical practice, such as the alteration of management decisions or avoidance of a procedure.
-
Level 5: Patient outcome efficacy The impact of the test on morbidity, mortality and/or quality of life (QoL).
-
Level 6: Societal efficacy The benefits and costs of the test from a societal perspective.
The requirement for studies to evaluate the clinical utility of diagnostic technologies in addition to their basic diagnostic accuracy characteristics has been reiterated by other authors. 31,33,34 The clinical utility of medical tests is measured by whether the information they provide affects patient-relevant outcomes, and although a medical test could directly affect outcomes, the effect is usually indirect by influencing downstream clinical decisions and treatment choices. 35 Clinicians need to know which diagnostic test contributes additionally to the estimation of disease probabilities given information that is already obtained. 34 Therefore, in order to inform clinical practice, studies need to investigate the clinical value of a test, and the impact of the results of that test on the decision-making process and subsequently on clinical outcomes and costs.
In 2006, an HTA report36 was published of a broad-ranging systematic review that evaluated neuroimaging technologies used to identify the seizure focus in patients with refractory epilepsy being considered for surgery. The prior HTA report highlighted a number of limitations of the evidence available at that time. The primary problem was a lack of effectiveness data, as this meant that the links between the test result, management decisions, and clinical outcomes could not be determined: of the 94 studies that met inclusion criteria, 75 assessed diagnostic accuracy and none of the studies identified assessed clinical effectiveness (none was a RCT or cohort study comparing patient outcomes between patients who received different combinations of imaging techniques) or cost-effectiveness. Studies providing knowledge about the added contribution of a test to diagnostic probability estimation are not commonly undertaken. 34 In the evaluation of diagnostic technologies used in the work-up for epilepsy surgery, the need for follow-up studies to quantify the effect of a diagnostic technology on patient outcome is particularly important because a reliable independent reference standard is not available. 36,37
The prior HTA report highlighted further serious limitations with the data from the diagnostic accuracy studies in imaging technologies used in the work-up for epilepsy surgery:
-
There is no established, reliable, reference standard test against which a new test can be compared.
-
When compared with a reference standard of other test(s), interpretation of the results was difficult; when the index test localised a seizure focus but the reference standard did not, it was unclear which technique was correct; where neither the index test nor reference standard identified a seizure focus, it was unclear whether the patients really did not have a seizure focus (i.e. had a generalised rather than a focal-onset epilepsy) or whether the tests were not able identify a seizure focus.
-
The data extracted could not be dichotomised, therefore standard measures of diagnostic performance [sensitivity, specificity, predictive values and diagnostic odds ratio (OR)] could not be calculated.
-
Imaging techniques were evaluated in isolation and did not allow an assessment of the effectiveness or diagnostic accuracy of tests used in combination.
-
Studies provided no data regarding the order in which tests should be carried out.
The last two limitations were considered to be major problems because in UK clinical practice the decision to undertake surgery and focus for excision is based on a consensus decision after multiple tests, which are conducted in sequence. The review recommended a large-scale cross-sectional diagnostic study in patients in whom epilepsy surgery may be indicated, in which all patients would first undergo the index tests under study and the combination of other diagnostic tests (such as history-taking, neurological examination and EEG), and a consensus decision made by an expert, multidisciplinary panel as to whether or not surgery is to be conducted. Such a study could assess which, and to what extent, imaging techniques contribute to the assessment of which patients should undergo surgery. At the time of the review, there was no consensus of opinion as to which combination of investigations should be conducted, what order they should be conducted in, or which investigation results should carry more weight when making recommendations for surgery, and this situation has not changed.
A systematic review conducted by Uijl et al. 38 evaluating a range of technologies used to lateralise or localise the seizure focus in patients with TLE, using the consensus diagnosis or the decision to perform surgery as the reference standard, was identified. 38 As did the prior HTA report, this review concluded that there was insufficient evidence to inform clinical practice, and recommended further studies evaluating the added value of consecutive tests on the decision-making process. Uijl et al. 38 recommended a study in which all patients with TLE who are potential candidates for temporal lobe epilepsy surgery were recruited during a specific period, to determine which diagnostic tests contributed to decision-making in the work-up for epilepsy surgery and in which order the tests should be performed. The patients would need to undergo the diagnostic tests in the chronological order commonly applied in clinical practice, and the results of each test documented for each patient along with the final decision ‘surgery or not’ as made by a multidisciplinary team using the consensus diagnosis method. With the consensus decision being made based on all available patient information, this would be considered an appropriate reference standard. From such a study, a multivariate analysis and modelling of the decision-making process could be undertaken, showing which test parameters contribute to the final decision for or against surgery. 38 Without such studies, the ability to assess the added clinical value of these tests beyond basic diagnostic accuracy was extremely limited.
The serious limitations of diagnostic accuracy studies and the lack of evidence on the impact of the results of these tests on patient management decisions and outcomes mean that there is still uncertainty as to how well these technologies perform in clinical practice.
Chapter 2 Definition of decision problem
Decision problem
In relation to the use of specialised, non-invasive, imaging or EEG technologies in the surgical work-up of epilepsy patients, the decision problem in clinical practice is ‘What is the clinical effectiveness and cost-effectiveness of non-invasive technologies over and above routine EEG/MRI, and what if any further diagnostic procedures should be undertaken in individuals for whom there is a reasonable hypothesis for the site of the seizure focus, but in whom that focus has not been reliably identified after the initial surface EEG and MRI?’
Overall aims and objectives of the assessment
The project attempted to address the question of the clinical effectiveness and cost-effectiveness of non-invasive technologies and, where possible, combinations and sequences of these technologies, for the presurgical work-up of patients with refractory epilepsy. A systematic review of the clinical evidence was conducted to identify studies that evaluated the clinical utility and diagnostic accuracy of the non-invasive technologies of interest. A review of the cost-effectiveness was also undertaken, and the added value of these technologies over and above routine surface video-EEG and MRI, to confirm the epileptic focus in people with indeterminate routine surface EEG/MRI results, was investigated using a decision-analytical model. Diagnostic ‘algorithms’, in which invasive tests were involved, were included in this exploration as these reflect current UK clinical practice.
The aims stated in the protocol were developed into a series of questions, which were addressed in a phased review:
-
What is the diagnostic accuracy of the non-invasive technologies of interest, and what are the limitations of the data from these studies when addressing such a decision problem?
-
Is a localising non-invasive test associated with a good outcome following surgery?
-
How do these non-invasive technologies impact on the decision-making process?
-
Which, if any, of these non-invasive tests, alone or in combination/sequence, is the most cost-effective option for patients with refractory epilepsy who are undergoing presurgical work-up?
-
What are gaps the in the current evidence base, how can these be addressed, and how can the new evidence be incorporated into the decision-analytical model?
To address the issues of a relevant reference standard and to capture studies of effectiveness, the current review searched for RCTs and restricted the inclusion of diagnostic accuracy studies to those that reported a decision to undertake surgery and/or the outcome following surgery. To reflect current practice, where only the decision to go to surgery was reported in a study iEEG had to be an option during the work-up for surgery.
On the basis of previous reviews of non-invasive tests in epilepsy and other methodological reviews, it is clear that studies should be considered within an overall framework to evaluate diagnostic and clinical utility as well as cost-effectiveness in order to inform decision-making in the NHS concerning the relative value of these tests. In Chapter 3, this report discusses in detail the reasons that diagnostic accuracy data are not sufficient to address such a clinical question. A decision-analytical approach is presented Chapter 4, which provides a potential framework for combining this information with additional resource use and value parameters that are also required to inform decisions concerning the cost-effectiveness of tests. The different approaches that may be used to obtain the information required to address the decision problem are provided in Chapter 5.
Chapter 3 Assessment of clinical effectiveness
Methods for reviewing effectiveness
The systematic review was conducted in accordance with CRD’s guidance for undertaking reviews in health care39 and Preferred Reporting Items for Systematic Reviews and Meta-Analyses (PRISMA). 40,41
Identification of studies
The screening of titles and abstracts was conducted by two independent reviewers. All potentially relevant studies were retrieved where available, and two independent reviewers applied the inclusion criteria to the full papers. Disagreements were resolved by discussion. Where consensus could not be reached at the title and abstract stage, the full paper was ordered. Where consensus could not be reached at the full-paper stage, a third reviewer was consulted. Abstracts were included if no associated full paper was identified, and there were sufficient outcome data to extract. Foreign-language papers were excluded unless there was an English- language translation available or if the study had been extracted for the previous review. 36
Search strategy
The literature search aimed to determine the diagnostic accuracy and clinical utility of SPECT, MEG, HD-EEG, PET and specialist MRI technologies in defining the seizure focus in patients with refractory epilepsy who were being considered for surgery.
The base search strategy was constructed using MEDLINE and then adapted to the other resources searched. The search included the following components:
-
epilepsy terms, and
-
imaging technology terms, and
-
surgery terms.
No diagnostic filters were used. The search was restricted to the period 2003 to date; older studies were harvested from a previous systematic review. 36 No language restrictions were used in the search.
Search terms were identified by scanning key papers that were identified at the beginning of the project, through discussion with the review team (including clinical experts) and the use of database thesauri. The creation of the search strategy was an iterative process originally using the MEDLINE database and then adapted as appropriate to the other sources searched. The search strategy was checked by a second information specialist.
The following databases were searched for relevant studies:
-
MEDLINE In-Process & Other Non-Indexed Citations and MEDLINE (via OVID, 2003 to July week 2 2010)
-
BIOSIS Previews (via Dialog, 2008–11/August week 5)
-
BIOSIS Previews (via Web of Knowledge, 2003–8)
-
ClinicalTrials.gov (via website www.clinicaltrials.gov/ to July 2010)
-
Cochrane Database of Systematic Reviews (CDSR) (via Wiley, The Cochrane Library website Issue 7 of 12, July 2010)
-
Cochrane Central Register of Controlled Trials (CENTRAL) (via Wiley, The Cochrane Library website Issue 7 of 12, July 2010)
-
Cochrane Register of Diagnostic Studies (searched on request by a Cochrane Trials Search Coordinator)
-
Current Controlled Trials (CCT) (via website www.controlled-trials.com/ to August 2010)
-
Database of Abstracts of Reviews of Effects (via Wiley, The Cochrane Library website Issue 7 of 12, July 2010)
-
EMBASE (via OVID, 2003–10 week 28)
-
HTA database (via Wiley, The Cochrane Library website Issue 7 of 12, July 2010)
-
Inside Conferences (via Dialog, 2003–10/September 20)
-
Latin American and Caribbean Health Sciences Literature (LILACS) (via website http://bases.bireme.br/cgi-bin/wxislind.exe/iah/online/ July 2010)
-
Medion (via website www.mediondatabase.nl/ July 2010)
-
PASCAL (via Dialog, 2003–10/August week 5)
-
Science Citation Index (SCI) (via Web of Knowledge, 2003 to July 2010)
-
WHO International Clinical Trials Registry Platform ICTRP (via website http://apps.who.int/trialsearch/AdvSearch.aspx to August 2010).
The reference lists of included papers and relevant reviews were scanned for additional relevant studies. A citation search of key papers in the field was carried out. Records were managed within an EndNote library (EndNote version X1, Thomson Reuters, CA, USA). The full search strategy is reported in Appendix 1.
Inclusion and exclusion criteria
Following development of the protocol review questions (see Chapter 2), the protocol was amended to include a broader range of studies. The protocol amendment impacted only on the designs of studies eligible for the review (see Chapter 3, Study designs). Studies were sought in order to address three clinical questions relating to the use of non-invasive technologies in patients with refractory epilepsy being considered for surgery.
Index tests being evaluated
The index tests evaluated in this review were non-invasive technologies: HD-EEG, relevant specialist MR technologies (volumetric, functional, MRS), SPECT, PET, MEG, SISCOM (SPECT and MRI coregistered), and MSI (MRI and MEG coregistered). To be included, studies using magnetic resonance technology had to use a magnet of 1.5 tesla (T) or above. Studies evaluating routine MRI, computed tomography (CT), or near-infrared spectroscopy (NIRS) were excluded. Routine MRI is standard practice during the early work-up prior to epilepsy surgery in the UK, and given its availability and ability to identify structural lesions such as tumours and vascular malformations, its role is not being questioned. CT has been superseded by other technologies, and NIRS has limited applications and is not seen as a viable option for routine practice. Studies reporting the location of memory or language centres only using fMRI were also excluded.
Population
We restricted inclusion to the population being considered for surgery who were most likely to have a successful localisation and clinical outcome, i.e. people with simple or complex refractory focal epilepsy who did not experienced generalised seizures. Studies in patients with tumours, vascular malformations or epilepsy as a result of trauma were also excluded, as these are often identified by routine MRI, which is not being evaluated in this review. 36 Adult was defined as ≥ 18 years. Studies of patients with psychogenic seizures were not included in the review.
Study designs
Study designs were sought that could address each of the research questions identified. To determine the diagnostic accuracy of the tests and their association with surgical outcome in those who underwent an excisional procedure, we sought:
-
Prospective diagnostic accuracy studies of a single-gate (cohort) design (‘diagnostic accuracy studies’). Studies that compared test results in patients suffering epilepsy (cases) with test results in healthy people (control subjects) in order to determine diagnostic accuracy were excluded, unless the required outcomes are reported separately for cases.
-
Studies that undertook a multivariate regression analysis in which an index test(s) of interest was an independent variable (‘outcome prediction studies’).
Studies had to include at least 20 patients to be included; we used this as a cut-off to distinguish between a case series and a diagnostic cohort. Where studies reported the results of a mixed population of which a subgroup met the inclusion criteria, or were case–control in design, there had to be at least 20 patients in subgroup/20 cases for the study to be included.
To evaluate the clinical utility of the diagnostic tests, and therefore their impact on the decision-making process and clinical outcomes, we sought:
-
RCTs of any size directly comparing two or more diagnostic tests of interest that reported the number in each arm that progressed to surgery and/or post-surgical outcome.
-
Cohort studies comparing outcomes between patients who received different combinations of imaging techniques that reported the decision to go to surgery and/or outcome following surgery.
-
Studies with at least 30 patients that reported the impact of an index test(s) of interest on the decision to go to surgery, and also reported the outcome following surgery (‘decision studies’).
No limit on sample size was imposed for the RCTs or cohort studies. The number of patients required for the decision studies was higher than that imposed for the diagnostic accuracy and outcome prediction studies, as both the decision to go to surgery and the outcome following surgery, and therefore a broader population, were required. Determining this cut-off was difficult, as we thought these types of studies would be rare, and we wanted to maximise the data available.
Comparators
Diagnostic accuracy studies
There is currently no accepted ‘reference standard’ or ‘gold standard’ test against which new technology can be compared as would normally be the case in diagnostic accuracy studies (this is discussed in detail in Methodological limitations of the diagnostic accuracy studies. In UK clinical practice, localisation of the focus, the subsequent decision to undertake surgery or not, and the site where excisional surgery is to be conducted, are generally based on a consensus of a combination of tests. The tests included in this sequence vary considerably across assessment centres depending on preference of clinician and patient, and the availability and cost of the test. Therefore, this review considered two reference standards:
-
The decision to undertake surgery and/or the site of surgery For studies reporting this but not outcome following surgery, routine MRI had to have been performed either as part of the reference standard or as a index test (to indicate the number of patients localised on MRI who in UK clinical practice may not have undergone further non-invasive evaluation), and iEEG had to be available as an option (depth electrodes, subdural strips or mats, or foramen ovale electrodes in patients with suspected mesial TLE). This was a change to the original criteria stated in the protocol, in which the decision to go to surgery had to have involved iEEG. This revision was made to reflect UK clinical practice; iEEG is an option for clinicians, but is not always conducted. The tests used to determine whether or not to undertake surgery, or the site of surgery, will be called the comparator tests; this may sometimes include the index test(s).
-
Outcome following surgery There was no restriction on inclusion based on how outcome following surgery was defined. Where possible, the appropriate Engel classification is given when alternative definitions were used by the authors. Data for different thresholds of a good outcome (SF/Engel Ia, Engel I and II, etc.) were extracted. Studies restricted to patients who had a good (or bad) outcome after surgery were excluded.
Studies comparing the results of the index test with one or more other tests without reporting any subsequent decision regarding surgery, or surgical outcome, were excluded.
Outcome prediction studies
The index test of interest had to be one of the independent variables in a multivariate regression in which outcome following surgery (however defined) is the dependent variable. Therefore, the index test was compared against other test results and/or epidemiological factors in terms of their association with the patient’s outcome following surgery.
Randomised controlled trials
The index diagnostic strategy had to be compared with an alternative diagnostic strategy; either arm could be an individual test or a combination/sequence of tests.
Decision studies
The requirement for decision studies was not a comparator test, but consensus diagnosis after an initial routine MRI and EEG, and the reporting of a modification of this diagnosis after the conduct of an index test, along with the final decision to go to surgery and the outcome following surgery.
Outcomes
Diagnostic accuracy studies reporting sufficient data to construct a contingency table were included. Studies reporting diagnostic outcomes that are derived from 2 × 2 contingency tables were also included. Outcome prediction studies had to report at least a p-value for an index test of interest, which was included as an independent variable in a multivariate regression analysis. RCTs were included if they reported any clinical outcome and/or QoL. Decision studies had to report the number of patients for whom the management strategy remained the same or changed, the final decision as to whether the patient was eligible or not for surgery, and the outcomes following surgery for those who underwent an excisional procedure.
Data extraction strategy
Data extraction forms were developed using Microsoft Access 2007 (Microsoft Corporation, Redmond, WA, USA), which was piloted on a small number of studies. Information relating to study design, patient populations, index test(s), comparators and methodological quality were extracted by one reviewer and checked by a second; disagreements were resolved by discussion. Where multiple publications of the same study were identified, data were extracted and reported as a single study. No RCTs were identified that met the inclusion criteria.
Data from the diagnostic accuracy studies were extracted in order to construct 2 × 4 contingency tables (Table 1). Sensitivity and specificity were extracted where reported when a contingency table could not be constructed.
One of two reference standards | ||
---|---|---|
Decision for surgery or good outcome following surgery | Decision against surgery or poor outcome following surgery | |
Concordant | A | B |
Non-localising | C | D |
Partially concordant | E | F |
Discordant | G | H |
To gain the maximum clinical utility from the data, we classified the index tests based on whether they were concordant, non-localising, partially concordant or discordant (therefore defining the rows of the 2 × 4 table) with either the final consensus localisation of the comparator tests (where the reference standard was the decision to go to surgery or not) or the site of surgery (where the reference standard was outcome following surgery):
-
Concordant The focus identified by the index test is the same as that identified by the comparator tests/final diagnosis, or the site of surgery (not concordance with the final decision whether to go to surgery, or outcome following surgery); this includes results where none of the tests was localising, as there was concordance that no focus could be identified.
-
Non-localising The comparator tests localised a focus, or surgery was undertaken, but the index test was non-localising.
-
Partially concordant The focus identified by the comparator tests/site of surgery overlapped, but was not identical to, the focus identified by the index test.
-
Discordant The focus identified by the comparator tests/site of surgery was a different focus to that identified by the index test.
Where possible, attempts were made to determine whether the final decision as to the site of surgery for the partially concordant and discordant test categories was based on the results of the comparator tests or the index test, when the decision was to go to surgery was the reference standard. From the 2 × 4 contingency tables in which outcome following surgery was the reference standard, hit, miss and error rates were calculated; the definitions and method of calculation are described in detail in Methodological limitation of the diagnostic accuracy studies. This terminology was adopted from Wheless et al. ,42 who reported the results of their test evaluations in this fashion.
Given the nature of the data reported in the diagnostic accuracy studies, there was a level of subjectivity surrounding the extraction of the data into the 2 × 4 contingency tables. This resulted in some disagreements between reviewers that could not be resolved by discussion. In these cases, the final decision was made by the team member with the most experience in epilepsy and the conduct of systematic reviews of diagnostic data (JB). In order to allow reproducibility of the extraction of the diagnostic accuracy data, and for readers to assess the methods used to derive the 2 × 4 contingency tables, a detailed description of these methods is provided for each study alongside the data extraction tables (see Appendix 3).
From outcome prediction studies the analysis used, measure of association, the dependent variable and independent variables included in the model, and the p-values for the individual index test(s) of interest and the model were extracted.
Data from the decision studies, the number of cases in which the index test resulted in a change, (or no change) in the management strategy to be undertaken, the outcome following surgery when an excisional procedure was undertaken, and the predictive values for each of the tests conducted, were extracted.
Critical appraisal strategy
The basis of the quality assessment tool for all three included study designs was the Quality Assessment of Diagnostic Accuracy Studies (QUADAS). 43 Some criteria were used either during the study selection process or were considered not to be appropriate for this review, and were therefore omitted from the final quality assessment. These were:
-
The appropriateness of the reference standard and partial verification bias The review was restricted to studies that reported the final decision to go to surgery or the outcome following surgery.
-
Differential verification bias Given the nature of this area of medicine, some foci are easier to identify than others, and patients will invariably have different combination of tests during their presurgical work-up. This reflects clinical practice and there may be ethical issues associated with withholding a test that could be beneficial, or conducting a test that could have adverse events associated with it if the prior tests have confirmed a seizure focus. In order to reflect clinical practice, we required iEEG to be an option where the decision to go to surgery was used as the reference standard.
-
Progression bias This criterion was not considered appropriate, as patients were known to have severe epilepsy, and it would be very unlikely that the seizure focus or severity of epilepsy would change between tests, even with considerable delays.
The QUADAS43 is a quality assessment tool specifically for diagnostic accuracy studies, and although these were the majority of the included studies, we included other study designs that required criteria relating more generally to observational studies. Two quality assessment tools suitable for this assessment are the Newcastle–Ottawa Scale for cohort studies44 and Downs and Black. 45 There are a number of areas of overlap between these scales and QUADAS,43 such as the assessment of spectrum bias, blinding of assessors and attrition bias. Criteria added to the quality assessment tool for the cohort studies were:
-
Was there sufficient description of the groups and distribution of the prognostic factors?
-
Was the test reliably evaluated in the outcome prediction studies?
-
Were the groups in the outcome prediction studies comparable on all important confounding factors?
-
Was there adequate adjustment for the effects of all important confounding factors in the outcome prediction studies?
One criterion from the observational scales was applied to all studies:
-
Was follow-up at least 1 year on all patients?
Criteria included in these tools for assessing the quality of observational studies that were not assessed during the quality assessment were:
-
The dose–response relationship between intervention and outcome This item was not considered relevant to these studies.
-
Adverse events We extracted the rate of adverse events wherever reported, therefore the absence of these outcomes were captured elsewhere.
-
Reporting of probability values These were only relevant to the outcome prediction studies, and a minimum requirement for inclusion was the reporting of a p-value for the index test of interest in the multivariate regression.
-
Was there evidence of ‘data dredging’ This did not apply to the included studies.
-
The use of a power calculation We restricted inclusion to studies based on a minimum number of required participants; the reporting of a power calculation is not common in diagnostic studies, and any further judgement as to what was an ‘adequate’ sample size on our part would have been subjective.
-
Date of recruitment Although in Downs and Black45 this relates to whether the intervention and control groups were drawn from the some populations in time, this does have some relevance for our review due to the rapid advancement in the diagnostic technology in this area. However, we restricted our review to studies that used MRI with a magnet strength of ≥ 1.5 T, so excluding older studies using technology deemed to be out of date in the UK; clinical advisors to the project were unable to identify significant changes in the specification of the other technologies being evaluated that would now be considered out of date, therefore any other criteria excluding technologies on this basis would be subjective.
Study quality was assessed by one reviewer and checked by a second; disagreements were resolved by discussion, or referral to a third reviewer.
Methods of data synthesis
Given the substantial clinical heterogeneity across the included studies, a narrative synthesis was used for each type of study design separately. Differences between studies are discussed in the text, and study details were tabulated in Appendix 3.
Some calculations were conducted on data extracted from the diagnostic accuracy studies.
Where individual patient data (IPD) were reported, binary logistic regression was conducted where there were sufficient data.
Likelihood ratios were calculated for each test category of the 2 × 4 contingency table to indicate whether the odds of a decision to go to surgery were greater or outcome following surgery was different between concordant, non-localising, partially concordant and discordant tests. The calculations used are given in Box 1.
Decision for surgery or good outcome following surgery | Decision against surgery or poor outcome following surgery | |
---|---|---|
Concordant | A | B |
Non-localising | C | D |
Partially concordant | E | F |
Discordant | G | H |
Totals | T+ | T– |
There are also limitations with the use of likelihood ratios in this indication. The likelihood ratios cannot be used to indicate the odds of a decision to go surgery or good outcome following surgery associated with a particular index test result, as the rows of the 2 × 4 tables are defined by concordance of an index test, either with the final diagnosis based on the comparator tests or on the site of surgery, depending on the reference standard used. They do not reflect the chance of a ‘correct’ decision, but rather reflect the change in the odds of the outcome. Therefore, the information that can be gained from these data is by a comparison of the likelihood ratio between concordant tests and the other index test categories. When considering the decision to go to surgery, if the results of an index test are given credence by the interpreter, the likelihood ratios will be higher in the concordant and partially concordant categories (as the confidence in the decision would be strengthened) and lower in the non-localising and discordant categories (as there would be less confidence in the location of any identified focus and therefore are less likely to go to surgery). Conversely, if there is no difference in likelihood ratio between the concordant and discordant categories, or the likelihood ratio is higher in the discordant category, the index test is of little or no use (or even counterproductive) when deciding whether surgery should be conducted and where. For the outcome following surgery, if the results of an index test alter the odds of a good outcome then the likelihood ratios will be higher in the concordant and partially concordant categories than those in the non-localising and discordant categories. Conversely, similar likelihood ratios across these categories would indicate no difference in the change in the odds of a good outcome based on the conduct of the index test. However, there are a number of factors that can have an impact on surgical outcome other than the accuracy of localisation. The likelihood ratios presented must, therefore, be interpreted with caution; the differences in likelihood ratios between categories need to be substantial and consistent across similar populations, if they are to be used as indicators of the benefit of conducting the index test.
Methodological limitations of the diagnostic accuracy studies
There are three major limitations to the use of the included diagnostic accuracy studies:
-
lack of an established reference standard
-
difficulties with extracting data into 2 × 2 tables of test performance
-
difficulty interpreting the data from the 2 × 4 contingency tables.
These will be discussed in turn, and must be kept in mind during the interpretation of the data in the subsequent results section (see Results).
Lack of an established reference standard
In some areas of medicine there is no agreement as to what constitutes an ideal reference (gold) standard. Where there is no widespread agreement, a test cannot be considered as a gold standard. Consensus is required in order to gain reliability; a diagnosis made using agreed-on criteria in one setting is more likely to be made the same way in a different setting than would be the case when no such consensus exists. 46 Using an imperfect reference standard will directly lead to bias in accuracy estimates. 47 The direction of the bias depends on whether errors by the index test and imperfect reference standard are correlated (a positive correlation will inflate the estimates of accuracy) and the magnitude will depend on the frequency of errors by the imperfect reference standard and the degree of correlation in errors between index test and reference standard. 47 There is currently no agreed, consistently measurable, independent, reference standard against which new technology to identify a seizure focus can be compared. 22 This was highlighted as a problem in the prior HTA report. 36 The most commonly used reference standards include continuous video-EEG, iEEG, consensus from a combination of tests, latent class models, and outcome following surgery; the limitations of each of these are discussed in turn.
Continuous video-EEG monitoring for a number of days to record ictal activity directly assesses the seizure onset but ictal EEG using surface electrodes may fail to find a seizure focus or may localise it inaccurately, particularly when the seizure activity arises from parts of the cerebral cortex some distance from the scalp. 36 Although video-EEG is a useful diagnostic modality, the proportion of patients in whom useful data were collected could not be considered sufficient for a gold standard test. 48
Invasive/intracranial EEG is considered the final decisive test of surgical decision-making in the large subpopulation of surgical candidates who do not have either localised ictal EEG or MRI findings, and therefore considered by some to be a suitable reference standard. 49 iEEG has very high sensitivity;22 however, there are significant limitations for its use as a reference standard. First, the placement of the electrodes is crucial; a single electrode has a field of view of only several millimetres, and the electrodes need to include the site of the focus to successfully provide an accurate location. 22,36,50 iEEG has been shown to have a relatively high percentage of non-localisation, negatively affecting diagnostic measure of the index tests being evaluated when used as a gold standard. 51 In addition, there is a potential for complications such as infection and bleeding,23 and the ability to repeat the test is limited because of local scarring after an initial evaluation,22 therefore there are ethical considerations in using such a test in all patients, which means that verification will be in selected cases only and can lead to biased estimates of sensitivity and specificity. 24
Combination of test results is often used as the reference standard, which is the currently the case when localising a seizure focus. 24,36 The composite reference standard used in studies needs to include a combination of tests which provide results that are meaningful when defining the target condition, and the likelihood for residual misclassification considered. 47 However, there is no consensus as to which tests should constitute this combination, and the combination/sequence of tests varies considerably across studies. Some concerns with the use of a composite of tests as the reference standard for the localisation of a seizure focus are:
-
The subjective nature of the interpretation of the test results, the subsequent observer variation, and the potential that the post-test probability could be a function of the accuracy of individual observers rather than the overall accuracy of the diagnostic pathway. 52,53
-
The destabilisation of a correct diagnosis with continued testing due to doubt being cast by additional information or the new information sufficiently changing the post-test likelihood as to suggest an alternative diagnosis. 52
The use of an expert panel to discuss test results and produce a consensus decision as to the presence or absence of the target condition based on all sources of information helps address these concerns with the use of a combination reference standard, and is an appropriate approach to address the issue of imperfect reference standard. 47 A number of factors have been identified as affecting the reliability of a consensus-based diagnosis by a multidisciplinary team – namely the number of experts and expertise mix, the way patient information is presented, and how to obtain a final classification54 – and these factors would need to be reported in order to determine the reliability of the reference standard.
An alternative to the consensus method is the use of a statistical model for combining multiple test results: latent class models. 47 Latent class models relate the observed patterns of test results to latent categories of patients with and without the target condition; using this analysis, specific test results associated with the patient categories can be identified, and sensitivity and specificity of the tests can be estimated. 47 A potential benefit of these models is their objectivity, as they examine the strength of statistical relationships among variables; the main disadvantage is that the target condition is not defined in a clinical way, so there can be lack of clarity about what the results stand for in practice. 47
Outcome following surgery could be considered the ultimate reference standard; however, surgical outcome can be affected by known and unknown variables that are unrelated to the accuracy of test localisation,22 and is therefore subject to a number of limitations as a reference standard. First, many individuals may not proceed to surgery, therefore surgical outcome does not provide information for the proportion of patients who are assessed and for whom the decision not to undertake surgery is made. Second, the index test may have been used in making the decision to proceed with surgery. These two factors would tend to increase the estimated diagnostic accuracy of the index test. 36 Third, the success, or otherwise, of surgery can be influenced by factors other than the results of the localisation decision, such as intra- or postoperative complications, incomplete resection of the seizure focus, excision of adjacent tissue to the focus identified that included the seizure focus, and post-surgical management regimen. Finally, surgical follow-up has to be sufficiently long in order to determine the persistence of the surgical outcome. 50
The major limitation that afflicts all reference standards that are currently available for diagnostic technologies for the work-up for epilepsy surgery is the inability to verify whether the index test was accurate, and whether the decision not to undertake surgery was appropriate. Neither iEEG nor SF surgical outcome is considered to reliably reflect epilepsy localisation, even in well-designed studies that minimise ascertainment bias and lack of independence,22 and there is no consensus as to the most appropriate combination reference standard.
In light of these limitations, and the need to determine the impact of test results on the decision-making process, this review concentrated on studies that reported the decision to undertake surgery or not based on the results of tests (where iEEG was an option in order to reflect clinical practice), and outcome following surgery using a range of thresholds for the definition of a good outcome; these are currently the best available, although imperfect, reference standards. The difficulties with extracting and interpreting the data from these studies are discussed in the following sections.
Difficulties with producing 2 × 2 tables of test performance and standard diagnostic outcome measures
The prior HTA report stated that the inability to dichotomise the data in order to construct 2 × 2 tables of test performance was a major limitation of the data from the diagnostic accuracy studies, as standard measures of diagnostic performance, such as sensitivity, specificity, and predictive values, could not be calculated. 36 In other areas of medicine for which an extended contingency table such as this is presented (risk stratification, cancer staging, threshold categories), the data can be dichotomised by selecting a single threshold (e.g. stages 1 and 2 vs stages 3 and 4). These approaches always risk reducing the applicability of the information derived, but with an advantage of increased statistical precision.
However, the previous review dichotomised the data when outcome following surgery was used as the reference standard in order to produce relative risks. 36 In order to collapse the data into a 2 × 2 table, the number of concordant and partially concordant tests were combined, as were the number of non-localising and incorrectly localising tests. Although combining the concordant and partially concordant tests may be valid (to produce an ‘at least partially correct’ category), combining the non-localising and discordant tests would mean combining two distinct populations for which the consequences of the test results would be very different. The consequences for patients non-localising and discordant tests would be significantly different; a non-localising test would likely result in a patient receiving medical management (MM), but an incorrect localisation could lead to surgery at an incorrect location, which could leave the patient with continued seizures, transient or long-term complications, alterations of function, and potentially alteration of personality. In addition, the combined non-localising and incorrectly localising categories will represent false- and true-negative tests; although the non-localising tests can be allocated as true- or false-negatives, discordant tests identified a focus and therefore are positive, and cannot be classified as negative tests.
Difficulties interpreting the data from the 2 × 4 contingency tables
Given the disadvantages of collapsing the data into a 2 × 2 table of test performance, we tried to determine (1) the proportion of scans that contributed to the decision whether or not to undertake surgery when a decision to go to surgery was the reference standard and (2) the proportion of patients in whom the index test identified a focus correctly, incorrectly or missed a resectable focus in those who underwent surgery. In order to do this, we would need to be able to classify the scans in each of the cells of the 2 × 4 contingency table (Table 2). However, this proved problematic. In order to determine whether or not the test was concordant for entry into the 2 × 4 contingency table, subjective decisions had to be made by the reviewer for some test results, which means that the numbers in each cell could vary with differences in the opinions of those interpreting the data. The full data extraction is reported in Appendix 3, along with a detailed description of how the 2 × 4 tables were derived for each study.
Decision for surgery | Decision against surgery | |
---|---|---|
Concordant | A | B |
Non-localising | C | D |
Partially concordant | E | F |
Discordant | G | H |
For studies reporting the decision to go to surgery or not, the following assessment of each cell of the 2 × 4 table (see Table 2) were considered.
-
A and B Concordance between the comparator tests (generally a consensus diagnosis) and the index test means that the decision to go to surgery (or not) may have been the same whether the established tests or the index test were used alone or in combination. Therefore, it could be argued that there was no value in conducting the index test in these patients. However, it could also be argued that the index test increased confidence in the site of the seizure focus and subsequently the decision to go to surgery or that the index test could have been used instead of the original combination of tests, avoiding the use of iEEG. None of these alternatives can be proven for any individual study or case within a study.
-
C and D Given a focus was identified by the comparator tests, the non-localising index test provided no additional information regarding the potential site of excision, and may cast doubt on the results of prior tests. Where the decision was not to go to surgery, we cannot tell from these data whether it was due to uncertainty of the location of the seizure focus as a result of the non-localising index test, discordance of individual comparator tests, or other reasons. Therefore, the change in the confidence, if any, and influence on the surgical decision cannot be reliably determined in these cells.
-
E and F The partial concordance of the index test with the comparator tests means that there may have been some added value in the performance of the test, in terms of increased confidence in the focus where there is partial overlap. It may be that different tests contained in the combination of comparator tests gave slightly different foci, and the index test may show closer concordance with some tests than others. Alternatively, additional potential sites for the seizure focus indicated by the index test may decrease confidence in the potential benefit of surgery and may have led to a move away from an operative approach. The confidence the surgical team places on the results of each of these tests will determine whether or not the index test seems to be confirmatory of the focus. The data presented do not allow the true additional value of the index test to be determined by this route.
-
G Despite the discordance between tests, surgery was performed. If the site of excision was based on the results of the comparator tests then the index test provided no added value, and indeed may have made the decision to undertake surgery more difficult. However, if the excision was at the site identified by the index test, there was not only value to undertaking the additional test, but there was believed to be greater value in the results of the index test than the other tests.
-
H Although the index test was discordant with the comparator tests, and there was a decision not to go to surgery, this may be due to uncertainty surrounding the focus as a result of the index test, discordance between the individual comparator tests or maybe other reasons why surgery was not considered appropriate, therefore the value of the index test in making this decision is completely uncertain.
From the descriptions for each cell of the 2 × 4 table, it can be seen that it is not possible to determine whether or not an index test was of value in the decision-making process using data from studies of a diagnostic accuracy design. This was compounded by the difficulties encountered when categorising test results to the cells of the 2 × 4 table during data extraction; results of the range of comparator tests were sometimes reported separately, with the site of surgery being the only consensus decision. In studies in which the site of surgery was based solely on the comparator tests, classification was relatively straightforward; however, the index test contributed to the final localisation decision in some studies. Detailed descriptions at to how we extracted data from each study are reported in Appendix 3, indicating which patients were difficult to classify and how we made our final decision. Our method was just one of several that could have been used to classify these patients; differences in interpretation of the data by different reviewers could significantly alter the conclusions drawn.
Even where IPD was presented, the reviewer could not determine the contribution of a test result to the final decision, as different tests would carry different weight and some test results would be clearer than others. When data could be extracted into a 2 × 4 contingency table, any generalisation applied to all of the test results in any one cell of that table is inappropriate; some concordant, partially concordant or discordant tests could be crucial in the decision-making process, whereas others are not, and non-localising test results could cast sufficient doubt on the site of a seizure focus to alter the management plan for a patient.
It is apparent that without clear documentation from the clinical teams detailing the process of decision-making, it is not possible to reliably classify tests in order to determine whether or not they provide added value during the decision-making process from such data sets.
Only the clinical team interpreting the test results can give an indication on whether or not a particular test result was informative for the decision-making process, and this level of information needs to be recorded at the time the study is being conducted. Therefore, in order to determine the impact of an index test on the management strategy for patients being considered for epilepsy surgery, the consensus decision pre- and post-index test(s) needs to be recorded at the time the study was conducted; the diagnostic accuracy studies as reported are inappropriate to evaluate the results of diagnostic test in this context.
Similar problems were encountered when the outcome following surgery was used as the reference standard. As with the decision to go to surgery, data regarding outcome following surgery were extracted from the diagnostic accuracy studies into 2 × 4 contingency tables (Table 3).
Good outcome following surgery | Poor outcome following surgery | |
---|---|---|
Concordant | A | B |
Non-localising | C | D |
Partially concordant | E | F |
Discordant | G | H |
For each cell of the 2 × 4 table, we tried to determine whether the test was a hit, miss or error, as described below:
-
A The index test identified the focus at which surgery was conducted, and the outcome from surgery was good; this shows that the index test of interest was correct (Hit). It could be argued that the index test may have been sufficient to confirm the seizure focus, and an iEEG avoided. However, there is the possibility that the tissue resected was more extensive than that identified by the tests, and the seizure focus could be the contained within the additional resected tissue that was not identified by the tests.
-
B The index test identified the seizure focus at which surgery was conducted but the outcome from surgery was poor, therefore these tests could be classified as Errors. However, a poor outcome following surgery could occur after surgery at the correct location due to intra- or postoperative complications or an incomplete resection of the focus, and therefore these tests are Unclassifiable.
-
C The index test was non-localising but the comparator tests identified a focus; surgery was conducted, and surgical outcome was good. Therefore, the comparator tests identified the correct seizure focus, and the index test failed to identify a resectable focus; the index test was a Miss.
-
D The index test was non-localising but the comparator tests identified a focus and surgery was conducted, but the outcome following surgery was poor. Therefore, the correct focus was identified by the comparator tests, but there were intra- or postoperative complications or incomplete resection of the focus; the focus identified by the comparator tests was not the correct focus, or only part of the focus; or the non-localising result of the index test was correct and the patients has no resectable focus. The correct interpretation and classification of these tests is therefore uncertain; these tests may be Misses or Correctly non-localising, therefore these tests are Unclassifiable.
-
E Partial concordance with the site of surgery means that the index test identified a focus that was either more or less extensive than the area excised. Given the good outcome following surgery, these tests could be deemed ‘Partial hits’; however, it is unclear whether such a good outcome would have been achieved if the area for excision was informed solely by the focus identified by the index test, therefore these tests are considered Unclassifiable.
-
F As with cell E, the index test identified a focus that was either more or less extensive than the area excised, these tests could be deemed ‘Partial errors’. However, not only is it unclear whether or not such a poor outcome would have been achieved if the area excised was informed solely by the index test focus, it could also be that the localisation was correct and intra- or postoperative complications or incomplete resection of the seizure focus resulted in the patients continuing to have seizures, therefore these tests are also Unclassifiable.
-
G As the index test was discordant with the actual site of surgery, the area of excision is based on the results of the comparator tests. Therefore, the good outcome following surgery indicates that the comparator tests identified the correct focus, and the index test result was an Error; these are the only index test results that can definitely be classified as errors.
-
H As with cell G, the site of surgery and the index test were discordant, and therefore the area of excision was based on the results of the comparator tests. Given the poor outcome following surgery, the comparator tests could either have identified an incorrect focus, or surgery could have been conducted at the correct location, but intra- or postoperative complications or incomplete resection of the seizure focus resulted in continued seizures. If it was assumed that the surgery was complete and complication free, it is still unknown whether or not the patient would have had a good outcome following surgery if the alternative site indicated by the index test had been excised. Therefore, these tests are Unclassifiable.
These descriptions highlight the complexity and limitations of the data retrieved from such studies, how interpretation of these data is difficult, and clinical utility limited. The only cells that provide unequivocal results are Cells C (Misses) and G (Errors) (the numbers of patients in Cell A who had a good outcome following inadvertent resection of the seizure focus by removing tissue adjacent to site identified by the tests were likely to be small, and we classified these as Hits in Table 6 in the results section (see Results, below). This section demonstrates that the accuracy of two or more tests when there is not complete concordance cannot be reliably evaluated using these data. For example, when patients had an index test that was discordant with the site of surgery and had a bad postoperative outcome (cells B, F and H), the poor outcome following surgery may not be because the surgery was conducted at the incorrect location but because of intra- or postoperative complications – such as gliosis, infection or bleeding – or there was incomplete resection of the seizure focus. Therefore, if a second operation is conducted at the alternative focus, the patient may undergo a second inappropriate and unnecessary surgical procedure. If the original surgery was conducted at the incorrect location, there is no way to know whether the alternate seizure focus identified by the index test is the true focus. If it was, and a second surgery was undertaken, there may be consequences of the first surgery, which may mean that reoperation would not give the good outcome it would have done if it was the original surgical procedure, therefore the index test may not appear to be accurate when in fact it was.
Results
Quantity and overall quality of research available
The searches identified 3251 citations; 534 were retrieved for full paper screening, of which 161 were abstracts. Figure 1 shows the flow of studies through the review. Eighteen studies met the inclusion criteria (n = 1312; range 24–469). 42,49,55–70 Nine studies49,55,56,58,59,62,66,68,70 evaluated PET, three49,57,61 evaluated SPECT, one67 evaluated SISCOM, two55,56 evaluated vMRI, three55,56,65 evaluated MRS, three42,63,69 evaluated MEG, two49,64 evaluated MSI, and one60 evaluated MRI/MRS. No studies met the inclusion criteria that evaluated HD-EEG, DTI or EEG-fMRI. Of the 18 studies, 13 were single-gate (cohort) diagnostic accuracy studies,42,49,55–58,61,63–66,68,69 seven reported outcome prediction using either a multivariate regression analysis or Bayesian classifiers,49,56,60,62,67,68,70 and one was a decision study. 59 Brief study details are provided in Table 4; full study details are provided in Appendix 3.
FIGURE 1.
Flow of studies through the review.
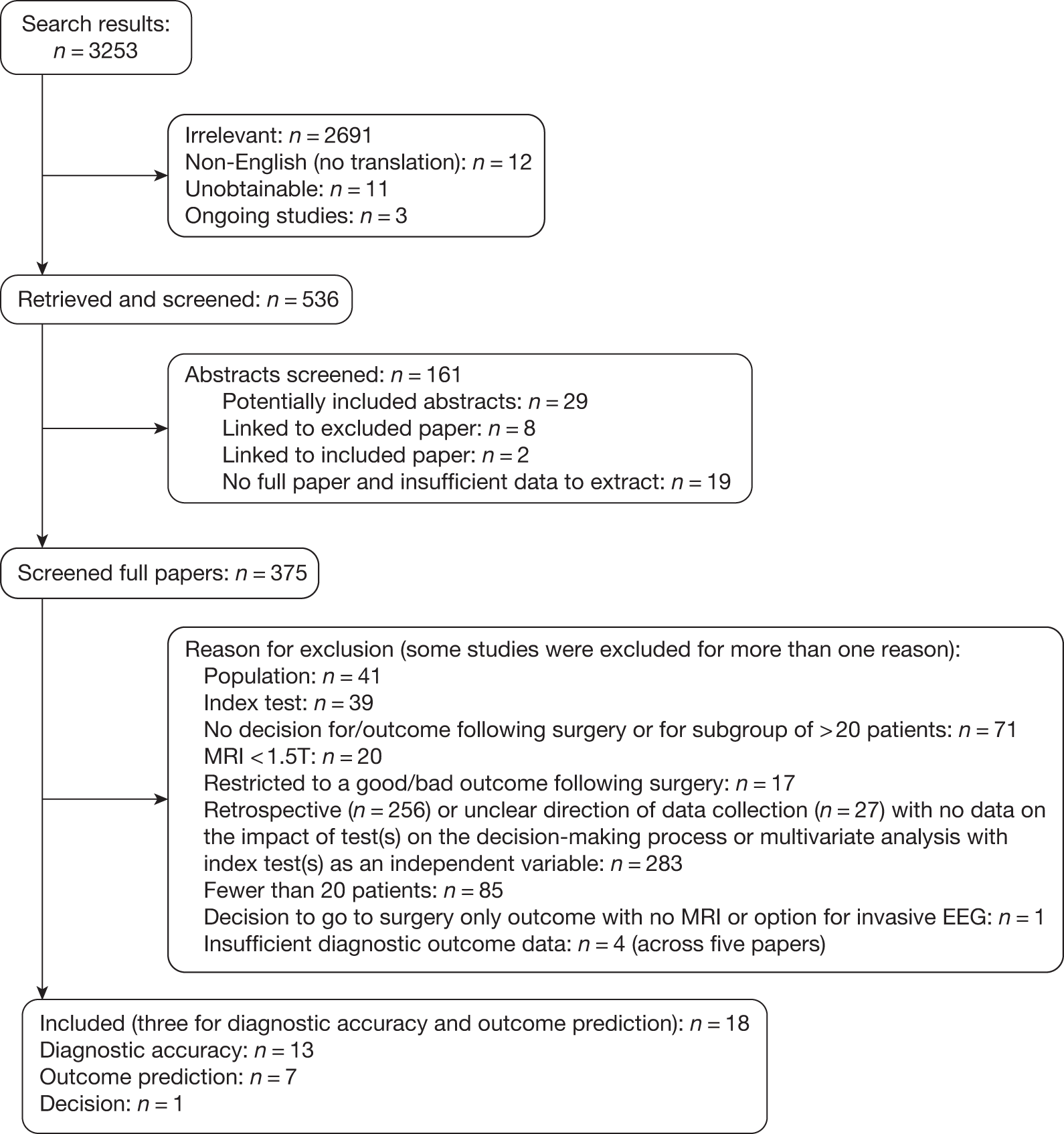
Study ID | Study size and population | Index tests | Comparator/analysis details |
---|---|---|---|
Diagnostic accuracy studies | |||
Achten (1997)55 |
n = 29 TLE |
MRS vMRI FDG-PET |
Decision to go to surgery Outcome following surgery |
Assaf (2004)63 |
n = 26 TLE |
MEG | Outcome following surgery |
Didelot (2008)58 |
n = 42 TLE |
FDG-PET |
Decision to go to surgery Outcome following surgery |
Kaiboriboon (2010)64 |
n = 25 TLE |
MSI | Outcome following surgery |
Knowlton (1997)56 |
n = 25 TLE |
FDG-PET MRS |
Outcome following surgery |
Knowlton (2008)49 |
n = 62 TLE/extra-TLE |
MSI HMPAO-SPECT FDG-PET |
Outcome following surgery |
Lee (2002)57 |
n = 24 TLE/extra-TLE |
HMPAO-SPECT |
Decision to go to surgery Outcome following surgery |
Lee (2005)65 |
n = 84 TLE/extra-TLE |
MRS | Outcome following surgery |
Lewis (1998)61 |
n = 35 Unclear |
HMPAO or ECD-SPECT |
Decision to go to surgery Outcome following surgery |
Smith (1995)69 |
n = 50 TLE/extra-TLE |
MEG | Outcome following surgery |
Theodore (1997)68 |
n = 46 TLE/extra-TLE |
FDG-PET |
Decision to go to surgery Outcome following surgery |
Wheless (1999)42 |
n = 58 TLE |
MEG | Outcome following surgery |
Wu (2010)66 |
n = 28 TLE/extra-TLE |
FDG-PET coregistered to MRI |
Decision to go to surgery Outcome following surgery |
Outcome prediction studies | |||
Antel (2002)60 |
n = 81 TLE |
MRI/MRS |
Analysis used: Bayesian classifiers used to predict outcome, based on conditional probability distributions Measure of association: Predictive values (%) Dependent variable(s): SF; > 90% reduction in seizures; not SF; no worthwhile improvement Other independent variable(s) in model: NR |
Dupont (2000)70 |
n = 30 TLE |
FGD-PET |
Analysis used: Discriminant analysis using as multiple dependent variables the regional metabolic asymmetries Dependent variable(s): Class A (SF) vs class C (rare DSs or worthwhile improvement) Other independent variable(s) in model: NR |
Knowlton (1997)56 |
n = 25 TLE |
vMRI FDG-PET MRS |
Analysis used: NR Measure of association: NR Dependent variable(s): SF Other independent variable(s) in model: NR |
Knowlton (2008)49 |
n = 62 TLE/extra-TLE |
MSI FDG-PET HMPAO-SPECT |
Analysis used: Logistic regression Measure of association: OR Dependent variable(s): Good outcome (Engel I only) Other independent variable(s) in model: Age; location of resection; routine MRI; video-EEG |
O’Brien (1999)67 |
n = 35 TLE/extra-TLE |
SPECT (hypoperfusion SISCOM and combined SISCOM) |
Analysis used: Logistic regression Measure of association: Beta coefficients Dependent variable(s): Excellent outcome: a seizure score of ≤ 4 (SF, with or without AEDs, or non-disabling nocturnal seizures only) Other independent variable(s) in model: Location of resection; routine MRI |
O’Brien (2001)62 |
n = 55 TLE/extra-TLE |
FDG-PET |
Analysis used: Linear regression Measure of association: Beta coefficients Dependent variable(s): Engel classes I–IV Other independent variable(s) in model: Routine MRI |
Theodore (1997)68 |
n = 46 TLE/extra-TLE |
FDG-PET |
Analysis used: Logistic regression Measure of association: NR Dependent variable(s): SF Other independent variable(s) in model: Constant; iEEG; routine MRI |
Decision study | |||
Uijl (2007)59 |
n = 469 TLE |
FDG-PET | Patients were classified as being eligible for surgery, not eligible for surgery, or eligibility was uncertain |
Overall, the quality of the available evidence was poor. Figure 2 shows the proportion of studies that met each of the quality criteria; full results of the quality assessment are given in Appendix 2. Of the 18 studies, 1242,49,55–59,63–66,68 described the inclusion criteria clearly but only two61,65 recruited a spectrum of patients that was representative of clinical practice, 1642,49,55–63. 65. 67–70 (89%) reported the execution of the index test, 1142,49,55,56,58,59,61,64,65,68,70 (58%) the comparator test(s)/surgical procedure, in sufficient detail to permit replication; seven studies55,57–59,62,67,68 (39%) interpreted the index test without knowledge of the comparator test(s)/site of surgery, five55,56,64,67,68 (26%) interpreted the comparator test(s)/decided the site of surgery without knowledge of the index test, and clinical data were available when test results were interpreted, as would be available in clinical practice in two studies59,66 (11%). Blinding of the interpreters of test results and other clinical data allows the independent assessment of the test results; however, it is worth noting that this does not reflect the work-up for surgery clinical practice, where decisions are made based on all the test results available. Only 10 studies42,55–59,61,66,69,70 reported uninterpretable results; given the nature of the index tests being evaluated, it is not uncommon to have uninterpretable results, and if these are omitted from data sets, accuracy could be overestimated. Twelve studies49,56,57,59,61,63–68,70 (68%) reported follow-up of at least 12 months in all patients. Of the seven outcome prediction studies, four60,62,68,70 (21%) reported dropouts to be similar across groups, one49 (5%) reported surgical outcome assessment being blinded to test result, it was unclear in any outcome prediction study whether the groups were comparable on all important confounding factors,71 and none adequately adjusted for its effects.
FIGURE 2.
Summary of the quality of the included studies. Q1: Was the spectrum of patients representative of the full range of patients who will receive the test in practice? Q2a: In the diagnostic accuracy and decision papers, were the selection criteria described clearly? Q2b: In the outcome prediction papers, were the prognostic factors described clearly and in full? Q3a: Was the execution of the index test described in sufficient detail to permit replication? Q3b: Was the execution of the reference test(s)/surgical procedure described in sufficient detail to permit replication? Q4a: Was the index test interpreted without knowledge of the comparator test(s)/site of surgery? Q4b: Was the comparator test(s) interpreted/site of surgery decided without knowledge of the index test? Q5: Were the same clinical data available when the test results were interpreted as would be available in clinical practice? Q6: Was outcome assessment blinded to exposure status? Q7: Was follow-up at least 12 months in all patients? Q8: Were uninterpretable/intermediate results reported? Q9: Were withdrawals from the study reported/explained? Q10: Were dropout rates and reasons for dropout similar across groups in the outcome prediction studies? Q11: Was the test reliably evaluated in the outcome prediction studies? Q12: Were the groups in the outcome prediction studies comparable on all important confounding factors? Q13: Was there adequate adjustment for the effects of all important confounding factors in the outcome prediction studies?
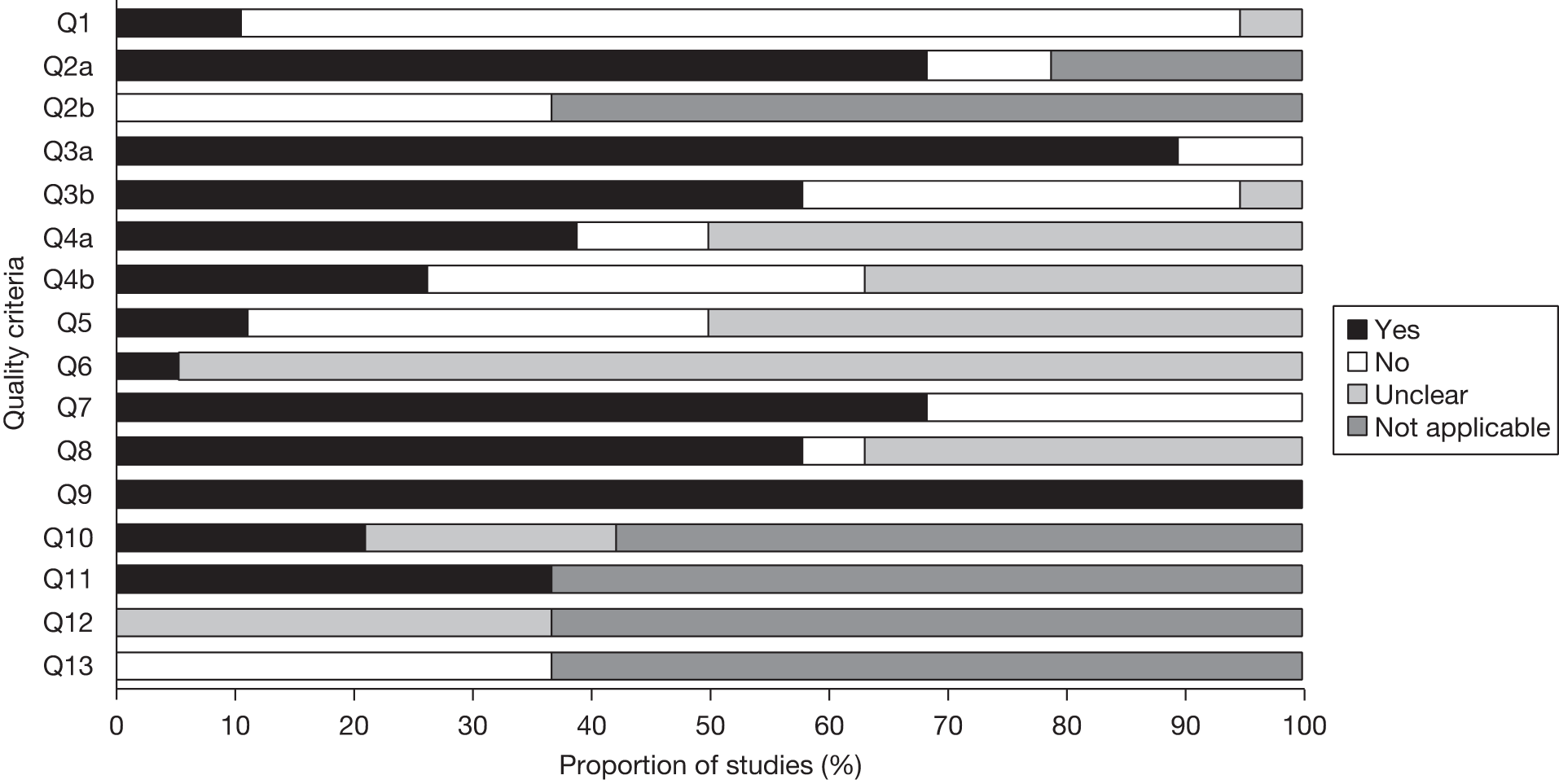
Assessment of clinical effectiveness
Critical review and synthesis of information
Diagnostic accuracy studies
Thirteen studies42,49,55–58,61,63–66,68,69 were single-gate diagnostic accuracy studies (n = 534; range 24–84); a table of study characteristics, and the results extracted from the studies, are given in Appendix 3.
Six studies55,57,58,61,66,68 reported the decision to go to surgery. As discussed in Chapter 3 (see Methodological limitations of the diagnostic accuracy studies), classification of the test results in order to determine the contribution of test results on the decision to undertake surgery was not possible. Table 5 gives the likelihood ratios for tests that were concordant, non-localising, partially concordant or discordant with the consensus diagnosis of the comparator tests. It is evident that the likelihood ratios are close to unity, and there is no consistent pattern of the ratios being higher in the concordant categories compared with the non-localising or discordant categories across studies, therefore these data are uninformative regarding how the decision in favour of surgery is altered by the index test. This is unsurprising, given the very small sample sizes and number of zero cells.
Study | Concordant | Non-localising | Partially concordant | Discordant |
---|---|---|---|---|
MRS: NAA/(Cho + Cr) | ||||
Achten (1997)55 | 0.81 | 0.17 | 3.50 | 0.17 |
FDG-PET | ||||
Achten (1997)55 | 1.93 | 0.90 | 1.17 | 0.07 |
Didelot (2008)58 | 1.18 | 0.50 | 0.83 | 0.50 |
Theodore (1997)68 | 3.30 | 0.35 | 1.29 | 0.12 |
FDG-PET coregistered to MRI | ||||
Wu (2010)66 | 0.29 | 0.71 | 7.86 | 3.67 |
HMPAO-SPECT | ||||
Lee (2002)57 | ||||
First SPECT | 0.29 | 3.07 | 0.72 | 1.20 |
Second SPECT | 0.72 | 3.60 | 0.63 | 1.47 |
First and second SPECT | 0.47 | 3.60 | 1.47 | 2.80 |
HMPAO or ECD-SPECT | ||||
Lewis (1998)61 | 1.11 | 0.52 | 1.11 | 0.52 |
vMRI | ||||
Achten (1997)55 | 2.17 | 0.17 | 0.21 | 0.17 |
All 13 studies42,49,55–58,61,63–66,68,69 used outcome following surgery as a reference standard; only 1242,49,55–58,61,63–65,68,69 provided sufficient data to allow an attempt to be made to calculated the overall rates of ‘Hits’, ‘Misses’ and ‘Errors’ (Table 6), as determined using the definitions given in Methodological limitation of the diagnostic accuracy studies, assuming that the surgical procedure was uncomplicated and that a poor outcome following surgery was due to an error in the localisation of the focus; the difficulties categorising test results in this way are discussed in detail in that chapter. From Table 6, it can be seen that the number of index tests that were correctly localising as indicated by a good surgical outcome ranged from 6% to 96%, depending on the index test and the definition used to define a good surgical outcome. PET correctly identified between 51% and 85% seizure foci, with the proportion increasing as the definition of a good outcome becomes more lax. MEG showed a similar range of correctly localising tests, from 44% to 96%. Ictal HMPAO-SPECT appears to have a particularly low number of hits; however, this was evaluated in a population in which localisation was still uncertain after MRI, EEG and a first SPECT. Thus, epilepsy was particularly difficult to localise in these patients and these findings may not represent the accuracy of SPECT when used in wider population of patients with refractory epilepsy. 57
Study | Definition of a good outcome | Nos. of patients with tests that are: | Proportion of tests that are: | |||||||
---|---|---|---|---|---|---|---|---|---|---|
Hits | Errorsa | Misses | Unclassified | Hits | Errors | Misses | Unclassified | |||
FDG-PET | ||||||||||
Achten (1997)55 | Engel Ia | 12 | 2 | 2 | 1 | 70.6 | 11.8 | 11.8 | 5.9 | |
Engel I and II | 12 | 2 | 2 | 1 | 70.6 | 11.8 | 11.8 | 5.9 | ||
Didelot (2008)58 | Engel Ia | 17 | 5 | 1 | 3 | 65.4 | 19.2 | 3.8 | 11.5 | |
Engel I | 21 | 1 | 1 | 3 | 80.8 | 3.9 | 3.9 | 11.5 | ||
Engel I and II | 22 | 0 | 2 | 2 | 84.6 | 0 | 7.7 | 7.7 | ||
Theodore (1997)68 | SF (Engel Ia) | 18 | 5 | 4 | 8 | 51.4 | 14.3 | 11.4 | 22.9 | |
Knowlton (1997)56 | Engel I | 15 | 4 | 1 | 2 | 68.2 | 18.2 | 4.5 | 9.1 | |
Engel I and II | 18 | 1 | 1 | 2 | 81.8 | 4.5 | 4.5 | 9.1 | ||
Ictal HMPAO-SPECT | ||||||||||
Lee (2002)57 |
Engel I and II First SPECT |
1 | 3 | 7 | 6 | 5.9 | 17.6 | 41.2 | 35.3 | |
Engel I and II Second SPECT |
2 | 6 | 2 | 7 | 11.8 | 35.3 | 11.8 | 41.2 | ||
Engel I and II First and second SPECT combined |
2 | 5 | 2 | 8 | 11.8 | 29.4 | 11.8 | 47.1 | ||
Interictal HMPAO or ECD-SPECT | ||||||||||
Lewis (1998)61 | SF (Engel Ia) | 4 | 5 | 0 | 8 | 23.5 | 29.4 | 0 | 47.1 | |
SF/significant or worthwhile improvement (Engel I–III) | 7 | 3 | 0 | 7 | 81.8 | 17.6 | 0 | 41.2 | ||
MEG | ||||||||||
Assaf (2004)63 | SF (Engel Ia) | 21 | 1 | 0 | 0 | 95.5 | 4.5 | 0 | 0 | |
Smith (1995)69 | SF (Engel Ia) | 14 | 5 | 4 | 9 | 43.8 | 15.6 | 12.5 | 28.1 | |
SF or rare seizures (Engel I and II) | 17 | 2 | 5 | 7 | 54.8 | 6.5 | 16.1 | 22.6 | ||
Over 90% reduction in seizures | 19 | 0 | 5 | 8 | 59.4 | 0 | 15.6 | 25.0 | ||
Wheless (1999)42 | SF or rare seizures (Engel I and II) | Overall | 23 | 5 | 6 | 10 | 52.3 | 11.4 | 13.6 | 22.7 |
Patients with TLE | 16 | 2 | 4 | 6 | 57.1 | 7.1 | 14.3 | 21.4 | ||
Patients with extra TLE | 7 | 3 | 2 | 4 | 43.8 | 18.8 | 12.5 | 25.0 | ||
MRS: NAA/(Cho + Cr) | ||||||||||
Achten (1997)55 | Engel Ia | 7 | 1 | 0 | 9 | 41.2 | 5.9 | 0 | 52.9 | |
Engel I and II | 7 | 1 | 0 | 9 | 41.2 | 5.9 | 0 | 52.9 | ||
Knowlton (1997)56 | Engel I | 10 | 2 | 5 | 5 | 45.5 | 9.1 | 22.7 | 22.7 | |
Engel I and II | 10 | 2 | 8 | 2 | 45.5 | 9.1 | 36.4 | 9.1 | ||
MRS: NAA/Cho | ||||||||||
Lee (2005)65 | SF (Engel Ia) | 13 | 1 | NR | 11 | 52.0 | 4.0 | NR | 44.0 | |
MSI | ||||||||||
Kaiboriboon (2010)64 | Engel Ia | 8 | 7 | 3 | 4 | 36.4 | 31.8 | 13.6 | 18.2 | |
Engel I | 9 | 6 | 3 | 4 | 40.9 | 27.3 | 13.6 | 18.2 | ||
Engel I and II | 13 | 2 | 3 | 4 | 59.1 | 9.1 | 13.6 | 18.2 | ||
vMRI | ||||||||||
Achten (1997)55 | Engel Ia | 14 | 2 | 0 | 1 | 82.4 | 11.8 | 0 | 5.9 |
Despite the use of the assumption that all surgery was uncomplicated, it is notable that the proportion of tests that could not be classified as a hit, miss or error was high – up to 47%. This highlights the serious limitation of these data. A potentially large proportion of tests cannot be used to inform the clinical question given the way they are currently recorded and reported. Given the high proportion of unclassifiable tests, it is clear that conclusions based only on those tests that could be classified would not be reliable enough to inform clinical practice. The numbers of unclassifiable tests is sufficiently high that the conclusions of the accuracy of the tests being evaluated would be significantly different if all tests were truly hits or errors.
Two diagnostic accuracy studies42,49 reported estimates of diagnostic accuracy outcomes. One study42 evaluating MEG combined the partial hits, misses and non-localising categories and calculated a sensitivity of 52% in the entire heterogeneous population, 57% for patients with TLE and 44% for patients with non-TLE. The other study evaluated MSI, PET and SPECT, apparently combined non-localising and indeterminate tests and reported sensitivity, specificity and predictive values with 95% CIs for each test individually and combinations of the three tests; full results are reported in Appendix 3. 49 Sensitivity was 55% (95% CI 44.2% to 63.7%) and specificity 75% (95% CI 57.4% to 88.4%) for MSI. Sensitivity was 59% (95% CI 47.4% to 67.4%) and specificity 79% (95% CI 58.7% to 92.5%) for FDG-PET. Sensitivity was 50% (95% CI 29.9% to 67.0%) and specificity 72% (95% CI 54.4% to 87.3%) for HMPAO-SPECT.
Table 7 gives the likelihood ratios for the concordant, non-localising, partially concordant and discordant test categories. As with the decision to undertake surgery, the likelihood ratios are close to unity and inconsistent across studies, and no conclusions can be drawn from these data.
Study | Definition of a good outcome | Concordant | Non-localising | Partially concordant | Discordant | |
---|---|---|---|---|---|---|
FDG-PET | ||||||
Achten (1997)55 | Engel Ia | 1.18 | 1.18 | 0.71 | 0.24 | |
Didelot (2008)58 | Engel Ia | 1.36 | 0.43 | 0.43 | 0.43 | |
Theodore (1997)68 | SF (Engel Ia) | 1.49 | 0.57 | 0.62 | 0.44 | |
Knowlton (1997)56 | Engel I | 1.53 | 0.27 | 0.44 | 0.44 | |
Didelot (2008)58 | Engel I | 2.21 | 0.15 | 0.77 | 0.15 | |
Didelot (2008)58 | Engel I and II | 3.21 | 0.36 | 0.36 | 0.07 | |
Knowlton (1997)56 | Engel I and II | 2.94 | 0.14 | 0.24 | 0.24 | |
Achten (1997)55 | Engel I and II | 1.18 | 1.18 | 0.71 | 0.24 | |
HMPAO-SPECT | ||||||
Lee (2002)57 | Engel I | First ictal | 0.45 | 1.25 | 0.75 | 2.25 |
Second ictal | 0.75 | 0.75 | 1.25 | 1.35 | ||
First and second ictal | 0.83 | 0.83 | 0.65 | 5.83 | ||
HMPAO or ECD-SPECT | ||||||
Lewis (1998)61 | SF (Engel Ia) | 0.70 | 1.10 | 1.73 | 0.37 | |
SF/significant or worthwhile improvement (Engel I–III) | 0.81 | 0.31 | 1.56 | 0.94 | ||
MEG | ||||||
Assaf (2004)63 | SF (Engel Ia) | 1.87 | 0.13 | 0.13 | 0.13 | |
Smith (1995)69 | SF (Engel Ia) | 1.32 | 1.50 | 0.30 | 0.70 | |
Smith (1995)69 | SF or rare seizures (Engel I and II) | 1.45 | 2.28 | 0.12 | 1.86 | |
Smith (1995)69 | Over 90% reduction in seizures | 6.29 | 1.77 | 0.10 | 0.48 | |
MRS: NAA/(Cho + Cr) | ||||||
Achten (1997)55 | Engel Ia | 1.87 | 0.13 | 0.13 | 0.13 | |
Knowlton (1997)56 | Engel I | 1.87 | 0.54 | 0.44 | 1.33 | |
Achten (1997)55 | Engel I and II | 1.18 | 0.24 | 1.33 | 0.24 | |
Knowlton (1997)56 | Engel I and II | 1.00 | 1.35 | 0.24 | 0.71 | |
MRS: NAA/Cho | ||||||
Lee (2005)65 | SF (Engel Ia) | 3.29 | NR | 0.43 | 0.37 | |
MSI | ||||||
Kaiboriboon (2010)64 | Engel Ia | 0.82 | 3.71 | 0.53 | 2.65 | |
Engel I | 0.78 | 2.58 | 0.86 | 1.84 | ||
Engel I and II | 3.52 | 0.91 | 0.30 | 0.65 | ||
vMRI | ||||||
Achten (1997)55 | Engel Ia (SF) | 1.36 | 0.24 | 0.71 | 0.24 |
All the studies reporting outcome following surgery were small in size, with event rates in the partially concordant and discordant categories being very low or zero, and none of the tests were investigated in a sufficient number of studies to allow firm conclusions to be drawn. In addition, there was substantial heterogeneity across studies precluding any average proportions to be determined, and the generalisability of the results of individual studies is limited.
Outcome prediction studies
Six included studies that reported the results of a multivariate regression in which at least one index test of interest was an independent variable met the inclusion criteria (n = 228; range 30–62),49,56,62,67,68,70 and a further study reported the results of an analysis using Bayesian classifiers (n = 81). 60 A table of study characteristics is given in Appendix 3. Four diagnostic accuracy studies provided sufficient IPD for binary logistic regression to be conducted. 57,58,61,64 These analyses were restricted to the variables reported in the studies. In addition, the studies were small, and inclusion of all the variables reported in some of the studies would have been appropriate; thus, age and/or gender were omitted if patient numbers were insufficient to allow the inclusion of all variables reported.
The results of the multivariate regression studies are given in Table 8, and the binary logistic regressions conducted on the IPD reported in the diagnostic accuracy studies in Table 9. The studies were very heterogeneous in terms of the index tests evaluated, the method of analysis, the definition of the dependent variable and the covariates included in the model. In addition, there is a range of limitations of these studies that makes the reliability of the results questionable. First, none of the studies adjusted for a range of important confounding variables (febrile seizures, MTS, EEG/MRI concordance and extent of surgical resection71). Second, all of the studies were small, with the number of patients ranging from 24 to 81; the total number of patients in the 11 studies was 460. These factors preclude any conclusions being drawn from these studies regarding the predictive ability of the tests evaluated.
Study | Analysis and sample size | Test evaluated | Measure (95% CI) | Variable p-value | R 2 | Model p-value | Other variables in model | |
---|---|---|---|---|---|---|---|---|
Knowlton (2008)49 |
Analysis: Logistic regression Measure of association: Adjusted OR (MSI in patients with MSI and SPECT is unadjusted) Dependent variable: Engel I n = 62 |
MSI in patients with MSI only | 4.4 (1.36 to 14.45) | 0.01 | NR | NR |
Age Location of resection Routine MRI Video-EEG |
|
MSI in patients with MSI and PET | 6.4 (1.49 to 27.28) | 0.01 | ||||||
MSI in patients with MSI and SPECT | 1.6 (0.37 to 6.62) | 0.55 | ||||||
MSI in patients with MSI, PET and SPECT | 5.6 (0.53 to 58.21) | 0.15 | ||||||
PET in patients with MSI and PET | 7.1 (1.68 to 30.21) | < 0.01 | ||||||
PET in patients with MSI, PET and SPECT | 4.9 (0.47 to 51.14) | 0.18 | ||||||
SPECT in patients with MSI and SPECT | 2.6 (0.54 to 12.13) | 0.23 | ||||||
SPECT in patients with MSI, PET and SPECT | 9.1 (1.0 to 82.16) | 0.05 | ||||||
Antel (2002)60 |
Analysis: Bayesian classifiers predicting outcome on conditional probability distributions Measure of association: Predictive values (%) n = 81 |
Dependent variable: SF | MRI/MRS | 75 (64 to 86) | N/A | N/A | N/A | A range of variables associated with the index test only |
Dependent variable: not SF | MRI/MRS | 72 (56 to 88) | ||||||
SF/not SF: The following combination of variables had highest classification accuracy for predicting SF following surgery: NAA/Cr in mid-temporal region ipsilateral to site of surgery (MRS), NAA/Cr in contralateral posterior temporal region (MRSI), asymmetry of NAA/Cr in contralateral posterior temporal region (MRSI), and hippocampal asymmetry (vMRI). With this combination of features, 39/52 patients who were SF, and 21/29 who were not SF, were correctly classified | ||||||||
Dependent variable: Engel I–III | MRI/MRS | 92 (89.3 to 95.3) | N/A | N/A | N/A | A range of variables associated with the index test only | ||
Dependent variable: Engel IV | MRI/MRS | 63 (50.4 to 74.6) | ||||||
Worthwhile improvement (> 90% reduction)/no worthwhile improvement: The following combination of variables had highest classification accuracy for predicting worthwhile improvement: NAA/Cr in mid-temporal region ipsilateral to site of surgery (MRS), asymmetry of NAA/Cr in mid-temporal region ipsilateral to site of surgery (MRS), ipsilateral hippocampal volume (vMRI), and hippocampal asymmetry (vMRI). With this combination of features, 60/65 patients who had worthwhile improvement, and 10/16 with no worthwhile improvement, were correctly classified | ||||||||
O’Brien (2001)62 |
Analysis: Linear regression Measure of association: Beta coefficient n = 55 |
Dependent variable: Prediction of Engel class |
PET Localising vs non-localising |
0.51 (SE 0.17) | 0.007 | 0.40 | 0.004 | Routine MRI |
Multiple regression analysis showed the FDG-PET results to be predictive of post-surgical outcome independent of the MRI findings. PET images provided prognostically significant localisation information incremental to that provided by vMRI and ictal EEG, particularly when one of these studies was non-localising | ||||||||
O’Brien (1999)67 |
Analysis: Logistic regression Measure of association: Beta coefficients n = 35 |
Dependent variable: SF with or without AEDs, or non-disabling nocturnal seizures | SPECT (hypo-perfusion SISCOM) | 2.39 (SE 1.25) | 0.07 | NR | NR |
Location of resection Routine MRI |
SPECT (combined SISCOM) | 2.89 (SE 1.33) | 0.042 | NR | NR | ||||
Theodore (1997)68 |
Analysis: Logistic regression Measure of association: NR n = 46 |
Dependent variable: Engel Ia | PET | Inferior lateral temporal and inferior mesial temporal AI on PET were significant predictors of outcome in group as a whole (p < 0.001) and for those who underwent surgery (p < 0.05) |
Routine MRI iEEG Constant |
|||
Dupont (2000)70 |
Analysis: Discriminant analysis using regional metabolic asymmetries as multiple dependent variables n = 30 |
Dependent variable: SF vs rare/DSs or worthwhile improvement | PET | 7.21 (NR) | 0.02 | NR | NR | NR |
The equation combining the metabolism of the temporal pole, the basofrontal cortex, the anterior part of the lateral temporal neocortex, and the medial temporal cortex was highly significant [F(4, 15) = 7.21, p = 0.02]. The multivariate equation correctly classified 14/14 category A patients and 6/6 category C patients. The mean normalised z-value for category A patients was 1.43 (SD = 1.10) and was 1.43 (SD = 0.65) for category C patients. This equation was tested in the 10 patients in category B and the equation generated individual normalised z-scores ranging from 1.99 to –1.89. The difference between the groups was highly significant (F = 15.4, p < 0.001) | ||||||||
Knowlton (1997)56 |
Analysis: Unclear Measure of association: NR n = 25 |
Dependent variable: Engel Ia | vMRI | NR | < 0.001 | NR | NR | NR |
PET | NR | 0.04 | NR | NR | ||||
MRS | Lateralised MRS not correlated with surgical outcome |
Study and sample size | Index test | Dependent variable | OR (95% CI) | p-value | Other variable in model |
---|---|---|---|---|---|
Kaiboriboon (2010)6 (n = 25) | MSI | Engel Ia | 0.67 (0.27 to 1.67) | 0.392 | Age |
Engel I | 0.58 (0.18 to 1.83) | 0.352 | Routine MRI | ||
Constant | |||||
Didelot (2008)58 (n = 42) | PET | Engel Ia | 2.40 (0.58 to 9.89) | 0.224 |
Age Duration of epilepsy SEEG Routine MRI Constant |
Lewis (1998)61(n = 35) | SPECT | SF (Engel Ia) | 0.29 (0.03 to 2.74) | 0.282 |
Ictal EEG Interictal EEG Routine MRI Constant |
Lee (2002)57 (n = 24) | First SPECT | Engel I | 0.80 (0.31 to 2.09) | 0.647 | Duration of epilepsy |
Second SPECT | 0.86 (0.28 to 2.70) | 0.798 | Site of surgery | ||
First and second SPECT | 1.03 (0.31 to 3.36) | 0.967 |
Pathology Constant |
||
Achten (1997)55 | Only two patients were not SF after surgery, therefore there were insufficient data to conduct a logistic regression | ||||
Wu (2010)66 | The IPD did not contain outcome following surgery |
Decision studies
A single-decision study [defined as a prospective or retrospective study, with at least 30 patients, which reported the impact of an index test(s) of interest on the decision to go to surgery and the outcome following surgery] met the inclusion criteria for the review. This study specifically assessed the impact of one of our index tests of interest – interictal FDG-PET – on the decision-making process. 59 This was a retrospective study of all patients referred to the Dutch Collaborative Epilepsy Surgery Program (DCESP) in the Netherlands between 1996 and 2002. Patients referred to this programme undergo a standard work-up including taking a clinical history, routine EEG and MRI (using at least a 1.5-T magnet and FLAIR), and prolonged surface video-EEG.
A multidisciplinary team (neurosurgeon, neurologist, neurophysiologist, neuropsychologist and radiologist) made a consensus decision, and decided whether or not further tests such as PET, SPECT, fMRI, MEG or iEEG were required; vMRI was not an option. It is the documentation of these meetings that was used as the basis for the study, with a comparison of the decision made by the team before and after the FDG-PET scan used to determine whether FDG-PET altered the original decision based on the clinical history, routine EEG and MRI, and video-EEG.
Magnetic resonance imaging results were categorised as no abnormalities, unilateral abnormalities, bilateral abnormalities or other abnormalities. EEG results were classified as showing or not showing unilateral temporal lobe abnormalities (interictal) or onset (ictal). Results of the FDG-PET were classified as normal (not lateralising), unilateral temporal hypometabolism (lateralising, including scans where confined ipsilateral frontobasal, ipsilateral thalamic, or contralateral cerebellar hypometabolism were present) or other (usually bilateral or extratemporal hypometabolism).
To determine the added value of FDG-PET, 2 × 2 tables of test performance were constructed with the results of the MRI/EEG being positive or negative, and the decision to go to surgery as the reference standard. Further 2 × 2 tables were then constructed, with the PET results being combined with those of the MRI/EEG. Positive (and negative) predictive values were calculated for the decision to go to surgery (or not); these give the proportion of patients accurately predicted to be eligible (or not eligible) for surgery. A sensitivity analysis was conducted to investigate the impact of work-up bias, by including patients evaluated prior to 1996 who would have received MRI with a magnet strength of < 1.5 T and without FLAIR. However, this technology is now standard in the UK, hence inclusion to the review being restricted to studies using MRI technologies with a tesla strength of at least 1.5, and results for patients prior to 1996 would not be representative of those being evaluated in current UK clinical practice.
Of the 110 patients who received FDG-PET, the decision for or against surgery was considered to be influenced by the results of the FDG-PET scan in 78 patients (71%): 48 influenced the decision in favour of surgery; 28 in favour of no surgery; and two patients had doubt cast on prior decisions and eligibility for surgery became uncertain (Table 10). The positive decision predictive value for PET was 65% (95% CI 53% to 77%) and negative decision predictive value was 60% (95% CI 45% to 72%); predictive values for all tests conducted are given in Table 11.
PET altered the surgical decision from: | ||||||||
---|---|---|---|---|---|---|---|---|
Surgery to unsure | No surgery to surgery | Unsure to surgery | No surgery to unsure | Surgery to no surgery | Unsure to no surgery | |||
1a | 1 | 47 | 1b | 3 | 25 | |||
SF | Not SF | SF | Not SF | SF | Not SF | |||
1 | 0 | 0 | 1 | 30 | 17 | |||
PET did not alter the surgical decision: | ||||||||
Surgery | Unsure | No surgery | ||||||
12 | 12c | 8 | ||||||
SF | Not SF | SF | Not SF | |||||
7 | 5 | 5 | 5 |
Test | Positive decision predictive value (%) (95% CI) | Negative decision predictive value (%) (95% CI) |
---|---|---|
FDG-PET | 65 (53 to 77) | 60 (45 to 72) |
MRI | 67 (54 to 78) | 57 (44 to 69) |
Interictal video-EEG | 59 (43 to 74) | 48 (37 to 59) |
Video-EEG seminology | 65 (51 to 77) | 54 (42 to 66) |
Ictal video-EEG | 63 (50 to 74) | 56 (42 to 69) |
When comparing the surgical outcome of those who underwent FDG-PET as part of their presurgical work-up with that of those who did not, the proportion of patients who were SF without auras (Engel Ia) at 1 year post operation was 60% and 66%, respectively. When compared with the entire population who had undergone surgery, those receiving FDG-PET had slightly (but not statistically significantly) lower rates of Engel Ia at 1 year (overall 64%, FDG-PET 60%) and final (overall 51%, FDG-PET 48%) follow-up.
This was a well-conducted retrospective review of the practices of a multidisciplinary epilepsy team, and the decision-making process during the work-up for epilepsy surgery. This study has distinct advantages over the single-gate diagnostic accuracy studies identified for this review, with the decision regarding surgery before and after the index test of interest being reported. There were some limitations to the study, such as the potential technological advancements since the assessment of the participants in the study from 1996 to 2002, limiting its generalisability to current practice; the combining of inconclusive and normal FDG-PET results in order to dichotomise the data to produce predictive values, as these may represent different populations (included patients with bilateral temporal foci, non-localising tests and indeterminate/uninterpretable results); the number of patients who did not undergo FDG-PET (359/469 patients) and therefore the potential for selection bias; the outcome following surgery being reported as the proportion of patients classified as Engel Ia (SF without auras), and although this is the ultimate goal of surgery, Engel I and II are more commonly considered to be good post-surgical outcomes; and the conduct of additional tests after FDG-PET in some patients prior to surgery (figure 1, p. 2124, of the published paper59), which could have influenced the decisions made by the multidisciplinary team, and hence the decision to go to surgery or the outcome following surgery. From this study, it could be concluded that FDG-PET may be useful in patients with temporal lobe epilepsy where there is discordance between the EEG and MRI.
There were some limitations of this study, such as the potential technological advancements, as the assessment of the participants in the study from 1996 to 2002 limited its generalisability to current practice; the large number of patients who did not undergo FDG-PET (359/469 patients) and therefore the potential for selection bias; the conduct of additional tests after FDG-PET in some patients prior to surgery that could have influenced the decision to go to surgery or the site of surgery; and only the outcome following surgery being reported for those achieving Engel Ia (SF without auras). 59
Discussion
This review aimed to evaluate the evidence available for the use of non-invasive technologies to identify the seizure focus in patients with refractory epilepsy being considered for surgery, and their impact on the decision-making process and subsequent clinical outcomes.
Key findings
The main finding of the review of clinical effectiveness is that the available evidence is inadequate, both in terms of methodological design and population sizes, to reliably inform clinical practice. Importantly, there is a distinct lack of studies evaluating the clinical utility of diagnostic tests, and therefore the impact of these tests on clinical decision-making and patient outcomes; data from the diagnostic accuracy studies are not sufficient to address these issues. One study met the inclusion criteria that reported the impact of one of the index tests of interest (FDG-PET) on the decision to go to surgery, and the outcome following surgery in those who underwent an excisional procedure. 59
Strengths and limitations of the review
The systematic review was based on an extensive search. Abstracts were included when there were sufficient data to be extracted; authors were not contacted. Foreign-language studies were included only if an English-language translation was available. Therefore, although publication and language bias are likely to be present, this was the pragmatic choice, given the nature of the evidence base. The inability to inform clinical practice was not due only to the lack of volume of evidence, but also to the type of evidence currently available; if the number of single-gate diagnostic accuracy studies were doubled, firm conclusions still could not have been drawn. In addition, we screened 161 studies that were retrieved as abstracts; none of these described studies that were relevant to the research question or that were different in design to those that were retrieved as full papers. Therefore, as the focus of the review was the limitations of the currently available studies rather than an assessment of the relative diagnostic accuracy, we feel that the lack of unpublished and foreign-language data does not compromise the conclusions of the review.
Studies were selected using inclusion criteria defined a priori. These criteria were extended in terms of study design to encompass all available evidence relevant to the research question. A further modification was made to the inclusion criteria concerning the requirement of iEEG in studies only reporting the decision to go to surgery. Originally, iEEG was a requirement; however, this was changed so that routine MRI was required (either as a component of the comparator tests or as an index test), and with iEEG being available as an option, as this reflects clinical practice more closely.
Limitations of the available evidence
There were a number of limitations of the available evidence, and overall, the quality of the available evidence was poor. Four studies were identified that evaluated the use of one of the index tests of interest on the decision-making process, of which three were decision studies59,72,73 and one was a RCT;74 unfortunately, only one of the decision studies passed the inclusion criteria for the review. 59 The specific limitations of the data from the diagnostic accuracy studies were discussed in detail in Chapter 3 (see Methodological limitations of the diagnostic accuracy studies). Further limitations were the generally small study sizes, patient selection bias, substantial clinical heterogeneity across the studies, terms of the combination of tests to determine the seizure focus, the technology used during the index tests and the populations recruited, even across those that evaluated the same index test, precluding the pooling of studies.
Comparison with previous systematic reviews
Some of the diagnostic technologies evaluated in this review have been evaluated in previous systematic reviews. 36,38,75 One was the prior HTA report36 which addressed a broad-ranging question evaluating any neuroimaging technology compared with any reference standard. The review included 75 diagnostic accuracy studies evaluating SPECT, PET, MRI, CT, SISCOM, MRS or NIRS, and nine outcome prediction studies evaluating SPECT, PET, MRI, SISCOM or MRS in univariate and/or multivariate regression analyses. This was a well-conducted, thorough review of the published and unpublished evidence available to 2003. Given the conclusions of that review, that MRI seemed to be the most consistent predictor of surgical outcome, probably due to MRI being effective in the localisation of lesions such as tumours and vascular malformations, and that the evidence available at the time could not reliably inform clinical practice, the present work did not attempt to update the previous review. Instead, this review concentrated on a focused but valuable clinical question that constituted a small section of the prior report, attempting to concentrate on the added value of non-invasive technologies over and above routine MRI and EEG, and restricting the inclusion of studies based on the reference standard used, and omitting routine MRI, CT and NIRS from the review. In addition, this review expanded in terms of the exploration of the data from diagnostic accuracy studies by maintaining the four different populations as categorised by test concordance rather than collapsing the data into a 2 × 2 table of test performance, by searching specifically for studies that reported the impact of the index tests of interest on the decision-making process, and including non-neuroimaging on-invasive technologies, HD-EEG and MEG, as index tests.
Two further systematic reviews are worth noting. 38,75 Uijl et al. 38 evaluated a range of technologies used to lateralise or localise the seizure focus in patients with TLE and used the consensus diagnosis or the decision to perform surgery as the reference standard. 38 Lau et al. 75 reviewed the use of MEG in a heterogeneous epilepsy population. Data in both of these reviews were dichotomised in order to produce 2 × 2 tables of test performance from which standard diagnostic outcomes were calculated. The method used to dichotomise the data was not reported in the review by Uijl et al. ;59 however, the review was conducted by the same authors as the decision study included in our review, and that study combined inconclusive and normal results, which included patients with bilateral temporal foci, non-localising tests and indeterminate/uninterpretable results. The review by Lau et al. 75 dichotomised data into concordant and discordant tests; it is unclear how non-localising tests were dealt with. As with the prior HTA report, both reviews concluded that there was insufficient evidence to inform clinical practice; Uijl et al. 59 recommended further studies evaluating the added value of consecutive tests on the decision-making process, and Lau et al. 75 recommended more controlled and consistent studies to determine whether or not MEG could replace iEEG.
Ours is the first systematic review of clinical evidence to attempt to determine the added value of a wide range of non-invasive technologies in a heterogeneous epilepsy population, without dichotomising the data and therefore avoiding combining distinct populations for whom the index test results could lead to different management strategies and long-term consequences for the patients.
Summary
It is clear from this and previous reviews that the currently available evidence is not sufficient to evaluate the clinical utility of diagnostic tests for the presurgical work-up of patents with refractory epilepsy. The use of data from diagnostic accuracy studies has serious limitations, and alternative methods of data collection, which assess the impact of the diagnostic tests of interest on the decision-making process and patient outcomes, are required. The options available are discussed in detail in Chapter 5.
Chapter 4 Assessment of cost-effectiveness evidence
Systematic review of existing cost-effectiveness evidence
A systematic literature search was undertaken to identify existing evidence on the cost-effectiveness of alternative technologies used to visualise seizure focus in people with refractory epilepsy being considered for surgery. The specific aims of the reviews were to:
-
critically appraise the existing evidence on cost-effectiveness and its relevance to the specific decision problem under investigation and to informing decision-making in the NHS
-
identify important structural assumptions, data sources for parameter inputs and to highlight key areas of uncertainty and potential data gaps
-
identify key parameter inputs requiring additional focused searching and literature reviews
-
inform the development and population of our own decision model, relevant to the UK NHS.
Methods of systematic review of existing cost-effectiveness evidence
The search strategy for the project is reported in Chapter 3 (see Search strategy). In addition to the databases listed in that section, the NHS Economic Evaluations Database (NHS-EED) was searched for economic evaluations (via Wiley, The Cochrane Library website, issue 7 of 12 July 2010). A broad range of studies were considered in the assessment of cost-effectiveness including economic evaluations conducted alongside trials, modelling studies and analyses of administrative databases. Only full economic evaluations that compare two or more options and consider both costs and consequences (including cost-effectiveness, cost–utility and cost–benefit analyses) were included in the review of economic literature.
Two reviewers independently assessed all obtained titles and abstracts for inclusion. Any discrepancies were resolved by discussion. The quality of the cost-effectiveness studies was assessed according to a checklist based on that developed by Drummond et al. 76 This checklist reflects the criteria for economic evaluation detailed in the methodological guidance developed by the National Institute for Health and Clinical Excellence (NICE)77 and is reported in Appendix 5.
The systematic literature search identified only one study that met the criteria for inclusion in the cost-effectiveness review. 78 The following section provides an overview of this paper and assesses its relevance to the scope of this technology assessment.
Review of O’Brien et al.78
This study by O’Brien et al. 78 evaluates the cost-effectiveness of a range of presurgical strategies for the lateralisation of temporal lobe epilepsy in patients; a summary of the paper is provided in Table 12. The costs of the strategies are evaluated over the lifetime of the patient from the perspective of the Australian health-care sector. It is not clear if the benefits of the strategies are also considered over the patient’s lifetime. There is no discussion of whether costs or benefits are discounted. The author used a deterministic decision tree to model the different strategies; there is no discussion of any formal long-term modelling.
Authors | O’Brien et al.78 |
Year of publication | 2008 |
Type of economic evaluation | Cost-effectiveness analysis |
Country of setting | Australia |
Currency used | Unclear if Australian dollar or US dollar |
Year to which costs apply | Not clearly stated |
Perspective used | Australian health provider |
Time frame | Lifetime |
Strategies compared |
|
Source of effectiveness data | Cohort study (SPECT data are taken from a separate published study) |
Source of QoL data | None used |
Source of utility data | None used |
Modelling approach used | Deterministic analysis |
Summary of effectiveness results | The effectiveness of the alternative strategies compared was based on the proportion of patients achieving Engel class I/II outcomes rather than Engel class III/IV. Estimated proportions of patients achieving class I/II outcomes were reported for the three strategies involving additional presurgical tests. Class I/II Engel outcomes were estimated to be 47.6% for Strategy 2, 85.8% for Strategy 3 and 97.8% for Strategy 4. The potential impact in terms of QoL was not considered |
Summary of cost results | The cost results were based on the cost of investigations and surgery for each of the three strategies involving additional presurgical tests, as well as the estimated long-term cost savings achieved following a class I/II outcome. The diagnostic and surgery interventions are assumed to cost (per patient) $10,632 for Strategy 2, $21,248 for Strategy 3 and $21,982 for Strategy 4. The cost savings achieved following a class I/II outcome relate to the long term and represent an anticipated reduction in direct medical costs due to improved Engel class. These cost savings were assumed to sum to $440,000 over the patient’s lifetime. However, it was unclear how this value was estimated |
Summary of cost-effectiveness results |
The cost-effectiveness results were presented in terms of the average cost per class I/II outcome. This was estimated to be $83,715 for Strategy 2, $92,785 for Strategy 3 and $84,222 for Strategy 4 ICERs were also presented, representing the incremental cost per additional class I/II outcome for Strategies 3 and 4 compared with Strategy 2. The ICER for Strategy 3 was $104,078 compared with Strategy 2 and for Strategy 4 is $84,703 compared with Strategy 2 |
Sensitivity analysis | Sensitivity analysis was conducted on a range of values based on the minimum and maximum reported values of each of a set of parameters. The parameters evaluated were prevalence of class I/II outcome; sensitivity and specificity of PET, SPECT and video-EEG monitoring/MRI; and the proportion of video-EEG monitoring/MRI that are indeterminate |
Main study conclusion | F-FDG PET is cost-effective in the presurgical evaluation of patients with a non-localising or non-concordant video-EEG monitoring or MRI results |
The four strategies evaluated are:
-
Strategy 1 No presurgical evaluation. All patients receive MM alone.
-
Strategy 2 Video-EEG and routine MRI alone. Patients with concordant (positive in both tests) results receive surgery; patients with concordant (negative in both tests) and discordant results receive MM.
-
Strategy 3 Video-EEG/routine MRI plus ictal SPECT. As Strategy 2, but patients with discordant results from video-EEG and routine MRI receive an additional test (ictal SPECT). Patients with positive results to ictal SPECT receive surgery and patients with negative results receive MM.
-
Strategy 4 Video-EEG/routine MRI plus FDG-PET. As Strategy 3 but patients with discordant results from video-EEG and routine MRI receive FDG-PET (rather than SPECT). Patients with positive results to FDG receive surgery and patients with negative results receive MM.
The main focus of the study is on the three imaging strategies (Strategies 2, 3 and 4) and specifically on the cost-effectiveness of FDG-PET (Strategy 4) compared with the use of video-EEG/routine MRI alone (Strategy 2). The data to inform the FDG-PET strategy (as well as the video-EEG/MRI strategy) are derived from an observational study undertaken by the authors, which is also used to estimate the concordance of video-EEG and routine MRI, whereas the data used for the SPECT comparison are derived from a separate published study. 79 The incremental cost-effectiveness of Strategies 3 and 4 are reported compared with Strategy 2.
The model used is based on a decision tree, which captures the pathways for each strategy depending on the results of the tests and the decisions concerning the use of surgery or MM. According to whether surgery or MM was provided, health outcomes differ: the proportion of patients having Engel class I/II outcomes (‘good outcome’) rather than Engel class III/IV outcomes (‘bad outcome’) depends on the treatment strategy. The Engel outcomes were then linked to estimates of lifetime cost savings through a reduction in longer-term direct costs due to improved Engel outcome.
The data collected by O’Brien et al. 78 was derived from a cohort of 176 patients who received FDG-PET scans at the Royal Melbourne Hospital as part of a presurgical evaluation for chronically refractory focal epilepsy from November 1996 to July 2001. In addition to F-FDG-PET all patients had initially undergone interictal EEG, prolonged video-EEG and MRI (16 of the 176 also received iEEG but the results and impact of undertaking this test are not reported). Although some patients in O’Brien et al. ’s sample78 had ictal and interictal SPECT (n = 15), given the small numbers the data that inform the decision tree are taken from an alternative study (Won et al. 79). However, this highlights that patients may have had a sequence of tests, which influences the decision to proceed to surgery and surgical outcomes; the implications of this sequencing are not well considered by the author or incorporated into the decision tree.
Clinical effectiveness of treatment strategies is based on patients achieving Engel class I/II outcomes. Because the cohort study also collected Engel outcome data, the results of the tests were linked directly to outcome. The sensitivity and specificity of each test for a class I/II outcome was calculated as well as the prevalence of class I/II outcomes and the proportion of indeterminate video-EEG and MRI scans. However, it is unclear from the paper how the author used these values to inform the inputs needed to fully specify the decision tree.
In terms of unit costs of visualisation technologies and surgery, the perspective of the purchaser is taken, and the author uses the Medicare schedule of costs (published by the Australian Government Department of Health and Aged Care). The lifetime cost savings after an Engel class I/II outcome (e.g. medication, hospital visits) are estimated for MM and surgery as $440,000 per patient. It is unclear how lifetime cost savings were estimated and applied in the model. It is also unclear whether or not surgical outcomes were assumed to impact on the life expectancy of patients.
The results of the decision tree analysis were provided for Strategies 2–4. It is not clear why Strategy 1 is excluded or not used to determine any of the ICERs; it can only be speculated that the author did not want to consider the broader question of the cost-effectiveness of the TLE surgery itself, so excluded the MM treatment only option. The sensitivity of the results to several parameter values is tested, including the prevalence of class I/II outcomes; the proportion of video-EEG/MRI results that are indeterminate; and the sensitivity and specificity of the visualisation tests. The calculations underlying the results provided are unclear, and no quality-of-life data are provided to inform the cost-effectiveness. As a result, it appears the ICER results provided for Strategies 3 and 4 against Strategy 2 represent the incremental cost per additional Engel class I/II outcome. Furthermore, it is unclear if these results include the estimation of cost savings over a lifetime, and it is therefore unclear if they represent only the results of the short-term decision tree analysis or the cost-effectiveness of the strategies over the lifetime of the patients.
Conclusion of review of existing cost-effectiveness evidence
The O’Brien et al. study78 was the only published study found in the review process that was considered to be in line with the scope of this assessment. The study was conducted from the viewpoint of an Australian health-care provider. The results indicate that Strategy 4 (video-EEG/routine MRI plus FDG-PET) is cost-effective. In considering the relevance of these findings to the NHS, it is important to outline the main requirements of an economic evaluation that would be appropriate for informing resource allocation decisions in the NHS:
-
The specification of the decision problem should ideally include the comparison of all diagnostic strategies that could feasibly be used in the NHS. This includes a range of non-invasive technologies over and above routine video-EEG/MRI, evaluated as single strategies or as part of a potential sequence (and also at different points within a proposed sequence). It is also important to recognise, however, that in practice these options may be constrained by the availability of evidence and the structural complexity of any model.
-
The analysis should make a clear link between the diagnostic accuracy of a given strategy, the impact on subsequent treatment decisions and the ultimate effect of these decisions on patient-related health outcomes. Hence, the relationships between the test results, the subsequent decision to proceed (or not to proceed) to surgery, and the health outcomes of alternative treatment options (surgery and continued MM) need to be assessed.
-
The ultimate health effects of the alternative strategies should be expressed in terms of a generic measure of health, such as a quality-adjusted life-year (QALY). This is because it is necessary to assess the value of improved outcomes from more accurate diagnostic tests and/or sequences of tests in units commensurate with those of programmes and interventions in other specialties and disease areas that are competing for finite health-care resources.
-
A sufficient time horizon is required to capture the long-term impact of the alternative treatment decisions. For chronic diseases, a lifetime time horizon may be appropriate.
-
The evidence used to estimate cost-effectiveness should be relevant to patients and clinical practice in the UK health service.
-
The uncertainty in the evidence base needs to be reflected in the model. This uncertainty can then be simultaneously propagated through the model using probabilistic sensitivity analysis (PSA) to reflect uncertainty surrounding the decision itself. This informs decision-makers about the probability of each strategy being the most cost-effective conditional on the value they assign to a unit of health gain. These methods can be used to provide an opportunity to use value of information methods to inform priority setting in further research.
In considering these general requirements, it is clear that there are several important limitations concerning the use of the O’Brien et al. study78 to inform decision-making in the context of the UK NHS. First, as the evaluation is conducted from the perspective of an Australian health provider, the results of the analyses may not be generalisable to the UK. Furthermore, as the description of the analytic methods provided in the paper was limited, it was not possible to either reproduce the results or to attempt to provide alternative estimates for particular inputs which are more relevant to the UK context (e.g. UK-specific costs of tests and treatments).
Second, the relationships between the test results, the subsequent decision to proceed (or not to proceed) to surgery, and the outcome of alternative treatment options in terms of their impact on QoL have not been explicitly considered. Instead, decisions to proceed to surgery were based solely on the results of specific tests or combinations of tests as opposed to being based on all available information (including patient-related information) that would occur in clinical practice, with consensus decision-making. In addition, the subsequent outcomes are expressed in terms of the proportion of patients achieving an Engel class I/II outcome, such that the final conclusions are expressed in terms of the additional cost per Engel class I/II outcome achieved. Inevitably this limits the usefulness of the results to informing system-level decision-making where it is necessary to ensure that any additional resource costs are worthwhile compared with other ways that these resources could be used in the NHS.
Although the model adopts a long-term time horizon in order to estimate potential longer-term cost savings, these estimates were not based on a formal model. Instead, a relatively simplistic calculation of projected cost-savings appears to have been made. The methods used in these calculations were not explicitly presented or discussed and hence the robustness of these projections cannot be reliably assessed. Moreover, by focusing only on the potential longer-term cost savings, this approach also ignored potential beneficial health effects. As a result, the long-term analysis is not considered sufficient to accurately represent the relevant long-term effects to the NHS.
As a result of these limitations we do not think it is possible to make an appropriate assessment of the relative cost-effectiveness of alternative visualisation strategies from this study based on the requirements of studies suitable for informing NHS decision-making. It was therefore considered necessary to develop a new decision-analytical model to assess the cost-effectiveness of technologies used to visualise the seizure focus in people with medically refractory epilepsy who are being considered for surgery, and who have already undergone a video-EEG and MRI that has resulted in an indeterminate result.
Cost-effectiveness analysis: analytical methods and model inputs
The new cost-effectiveness model provides an explicit analytic framework with which to synthesise the findings from the clinical effectiveness review (see Chapter 3) with a range of additional inputs necessary to estimate the long-term costs and outcomes of the alternative technologies used to visualise seizure focus. As previously stated, the specification of the decision problem for the cost-effectiveness analysis should ideally include the comparison of all diagnostic strategies that could feasibly be used in the NHS. However, inevitably the strategies that can be formally considered are constrained by the availability of appropriate clinical evidence. Given the limitations previously noted in Chapter 3 concerning the difficulties inherent in using existing studies to reliably inform clinical practice, the cost-effectiveness model is based largely on the single decision-study by Uijl et al. ,59 which assessed the impact of FDG-PET on the decision to go to surgery, and the outcome following surgery in those who underwent an excision procedure. This was the only study59 that was considered to appropriately address the question of which diagnostic tests actually contribute to decision-making in epilepsy surgery using consensus methods. However, as a result of using a single study that focuses on the additional value of using FDG-PET, inevitably the decision problem addressed in the cost-effectiveness analysis is more restricted that originally envisaged, being limited to assessing the potential value of a single additional non-invasive test (FDG-PET) for patients with temporal lope epilepsy who have an indeterminate result following an initial video-EEG and routine MRI.
Although the economic model can address only part of the overall decision problem (i.e. where appropriate clinical data exists), we considered that developing a model and presenting the provisional results are helpful in several respects: (1) they will demonstrate whether or not it is feasible to assess cost-effectiveness based on appropriately designed clinical studies; (2) they will help to illustrate the range of additional inputs and assumptions required and where existing data may be limited/uncertain; and (3) the model could provide a framework and an associated set of inputs and initial results that could be revised and updated in the future as new evidence emerges. Finally, although the restricted analysis inevitably means that it will not be possible to determine whether FDG-PET is more or less cost-effective than other non-invasive testing strategies or compared with a sequence of non-invasive tests, the current model does allow at least a provisional assessment of the potential value of some form of additional non-invasive testing (at least with FDG-PET).
Importantly, the benefits of the strategies being used to localise the seizure focus are dependent on the success of surgery given that the seizure focus is localised. Alongside the immediate health benefits achieved following a successful surgical procedure, the model also needs to explicitly account for the potential long-term costs and outcomes associated with both successful and unsuccessful procedures as well as those for patients who are not considered appropriate candidates for surgery and continue to receive MM. A range of data sources in addition to the Uijl et al. study59 were required to estimate the long-term costs and outcomes associated with the decisions to proceed to surgery or to continue with MM. We also sought to reflect the most recent NICE guidelines on the epilepsies (CG20 2011 draft)80 in designing and populating the longer-term model.
In developing the model, the requirements for decision-making described in Chapter 4 (see Systematic review of existing cost-effectiveness evidence) were important considerations. Therefore, a lifetime time horizon is used and costs are evaluated from the perspective of the NHS and Personal Social Services (PSS), expressed in UK pounds sterling at a 2010 price base. Outcomes in the model are also expressed in terms of QALYs. Both costs and outcomes are discounted using a 3.5% annual discount rate as is consistent with current guidelines (NICE 200877). The model itself was developed using software R (The R Foundation for Statistical Computing, Vienna, Austria). 81
The model is also probabilistic in that many of the parameters that inform the model are described by a distribution that reflects the second order uncertainty around their mean values. Monte Carlo simulation is used to propagate this uncertainty in input parameters in order to report cost-effectiveness results alongside decision uncertainty. The probabilistic approach also provides a methodological framework for testing the consequences of parameter uncertainty and can be used to highlight areas for further research.
The following sections outline the decision problem and the structure of the model as well as providing an overview of the key assumptions and data sources used to populate the model.
Population
The decision problem addressed by the decision model is the cost-effectiveness of presurgical visualisation strategies for the lateralisation of seizure focus in medically refractory epileptic patients who have had discordant findings from initial video-EEG and MRI scans. Patients who are deemed eligible for a presurgical evaluation have been defined as medically refractory through their failure to respond suitably to at least two antiepileptic drugs. This definition of patients as medically refractory as a prerequisite of surgical candidacy is commonly used and is consistent with the studies used to populate the model. However, owing to the nature of the clinical data available the decision was made to constrain the analysis of the model to only patients with TLE.
Model structure
The main objective of the decision-analytic model is to estimate the incremental cost-effectiveness of FDG-PET in people with medically refractory TLE who are being considered for surgery, and who have already undergone a video-EEG and MRI that has resulted in an indeterminate result (i.e. the decision to proceed to surgery is uncertain). Rather than providing a complete picture of the cost-effectiveness of the full range of alternative strategies, it is intended that the development of the model serves to provide an illustration of how data from appropriately designed clinical studies can be used to inform a decision model to evaluate the cost-effectiveness of visualisation technologies in the presurgical evaluation of TLE surgery. Through this process the model also highlights the range of additional data that are required to appropriately assess the long-term impact of the initial decisions on costs and outcomes suitable for assessments of cost-effectiveness.
The model itself has two components: a short-term element, which characterises the period over which these localisation strategies are applied, and, if appropriate, surgery is conducted, and a long-term element, which considers the costs and outcomes over the remaining lifetime of the patient. The model is designed to evaluate the impact of the application of further tests on the decision made by a clinician after an initial round of feasibility tests for TLE surgery.
The technologies we could evaluate were conditioned by the availability of data. We thus focused on FDG-PET and iEEG, the technologies evaluated in the only ‘decision study’ identified. 59 Even with this restricted set of technologies, a number of separate strategies can be considered based on how the technologies could be used, i.e. combination of tests and the order in which these are applied. The following strategies, to be applied to patients who have had discordant findings from initial video-EEG and MRI scans, were included in the current evaluation:
-
Strategy 1 No additional tests are performed.
-
– All patients with indeterminate results from MRI/EEG receive MM.
-
-
Strategy 2 FDG-PET is performed; if the result of this test does not lead to a positive (S+) or negative (S–) decision to undertake surgery and still leaves the clinician uncertain whether or not to proceed to surgery (S?) then no further tests are performed.
-
– If S+ after FDG-PET: patients are offered surgery.
-
– If S– after FDG-PET: patients are offered MM.
-
– If S? after FDG-PET: patients are offered MM.
-
-
Strategy 3 FDG-PET is performed; if the result of this test does not lead to a clear decision on whether or not the patient should proceed to surgery (S?), iEEG is offered.
-
– If S+ after FDG-PET: patients are offered surgery.
-
– If S– after FDG-PET: patients are offered MM.
-
– If S? after FDG-PET: patients are offered iEEG and the evaluation of the result of this test determines the provision of treatment (S+ after iEEG implies a decision to proceed to surgery, whereas S– and S? assume MM).
-
As the model is based directly on the approach and results of the single study by Uijl et al. ,59 the model incorporates a series of assumptions about the sequencing of visualisation technologies that are inherent within the Uijl et al. study:59
-
Owing to the invasive nature of the iEEG, it is assumed that it would always be the last preoperative test available to the clinician; therefore, in the situation outlined above, FDG-PET will always precede it.
-
iEEG never directly follows the initial MRI and video-EEG. This assumption is made because an uncertain MRI and video-EEG may lack the potential to accurately define a sufficiently specific location for the iEEG to visualise (iEEGs can be carried out over only a small brain section at any time). An additional FDG-PET that results in an ‘S?’ is assumed to provide enough additional evidence to facilitate investigation by iEEG. In clinical practice, further non-invasive imaging may be undertaken; given the absence of evidence, however, it was not possible to explicitly evaluate such strategies in the current assessment.
Non-invasive tests are assumed to have to potential to provide sufficient information to facilitate TLE surgery. This assumption is consistent with our understanding of clinical practice. 82
Short-term model
The short-term model is structured as a decision tree as shown in Figure 3, reflecting the short-term clinical outcomes and adverse events associated with each of three localisation strategies. For modelling purposes the short-term model is assumed to have a time span of 1 year for all of the strategies analysed. This time span was chosen to be consistent with the main study used to inform the longer-term modelling. 83
FIGURE 3.
Structure of the short-term decision tree. DS, experiencing at least one disabling seizure.
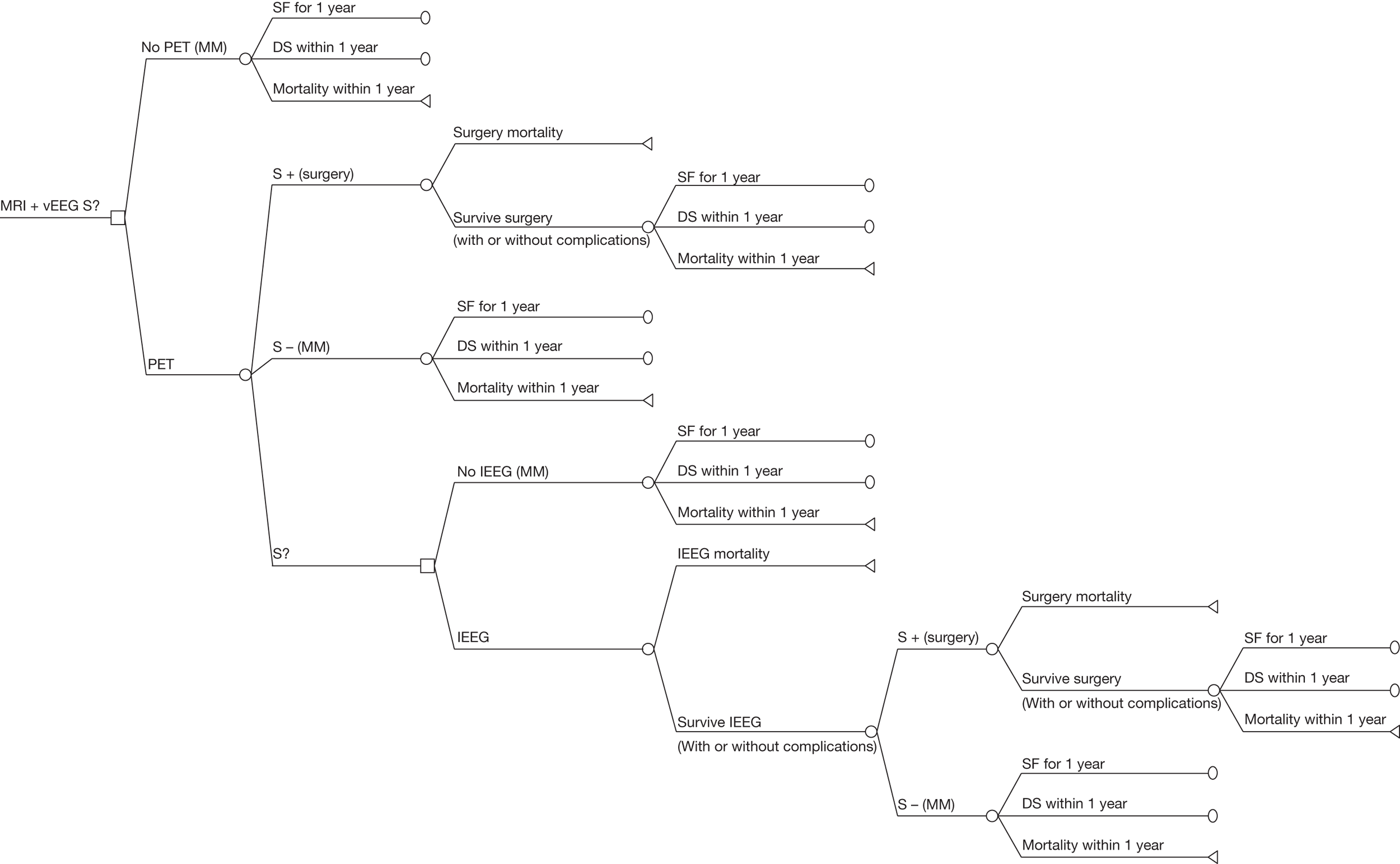
The short-term model defined in this section was based on the structure of the available evidence in the study by Uijl et al. ,59 in that it considered the use of MRI and video-EEG followed by the possibility of FDG-PET and iEEG as screening strategies. As a result, the three strategies outlined in Figure 3 are directly informed by the data presented by Uijl et al. 59 as discussed in previous sections.
Within Strategy 1, no further visualisation technologies are used after an indeterminate result of previous tests (video-EEG + MRI ‘S?’). Given that the focus is not localised, surgery is not provided, and the patients are assumed to be treated with MM alone.
Strategy 2 is defined as the provision of FDG-PET to the patient population. The results of FDG-PET define the treatment strategy recommended to the patient. The FDG-PET scan may yield a result that is consistent with clinical requirements to proceed to surgery (represented by S+ after FDG-PET in Figure 3). These patients are offered surgery, to which they may comply, or not. Patients for whom the results of the FDG-PET scan lead to a decision not to proceed to surgery (S– after FDG-PET) or are inconclusive (S? after FDG-PET) are assumed to be provided with MM.
Strategy 3 also includes the provision of FDG-PET; however, if the result of the FDG-PET scan is inconclusive then the patient is a candidate for a further visualisation technology – an iEEG. The iEEG test is assumed to be definitive and has two possible outcomes: surgical candidacy or not.
Both invasive procedures that may occur during the short-term model (iEEG and surgery) are associated with risk of death and complications. Non-fatal complications, which may occur as a result of invasive procedures, are defined as either transient (i.e. only affecting the individual for a year after surgery, e.g. postoperative infections) or permanent [i.e. having a permanent impact on health-related quality of life (HRQoL), e.g. verbal decline, hemianopsia or hemiparesis]. For simplicity of presentation and as complications are not assumed to have additional prognostic implications, neither transient nor permanent complications are represented in the short-term model in Figure 3. However, the cost and impact on QoL are incorporated. It is also assumed that all patients who experience a permanent complication during an iEEG are not offered surgery and receive only MM.
In the base-case, short-term model it will be assumed that compliance to surgery and iEEG is 100%, such that all patients who are deemed suitable for surgery or an iEEG subsequently receive them. This assumption, and the sensitivity of results to it, will be considered later in this chapter. In addition compliance with FDG-PET will also be assumed to be 100%.
The short-term tree considers three treatment end points: (1) patients can be deemed unsuitable for surgery and continue to receive MM alone for their epilepsy, (2) the patients can be referred for surgery and survive the procedure or (3) the patients can die as a result of procedure-related mortality due to the use of iEEG (Strategy 3 only) or surgery (Strategies 2 and 3). For all patients in Strategy 1, and those patients who survive the initial procedure for Strategies 2 and 3, there are three possible outcomes: (1) achieving seizure freedom for a year after the treatment option was provided, (2) having a disabling seizure (DS) within that year or (3) dying within that year from epilepsy- or non-epilepsy-related events. In the event of death (procedural or during the course of the year), the patient’s progression through the model stops.
There are a limited number of costs associated with the short-term model:
-
cost of FDG-PET and iEEG
-
cost of TLE surgery
-
additional cost of complications occurring during surgery or iEEG.
No costs other than those directly related to the pathways of the patients through the decision tree are considered.
Similarly, the impact on HRQoL – expressed in terms of heath disutility – associated with these patients is reduced due to factors directly related to the patient pathways considered; these are:
-
disutility of transient and permanent complications.
Long-term Markov model
The long-term model aims to characterise the long-term prognosis for patients leaving the short-term model after (1) achieving seizure freedom for a year after the treatment option was provided or (2) having a DS within that year. As we have highlighted previously, imaging strategies allow for the identification of patients eligible for surgery, and thus their benefits are largely dependent on how much better the outcomes attained with surgery are in comparison with MM. The long-term structure of the model will reflect the differences between surgery and MM. Important factors in the evaluation of the long-term prognosis are whether the patients have seizures (or DSs) and whether therapy with antiepileptic drugs is sustained. 84
As the focus of our research project was on the performance and value of imaging technologies as opposed to specifically evaluating the clinical effectiveness and cost-effectiveness of the subsequent treatment strategies, the long-term model was based largely on a previously published study. 83 This study by Choi et al. 83 used a decision model (including a long-term Markov model) to estimate life expectancy and quality-adjusted life expectancy of surgery and MM for patients with refractory epilepsy. Despite implications on costs not having been quantified in Choi et al. ’s study,83 given the aims and methods used we believe this study constitutes an appropriate basis to inform the current model. Also, the study by Choi et al. 83 was informed by a seemingly comprehensive search of the evidence base: a series of detailed reviews of clinical, epidemiological and QoL data were undertaken. Given the constraints of our project, we did not attempt to update or critically appraise these reviews. Instead, we focused on adapting the study for the purposes of assessing long-term costs and QALYs for a UK cost-effectiveness study.
The model we use is presented in Figure 4 and is adapted from the long-term Markov model implemented by Choi et al. 83 In a Markov model, hypothetical individuals reside in one out of a set of mutually exclusive health states at particular points in time. During discrete time intervals of equal length (normally referred to as Markov cycles), individuals can either remain in a particular health state or move to a separate health state (e.g. because of a patient experiencing a particular clinical event). The movements between states represent the potential clinical pathways that a patient may follow at different time points and over his or her remaining lifetime. The likelihood that an individual remains in a particular health state, or moves to a separate state, is specified in terms of transition probabilities. Defining and subsequently estimating these transition probabilities represent key structural and analytical elements of any decision model. Here, patients enter the Markov model directly from the short-term model either having been SF for the first year, having a DS within that year or having died. The probability of entering each of these states is therefore different depending on whether the patients were treated by MM or surgery.
FIGURE 4.
Structure of the long-term Markov model.
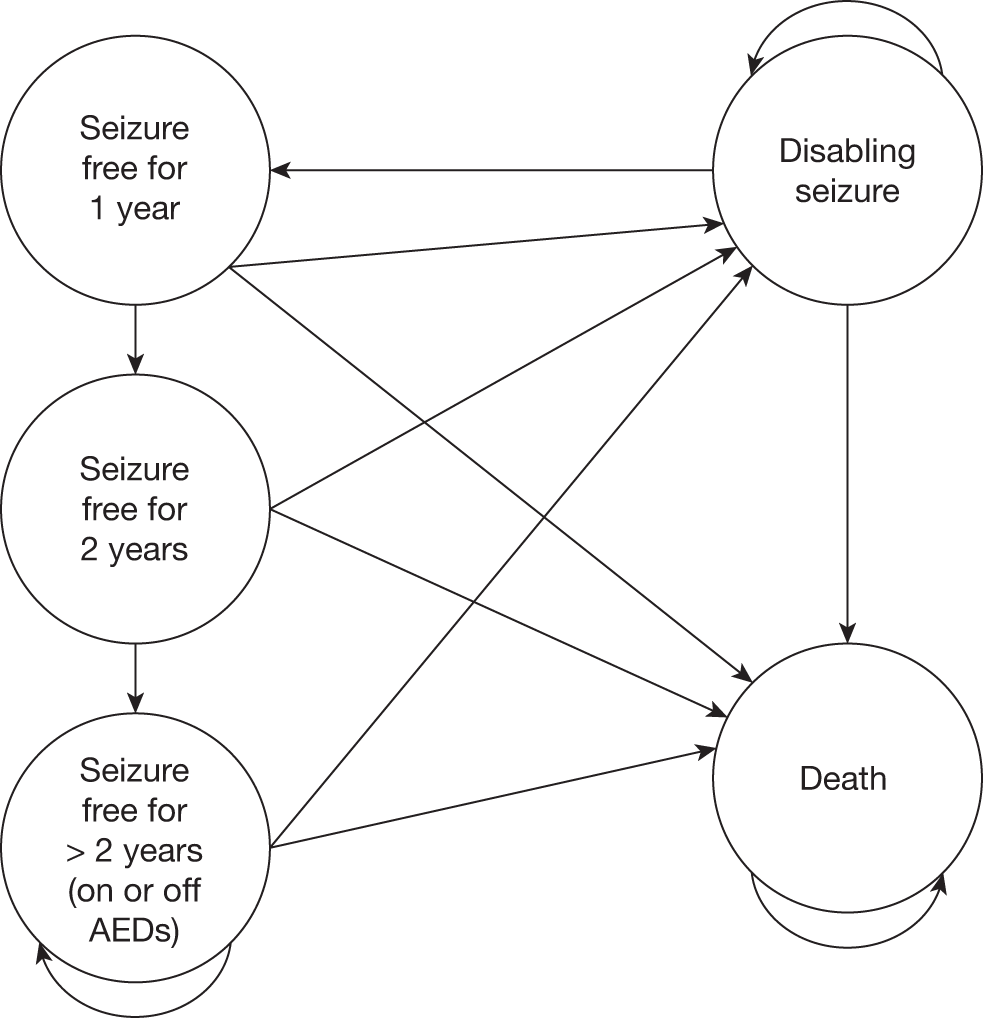
Patients who are long-term SF in the Markov model (defined here as having more than 2 years of seizure freedom) may be given the possibility of having the treatment with AEDs stopped, as is consistent with NICE clinical guidelines. 80 We expect that accounting for this will impact mainly on the long-term costs of care for patients, and represents an additional possible benefit of surgery. To incorporate this into the model, the state representing seizure freedom was separated into a set of ‘tunnel states’: SF for 1 year, SF for 2 years, and SF for 3 years and longer. This modelling ‘trick’ allows for tracking the time patients are SF, incorporating time dependency into the model without having to define a more complex structure. Specifically, when in the first two health states, patients face a probability of dying, of having a DS or progressing to the next state. Patients who maintain SF for more than 2 years arrive at the health state ‘SF for 2 or more years’. Patients in this SF state can be either on or off AEDs, such that not all patients who are long-term SF are removed from treatment to allow for the implicit inclusion of other factors that may relate to the decision to discontinue medication. The proportion that is removed is defined as ‘pAED’ and is discussed later in this chapter. 85,86 In the next time cycle, patients can remain in this state, die or have DSs again, in which case patients that were off AEDs are assumed to restart MM. All MM and surgically treated patients are assumed to receive AED treatment in the short-term; however, all patients have the possibility to become long-term AED-free through prolonged seizure freedom. The long-term model developed from Choi et al. 83 also assumes that there is no discontinuation of AEDs in either the MM or the surgical treatment strategies other than discontinuation due to prolonged seizure freedom or death.
Patients who have some form of DS in a year can either remain in this state (such that in the next year they have another DS), die or become SF and as such transit to state ‘SF for 1 year’ as shown in Figure 4. Owing to limitations in the available data the model does not correlate seizure history with the risk of having seizures in the following year.
The patients who have experienced a transient or permanent complication during an invasive procedure are assumed to have the same long-term transition probabilities but have an additional QoL disutility applied in the model.
From all states in the long-term model patients face the risk of death; this can be epilepsy related or due to unrelated reasons. The impact of epilepsy on mortality for any given cycle of the model is assumed to be different for patients who are SF from those who have had a DS in that year.
Patients’ transition probabilities in the long-term model depend on whether they are being treated with MM or have had surgery, but the same structure represented in the figure above applies to both treatment options. The difference between the sets of transition probabilities will be discussed later in this chapter.
The efficacy of the AED used in the MM treatment strategy (and therefore in the NHS) is assumed to be consistent with that used in Choi’s analysis; for simplicity this will be assumed to represent an average of AEDs recommended by NICE. 80 The efficacy of specific individual AEDs or adverse events associated with their use were not considered in the model.
The costs considered in the long-term model are as follows:
-
cost of an annual course of AEDs
-
non-drug costs of provision of treatment to epilepsy sufferers who are SF and on AEDs (e.g. general practitioner visits)
-
non-drug costs of provision of treatment to epilepsy sufferers who have had a DS in that year (e.g. hospital admissions, outpatient visits, etc.).
The impact on QoL was assessed by applying utility decrements to age/sex match estimates of QoL from the UK general population. The HRQoL decrements reflect the disutility of having:
-
at least one DS in a given year
-
a transient complication (only applied during the first year after surgery)
-
a permanent complication following an invasive procedure (applied throughout the remainder of a patient’s lifetime).
Model inputs
The sources of input data and a description of the available evidence used to inform the decision model are reported in this section. In the base case, it is assumed that the age at the start of the model is 35 years old. 83 The Choi et al. study83 (a study based on American data) is used for the base-case age for two reasons: first, a suitable study identifying the relevant distribution of epilepsy suffers’ ages in the UK was not identified, and, second, the long-term model relies on many transition probabilities from Choi et al. ’s study,83 which may be less applicable to patients of different ages. The proportion of male to female patients was assumed to be 0.49, based on data derived from the CIA World Factbook (2011 estimates) for that population age. No data were available on the male–female ratio in epilepsy specifically, which is often claimed to affect women more than men. 87
Short- and long-term transition probabilities
Both the short- and long-term models are specified using a set of probability parameters that determine the likelihood of the possible outcomes with each of the three different visualisation strategies. The expected values assumed for these probability parameters, and associated uncertainty, are reported in Tables 13 and 14, respectively, for the short and long term.
Parameter | Base-case value (95% CI) | Distribution | Source |
---|---|---|---|
Surgical eligibility after FDG-PET | |||
Probability of S+ after FDG-PET | 0.56 (0.46 to 0.65) | Beta | Uijl et al.59 |
Probability of S– after FDG-PET | 0.20 (0.12 to 0.29) | ||
Probability of S? after FDG-PET | 0.14 (0.08 to 0.22) | ||
Surgical eligibility after iEEG | |||
Probability of S+ after iEEG | 0.83 (0.74 to 0.89) | Beta | Uijl et al.59 |
Probability of S– after iEEG | 0.17 (0.10 to 0.25) | ||
Adverse events with invasive procedures | |||
Probability of transient complications, surgery or iEEG | 0.08 (0.06 to 0.1) | Beta | Choi et al.83 |
Probability of permanent complications, surgery or iEEG | 0.04 (0.02 to 0.06) | ||
Probability of mortality after surgery or iEEG | 0.003 (0 to 0.0075) | ||
Outcomes of treatment | |||
TP of SF patients in the first year, MM | 0.08 (0 to 0.16) | Beta | Choi et al.83 |
TP of SF patients in the first year, surgery | 0.719 (0.695 to 0.743) |
Parameter | Base-case value (95% CI) | Distribution | Source |
---|---|---|---|
TPs for MM | |||
TP from SF to DS after the first year, MM | 0.254 (0.109 to 0.462) | Beta | Choi et al.83 |
TP from DS to SF between first and fifth years, MM | 0.047 (0.03 to 0.07) | ||
TP from DS to SF after the fifth year, MM | 0.016 (0.01 to 0.023) | ||
TPs for surgery patients | |||
TP from SF to DS between first and fifth years, surgery | 0.056 (0.029 to 0.083) | Beta | Choi et al.83 |
TP from SF to DS after the fifth year, surgery | 0.042 (0.016 to 0.068) | ||
TP from DS to SF between first and fifth years, surgery | 0.059 (0.009 to 0.11) | ||
TP from DS to SF after the fifth year, surgery | 0.02 (0.002 to 0.072) | ||
Relative mortality risks relative to general population | |||
For patients in seizure freedom | 1.11(0.63 to 1.93) | Log-normal | Choi et al.83 |
For patients in DS, MM | 5.64 (3.49 to 9.12) | ||
For patients in DS, surgery | 5.42 (3.97 to 7.77) | ||
AED treatment | |||
pAED | 0.157 | N/A | Wieser et al.86 |
Of relevance for the short-term model are the characterisation of surgical eligibility after knowing the results of the visualisation technologies and the compliance and complications (including mortality) rates associated with invasive procedures. The data used to inform the expected outcome of visualisation technologies (in terms of S+, S– and S?) were derived from the Uijl et al. study. 59 In this study, a sample of 469 patients was analysed for the clinical consensus on a decision to undertake surgery after video-EEG and MRI. This decision was then was revised after a further test was applied. To populate the model we used the decision after FDG-PET, in patients for whom the consensus panel did not reach an initial decision (our scoped population: note that although Uijl et al. ’s study59 population was exposed to several other tests, she notes that the results of all of these tests were associated with the final consensus decision as to surgical candidacy). Conditional beta distributions were fitted to characterise the underlying uncertainty in the probabilistic model. The probability of a complication (mortality and transient and permanent complications) occurring after an invasive procedure was based on data from Choi et al. 83
In addition to the short-term visualisation treatment-related decisions and events it is necessary to consider the outcome of patients receiving MM or surgery in the decision tree. Although Uijl et al. ’s study59 reports the outcome of surgery, Choi et al. ’s study83 has been used to populate the base-case model. This was because the study by Uijl et al. 59 did not report similar outcomes for patients who did not have surgery. In addition, the definitions applied in the study by Uijl et al. differed to those applied in the long-term model by Choi et al. ;83 the study by Uijl et al. 59 presents outcomes in terms of the proportion of patient achieving Engel Ia (SF without auras), whereas the long-term model adapted from Choi et al. 83 assessed seizure freedom defined as entirely SF or having only simple partial seizures that do not affect consciousness (i.e. auras).
The parameters defining disease progression through the long-term Markov model are transition probabilities, characterising the occurrence and speed of transitions between the different health states defined in the model (discussed earlier in this chapter and represented graphically in Figure 4). Relative mortality risks were also used to adapt the mortality risks of the general population to reflect the additional mortality risk due to epilepsy. As previously described, these inputs were based on those reported in the study by Choi et al. 83 Table 14 lists the probabilities applied in the long-term model.
Resource use and cost estimates
The costs used in the full model are provided in Table 15. All of the data used to inform the costing of different arms of the model were sourced from NHS publications or NHS data directly. The cost categories considered were costs of visualisation strategies, costs of treatments (surgery and MM) and the non-drug treatment costs associated with the permanence in the health states of the long-term model (SF and DS). The costs associated with the short-term model (i.e. visualisation and surgery costs) were all obtained from the 2009–10 NHS national schedule of reference costs. 88
Parameter | Base-case value (£) | Distribution | Source of data |
---|---|---|---|
FDG-PET | 398 | Fixed | NHS reference costs 2009–10,88 nuclear medicine category 5 |
iEEG | 3306 |
NHS reference costs 2009–10 88 Elective inpatient – code AA20Z (category 1/2) |
|
Surgery (no complications) | 6171 |
NHS reference costs 2009–10 88 Elective inpatient – code AA20Z (category 4) |
|
Surgery complication | (1990 – 1290) = 700 |
NHS reference costs 2009–10 88 Difference between elective inpatient ‘epilepsy syndrome’ with and without CC |
|
Average cost of an annual course of AEDs to MM and surgery patients | 448.80/6 months | NICE guideline 20, 201180 and author assumptions | |
Non-drug costs of a SF patient | 110 | Gamma | NICE guideline 20, 201180 (see appendix P)80 |
Non-drug costs of a seizure-persistent patient (for MM and surgery) | 482 |
The assumptions regarding drug costs associated with treatment with AEDs were based on the NICE draft guidelines on the epilepsies (2011). 80 The guidelines suggest that the drug treatment of focal epilepsy with or without secondary generalisation should comprise:
-
first-line AED: carbamazepine, lamotrigine, levetiracetam, oxcarbazepine and sodium valproate
-
adjunctive AEDs: carbamazepine, clobazam, gabapentin, lamotrigine, levetiracetam, oxcarbazepine, sodium valproate and topiramate
-
other AEDs that may be considered: eslicarbazepine acetate, lacosamide, phenobarbital, phenytoin, pregabalin, tiagabine, vigabatrin and zonisamide.
Given the absence of relevant evidence on the literature on the use, in the UK, of these alternative treatments, the costs of AED treatment were here assumed to be the average of the cost of first-line AEDs added to the average of costs of carbamazepine and vigabatrin (adjunctive therapies). Carbamazepine and vigabatrin were chosen as they are the only two cost-effective adjunctive therapies presented in the NICE guideline (2011). 80 The assumptions used will be further explored using sensitivity analyses (see Impact of parameter uncertainty).
The non-drug costs associated with epilepsy sufferers were also obtained from the NICE 2011 guidelines documents80 and provided estimates of costs for SF and for seizure-persistent patients, based on their expected use of inpatient and outpatient clinics, as well as accident and emergency and general practice time.
Health-related quality-of-life model inputs
Table 16 provides the HRQoL model inputs used in the base-case analysis, as well as the sources and the distribution used in the probabilistic analysis (if there is no uncertainty considered in the model then the distribution is given as fixed).
Parameter | Base-case value (95% CI) | Distribution | Source |
---|---|---|---|
Disutilities relative to general population | |||
Disutility for SF in MM | 0.00 (–0.08 to 0.08) | Normal | Choi et al.83 |
Disutility for SF in surgery, no complication | 0.00 (–0.06 to 0.06) | ||
Disutilities relative to correspondent SF states | |||
Disutility for DSs in MM | –0.21 (–0.62 to –0.02) | Gamma | aChoi et al.83 |
Disutility for SF in surgery, permanent complication | –0.20 (–0.65 to –0.01) | ||
Disutility for SF in surgery, transient complication | –0.01 (–0.01 to 0.00) | ||
Disutility for DSs in surgery, permanent complication | –0.31 (–0.83 to –0.04) | ||
Disutility for DSs in surgery, transient complication | –0.22 (–0.62 to –0.03) |
Health-related quality of life was determined by assuming disease-specific decrements (disutilities) in relation to the HRQoL of the general population. The study of Kind et al. 89 was used to inform the HRQoL of the general population. This study applied European Quality of Life-5 Dimensions (EQ-5D) questionnaires to a sample of individuals from the general population and presents results for a given age and gender. The standard deviations (SDs) and subsample sizes provided in the Kind et al. study89 were used to define SEs, allowing uncertainty over these estimates to be incorporated into the probabilistic model.
Decrements applied to these general population estimates were again derived directly from the Choi et al. study83 and are also given in Table 16. These decrements represent the reduction in HRQoL related to the SF state and disabling states (separately for MM or surgery treatments). Uncertainty over these parameters was assumed to be described by a gamma distribution.
Assumptions necessitated by available data
Several assumptions were necessary to consider the cost-effectiveness of visualisation strategies in the manner presented in the previous section, relating to both the short- and long-term models. In addition to the model structures being derived from respective studies of Uijl et al. 59 and Choi et al. ,83 a significant number of data were also sourced from these studies. The assumptions necessary to use these data, as well as those needed to link the two parts of the model together, are discussed in the following section.
All patients were assumed to comply with invasive procedures in the base case (also to tests and treatments), with no associated uncertainty. This assumption was applied, as Uijl et al. 59 did not report compliance data. This assumption will be evaluated further in the scenario analysis, reported later in this report.
In addition, in using data from the study by Uijl et al. ,59 several other assumptions had to be made about the nature of the data reported and the patient pathways:
-
all patients in the study by Uijl et al. 59 who have an S– result from FDG-PET are assumed in the model to receive the MM treatment strategy
-
all patients who have an S+ result from the FDG-PET are assumed to receive the surgery treatment strategy.
Several other assumptions had to be made in informing the short-term model:
-
Mortality due to an invasive procedure is assumed to be the same for iEEG and TLE surgery. In Choi et al. ’s study83 it is recognised that, although there is no evidence suggesting a mortality risk associated with the TLE surgery, there is a risk associated with the use of anaesthetic in such procedures.
-
In the cases of all visualisation and surgical technologies it is assumed that the technology is already in place, such that the cost considered is equal to the marginal cost as reported in the NHS schedule for reference costs 2009–10. 88 This can be extended to assume that all of the relevant expertise necessary exists as spare capacity, such that there is no ‘learning-curve’ associated with any of the strategies.
The long-term model is also based on a several additional assumptions:
-
As clinical recommendations suggest (NICE guidance 20, 201181), the models assumes that a proportion of patients are taken off treatment with AEDs if they are SF for a minimum period of 3 years.
-
Owing to limitations in the available data, it is assumed that patients who are taken off AEDs in our model have the same mortality and the same likelihood of having a DS as those who are SF but still on AEDs. The decision to discontinue AEDs is also being considered independent of the probability of a relapse occurring.
-
The evidence from Choi et al. 83 is used throughout the time horizon of the model (e.g. the likelihood of relapse). As this evidence is drawn from the wider literature, it is not always clear what time frames were used in the input data.
Similarly, several assumptions have been made to incorporate Choi et al. ’s83 QoL scores into this study’s model:
-
Choi et al. 83 assume the uncertainty over the QoL scores to be represented by a uniform distribution. Given that we, here, used disutilities rather than the scores themselves, gamma distributions were used instead. Although this assumption may cause a degree of error from fitting a new distribution using the CIs provided, this effect is likely to be small.
-
Quality-of-life decrements due to events in the model are applied against the expected QoL for an individual of a certain age and gender. 89
As with all decision models, by using distinct sources of data these need to be assumed valid for their purpose. The link between the short-term results and the longer-term progression is based on different data sets, which, in their own rights, were considered here to represent the best available evidence to populate the cost-effectiveness model. The sample considered in the Uijl et al. study59 is thus assumed to be representative of the population defined in our decision problem. Similarly, the evidence from Choi et al. 83 is assumed to represent adequately the progression in the disease, conditional on knowing the treatment decision of whether or not to proceed to surgery. The assumptions were necessary, given the limited evidence available to populate both the short- and long-term models and also given the resource constraints of this project, which meant it was not possible to develop our own long-term model. However, the robustness of the results to several key assumptions is explored within later sections.
Cost-effectiveness: results
The results of the decision model are presented in two ways. First, the mean lifetime costs and QALYs of the alternative strategies are presented and their cost-effectiveness compared, estimating incremental cost-effectiveness ratios (ICERs) where appropriate. When more than two interventions are being compared, the ICERs are calculated using the following process:
-
The strategies are ranked in terms of cost (from the least expensive to the most costly).
-
If a strategy is more expensive and less effective than any previous strategy then this strategy is said to be dominated and is excluded from the calculation of the ICERs.
-
The ICERs are calculated for each successive alternative, from the cheapest to the most costly. If the ICER for a given strategy is higher than that of any more effective strategy then this strategy is ruled out on the basis of extended dominance.
-
Finally, the ICERs are recalculated excluding any strategies that are ruled out by principles of extended dominance.
The threshold cost per QALY estimates used by NICE (£20,000–30,000) is used to provide an indication of whether or not the visualisation strategies represent good value for money in the NHS.
Second, the results of the probabilistic analysis using Monte Carlo simulation are used to calculate the combined impact of the model’s various uncertainties on the overall uncertainty surrounding the cost-effectiveness results themselves. To present the uncertainty in the cost-effectiveness of the alternative strategies, cost-effectiveness acceptability curves (CEACs) are used. The CEAC shows the probability that each strategy is cost-effective using alternative values for the threshold cost per QALY. Separate cost-effectiveness estimates are also reported for different scenarios reflecting the uncertainty in several of the key inputs and assumptions.
The results from the overall model are presented in the following two sections. The first section provides the results from the base-case analysis, in which all of the assumptions set out in the previous sections of this chapter are implemented. As part of this section we will present results of assessments of the importance of parameters on the results of the base-case model. The second section provides the results for a set of sensitivity analyses, in which several assumptions made in the aforementioned section are relaxed or changed and any changes to the results are recorded.
Base-case analysis
The results of the base case are presented in Table 17 for the three strategies.
Strategy | Cost (£) | QALYs | ΔCost (£) | ΔQALYs | ICER (£) | Probability of being cost-effective at | |
---|---|---|---|---|---|---|---|
£20,000 | £30,000 | ||||||
Strategy 1 | 23,783 | 12.88 | – | – | N/A | 0.14 | 0.13 |
Strategy 2 | 26,637 | 14.59 | 2854 | 1.71 | 1671 | 0.03 | 0.03 |
Strategy 3 | 27,710 | 14.92 | 1074 | 0.34 | 3201 | 0.83 | 0.84 |
The results show that Strategy 2 (use of FDG-PET following an indeterminate result with video-EEG and MRI) represents a more expensive but more effective strategy than Strategy 1 (no further non-invasive or invasive tests). The ICER of Strategy 2 compared with Strategy 1 is £1671 per QALY. Strategy 3 (use of iEEG following an indeterminate result with FDG-PET and video-EEG/MRI) is also more costly and also more effective than Strategy 2. The ICER of Strategy 3 compared with Strategy 2 is £3201 per additional QALY.
At a threshold of £20,000 per QALY, the probability that Strategy 3 is the most cost-effective strategy is 0.83. This probability increases marginally to 0.84 at a threshold of £30,000 per QALY. Figure 5 presents the decision uncertainty surrounding the probability that each strategy is cost-effective using multiple CEACs across a range of potential threshold amounts a decision-maker might be prepared to pay for a QALY.
FIGURE 5.
Cost-effectiveness acceptability curves for the base-case analysis.
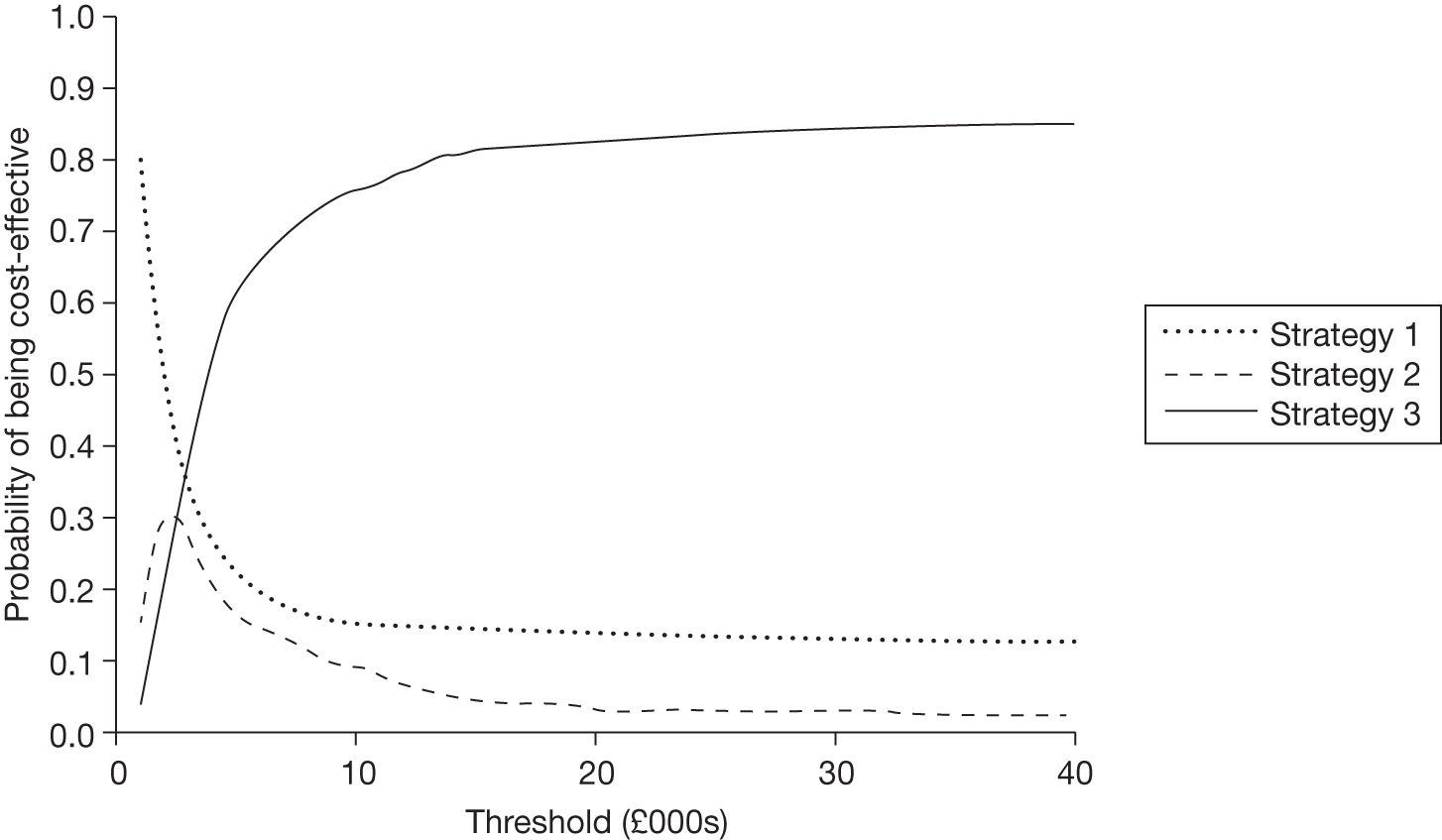
Consequently, at conventional thresholds used to determine value for money in the NHS, Strategy 3 – the use of FDG-PET for indeterminate cases following video-EEG and routine MRI, together with iEEG for any cases that are still indeterminate following the FDG-PET – appears to be the most cost-effective strategy, with low uncertainty surrounding this decision.
Impact of parameter uncertainty
The probabilistic nature of the model used in this study implies that uncertainty about the expected values of many of the parameters was explicitly considered in evaluating cost-effectiveness results. An understanding of the uncertainty and of its likely consequences is useful in identifying the sources of uncertainty that matter to the decision problem.
First, we considered the impact of alternative parameter values in the adoption decision. By taking each parameter in turn, we evaluated cost-effectiveness for the range of values a parameter can take. Parameter values leading to different adoption decisions (different strategies being considered cost-effective) were noted and Table 18 summarises the results. The mean values of each uncertain parameter assumed in the analyses are presented alongside. These results assumed a £20,000 per QALY threshold value. It is important to note that the theoretical range of values a variable can take is not the same in all cases: it is 0–1 in the case of probabilities and baseline utility, 0 to infinity (in the case of ratios) or minus infinity to 0 (in the case of utility decrements).
Variable | Mean value | Strategy 1 | Strategy 2 | Strategy 3 |
---|---|---|---|---|
Short-term probabilities | ||||
Probability of S+ after FDG-PET | 0.56 | – | – | 0 to 1 |
Probability of S– after FDG-PET, given not S+ | 0.68 | – | – | 0 to 1 |
Probability of S+ after iEEG | 0.83 | – | 0 to 0.125 | 0.125 to 1 |
Probability of permanent complications, iEEG | 0.04 | – | – | 0 to 1 |
Probability of transient complications, surgery | 0.08 | – | – | 0 to 1 |
Probability of permanent complications, surgery | 0.04 | 0.93 to 1 | 0.80 to 0.93 | 0 to 0.80 |
Probability of mortality after surgery | 0.003 | 0.20 to 1 | 0.17 to 0.20 | 0 to 0.17 |
Probability of mortality after iEEG | 0.003 | – | 0.08 to 1 | 0 to 0.08 |
TP of SF patients in the first year, MM | 0.08 | – | – | 0 to 1 |
TP of SF patients in the first year, surgery | 0.719 | – | – | 0 to 1 |
Long-term TPs | ||||
TP from SF to DS after the first year, MM | 0.254 | – | – | 0 to 1 |
TP from SF to DS between first and fifth years, surgery | 0.056 | – | – | 0 to 1 |
TP from SF to DS after the fifth year, surgery | 0.042 | – | – | 0 to 1 |
TP from DS to SF between first and fifth years, MM | 0.047 | – | – | 0 to 1 |
TP from DS to SF after the fifth year, MM | 0.016 | 0.45 to 1 | 0.34 to 0.45 | 0 to 0.34 |
TP from DS to SF between first and fifth years, surgery | 0.059 | – | – | 0 to 1 |
TP from DS to SF after the fifth year, surgery | 0.02 | – | – | 0 to 1 |
Long-term mortality ratios (in relation to the general population) | ||||
For patients in SF | 1.11 | – | – | 0 to max. |
For patients in DS, MM | 5.64 | 0 to 0.6 | 0.6 to 1 | 1 to max. |
For patients in DS, surgery | 5.42 | – | – | 0 to max. |
HRQoL and disutilities | ||||
Baseline utility | 0.91 | – | – | 0 to 1 |
Disutility for SF in MM | 0 | – | – | Min. to 0 |
Disutility for SF in surgery, no complication | 0 | Min. to –0.27 | –0.27 to –0.23 | –0.23 to 0 |
Disutility for SF in surgery, permanent complication | –0.2 | – | – | Min. to 0 |
Disutility for SF in surgery, transient complication | –0.01 | – | – | Min. to 0 |
Disutility for DS in MM | –0.21 | –0.04 to 0 | –0.06 to –0.04 | Min. to –0.06 |
Disutility for DS in surgery, no complication | –0.19 | Min. to –0.55 | –0.55 to –0.50 | –0.50 to 0 |
Disutility for DS in surgery, permanent complication | –0.31 | – | – | Min. to 0 |
Disutility for DS in surgery, transient complication | –0.22 | – | – | Min. to 0 |
Other | ||||
Proportion of patients off AEDs (SF) after 5 years | 0.36 | – | – | 0 to 1 |
In addition to considering the impact of the full theoretical range of values associated with uncertain variables, it is important to consider this alongside the probability of actually observing these parameter values, based on the uncertainty characterised by the distributions these values are assigned in the PSA. Consequently, the probability of actually observing parameter values that would lead to an alternative strategy being most cost-effective and therefore a different adoption decision is shown in Table 19.
Variable | Strategy 1 | Strategy 2 | Strategy 3 |
---|---|---|---|
Short-term probabilities | |||
Probability of S+ after FDG-PET | 0 | 0 | 1 |
Probability of S– after FDG-PET, given not S+ | 0 | 0 | 1 |
Probability of S+ after iEEG | 0 | 0 | 1 |
Probability of permanent complications, iEEG | 0 | 0 | 1 |
Probability of transient complications, surgery | 0 | 0 | 1 |
Probability of permanent complications, surgery | 0 | 0 | 1 |
Probability of mortality after surgery | 0 | 0 | 1 |
Probability of mortality after iEEG | 0 | 0 | 1 |
TP of SF patients in the first year, MM | 0 | 0 | 1 |
TP of SF patients in the first year, surgery | 0 | 0 | 1 |
Long-term TPs | |||
TP from SF to DS after the first year, MM | 0 | 0 | 1 |
TP from SF to DS between first and fifth years, surgery | 0 | 0 | 1 |
TP from SF to DS after the fifth year, surgery | 0 | 0 | 1 |
TP from DS to SF between first and fifth years, MM | 0 | 0 | 1 |
TP from DS to SF after the fifth year, MM | 0 | 0 | 1 |
TP from DS to SF between first and fifth years, surgery | 0 | 0 | 1 |
TP from DS to SF after the fifth year, surgery | 0 | 0 | 1 |
Long-term mortality ratios (in relation to the general population) | |||
For patients in SF | 0 | 0 | 1 |
For patients in DS, MM | 0 | 0 | 1 |
For patients in DS, Surgery | 0 | 0 | 1 |
HRQoL and disutilities | |||
Baseline utility | 0 | 0 | 1 |
Disutility for SF in MM | 0 | 0 | 1 |
Disutility for SF in surgery, no complication | 0 | 0 | 1 |
Disutility for SF in surgery, permanent complication | 0 | 0 | 1 |
Disutility for SF in surgery, transient complication | 0 | 0 | 1 |
Disutility for DS in MM | 0.05 | 0.01 | 0.85 |
Disutility for DS in surgery, no complication | 0.05 | 0.02 | 0.93 |
Disutility for DS in surgery, permanent complication | 0 | 0 | 1 |
Disutility for DS in surgery, transient complication | 0 | 0 | 1 |
Other | |||
Proportion of patients off AEDs (SF) after 5 years | 0 | 0 | 1 |
Although there were many cases when theoretical alternative values of a single variable could change the conclusion of the base-case analysis, Table 19 demonstrates that only two parameters could change these conclusions based on their most likely range (i.e. according to the distributions of uncertainty used in the PSA analysis of the base-case model). These two parameters relate to the disutility of experiencing a DS in surgery (with no complications) or MM. However, even for these two parameters, there appears a very low probability that these would actually alter the conclusions based on the base-case analysis. For the remaining parameters, Strategy 3 remained the optimal strategy in terms of cost-effectiveness across the full range of values that were most likely for individual parameters. These results indicate that the uncertainty surrounding the optimal cost-effectiveness strategy appears minimal based on the assumptions used in the base-case analysis, reinforcing the conclusions based simply on the results from the CEACs.
Scenario analysis
The previous sections show that applying the inputs and assumptions in the base-case analysis, Strategy 3 appears the most cost-effective strategy and decision uncertainty is minimal. However, given the series of assumptions which were required within the base-case analysis, additional analyses were undertaken in order to assess the robustness of the results of the base-case model. These additional scenarios focused on several critical assumptions required to both link the separate sources (Uijl et al. 59 and Choi et al. 83) within the cost-effectiveness modelling framework and to populate the long-term model itself.
Inevitably the additional value of any visualisation strategy is closely related both to the impact on treatment decisions as well as the cost-effectiveness of the treatments themselves (MM or surgery). However, the results from the theoretical ranges for individual parameters considered in the base case indicated that the cost-effectiveness conclusions were insensitive to the short-term efficacy of surgery and MM (i.e. Strategy 3 appeared optimal irrespective of whether the short-term success of surgery in terms of the probability of being SF at 1 year was 0 or 1). This clearly demonstrates the importance of the additional benefits being assumed for surgery within the long-term model in driving the cost-effectiveness results. As previously noted these estimates were informed using a recently published study,83 which, although considered most appropriate to inform our long-term model, includes a number of assumptions, particularly in relation to the maintenance of additional long-term benefits for surgery compared with MM (i.e. over and above the impact that surgery has on the proportion of patients assumed to be SF at the end of the short-term model). As a result, it was considered important to explore the robustness of the base-case results to these additional long-term benefits using a separate scenario analysis.
Impact of the long-term benefits associated with surgery
The study by Choi et al. 83 assumes that TLE surgery confers two main advantages over MM: (1) a significantly higher proportion of patients become SF in the first year after surgery compared with MM and (2) surgery is also assumed to further reduce the long-term impact of epilepsy (avoiding relapses, increasing the likelihood of remission and improving mortality). As we were unable to critically appraise the full range of data sources used to inform these assumptions, we considered an alternative scenario in which the impact of surgery is only assumed to alter the proportion of patients who become SF in the first year. That is, the long-term transition probabilities, costs and HRQoL of patients conditional on becoming SF after the first year are assumed to be the same regardless of whether this was achieved via surgery or MM. Within this scenario, the additional benefits from surgery are thus constrained to increasing the probability of becoming SF within the first year.
Table 20 presents the results of this scenario alongside the results of the base-case analysis. The ICER estimates for Strategies 2 and 3 increase significantly compared with the base-case results. Although the ICER of Strategy 2 compared with Strategy 1 remain below conventional thresholds used to determine value for money in the NHS (£20,000–30,000 per QALY), the ICER of Strategy 3 compared with Strategy 2 now exceeds these thresholds. Consequently, the assumptions regarding the additional long-term benefits of surgery appear critical to determining which visualisation strategy appears optimal in terms of cost-effectiveness considerations. Although the use of FDG-PET still appears to be potentially cost-effective (ICER = £11,526 per QALY), the use of an additional test (iEEG) for patients in whom the decision to proceed to surgery is still uncertain following the results of FDG-PET no longer appears to be cost-effective. In other words, if the benefits of surgery do not extend beyond the probability of becoming SF within the short-term model, MM now appears to be the most appropriate management option for patients in whom the surgical decision following FDG-PET remains uncertain.
Strategy | Cost (£) | QALYs | ΔCost (£) | ΔQALYs | ICER (£) | Probability of being cost-effective at | |
---|---|---|---|---|---|---|---|
£20,000 | £30,000 | ||||||
Base-case analysis | |||||||
Strategy 1 | 23,775 | 12.88 | – | – | – | 0.14 | 0.13 |
Strategy 2 | 26,621 | 14.58 | 2846 | 1.70 | 1679 | 0.03 | 0.03 |
Strategy 3 | 27,696 | 14.91 | 1075 | 0.33 | 3227 | 0.83 | 0.84 |
Alternative scenario (no long-term benefits of surgery) | |||||||
Strategy 1 | 23,726 | 12.89 | – | – | N/A | 0.36 | 0.25 |
Strategy 2 | 27,207 | 13.19 | 3482 | 0.30 | 11,526 | 0.37 | 0.36 |
Strategy 3 | 28,416 | 13.23 | 1208 | 0.04 | 32,876 | 0.27 | 0.39 |
Figure 6 presents the probability that each strategy is cost-effective over a range of threshold values. When compared with the base-case analysis it is now evident that at conventional threshold values (of between £20,000 and £30,000) there is considerably more uncertainty surrounding the decision regarding which is the optimal strategy based on cost-effectiveness considerations in this alternative scenario.
FIGURE 6.
Cost-effectiveness acceptability curves for the alternative scenario.
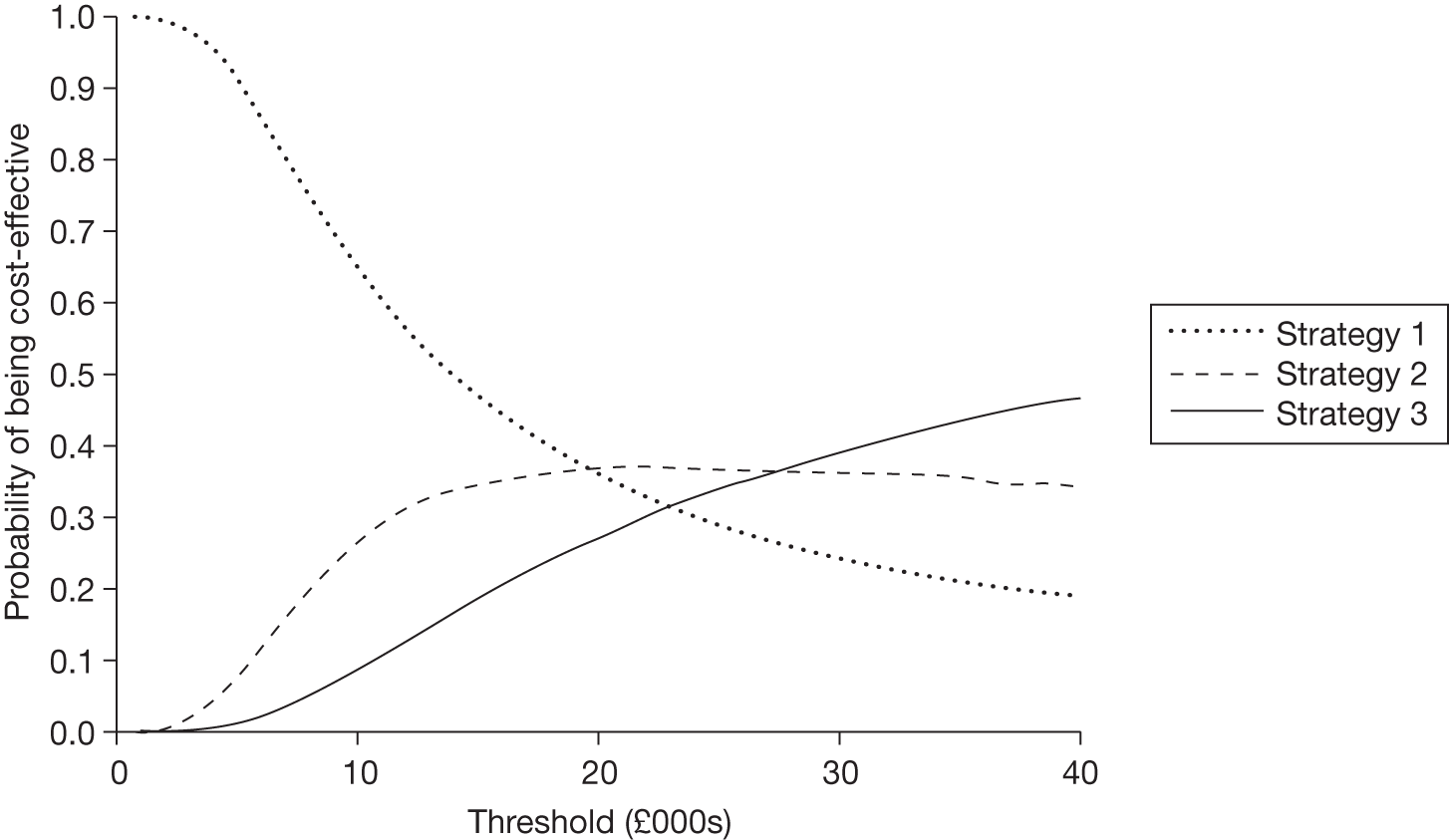
Given the higher uncertainty surrounding the cost-effectiveness results for this alternative scenario, we replicated the approaches to assessing the sensitivity to parameter uncertainty applied to the base-case analysis. Tables 21 and 22 are thus analogous to those reported for the base case and present the theoretical range of parameter values that will lead to the decision to adopt each strategy (see Table 21) and the probability of actually observing values leading to different adoption decisions (see Table 22).
Variable | Mean value | Strategy 1 | Strategy 2 | Strategy 3 |
---|---|---|---|---|
Short-term probabilities | ||||
Probability of S+ after FDG-PET | 0.56 | 0 to 0.05 | 0.05 to 1 | |
Probability of S– after FDG-PET, given not S+ | 0.68 | 0 to 1 | ||
Probability of S+ after iEEG | 0.83 | 0 to 1 | ||
Probability of permanent complications, iEEG | 0.04 | 0 to 1 | ||
Probability of transient complications, surgery | 0.08 | 0.25 to 1 | 0 to 0.25 | |
Probability of permanent complications, surgery | 0.04 | 0.15 to 1 | 0 to 0.15 | |
Probability of mortality after surgery | 0.003 | 0.05 to 1 | 0 to 0.05 | |
Probability of mortality after iEEG | 0.003 | 0 to 1 | ||
TP of SF patients in the first year, MM | 0.08 | 0.35 to 1 | 0 to 0.35 | |
TP of SF patients in the first year, surgery | 0.719 | 0 to 0.55 | 0.55 to 0.95 | 0.95 to 1 |
Long-term TPs | ||||
TP from SF to DS after the first year, MM | 0.254 | 0.35 to 1 | 0.15 to 0.35 | 0 to 0.15 |
TP from SF to DS between first and fifth years, surgery | 0.254 | 0 to 1 | ||
TP from SF to DS after the fifth year, surgery | 0.254 | 0 to 1 | ||
TP from DS to SF between first and fifth years, MM | 0.047 | 0.15 to 1 | 0 to 0.15 | |
TP from DS to SF after the fifth year, MM | 0.016 | 0 to 1 | ||
TP from DS to SF between first and fifth years, surgery | 0.047 | 0 to 1 | ||
TP from DS to SF after the fifth year, surgery | 0.016 | 0 to 1 | ||
Long-term mortality ratios (in relation to the general population) | ||||
For patients in SF | 1.11 | 0 to max. | ||
For patients in DS, MM | 5.64 | 0 to 1 | 1 to 15 | 15 to max. |
For patients in DS, surgery | 5.64 | 0 to max. | ||
HRQoL and disutilities | ||||
Baseline utility | 0.91 | 0.05 to 1 | 0 to 0.05 | |
Disutility for SF in MM | 0 | Min. to –0.1 | –0.1 to 0.1 | 0.1 to 1 |
Disutility for SF in surgery, no complication | 0 | Min. to 1 | ||
Disutility for SF in surgery, permanent complication | –0.2 | Min. to 0 | ||
Disutility for SF in surgery, transient complication | –0.01 | Min. to 0 | ||
Disutility for DS in MM | –0.21 | –0.15 to 0 | –0.25 to –0.15 | Min. to –0.25 |
Disutility for DS in surgery, no complication | –0.19 | Min. to 0 | ||
Disutility for DS in surgery, permanent complication | –0.31 | Min. to –0.65 | –0.65 to 0 | |
Disutility for DS in surgery, transient complication | –0.22 | Min. to 0 | ||
Other | ||||
Proportion of patients off AEDs (SF) after 5 years | 0.36 | Min. to 0 |
Variable | Strategy 1 | Strategy 2 | Strategy 3 |
---|---|---|---|
Short-term probabilities | |||
Probability of S+ after FDG–PET | – | 1 | – |
Probability of S– after FDG–PET, given not S+ | – | 1 | – |
Probability of S+ after iEEG | – | 1 | – |
Probability of permanent complications, iEEG | – | 1 | – |
Probability of transient complications, surgery | – | 1 | – |
Probability of permanent complications, surgery | – | 1 | – |
Probability of mortality after surgery | – | 1 | – |
Probability of mortality after iEEG | – | 1 | – |
TP of SF patients in the first year, MM | – | 1 | – |
TP of SF patients in the first year, surgery | – | 1 | – |
Long-term TPs | |||
TP from SF to DS after the first year, MM | 0.19 | 0.67 | 0.14 |
TP from SF to DS between first and fifth years, surgery | – | 1 | – |
TP from SF to DS after the fifth year, surgery | – | 1 | – |
TP from DS to SF between first and fifth years, MM | – | 1 | – |
TP from DS to SF after the fifth year, MM | – | 1 | – |
TP from DS to SF between first and fifth years, surgery | – | 1 | – |
TP from DS to SF after the fifth year, surgery | – | 1 | – |
Long-term mortality ratios (in relation to the general population) | |||
For patients in SF | – | 1 | – |
For patients in DS, MM | – | 1 | – |
For patients in DS, surgery | – | 1 | – |
HRQoL and disutilities | |||
Baseline utility | – | 1 | – |
Disutility for SF in MM | – | 1 | – |
Disutility for SF in surgery, no complication | – | 1 | – |
Disutility for SF in surgery, permanent complication | – | 1 | – |
Disutility for SF in surgery, transient complication | – | 1 | – |
Disutility for DS in MM | 0.33 | 0.43 | 0.24 |
Disutility for DS in surgery, no complication | – | 1 | – |
Disutility for DS in surgery, permanent complication | 0.05 | 0.95 | – |
Disutility for DS in surgery, transient complication | – | 1 | – |
Other | |||
Proportion of patients off AEDs (SF) after 5 years | – | 1 | – |
The results of the analyses of parameter uncertainty highlight that removal of the additional longer-term benefits of surgery greatly increases the sensitivity of the model to parameter uncertainty. Despite this greater sensitivity of the model, when the probability of actually observing parameter values that would results in changes to the optimal strategy were considered, in the majority of cases changes in the parameter values do not appear to alter the conclusion that Strategy 2 now appears to be the most cost-effective strategy.
Table 21 shows that more parameters within their theoretical range now have an influence in the adoption decision and that, for most of these, the parameter values in which this shift occurs are closer to the mean. Perhaps of most note from these results is the finding that the cost-effectiveness results are now sensitive to the initial success rates of surgery in terms of the probability of patients becoming SF at 1-year (see ‘TP of SF patients in the first year, surgery’ in Table 21). If the success rate of surgery is < 55% then Strategy 1 would appear to be the optimal strategy. Strategy 2 appears optimal for success rates between 55% and 95% and Strategy 3 would only become optimal if the success rate of surgery was > 95%. This finding contrasts with the base-case analysis, which demonstrated that the optimal strategy (Strategy 3) was insensitive to the success rate when the range of additional long-term benefits was assumed for patients who received surgery.
Despite the greater sensitivity of the model to theoretical changes in individual parameters, when the probability of actually observing parameter values that would result in changes to the optimal strategy was considered, in the majority of cases changes in the parameter values do not appear to alter the conclusion that Strategy 2 now appears to be the most cost-effective strategy.
As previously stated, it was assumed in both the base-case analysis and alternative scenario that the compliance to iEEG and surgery was assumed to be 100%. We also explored the robustness of the cost-effectiveness results to a range of alternative estimates. Our results indicated that the conclusions from the base case and alternative scenario would not be altered unless the compliance to iEEG and surgery was < 20%. During the clinical effectiveness review any papers that recorded compliance to surgery and/or to iEEG were recorded, and the relevant compliance values were extracted. Thirteen papers reported compliance: all had sufficient data on compliance to surgery but only four on compliance to iEEG. 42,49,55–58,61–63,65,66,68,69 The compliance rates for surgery ranged from 63% to 100% and for iEEG ranged from 89% to 100%. We therefore considered that the conclusions from the base case and alternative scenario were robust to this assumption.
Discussion
The results of the base-case analysis suggested that the use of FDG-PET for those patients in whom the decision to proceed to surgery was unclear following video-EEG and routine MRI, followed by iEEG for patients with indeterminate FDG-PET results (i.e. Strategy 3) appeared the most cost-effective strategy at conventional cost-effectiveness thresholds of £20,000–30,000. These conclusions were sensitive to the additional benefits that were assumed to be conferred over the longer-term for patients who received surgery compared with MM alone (i.e. the benefits over and above those attributed to the additional success rate of surgery in increasing the probability of patients becoming SF at 1 year). When these additional benefits were excluded, the use of FDG-PET remained cost-effective; however, MM, as opposed to the use of iEEG, now appeared to be the more appropriate management strategy for patients in whom the decision to proceed to surgery was still unclear following the results of FDG-PET.
We consider that these initial results are important in several respects. First, they provide a provisional indication that some form of non-invasive testing (at least with FDG-PET) appears cost-effective. Second, our analysis demonstrates that it is feasible to assess cost-effectiveness based on appropriately designed clinical effectiveness studies. Thirdly, the model structure highlights the range of additional inputs and assumptions required in estimating cost-effectiveness appropriately and the analysis presented here could now serve to provide both a framework and set of inputs/results that could be revised and updated as new evidence emerges. Finally, the model demonstrates that the value of the visualisation strategies is inextricably linked both to their impact on the decision to proceed to surgery or not and the cost-effectiveness of the subsequent treatments themselves. Our results indicate that the long-term effectiveness and cost-effectiveness of surgery is likely to be an important driver of the cost-effectiveness of alternative visualisation strategies and possible sequences. As such, the number of non-invasive tests used as part of a possible sequence will clearly depend on the long-term maintenance of benefits with surgery. Our provisional findings have indicated that the cost-effectiveness of one possible sequence of an additional non-invasive test (after video-EEG and routine MRI) followed by a further invasive test for patients in whom the decision is still unclear, is sensitive to the potential longer-term benefits for patients receiving surgery beyond the impact on the initial success rate in terms of patients becoming SF.
An important limitation of our work, as we noted from the outset, is that these findings need to be considered in light of the limited clinical data identified from the clinical effectiveness review and the assumptions required to link these data to long-term consequences (costs and outcomes) suitable for informing cost-effectiveness analysis. It was not considered feasible to assess the full range of potential strategies that would be required in order to appropriately inform NHS practice concerning the optimal use of the range of existing visualisation strategies that are available. As such, it is not possible to conclude that FDG-PET is more or less cost-effective than other non-invasive testing strategies or compared with a sequence of non-invasive tests until such time that additional clinical studies are undertaken using appropriate methods suitable for informing these assessments.
Chapter 5 Discussion
Diagnostic test evaluation is a challenging area with limited available data. A review of the literature in 2009 identified 19 similar hierarchical frameworks for the phased evaluation of diagnostic tests;32 the most comprehensive was the six-level framework suggested by Fryback and Thornbury. 31 An alternative framework identified six criteria for a study to evaluate whether or not a new technology provides value for money and to enable implementation of the new technology in a timely fashion. 24
Our review of the available evidence for diagnostic technologies for the localisation of the focus during the work-up for epilepsy surgery has made clear that most of the evidence currently available is at the level of diagnostic accuracy (level 2), with few studies available at subsequent levels. Where evidence is available at level 4 or 5, it tends to be in restricted populations and therefore subject to selection bias (i.e. outcomes being reported only in patients who underwent surgery). Importantly, the diagnostic accuracy studies are of limited value given the lack of any recognised gold standard. Our review attempted to circumvent the problem of a lack of an acceptable reference standard by restricting the inclusion of diagnostic accuracy studies to those using the decision to go to surgery and the outcome following surgery, so providing some elements of clinical decision-making and patient outcome. However, even these provided extremely limited evidence and did not inform the clinical utility of the diagnostic tests.
The clinical utility of medical tests is measured by whether or not the information they provide affects patient-relevant outcomes; a medical test may directly affect patient outcomes, but the effect is usually via influencing downstream treatment choices. 35 Studies need to investigate the clinical value of performing a test, and the impact of the results of that test on the decision-making process and subsequently on clinical outcomes. However, the link between test performance and clinical outcomes is complex: good test performance does not guarantee that a chosen intervention will be effective, that physicians will act on the results or that patients will comply with the recommended management strategy. 35 Thus, the evaluation of these imaging technologies is dependent on studies at levels 4 and 5 of the framework, i.e. those that investigate the impact of the results of imaging technologies on treatment decisions made by clinicians, and patient outcomes and QoL.
Four studies were identified that evaluated the use of one of the index tests of interest on the decision-making process;59,72–74 unfortunately, only one passed the inclusion criteria for the review. 59 The study by Uijl et al. 59 was a well-conducted retrospective review of the practices of a multidisciplinary epilepsy team, and the decision-making process during the work-up for epilepsy surgery. 59 There were some limitations of this study, such as the potential technological advancements since the assessment of the participants in the study from 1996 to 2002, limiting its generalisability to current practice; the large number of patients who did not undergo FDG-PET (359/469 patients) and therefore the potential for selection bias; the conducted of additional tests after FDG-PET in some patients prior to surgery, which could have influenced the site of surgery; and only the outcome following surgery being reported for those achieving Engel Ia (SF without auras). 59
Following the insights provided regarding the appropriate evaluation of clinical effectiveness, a framework was also developed to inform evaluations of the cost-effectiveness of alternative imaging strategies in people with medically refractory epilepsy who are being considered for surgery. This consisted of the development of a decision-analytic model that linked the added value of a test with subsequent treatment decisions (surgery or MM) and health outcomes after treatment. Also, different aspects of patients’ progression were considered, such as costs and HRQoL, allowing the overall clinical benefits and costs associated with alternative interventions to be appraised.
We have demonstrated this framework to be feasible by applying it using the sparse evidence currently available. However, because of this paucity of evidence, conclusions on cost-effectiveness are limited given that we have restricted the decision problem by incorporating only the small set of alternative imaging strategies (no further invasive tests, FDG-PET and iEEG) for which appropriate evidence existed and also by restricting the population to patients with temporal lobe epilepsy that have already undergone a video-EEG and a MRI, which have resulted in an indeterminate result (i.e. the decision to proceed to surgery is uncertain). Rather than providing a complete picture of the cost-effectiveness of the full range of alternative strategies, the development of the model thus intended to illustrate how data from appropriately designed clinical studies can be used to inform a decision model. Also, an analysis of the impact of uncertainty in the existing evidence was undertaken, highlighting which information could be important to be collected in order to inform such a decision-analytical model, placing particular emphasis on the impact of the assumptions related to the longer-term benefits of surgery.
The decision-analytical model developed in this report could be extended to accommodate new data as it becomes available. The model could also be adapted to evaluate additional alternative treatment strategies consisting of either single tests or combination/sequence of tests, i.e. evaluating more comparators and expanding the existing tree ‘vertically’. How this is done will impact on the type of data required. Also, clinical practice may suggest that additional subsequent tests should be undertaken following a non-definite decision to undertake surgery (S?), i.e. strategies may be more complex, involving a larger number of tests used sequentially. Data acquisition to inform such a ‘horizontal’ extension of the decision tree would probably be complex, but the model would be flexible to accommodate these.
The analyses of the clinical effectiveness and cost-effectiveness evidence also highlighted that there is a paucity of relevant evidence and that future research needs to be considered more closely in relation to how the research informs the different levels of the diagnostic evaluation framework and ultimately how this links to the actual decision problem(s) faced by clinicians and the NHS. It is integral to the evaluation of imaging technologies in the work-up for epilepsy surgery that the impact of the use of these technologies on clinical decision-making, and on further treatment decisions, is considered. We also advocate that it is important that the findings of these studies are used alongside assessments of the long-term consequences (both in terms of clinical effects and of costs) of such treatments. The role of decision modelling is central to this, ultimately helping the NHS making informed decisions over the appropriate use of imaging technologies in this context.
Options for future research
The prior HTA highlighted limitations of the data available at that time, which comprised mainly diagnostic accuracy, and that these types of studies will not provide clinical effectiveness or cost-effectiveness information. 36 The report recommended a large-scale, cross-sectional diagnostic study of patients in whom epilepsy surgery may be indicated with consensus decisions made by an expert, multidisciplinary panel as to whether or not surgery is to be conducted, and that patients rejected from surgery are also measured in studies of effectiveness. It also recommended that clinicians, patient groups, policy-makers and funders of health care and/or research meet and debate the feasibility and ethical acceptability of RCTs to assess effectiveness and cost-effectiveness; the individual tests or combinations of tests that should be examined; patient populations that should be recruited; and the most appropriate reference standard and populations to study to identify which tests could be evaluated in diagnostic accuracy studies in order to inform which tests are worthwhile evaluating with a RCT.
Several authors have suggested RCTs for evaluating diagnostic tests,22,24,36,90 especially where there is no evidence of a link between diagnostic test results and evidence of treatment efficacy,90 and so providing evidence at level 5 of Fryback and Thornbury’s framework for diagnostic evaluation. 31 There have been a number of suggestions as to the conduct of such RCTs, with randomisation prior to testing with the new or old strategy24 or after the conduct of the test to relevant management strategies. 24,90,91 However, the conduct of a RCT to evaluate tests identifying the seizure focus in work-up for epilepsy surgery may be considered impractical for a number of reasons: the inability to match populations of very heterogeneous neocortical epilepsies; small study populations; large variation in clinical practice; the integral link between practitioners’ experience and skill and the interpretation of results; the rapid advancements in the technology and the duration of follow-up required (by the completion of the trial, the results may not be applicable to current clinical practice); ethical considerations; the new diagnostic strategy is far safer and/or less burdensome to the patient than the currently used diagnostic test; or cost. 22,24,35,92,93 In addition, RCTs evaluating long-term quality-of-life and survival measures will have to be large, as the differences in these measures across diagnostic strategies may be small. 24
Ethical issues are likely to be a key consideration in this area of research if planning a RCT. First, despite the lack of evidence there may well be concerns over randomising patients to diagnostic regimens that omit a potentially beneficial test, and the consequences this may have. The issue of regret experienced by clinicians related to the choices they made and whether there is a level of ‘acceptable’ regret has been investigated. 94,95 Despite these concerns, a RCT has been performed in this area that randomised patients with mesial TLE to a diagnostic regimen with or without ictal SPECT. 74 This RCT did not pass the inclusion criteria for this review as it included a large number of participants (approximately 43%) who had precipitating trauma; the RCT concluded that there was no benefit of SPECT beyond that of video-EEG and structural MRI in terms of surgical decision and outcome in this population. 74 Second, given the treatment options being considered (MM vs excisional surgery), randomisation post test to different treatment strategies would be unacceptable. This latter point has significant implications for the design of studies evaluating the long-term benefits of surgery for epilepsy.
Where a RCT is not practicable or considered warranted, bias can be reduced by conducting a direct comparison where all patients are given both diagnostic technologies using observational study methodology. 93 These data will be informative as long as the necessary information is gathered, and patients are selected based on their clinical problem, so representing clinical practice. 34 When considering a study design that uses a consensus-based diagnosis by a multidisciplinary team, a number of factors that affect reliability need to be considered, namely the number of experts and expertise mix; the way patient information is presented; and how to obtain a final classification. 54 Additionally, there remains the problem of how to verify the accuracy/appropriateness of the decision not to go to surgery, and the accuracy of discordant tests where the outcome following surgery was poor; clinical outcomes for all patients regarding the final management strategy, and results after any re-operation, would need to be reported.
A potential starting point for informative studies of imaging technologies could be routine care databases or electronic patient records, which commonly include all information related to patient care. 96 Routinely documented data can quantify the value of a test additional to other information, and covers the entire diagnostic and therapeutic process allowing stepwise analysis of tests in the sequence as they occurred in routine practice and an estimate of the added value of tests to previous findings. 96 Data collection could be optimised through the use of a national registry, where the results of the tests and the decisions made based on these were entered using a standardised format. This would be a prospective registry, although patients who were considered to have been evaluated using appropriate technology could be entered retrospectively. A national database would allow trends in clinical practice to be observed, and interventions and populations that would benefit from further investigation identified, as well as providing data for any future decision-analytical modelling.
Given the potential problems with conducting RCTs, and the apparent need for a range of methods to collect the varied clinical information required to address the clinical question, decision modelling will be an important analytical method, allowing estimates based on alternative study data such as that obtained from the alternatives described above and expert opinion to be included in the synthesis, and can simulate the impact of various diagnostic strategies including which combinations of tests is optimal on patient outcomes. 24,35,92 Modelling can also be used to investigate evidentiary assumptions about test performance and clinician decision-making, and examine all benefits, harms and costs together to provide cost-effectiveness and cost–utility information to decision-makers. 24,92 The major limitation of decision modelling is insufficient reliable data relating to key components of the model. 35 The reliability of the data available can depend on the clinical context in which the test is conducted (sole diagnostic modality, triage, further work-up, confirmatory test). 35,97 It may not be appropriate to compare data from a test used as the sole diagnostic tool, with alternative diagnostic strategies deriving data from a confirmatory test after a positive or inconclusive prior test. 35 As well as data on the influence of test results on consensus diagnosis and resultant management decision, clinical outcomes in patients who do not undergo surgery, compliance with tests and surgery, QoL, and complication and re-operation rates are important.
The development and population of the decision-analytical model identified that assumptions regarding the effects of surgery and MM in reducing the long-term impact of epilepsy – for example, avoiding relapses and/or increasing the likelihood of remission and/or improving mortality – may substantially impact on the evaluation of the clinical effectiveness of the imaging technologies. Further research should also be prioritised to help quantify the magnitude and precision of these effects.
Chapter 6 Conclusions
Clinical research into imaging for the localisation of epileptic foci is abundant but not adequately informative. The many studies do not comprise a strong evidence base because:
-
There is currently no acceptable reference standard for the assessment of the diagnostic accuracy of tests to identify a seizure focus in patients with refractory epilepsy.
-
Diagnostic accuracy studies that provide information on clinical outcomes tend to do so only following surgery, and are therefore subject to selection bias.
-
The outcome prediction studies identified are also subject to selection bias, being based on only those patients who have undergone surgery, and are further limited by inadequate sample sizes.
-
Decision level and effectiveness studies are lacking.
Given that diagnostic accuracy studies cannot provide information relating to the clinical utility of the tests being evaluated, future studies of these diagnostic technologies should concentrate on their impact on the decision-making process, therapeutic management decisions and patient outcomes.
Inevitably, the additional value of any imaging strategy for the localisation of epileptic foci is closely related to the impact on treatment decisions as well as to the value of the treatments themselves (MM or surgery). This needs to be considered fully in informing cost-effectiveness assessments in this context, further used to help the NHS to make informed decisions over the appropriate use of imaging technologies.
Implications for service provision
With the currently available data, we are unable to inform clinical practice in terms of which non-invasive test, or combination/sequence of tests is most clinically or cost-effective for the work-up of patients with refractory epilepsy who are being considered for surgery. The review has demonstrated that at least one non-invasive test (FDG-PET) is cost-effective in those patients for whom there was uncertainty regarding their eligibility for surgery after the initial EEG/MRI. This highlights the potential for the use of these tests and the need for further evaluation.
Suggested research priorities
The research priorities are:
-
Clinicians, patient groups, policy-makers, and health-care/research funders need to meet and debate the most appropriate way to conduct primary research investigating these technologies for the localisation of the epileptic focus in people with refractory epilepsy being considered for surgery, with a view to undertaking decision-analytic modelling.
-
A national registry should be initiated that collects standardised information regarding the diagnostic pathway, decisions made along the pathway, and clinical outcomes, for all patients who receive work-up to determine whether they are eligible for epilepsy surgery.
-
Appropriately designed studies should be conducted that help determine the added value of tests, or combinations/sequences of tests, in terms of informing decisions on the appropriateness of surgery and in terms of clinical effectiveness and cost-effectiveness.
Existing and future research needs to be considered closely in relation to how the research informs the different levels of the diagnostic evaluation framework and ultimately how this links to the actual decision problem(s) faced by clinicians and the NHS; the role of decision modelling is central to this more general issue.
Acknowledgements
We would like to thank Professor Mark Richardson, Kings College London, for his clinical advice both during the development of the protocol and the conduct of the review. We would also like to thank Dr Robert Phillips, Centre for Reviews and Dissemination (CRD), York, for his advice during the data extraction process and interpretation of the diagnostic accuracy data. The views expressed in this report are those of the authors and not necessarily those of the NIHR HTA Programme. Any errors are the responsibility of the authors.
Contribution of authors
Dr Jane Burch Reviewer responsible for study selection, data extraction, validity assessment, data analysis and writing of the report.
Sebastian Hinde Contributed to the review of cost-effectiveness evidence, development of the economic model and writing of the report.
Professor Stephen Palmer Contributed to all aspects of the economic sections and provided comments on drafts of the report.
Fiona Beyer Devised the search strategy, carried out the literature searches and wrote the search methodology sections of the report.
Dr Jon Minton Assisted in study selection, data extraction, validity assessment, data analysis and writing the report.
Dr Anthony Marson Provided clinical advice throughout the review and comments on drafts of the protocol and report.
Dr Udo Wieshmann Provided clinical advice throughout the review and comments on drafts of the protocol and report.
Dr Nerys Woolacott Contributed to all aspects of the clinical review and provided comments on drafts of the report.
Marta Soares Managed and contributed to all aspects of the economic sections and writing of the report.
Disclaimers
The views expressed in this publication are those of the authors and not necessarily those of the HTA programme or the Department of Health.
References
- Elger CE, Schmidt D. Modern management of epilepsy: a practical approach. Epilepsy Behav 2008;12:501-39.
- Epilepsy Foundation n.d. www.epilepsyfoundation.org.
- Philips Z, Ginnelly L, Sculpher M, Claxton K, Golder S, Riemsma R, et al. Review of guidelines for good practice in decision-analytic modelling in health technology assessment. Health Technology Assess 2004;8.
- Pugliatti M, Beghi E, Forsgren L, Ekman M, Sobocki P. Estimating the cost of epilepsy in Europe: a review with economic modeling. Epilepsia 2007;48:2224-33.
- Tidman L, Saravanan K, Gibbs J. Epilepsy in mainstream and special educational primary school settings. Seizure 2003;12:47-51.
- National Society for Epilepsy n.d. www.epilepsysociety.org.uk/Homepage (accessed 15 January 2010).
- HESonline . NHS Information Centre n.d. www.hesonline.nhs.uk/Ease/servlet/ContentServer?siteID = 1937.
- NHS Choices . Epilepsy n.d. www.nhs.uk/epilepsy (accessed 19 January 2010).
- Jacoby A, Buck D, Baker G, McNamee P, Graham-Jones TS, Chadwick D. Uptake and costs of care for epilepsy: findings from a UK regional study. Epilepsia 1998;39:776-86.
- Jobst BC. Treatment algorithms in refractory partial epilepsy. Epilepsia 2009;50:51-6.
- Cramer J, Menachem E, French J. Review of treatment options for refractory epilepsy: new medications and vagal nerve stimulation. Epilepsy Res 2001;47:17-25.
- Wiebe S, Blume WT, Irvin JPG, Eliasziw M. A randomized controlled trial of surgery for temporal lobe epilepsy. N Engl J Med 2001;345:311-18.
- Lhatoo SD, Solomon JK, McEvoy AW, Kitchen ND, Shorvon SD, Sander JW. A prospective study of the requirement for and the provision of epilepsy surgery in the United Kingdom. Epilepsia 2003;44:673-6.
- Epilepsy Action . Anti-Epileptic Medication Available in the United Kingdom 2009. www.epilepsy.org.uk/info/drugslist.html (accessed 15 January 2010).
- Epilepsy Action n.d. www.epilepsy.org.uk (accessed 20 January 2010).
- Langfitt JT, Holloway RG, McDermott MP, Messing S, Sarosky K, Berg AT, et al. Health care costs decline after successful epilepsy surgery. Neurology 2007;68:1290-8.
- Zijlmans M, Huiskamp G, Hersevoort M, Seppenwoolde J, Van HA, Leijten F. Added value of EEG-fMRI in the pre-operative workup for epilepsy surgery. Epilepsia 2007;48.
- Thornton R, Laufs H, Rodionov R, Salek–Haddadi A, Carmichael D, Walker M, et al. Correlation of pre-surgical EEG fMRI and post-surgical imaging and outcome in patients with focal epilepsy. Epilepsia 2007;48.
- Seeck M, Lazeyras F, Michel CM, Blanke O, Gericke CA, Ives J, et al. Non-invasive epileptic focus localization using EEG-triggered functional MRI and electromagnetic tomography. Electroencephalogr Clin Neurophysiol 1998;106:508-12.
- Richardson M. Update on neuroimaging in epilepsy. Expert Rev Neurother 2010;10:961-73.
- Richardson M. Current themes in neuroimaging of epilepsy: brain networks, dynamic phenomena, and clinical relevance. Clin Neurophysiol 2010;121:1153-75.
- Stefan H. Magnetoencephalography adds to the surgical evaluation process. Epilepsy Behav 2011;20:172-7.
- Burneo JG, Steven DA, McLachlan RS, Parrent AG. Morbidity associated with the use of intracranial electrodes for epilepsy surgery. Can J Neurol Sci 2006;33:223-7.
- Hunink MGM, Krestin GP. Study design for concurrent development, assessment, and implementation of new diagnostic imaging technology. Radiology 2002;222:604-14.
- Webb J, Guimond A, Eldridge P. Automatic detection of hippocampal atrophy on magnetic resonance images. Magn Reson Imaging 1999;17:1149-61.
- Roberts N, Puddephat MJ, McNulty V. The benefit of stereology for quantitative radiology. Br J Radiol 2000;73:679-97.
- Holmes MD. Dense array EEG: methodology and new hypothesis on epilepsy syndromes. Epilepsia 2008;49:3-14.
- Department of Health . Reference Costs 2007 8 (dh 098948) n.d. www.dh.gov.uk/en/Publicationsandstatistics/Publications/PublicationsPolicyAndGuidance/DH_082571.
- Hugg JW, Butterworth EJ, Martin RC, Hetherington HP, Chu W-J, Gilliam FG, et al. Comparative localization of temporal lobe epilepsy by 1H-MRSI T2 and T1 relaxometry, and volumetry. Epilepsia 1998;39.
- Hoo KS, Francisco S, Knowlton RC, Wong ST, Laxer KD, Hawkins RA. Relative accuracy of MR imaging hippocampal volumetry, T2 relaxometry, 1H-MRSI, and FDG-PET in lateralizing temporal lobe epilepsy. Radiology 1997;205.
- Fryback DG, Thornbury JR. The efficacy of diagnostic imaging. Med Decis Making 1991;11:88-94.
- Lijmer J, Leeflang M, Bossuyt P. Proposals for a phased evaluation of medical tests. Med Decis Making 2009;29:E13-21.
- Moons KGM, Biesheuvel CJ, Grobbee DE. Test research versus diagnostic research. Clin Chem 2004;50:473-6.
- Moons KG, van Es GA, Michel BC, Buller HR, Habbema JD, Grobbee DE. Redundancy of single diagnostic test evaluation. Epidemiology 1999;10:276-81.
- Trikalinos T, Siebert U, Lau J. Decision-analytic modelling to evaluate benefits and harms of medical tests: uses and limitations. Med Decis Making 2008;29:E22-9.
- Whiting P, Gupta R, Burch J, Mota REM, Wright K, Marson A, et al. A systematic review of the effectiveness and cost-effectiveness of neuroimaging assessments used to visualise the seizure focus in people with refractory epilepsy being considered for surgery. Health Technol Assess 2006;10.
- Moons KGM, Grobbee DE. Diagnostic studies as multivariable, prediction research. J Epidemiol Community Health 2002;56:337-8.
- Uijl SG, Leijten FSS, Parra J, Arends JBAM, van Huffelen AC, Moons KGM. What is the current evidence on decision-making after referral for temporal lobe epilepsy surgery? A review of the literature. Seizure 2005;14:534-40.
- Centre for Reviews and Dissemination . Systematic Reviews: CRD’s Guidance for Undertaking Reviews in Health Care 2009.
- PRISMA Group . PRISMA Statement Website n.d. www.prisma-statement.org (accessed 16 March 2011).
- Liberati A, Altman DG, Tetzlaff J, Mulrow C, Gøtzsche PC, Ioannidis JPA, et al. The PRISMA statement for reporting systematic reviews and meta-analyses of studies that evaluate healthcare interventions: explanation and elaboration. BMJ 2009;339.
- Wheless JW, Willmore LJ, Breier JI, Kataki M, Smith JR, King DW, et al. A comparison of magnetoencephalography, MRI, and V-EEG in patients evaluated for epilepsy surgery. Epilepsia 1999;40:931-41.
- Whiting P, Rutjes A, Reitsma J, Bossuyt P, Kleijnen J. The development of QUADAS: a tool for the quality assessment of studies of diagnostic accuracy included in systematic reviews. BMC Med Res Methodol 2003;3.
- Wells GA, Shea B, O’Connell D, Peterson J, Welch V, Losos M. Newcastle–Ottawa Scale (NOS) for Assessing the Quality If Nonrandomized Studies in Meta-Analyses n.d. www.ohri.ca/programs/clinical_epidemiology/oxford.asp (accessed 29 July 2011).
- Downs SH, Black N. The feasibility of creating a checklist for the assessment of the methodological quality both of randomised and non-randomised studies of health care interventions. J Epidemiol Community Health 1998;52:377-84.
- Graham B. Diagnosis, diagnostic criteria, and consensus. Hand Clin 2009;25:43-8.
- Reitsma JB, Rutjes AWS, Khan KS, Coomarasamy A, Bossuyt PM. A review of solutions for diagnostic accuracy studies with an imperfect or missing reference standard. J Clin Epidemiol 2009;62:797-806.
- Alving J, Beniczky S. Diagnostic usefulness and duration of the inpatient long-term video-EEG monitoring: findings in patients extensively investigated before the monitoring. Seizure 2009;18:470-3.
- Knowlton RC, Elgavish RA, Bartolucci A, Ojha B, Limdi N, Blount J, et al. Functional imaging: II. Prediction of epilepsy surgery outcome. Ann Neurol 2008;64:35-41.
- Duncan J. The current status of neuroimaging for epilepsy. Curr Opin Neurol 2009;22:179-84.
- Knowlton RC, Elgavish RA, Limdi N, Bartolucci A, Ojha B, Blount J, et al. Functional imaging: I. Relative predictive value of intracranial electroencephalography. Ann Neurol 2008;64:25-34.
- Warner J, Najarian R, Tierney LJ. Perspective: uses and misuses of thresholds in diagnostic decision making. Clin Decis Making 2010;85:556-63.
- Sadatsafavi M, Moayyeri A, Bahrami H, Soltani A. The value of Bayes theorem in the interpretation of subjective diagnostic findings: what can we learn from agreement studies?. Med Decis Making 2011;27:735-43.
- Rutjes A, Reitsma J, Coomarasamy A, Khan K, Bossuyt P. Evaluation of diagnostic tests when there is no gold standard. A review of methods. Health Technol Assess 2007;11.
- Achten E, Santens P, Boon P, De Coo D, Van De Kerckhove T, De Reuck J, et al. Single-voxel proton MR spectroscopy and positron emission tomography for lateralization of refractory temporal lobe epilepsy. Am J Neuroradiol 1998;19:1-8.
- Knowlton RC, Laxer KD, Ende G, Hawkins RA, Wong STC, Matson GB, et al. Presurgical multimodality neuroimaging in electroencephalographic lateralized temporal lobe epilepsy. Ann Neurol 1997;42:829-37.
- Lee DS, Lee SK, Kim YK, Kang E, Lee JS, Chung JK, et al. The usefulness of repeated ictal SPET for the localization of epileptogenic zones in intractable epilepsy. Eur J Nucl Med Mol Imaging 2002;29:607-14.
- Didelot A, Ryvlin P, Lothe A, Merlet I, Hammers A, Mauguiere F. PET imaging of brain 5-HT1A receptors in the preoperative evaluation of temporal lobe epilepsy. Brain 2008;131:2751-64.
- Uijl SG, Leijten FSS, Arends JBAM, Parra J, Van Huffelen AC, Moons KGM. The added value of [18F]-fluoro-d-deoxyglucose positron emission tomography in screening for temporal lobe epilepsy surgery. Epilepsia 2007;48:2121-9.
- Antel SB, Li LM, Cendes F, Collins DL, Kearney RE, Shinghal R, et al. Predicting surgical outcome in temporal lobe epilepsy patients using MRI and MRSI. Neurology 2002;58:1505-12.
- Lewis DH, Ory P, Holmes MD, Wilensky AJ, Cohen WA, Schneider J, et al. High-resolution inter-ictal SPECT and phased-array MRI in partial epilepsy: an imaging comparison with video/EEG and outcome correlation. Nucl Med Commun 1998;19:199-206.
- O’Brien TJ, Hicks RJ, Ware R, Binns DS, Murphy M, Cook MJ. The utility of a 3-dimensional, large-field-of-view, sodium iodide crystal-based PET scanner in the presurgical evaluation of partial epilepsy. J Nucl Med 2001;42:1158-65.
- Assaf BA, Karkar KM, Laxer KD, Garcia PA, Austin EJ, Barbaro NM, et al. Magnetoencephalography source localization and surgical outcome in temporal lobe epilepsy. Clin Neurophysiol 2004;115:2066-76.
- Kaiboriboon K, Nagarajan S, Mantle M, Kirsch HE. Interictal MEG/MSI in intractable mesial temporal lobe epilepsy: spike yield and characterization. Clin Neurophysiol 2010;121:325-31.
- Lee SK, Kim DW, Kim KK, Chung CK, Song IC, Chang KH. Effect of seizure on hippocampus in mesial temporal lobe epilepsy and neocortical epilepsy: an MRS study. Neuroradiology 2005;47:916-23.
- Wu JY, Salamon N, Kirsch HE, Mantle MM, Nagarajan SS, Kurelowech L, et al. Noninvasive testing, early surgery, and seizure freedom in tuberous sclerosis complex. Neurology 2010;74:392-8.
- O’Brien TJ, So EL, Mullan BP, Hauser MF, Brinkmann BH, Jack CR, et al. Subtraction SPECT co-registered to MRI improves postictal SPECT localization of seizure loci. Neurology 1999;52:137-46.
- Theodore WH, Sato S, Kufta CV, Gaillard WD, Kelley K. FDG-positron emission tomography and invasive EEG, seizure focus detection and surgical outcome. Epilepsia 1997;38:81-6.
- Smith JR, Schwartz BJ, Gallen C, Orrison W, Lewine J, Murro AM, et al. Utilization of multichannel magnetoencephalography in the guidance of ablative seizure surgery. J Epilepsy 1995;8:119-30.
- Dupont S, Semah F, Clemenceau S, Adam C, Baulac M, Samson Y. Accurate prediction of postoperative outcome in mesial temporal lobe epilepsy: a study using positron emission tomography with 18fluorodeoxyglucose. Arch Neurol 2000;57:1331-6.
- Tonini C, Beghi E, Berg AT, Bogliun G, Giordano L, Newton RW, et al. Predictors of epilepsy surgery outcome: a meta-analysis. Epilepsy Res 2004;62:75-87.
- Sutherling WW, Mamelak AN, Thyerlei D, Maleeva T, Minazad Y, Philpott L, et al. Influence of magnetic source imaging for planning intracranial EEG in epilepsy. Neurology 2008;71:990-6.
- Rathore C, Kesavadas C, Ajith J, Sasikala A, Sarma S, Radhakrishnan K. Cost-effective utilization of single photon emission computed tomography (SPECT) in decision making for epilepsy surgery. Seizure 2011;20:107-14.
- Velasco TR, Wichert-Ana L, Mathern GW, Araujo D, Walz R, Bianchin MM, et al. Utility of ictal single photon emission computed tomography in mesial temporal lobe epilepsy with hippocampal atrophy: a randomized trial. Neurosurgery 2011;68:431-6.
- Lau M, Yam D, Burneo JG. A systematic review on MEG and its use in the presurgical evaluation of localization-related epilepsy. Epilepsy Res 2008;79:97-104.
- Drummond M, Sculpher M, Torrence G, O’Brien B, Stoddart G. Methods for the economic evaluation of health care programmes. Oxford: Oxford University Press; 2005.
- National Institute for Clinical Excellence (NICE) . Guide to the Methods of Technology Appraisal 2008.
- O’Brien TJ, Miles K, Ware R, Cook MJ, Binns DS, Hicks RJ. The cost-effective use of 18F-FDG PET in the presurgical evaluation of medically refractory focal epilepsy. J Nucl Med 2008;49:931-7.
- Won HJ, Chang KH, Cheon JE, Hong Dae K, Dong Soo L, Moon Hee H, et al. Comparison of MR imaging with PET and ictal SPECT in 118 patients with intractable epilepsy. Am J Neuroradiol 1999;20:593-9.
- National Institute for Clinical Excellence (NICE) . The Epilepsies: The Diagnosis and Management of the Epilepsies in Adults and Children in Primary and Secondary Care (partial Update of NICE Clinical Guideline 20) 2011.
- R Development Core Team . R: a Language and Environment for Statistical Computing 2009.
- Zumsteg D, Wieser HG. Presurgical evaluation: current role of invasive EEG. Epilepsia 2000;41:55-60.
- Choi H, Sell RL, Lenert L, Muennig P, Goodman RR, Gilliam FG, et al. Epilepsy surgery for pharmacoresistant temporal lobe epilepsy: a decision analysis. JAMA 2008;300:2497-505.
- Tellez-Zenteno J, Dhar R, Hernandez-Ronquillo L, Wiebe S. Long-term outcomes in epilepsy surgery: antiepileptic drugs, mortality, cognitive and psychosocial aspects. Brain 2007;130:334-45.
- Boundy KL, Rowe CC, Black AB, Kitchener MI, Barnden LR, Sebben R, et al. Localization of temporal lobe epileptic foci with iodine-123 iododexetimide cholinergic neuroreceptor single-photon emission computed tomography. Neurology 1996;47:1015-20.
- Wieser HG, Ortega M, Friedman A, Yonekawa Y. Long-term seizure outcomes following amygdalohippocampectomy. J Neurosurg 2003;98:751-63.
- Christensen J, Kjeldsen MJ, Andersen H, Friis ML, Sidenius P. Gender differences in epilepsy. Epilepsia 2005;46:956-60.
- Department of Health . NHS Reference Costs 2009–10 n.d. www.dh.gov.uk/en/Publicationsandstatistics/Publications/PublicationsPolicyAndGuidance/DH_123459.
- Kind P, Hardman G, Macran S. UK population norms for EQ-5D. York: Centre for Health Economics, University of York; 1999.
- Lord SJ, Irwig L, Simes RJ. When is measuring sensitivity and specificity sufficient to evaluate a diagnostic test, and when do we need randomized trials?. Ann Intern Med 2006;144:850-5.
- Bossuyt PMM, Lijmer JG, Mol BWJ. Randomised comparisons of medical tests: sometimes invalid, not always efficient. Lancet 2000;356:1844-7.
- Pearson SD, Knudsen AB, Scherer RW, Weissberg J, Gazelle GS. Assessing the comparative effectiveness of a diagnostic technology: CT colonography. Health Aff 2008;27:1503-14.
- National Institute for Clinical Excellence (NICE) . Briefing Paper for Methods Review Workshop on Diagnostic Technologies 2007.
- Hozo I, Djulbegovic B. When is diagnostic testing inappropriate or irrational? Acceptable regret approach. Med Decis Making 2008;28:540-53.
- DeKay M. Physicians’ anticipated regret and diagnostic testing: comment on Hozo and Djulbegovic, 2008. Med Decis Making 2009;29:317-19.
- Oostenbrink R, Moons KGM, Bleeker SE, Moll HA, Grobbee DE. Diagnostic research on routine care data prospects and problems. J Clin Epidemiol 2003;56:501-6.
- Bossuyt PM, Irwig L, Craig J, Glasziou P. Diagnosis: comparative accuracy: assessing new tests against existing diagnostic pathways. BMJ 2006;332:1089-92.
- Bell ML, Rao S, So EL, Trenerry M, Kazemi N, Stead SM, et al. Epilepsy surgery outcomes in temporal lobe epilepsy with a normal MRI. Epilepsia 2009;50:2053-60.
- Berg AT, Vickrey BG, Langfitt JT, Sperling MR, Walczak TS, Shinnar S, et al. The multicenter study of epilepsy surgery: recruitment and selection for surgery. Epilepsia 2003;44:1425-33.
- Debets RM, Sadzot B, van Isselt JW, Brekelmans GJ, Meiners LC, van Huffelen AO, et al. Is 11C-flumazenil PET superior to 18FDG PET and 123I-iomazenil SPECT in presurgical evaluation of temporal lobe epilepsy?. J Neurol Neurosurg Psychiatry 1997;62:141-50.
- Duncan R, Patterson J, Roberts R, Hadley DM, Bone I. Ictal/postictal SPECT in the pre–surgical localisation of complex partial seizures. J Neurol Neurosurg Psychiatry 1993;56:141-8.
- Feucht M, Gelpi E, Urak L, Mayer H, Geldner J, Kaprian G, et al. Clinical and pathological findings in children with refractory temporal lobe epilepsy. Epilepsia 2004;45.
- Gallen CC, Tecoma E, Iragui V, Sobel DF, Schwartz BJ, Bloom FE. Magnetic source imaging of abnormal low-frequency magnetic activity in presurgical evaluations of epilepsy. Epilepsia 1997;38:452-60.
- Goncalves PM, Oliveira E, Rosado P. Apparent diffusion coefficient mapping of the hippocampus and the amygdala in pharmaco-resistant temporal lobe epilepsy. Am J Neuroradiol 2006;27:671-83.
- Hessen E, Lossius MI, Gjerstad L. Behavioural adjustment in seizure-free epilepsy patients on monotherapy. Seizure 2008;17:422-30.
- Jayakar P, Dunoyer C, Dean P, Ragheb J, Resnick T, Morrison G, et al. Epilepsy surgery in patients with normal or nonfocal MRI scans: integrative strategies offer long-term seizure relief. Epilepsia 2008;49:758-64.
- Jeong S-W, Lee SK, Hong K-S, Kim K-K, Chung C-K, Kim H. Prognostic factors for the surgery for mesial temporal lobe epilepsy: longitudinal analysis. Epilepsia 2005;46:1273-9.
- Joo EY, Tae WS, Kim SH, Seo DW, Hong SB. Longitudinal changes of hippocampal volume and shape in non–surgical and post-surgical patients with mesial temporal lobe epilepsy. Epilepsia 2006;47.
- Kim DW, Lee SK, Chu K, Park KI, Lee SY, Lee CH, et al. Predictors of surgical outcome and pathologic considerations in focal cortical dysplasia. Neurology 2009;72:211-16.
- Kim SK, Lee DS, Cheon GJ, Lee SK, Kim JY, Chung CK, et al. The lateralization of ictal Tc-99m-HMPAO SPECT in medial temporal lobe epilepsy: the relationship with dominance of simultaneous ictal EEG recording. Eur J Nucl Med 1999;26.
- Kumar RS, Ashalatha R, Radhakrishanan K. Prognostic indicators and long term seizure outcome after surgery in 68 patients with posterior cortex epilepsy: an experience from a developing country. J Neurol Sci 2009;285.
- Lee SK, Kim KK, Lee SY, Chung CK, Lee DS. Occipital lobe epilepsy: the semiology, yield of diagnostic work up, and surgical outcome. Eur J Neurol 2004;11.
- Leiderman DB, Balish M, Sato S, Kufta C, Reeves P, Gaillard WD, et al. Comparison of PET measurements of cerebral blood flow and glucose metabolism for the localization of human epileptic foci. Epilepsy Res 1992;13:153-7.
- Mastin ST, Drane WE, Gilmore RL, Helveston WR, Quisling RG, Roper SN, et al. Prospective localization of epileptogenic foci: comparison of PET and SPECT with site of surgery and clinical outcome. Radiology 1996;199:375-80.
- Matsuda K, Mihara T, Tottori T, Otubo T, Usui N, Baba K, et al. Neuroradiologic findings in focal cortical dysplasia: histologic correlation with surgically resected specimens. Epilepsia 2001;42:29-36.
- Matsuda H, Matsuda K, Nakamura F, Kameyama S, Masuda H, Otsuki T, et al. Contribution of subtraction ictal SPECT coregistered to MRI to epilepsy surgery: a multicenter study. Ann Nucl Med 2009;23:283-91.
- O’Brien TJ, So EL, Mullan BP, Cascino GD, Hauser MF, Brinkmann BH, et al. Subtraction peri-ictal SPECT is predictive of extratemporal epilepsy surgery outcome. Neurology 2000;55:1668-77.
- Pan JW, Zaveri HP, Spencer DD, Hetherington HP, Spencer SS. Intracranial EEG power and metabolism in human epilepsy. Epilepsy Res 2009;87:18-24.
- Pondal-Sordo M, Diosy D, Tellez-Zenteno JF, Sahjpaul R, Wiebe S. Usefulness of intracranial EEG in the decision process for epilepsy surgery. Epilepsy Res 2007;74:176-82.
- Rossi GF, Colicchio G, Scerrati M. Resection surgery for partial epilepsy. Relation of surgical outcome with some aspects of the epileptogenic process and surgical approach. Acta Neurochir 1994;130:101-10.
- Rowe CC, Berkovic SF, Sia ST, Austin M, McKay WJ, Kalnins RM, et al. Localization of epileptic foci with postictal single photon emission computed tomography. Ann Neurol 1989;26:660-8.
- Seki G, Hoshida T, Goda K, Hashimoto H, Nakase H, Hirabayashi H, et al. EEG, MRI, and SPECT in epilepsy: relative contributions to preoperative evaluation. Japan J Neurosurg 1998;7:421-8.
- Shih Y-H, Lirng J-F, Yen D-J, Ho DM, Yiu C-H. Surgery of intractable temporal lobe epilepsy presented with structural lesions. J Chin Med Assoc 2003;66:565-71.
- Stefan H, Schuler P, Abraham-Fuchs K, Schneider S, Gebhardt M, Neubauer U, et al. Magnetic source localization and morphological changes in temporal lobe epilepsy: comparison of MEG/EEG, ECoG and volumetric MRI in presurgical evaluation of operated patients. Acta Neurol Scand Suppl 1994;152:83-8.
- Tan KM, Britton JW, Buchhalter JR, Worrell GA, Lagerlund TD, Shin C, et al. Influence of subtraction ictal SPECT on surgical management in focal epilepsy of indeterminate localization: a prospective study. Epilepsy Res 2008;82:190-3.
- Thadani VM, Siegel A, Lewis P, Siegel AM, Jobst BC, Gilbert KL, et al. Validation of ictal single photon emission computed tomography with depth encephalography and epilepsy surgery. Neurosurg Rev 2004;27:27-33.
- Theodore WH, Sato S, Kufta C, Balish MB, Bromfield EB, Leiderman DB. Temporal lobectomy for uncontrolled seizures: the role of positron emission tomography. Ann Neurol 1992;32:789-94.
- Tripathi M, Garg A, Gaikwad S, Bal CS, Chitra S, Prasad K, et al. Intra-operative electrocorticography in lesional epilepsy. Epilepsy Res 2010;89:133-41.
- Uijl SG, Leijten FSS, Arends J, Parra J, van Huffelen AC, Moons KGM. Prognosis after temporal lobe epilepsy surgery: the value of combining predictors. Epilepsia 2008;49:1317-23.
- von Oertzen TJ, Mormann F, Reichmann K, Urbach H, Biersack H-J, Elger CE. Prospective use of SISCOM in presurgical evaluation. Epilepsia 2006;47.
- von Oertzen J, Reichmann K, Koenig R, Lengler U, Urbach H, Biersack HJ, et al. Value of SISCOM (subtraction ictal SPECT co-registered to MRI) in presurgical evaluation of epilepsy: a prospective study. Epilepsia 2002;43.
- Wang R, Li S-Y, Chen M, Zhou C. Diagnostic value of interictal diffusion–weighted imaging in evaluation of intractable temporal lobe epilepsy. Chin Med Sci J 2008;23:68-72.
- Weitemeyer L, Kellinghaus C, Weckesser M, Matheja P, Loddenkemper T, Schuierer G, et al. The prognostic value of [F]FDG-PET in nonrefractory partial epilepsy. Epilepsia 2005;46:1654-60.
- Yasuda C, Betting L, Coan A, Morita M, Tedeschi H, Oliveira E, et al. MTLE’s surgical outcome and patterns of gray matter atrophy revealed by voxel-based morphometry (VBM). Epilepsia 2007;48.
- Zijlmans M, Huiskamp G, Hersevoort M, Seppenwoolde J-H, van Huffelen AC, Leijten FSS. EEG–fMRI in the preoperative work-up for epilepsy surgery. Brain 2007;130:2343-53.
- Ahmad FU, Tripathi M, Padma MV, Gaikwad S, Gupta A, Bal CS, et al. Health-related quality of life using QOLIE-31: before and after epilepsy surgery a prospective study at a tertiary care center. Neurology India 2007;55:343-8.
- Ansari SF, Maher CO, Tubbs RS, Terry CL, Cohen-Gadol AA. Surgery for extratemporal nonlesional epilepsy in children: a meta-analysis. Childs Nerv Syst 2010;26:945-51.
- Asadi-Pooya AA, Nei M, Sharan A, Mintzer S, Evans JG, Skidmore C, et al. Antiepileptic drugs and relapse after epilepsy surgery. Neurology 2008;70:A401-2.
- Azevedo AM, Alonso NB, Vidal-Dourado M, Noffs MHdS, Pascalicchio TF, Caboclo LOSF, et al. Validity and reliability of the Portuguese–Brazilian version of the Quality of Life in Epilepsy Inventory-89. Epilepsy Behav 2009;14:465-71.
- Berg AT, Langfitt JT, Spencer SS, Vickrey BG. Stopping antiepileptic drugs after epilepsy surgery: a survey of US epilepsy center neurologists. Epilepsy Behav 2007;10:219-22.
- Beleza P, Remi J, Feddersen B, Peraud A, Noachtar S. Epidural and foramen–ovale electrodes in the diagnostic evaluation of patients considered for epilepsy surgery. Epileptic Disord 2010;12:48-53.
- Boon P, D’Have M, Vanrumste B, Van Hoey G, Vonck K, Van Walleghem P, et al. Ictal source localization in presurgical patients with refractory epilepsy. J Clin Neurophysiol 2002;19:461-8.
- Boshuisen K, Braams O, Jennekens-Schinkel A, Braun KP, Jansen FE, van Rijen PC, et al. Medication policy after epilepsy surgery. Pediatr Neurol 2009;41:332-8.
- Brooks BS, King DW, Gammal TE, Meador K, Yaghmai F, Gay JN, et al. MR imaging in patients with intractable complex partial epileptic seizures. Am J Neuroradiol 1990;11:93-9.
- Chaudhry N, Radhakrishnan A, Abraham M, Kesavadas C, Radhakrishnan VV, Sankara Sarma P, et al. Selection of ideal candidates for extratemporal resective epilepsy surgery in a country with limited resources. Epileptic Disord 2010;12:38-47.
- Clusmann H, Kral T, Fackeldey E, Blumcke I, Helmstaedter C, von Oertzen J, et al. Lesional mesial temporal lobe epilepsy and limited resections: prognostic factors and outcome. J Neurol Neurosurg Psychiatry 2004;75:1589-96.
- Costello DJ, Shields DC, Cash SS, Eskandar EN, Cosgrove GR, Cole AJ. Consideration of epilepsy surgery in adults should be independent of age. Clin Neurol Neurosurg 2009;111:240-5.
- Cukiert C, Cukiert A, Burattini J, Mariani P, Agapito D, Forster C, et al. Results of surgery for refractory epilepsy: highlights from a series of 1270 patients submitted to surgery. Epilepsia 2009;50.
- Fauser S, Bast T, Altenmuller DM, Schulte-Monting J, Strobl K, Steinhoff BJ, et al. Factors influencing surgical outcome in patients with focal cortical dysplasia. J Neurol Neurosurg Psychiatry 2008;79:103-5.
- Foldvary N, Nashold B, Mascha E, Thompson EA, Lee N, McNamara JO, et al. Seizure outcome after temporal lobectomy for temporal lobe epilepsy: a Kaplan–Meier survival analysis. Neurology 2000;54:630-4.
- Gilliam F, Faught E, Martin R, Bowling S, Bilir E, Thomas J, et al. Predictive value of MRI-identified mesial temporal sclerosis for surgical outcome in temporal lobe epilepsy: an intent-to-treat analysis. Epilepsia 2000;41:963-6.
- Jackson G, Berkovic S, Tress B, Kalnins R, Fabinyi G, Bladin P. Hippocampal sclerosis can be reliably detected by magnetic resonance imaging. Neurology 1990;40:1869-75.
- Jacobs J, Zijlmans M, Zelmann R, Chatillon CE, Hall J, Olivier A, et al. High-frequency electroencephalographic oscillations correlate with outcome of epilepsy surgery. Ann Neurol 2010;67:209-20.
- Kuzniecky R, Burgard S, Faught E, Morawetz R, Bartolucci A. Predictive value of magnetic resonance imaging in temporal lobe epilepsy surgery. Arch Neurol 1993;50:65-9.
- Mihara T, Matsuda K, Tottori T, Otsubo T, Baba K, Nishibayashi H, et al. Long-term seizure outcome following resective surgery at National Epilepsy Center in Shizuoka, Japan. Psychiatry Clin Neurosci 2004;58:S22-5.
- Mikati MA, Comair Y, Ismail R, Faour R, Rahi AC. Effects of epilepsy surgery on quality of life: a controlled study in a Middle Eastern population. Epilepsy Behav 2004;5:72-80.
- Moritake K, Mikuni N, Akiyama Y, Nagai H, Maruyama N, Takada D, et al. Postoperative quality of life outcome and employment in patients undergoing resection of epileptogenic lesions detected by magnetic resonance imaging. Neurol Med Chir 2009;49:281-5.
- Paglioli E, Palmini A, Paglioli E, da Costa JC, Portuguez M, Martinez JV, et al. Survival analysis of the surgical outcome of temporal lobe epilepsy due to hippocampal sclerosis. Epilepsia 2004;45:1383-91.
- Quarato PP, Di Gennaro G, Mascia A, Grammaldo LG, Meldolesi GN, Picardi A, et al. Temporal lobe epilepsy surgery: different surgical strategies after a non-invasive diagnostic protocol. J Neurol Neurosurg Psychiatry 2005;76:815-24.
- Roberti F, Potolicchio SJ, Caputy AJ. Tailored anteromedial lobectomy in the treatment of refractory epilepsy of the temporal lobe: long term surgical outcome and predictive factors. Clin Neurol Neurosurg 2007;109:158-65.
- Salmenpera T, Kononen M, Roberts N, Vanninen R, Pitkanen A, Kalviainen R. Association of Imaging Findings With Clinical Outcome 2002;43:304-5.
- Selwa LM, Schmidt SL, Malow TA, Beydoun A. Long–term outcome of nonsurgical candidates with medically refractory localization-related epilepsy. Epilepsia 2003;44:1568-72.
- Spooner CG, Berkovic SF, Mitchell LA, Wrennall JA, Harvey AS. New-onset temporal lobe epilepsy in children: lesion on MRI predicts poor seizure outcome. Neurology 2006;67:2147-53.
- Stefan H, Hildebrandt M, Kerling F, Kasper BS, Hammen T, Dorfler A, et al. Clinical prediction of postoperative seizure control: structural, functional findings and disease histories. J Neurol Neurosurg Psychiatry 2009;80:196-200.
- Strandberg M, Larsson E-M, Backman S, Kallen K. Pre-surgical epilepsy evaluation using 3T MRI. Do surface coils provide additional information?. Epileptic Disord 2008;10:83-92.
- Tanriverdi T, Olivier NP, Olivier A. Quality of life after extratemporal epilepsy surgery: a prospective clinical study. Clin Neurol Neurosurg 2008;110:30-7.
- Tanriverdi T, Poulin N, Olivier A. Life 12 years after temporal lobe epilepsy surgery: a long-term, prospective clinical study. Seizure 2008;17:339-49.
- Tatum WOIV, Benbadis SR, Heriaud LS, Vale FL. Normal brain MRI and temporal lobectomy: outcome and concordance in preoperative assessment. Epilepsia 2003;44.
- Tellez–Zenteno J, Dhar R, Wiebe S. Long-term seizure outcomes following epilepsy surgery: a systematic review and meta-analysis. Brain 2005;128:1188-98.
- Uijl SG, Leijten FSS, Arends JBAM, Parra J, van Huffelen AC, Moons KGM. Decision-making in temporal lobe epilepsy surgery: the contribution of basic non–invasive tests. Seizure 2008;17:364-73.
- Velasco TR, Sakamoto AC, Alexandre V, Walz R, Dalmagro CL, Bianchin MM, et al. Foramen ovale electrodes can identify a focal seizure onset when surface EEG fails in mesial temporal lobe epilepsy. Epilepsia 2006;47:1300-7.
- Yasuda CL, Tedeschi H, Oliveira ELP, Ribas GC, Costa ALC, Cardoso TAMO, et al. Comparison of short-term outcome between surgical and clinical treatment in temporal lobe epilepsy: A prospective study. Seizure 2006;15:35-40.
- Antar MA, Go RT, Macintyre WJ, Morris HH, Luders H, Van Ness P, et al. Localization of epileptic foci with fluorine-18 FDG PET scan in surgical candidate patients with complex partial seizures correlation with other modalities. Eur J Nucl Med 1991;18.
- Antar MA, Go RT, Macintyre WJ, Saha GB. Presurgical evaluation of patients with medically intractable temporal-lobe epilepsy and having normal MRI with F-18 FDG PET scan. Eur J Nucl Med 1992;19.
- Aung M, Sobel DF, Gallen CC, Hirschkoff EC. Potential contribution of bilateral magnetic source imaging to the evaluation of epilepsy surgery candidates. Neurosurgery 1995;37:1113-20.
- Berg AT, Walczak T, Hirsch LJ, Spencer SS. Multivariable prediction of seizure outcome one year after resective epilepsy surgery: development of a model with independent validation. Epilepsy Res 1998;29:185-94.
- Bilir E, Kuzniecky R, Gilliam F, Faught E, James H. Utility of 1H MRSI and hippocampal volumetry in mesial temporal lobe epilepsy: a comparative study. Annual Meeting of the American Epilepsy Society, San Diego, CA, USA, 6–9 December, 1998. Epilepsia 1998;39.
- Blum AS, Lampen KM, Tremont G, Tung GA. Is proton magnetic resonance spectroscopy imaging useful in the presurgical evaluation of patients with temporal lobe epilepsy?. Epilepsia 2003;44.
- Bohnen NI, O’Brien T, Mullan BP, So EL. Pathogenesis of cerebellar atrophy in epilepsy patients: A study using quantitative subtraction ictal SPECT and MRI cerebellar volumetry. Annual Meeting of the American Epilepsy Society, San Francisco, California, USA, 7–10 December, 1996. Epilepsia 1996;37.
- Bouvard S, Ryvlin P, Isnard J, Le Bars D, Kahane P, Guenot M, et al. Clinical utility of flumazenil-PET versus FDG-PET and MRI in refractory partial epilepsy: A prospective study in 100 patients. Annual Meeting of the American Epilepsy Society, San Diego, CA, USA, 6–9 December, 1998. Epilepsia 1998;39:102-3.
- Buchpiguel CA, Cukiert A, Hironaka FH, Cerri GG, Magalhäes AEA, Marino Júnior R. Brain SPECT in the pre-surgical evaluation of epileptic patients: preliminary results. Arq Neuropsiquiatr 1992;50:37-42.
- Cendes F, Andermann F, Dubeau F, Arnold D. MR spectroscopy imaging improves the lateralization of the epileptogenic lesion in patients with temporal lobe epilepsy. Neurology 1995;45.
- Cendes F, Caramanos Z, Andermann F, Dubeau F, Arnold D. Proton MR spectroscopic imaging and MRI volumetry in the lateralization of temporal lobe epilepsy: a series of 100 patients. Ann Neurol 1997;42:737-46.
- Chacon LMM, Catasus CS, Bender JE, Bayard JB, Garcia ME, Maeso IG, et al. A neurofunctional evaluation strategy for presurgical selection of temporal lobe epilepsy patients. MEDICC Rev 2009;11:29-35.
- Chee MWL, Morris HH, Antar M, Van Ness PC, Dinner DS. Concordant interictal temporal spikes and FDG–PET hypometabolism can accurately lateralize the epileptogenic focus in temporal lobe epilepsy. Epilepsia 1991;32.
- Cook MJ, Fish DR, Shorvon SD, Straughan K, Stevens JM. Hippocampal volumetric and morphometric studies in frontal and temporal lobe epilepsy. Brain 1992;115:1001-15.
- Cook MJ. Mesial temporal sclerosis and volumetric investigations. Acta Neurol Scand Suppl 1994;152:109-14.
- Doi T, Matsuda K, Yagi K, Seino M. Clinical usability of 123I-iomazenil SPECT for intractable localization-related epilepsies. J Japan Epilepsy Soc 1995;13:184-94.
- Eliashiv DS, Waxman A, D’Agnolo A, Gupta P. Ictal SPECT as a predictor of the epileptogenic region with long term post–resection outcome and invasive electrode confirmation in patients undergoing resective epilepsy surgery: a prospective study. J Nucl Med 1999;40.
- Eliashiv DS, D’Agnolo A, Waxman A. Substraction ictal SPECT and ictal SPECT with visual analysis as compared to invasive electrode localizations and post-surgical outcome in intractable partial epilepsy: an update on an ongoing prospective study. J Nucl Med 2000;41.
- Fischer MJM, Scheler G, Stefan H. Utilization of magnetoencephalography results to obtain favourable outcomes in epilepsy surgery. Brain 2005;128:153-7.
- Garcia JR, Simo M, Russi A, Soler M, Perez G, Lopez S, et al. Fused interictal FDG-PET and MRI guided surgery for the treatment of refractory epilepsy. Eur J Nucl Med Mol Imaging 2006;33.
- Gilliam F, Perucca P, Viikinsalo M, Morawetz R, Faught E. Improvement in adverse medication effects, symptoms of depression and seizures independently predict quality of life after temporal lobe surgery. Epilepsia 2008;49:279-80.
- Gram L, Diemer H, Kjaer L, Fuglsang-Frederiksen A, Holm S, Herning GM, et al. A comparative study of neuroimaging technics and EEG in localization of a focus in partial epilepsy. Ugeskr Laeger 1988;150:2381-3.
- Harvey AS, Hopkins IJ, Bowe JM, Cook DJ, Shield LK, Berkovic SF. Frontal lobe epilepsy: clinical seizure characteristics and localization with ictal 99mTc-HMPAO SPECT. Neurology 1993;43:1966-80.
- Kagawa K, Chugani DC, Asano E, Juhasz C, Muzik O, Shah A, et al. Epilepsy surgery outcome in children with tuberous sclerosis complex evaluated with alpha-[11C]methyl-l-tryptophan positron emission tomography (PET). J Child Neurol 2005;20:429-38.
- Knake S, Halgren E, Shiraishi H, Hara K, Hamer HM, Grant PE, et al. The value of multichannel MEG and EEG in the presurgical evaluation of 70 epilepsy patients. Epilepsy Res 2006;69:80-6.
- Knowlton RC, Burneo J, Miller D, Martin R, Prassad A, Faught RE, et al. MEG and FDG-PET in the presurgical epilepsy evaluation. Epilepsia 2003;44:142-3.
- Knowlton R, Howell J, Buddhiwardhan O, Limdi N, Faught RE, Burneo J. Ictal SPECT-PET subtraction in the presurgical epilepsy evaluation. Epilepsia 2005;46.
- Knowlton RC, Buddhiwarhdan O, Howell J, Blount J, Morawetz R, Faught RE, et al. Ictal SPECT compared to MEG in the presurgical evaluation of epilepsy. Epilepsia 2005;46.
- Knowlton R, Elgavish R, Ojha B, Blount J, Limdi N, Burneo JG, et al. The role of MEG, FDG-PET, and ictal SPECT in epilepsy surgery: relative predictive value of intracranial EEG. Epilepsia 2007;48.
- Knowlton RC, Laxer KD, Aminoff MJ, Roberts TP, Wong ST, Rowley HA. Magnetoencephalography in partial epilepsy: clinical yield and localization accuracy. Ann Neurol 1997;42:622-31.
- Knowlton R, Limdi N, Elgavish R, Paige AL, Kim H, Riley K, et al. Utility of functional imaging localization tests on epilepsy surgery decision-making. Epilepsia 2009;50:458-9.
- Kumar A, Juhasz C, Asano E, Muzik O, Chugani HT. Voxel based analysis of FDG PET images for the presurgical lateralization and localization of epileptic foci in children with intractable epilepsy. Epilepsia 2009;50.
- Kuzniecky R, Hugg JW, Hetherington H, Butterworth E, Bilir E, Faught E, et al. Relative utility of 1H spectroscopic imaging and hippocampal volumetry in the lateralization of mesial temporal lobe epilepsy. Neurology 1998;51:66-71.
- Lee MC, Lee DS, Kim ES, Lee SK, Chung JK, Koh CS. Advantage of ictal Tc-99m-IMPAO SPECT over ictal Tc-99m-ECD SPECT in localization of epileptogenic zones. J Nucl Med 1996;37.
- Lee KH, Rose DF, Fujiwara H, Hemasilpin N, Xiang J, Gelfand M. Comparison of MEG (synthetic aperture magnetometry) and SISCOM (subtraction ictal SPECT co-registered to MRI) in the presurgical evaluation of children with epilepsy. Epilepsia 2007;48.
- Medina LS, Bernal B, Dunoyer C, Cervantes L, Rodriguez M, Pacheco E, et al. Seizure disorders: functional MR imaging for diagnostic evaluation and surgical treatment: prospective study. Radiology 2005;236:247-53.
- Miller D, Burneo J, Martin RC, Prasad A, Faught RE, Morawetz R, et al. Comparison of interictal MEG and ictal SPECT in the presurgical epilepsy evaluation. Epilepsia 2003;44.
- Newton MR, Berkovic SF, Austin MC, Rowe CC, McKay WJ, Bladin PF. The lateralizing power of ictal technetium-99m HMPAO SPECT in the presurgical evaluation of temporal lobe epilepsy. J Nucl Med 1992;33.
- Newton MR, Austin MC, Chan JG, McKay WJ, Rowe CC, Berkovic SF. Ictal SPECT using technetium-99m-HMPAO: Methods for rapid preparation and optimal deployment of tracer during spontaneous seizures. J Nucl Med 1993;34:666-70.
- Newton MR, Berkovic SF, Austin MC, Rowe CC, McKay WJ, Bladin PF. Ictal postictal and interictal single-photon emission tomography in the lateralization of temporal lobe epilepsy. Eur J Nucl Med 1994;21:1067-71.
- Newton MR, Berkovic SF, Austin MC, Rowe CC, McKay WJ, Bladin PF. SPECT in the localisation of extratemporal and temporal seizure foci. J Neurol Neurosurg Psychiatry 1995;59:26-30.
- Ng TC, Comair YG, Xue M, So N, Majors A, Kolem H, et al. Temporal lobe epilepsy: presurgical localization with proton chemical shift imaging. Radiology 1994;193:465-72.
- O’Brien TJ, So EL, Mullan BP, Hauser MF, Brinkmann BH, Bohnen NI, et al. Detection of localized hypoperfusion by subtraction SPECT co-registered to MRI (SISCOM) improves the sensitivity and specificity of postictal SPECT in intractable partial epilepsy. Neurology 1998;50.
- Okpaku AS, Jang S, Alavi A. FDG–PET verse NMR spectroscopy in seizure, focus localization: an analysis of concordance rates for diagnostic procedures used in stage 1 epileptic surgery evaluations. J Nucl Med 1999;40.
- Oommen KJ, King JA, Carter LP, Chacko G, Wilson D. Utility of combined interictal and immediate postictal SPECT (IISPECT and PISPECT) in localization of epileptogenic foci by using 99mTcECD (neurolite). Epilepsia 1997;38.
- Oommen KJ. Utility of combined interictal and immediate postictal SPECT in localisation of epileptogenic foci by using 99m-Tc-ECD n.d.
- Oommen KJ, Saba S, Oommen JA, Francel PC, Arnold CD, Wilson DA. The relative localizing value of interictal and immediate postictal SPECT in seizures of temporal lobe origin. J Nucl Med 2004;45:2021-5.
- Otsuki T. Neuroimaging and presurgical evaluation of symptomatic epilepsies. Psychiatry Clin Neurosci 2004;58:S13-S15.
- Pataraia E, Simos PG, Castillo EM, Billingsley RL, Sarkari S, Wheless JW, et al. Does magnetoencephalography add to scalp video-EEG as a diagnostic tool in epilepsy surgery?. Neurology 2004;62:943-8.
- Radhakrishnan A, Kesavadas C, Bahuleyan B, Radhakrishnan K. Diffusion Tensor Imaging (DTI) aids in the presurgical evaluation and surgical planning in patients with lesional temporal and extratemporal epilepsy. Epilepsia 2009;50.
- Rowe CC, Berkovic SF, Bladin PF, Austin M, Sia B, McKay WJ. Localization of epileptic foci by post-ictal single photon computerized tomography SPECT and Technetium-99m HMPAO comparison with ictal EEG in 22 patients with complex partial seizures. Aust NZ J Med 1988;18.
- Rowe CC, Berkovic SF, Sia B, Austin M, Bladin PF, McKay WJ. Localization of epileptic foci by postictal single photon computed tomography (SPECT) and 99mTc-HMPAO: comparison with ictal EEG in 22 patients. Neurology 1988;38.
- Runge U, Kirsch G, Petersen B, Kallwellis G, Gaab MR, Piek J, et al. Ictal and interictal ECD-SPECT for focus localization in epilepsy. Acta Neurol Scand 1997;96:271-6.
- Ryvlin P, Bouvard S, Le Bars D, De Lamerie G, Gregoire MC, Kahane P, et al. Clinical utility of flumazenil-PET versus [18F]fluorodeoxyglucose-PET and MRI in refractory partial epilepsy: a prospective study in 100 patients. Brain 1998;121:2067-81.
- Seidenberg M, Kelly KG, Parrish J, Geary E, Dow C, Rutecki P, et al. Ipsilateral and contralateral MRI volumetric abnormalities in chronic unilateral temporal lobe epilepsy and their clinical correlates. Epilepsia 2005;46:420-30.
- Smith JR, King DW, Park YD, Murro AM, Lee GP, Jenkins PD. A 10-year experience with magnetic source imaging in the guidance of epilepsy surgery. Stereotact Funct Neurosurg 2003;80:14-7.
- So EL, O’Brien TJ, Mullan BP, Bohnen NI, Jack CR, Cascino GD, et al. Seizure localization in intractable partial epilepsy by subtraction ictal SPECT co-registered to MRI: a study of 47 consecutive patients. Epilepsia 1996;37.
- So EL, O’Brien TJ, Hanson DP, O’Connor MK, Mullan BP, Brinkmann BH, et al. Subtraction ictal SPECT coregistered to MRI in partial epilepsy: Validation of the technique with phantom and patient studies. Annual Meeting of the American Epilepsy Society, Boston, MA, USA, 7–10 December 1997. Epilepsia 1997;38.
- Swartz BE, Theodore WH, Sanabria E, Fisher RS. Positron emission and single photon emission computed tomographic studies in the frontal lobe with emphasis on the relationship to seizure foci. Adv Neurol 1992;57:487-97.
- Tecoma ES, Iragui VJ, Gallen C, Schwartz BJ. Comparison of magnetic source imaging (MSI) with standard techniques in the presurgical localization of temporal lobe epilepsy. Epilepsia 1995;36.
- Thomsen G, Skouboe G, Frokjaer V, de Nijs R, Knudsen GM. Interictal/ictal Tc-99m-SHMPAO SPET co-registered to MRI as a diagnostic tool in patients with epilepsy that are candidates for surgical therapy. Eur J Nucl Med Mol Imaging 2006;33.
- Tsimerinov EI, Eliashiv SD. Non-invasive MEG highly correlates with intracranial EEG recordings during resective epilepsy surgery evaluation. Epilepsia 2007;48.
- Tsimerinov EI, Muthukumaran A, Eliashiv D. Non invasive MEG correlates with intraoperative electrocorticography: absent spikes on MEG will result in absent spikes during ECoG. Neurology 2007;68.
- Venz S, Cordes M, Straub HB, Hierholzer J, Schroder R, Richter W, et al. Preoperative evaluation of drug resistant focal epilepsies with 123I-iomazenil SPECT. Comparison with video/EEG monitoring and postoperative results. Nuklearmedizin 1994;33:189-93.
- Vera P, Kaminska A, Cieuta C, Hollo A, Stievenart JL, Gardin I, et al. Use of subtraction ictal SPECT co-registered to MRI for optimizing the localization of seizure foci in children. J Nucl Med 1999;40:786-92.
- Watanabe E, Nagahori Y, Mayanagi Y. Focus diagnosis of epilepsy using near–infrared spectroscopy. Epilepsia 2002;43:50-5.
- Xiang J, Liu Y, Wang Y, Kirtman EG, Kotecha R, Chen Y, et al. Frequency and spatial characteristics of high-frequency neuromagnetic signals in childhood epilepsy. Epileptic Disord 2009;11:113-25.
- Yu SM, Liu RS, Yiu CH, Shih YH, Su MS, Yeh SH. Effectiveness of delayed postictal Tc-99m HMPAO SPECT in localization of epileptic foci in temporal lobe seizure. J Nucl Med 1995;36.
- Zhou Y, Li L, Jiang XH, Wu H. Clinical application of SPECT to the localization of corticoepileptogenic focus of intractable epilepsy. J Tongji Med Univ 1994;14:186-7.
- Assadi M, Jacobson MP, Podell K, Desantis SM, Koffler SP, Mack JM. Clinical application and EEG correlation of neuropsychological testing, MRI, and SPECT functional brain imaging in epilepsy focus localization. Epilepsia 1997;38.
- Debets RMC, Sadzot B, Maquet P, Van Veelen CWM, Van Emde Boas W, Velis D, et al. Comparison of non–invasive and invasive methods in comprehensive presurgical evaluation of refractory partial epilepsy. Second Meeting of the European Neurological Society, Brighton, UK, 30 June to 5 July 1990. J Neurol 1990;237.
- Hong K-S, Lee SK, Kim J-Y, Lee D-S, Chung C-K. Presurgical evaluation and surgical outcome of 41 patients with non-lesional neocortical epilepsy. Seizure 2002;11:184-292.
- Jabbari B, Van Nostrand D, Gunderson CH, Bartoszek D, Mitchell MH, Lombardo M, et al. EEG and neuroimaging localization in partial epilepsy. Electroencephalogr Clin Neurophysiol 1991;79:108-13.
- Kim DW, Lee SK, Yun C-H, Kim K-K, Lee DS, Chung C-K, et al. Parietal lobe epilepsy: the semiology, yield of diagnostic workup, and surgical outcome. Epilepsia 2004;45:641-9.
- Kun Lee S, Young Lee S, Kim D-W, Soo Lee D, Chung C-K. Occipital lobe epilepsy: clinical characteristics, surgical outcome, and role of diagnostic modalities. Epilepsia 2005;46:688-95.
- Kuzniecky R, Suggs S, Gaudier J, Faught E. Lateralization of epileptic foci by magnetic resonance imaging in temporal lobe epilepsy. J Neuroimaging 1991;1:163-7.
- Lee SK, Lee SY, Kim K-K, Hong K-S, Lee D-S, Chung C-K. Surgical outcome and prognostic factors of cryptogenic neocortical epilepsy. Ann Neurol 2005;58:525-32.
- Lee JJ, Lee SK, Lee S-Y, Park K-I, Kim DW, Lee DS, et al. Frontal lobe epilepsy: clinical characteristics, surgical outcomes and diagnostic modalities. Seizure 2008;17:514-23.
- Oliveira AJ, da Costa JC, Hilario LN, Anselmi OE, Palmini A. Localization of the epileptogenic zone by ictal and interictal SPECT with 99mTc-ethyl cysteinate dimer in patients with medically refractory epilepsy. Epilepsia 1999;40:693-702.
- Rowe CC, Berkovic SF, Austin MC, Saling M, Kalnins RM, McKay WJ, et al. Visual and quantitative analysis of interictal SPECT with technetium-99m-HMPAO in temporal lobe epilepsy. J Nucl Med 1991;32:1688-94.
- Shukla G, Bhatia M, Singh VP, Jaiswal A, Tripathi M, Gaikwad S, et al. Successful selection of patients with intractable extratemporal epilepsy using non-invasive investigations. Seizure 2003;12:573-6.
- Son YJ, Chung CK, Lee SK, Chang KH, Lee DS, Yi YN, et al. Comparison of localizing values of various diagnostic tests in non-lesional medial temporal lobe epilepsy. Seizure 1999;8:465-70.
- Sperling MR, Wilson G, Engel J, Babb TL, Phelps M, Bradley W. Magnetic resonance imaging in intractable partial epilepsy: correlative studies. Ann Neurol 1986;20:57-62.
- Theodore WH, Katz D, Kufta C, Sato S, Patronas N, Smothers P, et al. Pathology of temporal lobe foci: correlation with CT, MRI, and PET. Neurology 1990;40:797-803.
- Zaknun JJ, Bal C, Maes A, Tepmongkol S, Vazquez S, Dupont P, et al. Comparative analysis of MR imaging, ictal SPECT and EEG in temporal lobe epilepsy: a prospective IAEA multi-center study. Eur J Nucl Med Mol Imaging 2008;35:107-15.
- Eliashiv SD, Waxman AD, Fried I, Engel JJ. Invasive electrode assessment and surgical outcome confirmation of ictal SPECT as a predictor for epileptogenic zone. J Nucl Med 1998;39.
- Fukuda M, Masuda H, Honma J, Kameyama S, Tanaka R. Ictal SPECT analyzed by three–dimensional stereotactic surface projection in frontal lobe epilepsy patients. Epilepsy Res 2006;68:95-102.
- Heinz R, Ferris N, Lee EK, Radtke R, Crain B, Hoffman JM, et al. MR and positron emission tomography in the diagnosis of surgically correctable temporal lobe epilepsy. Am J Neuroradiol 1994;15:1341-8.
- Hong K–S, Lee SK, Kim J–Y, Lee D–S, Chung C–K. Presurgical evaluation and surgical outcome of 41 patients with non–lesional neocortical epilepsy. Proceedings of the 52nd Annual Meeting of the American Academy of Neurology, San Diego, CA, USA, April 29 to 6 May 2000. Neurology 2000;54.
- Kang J-K, Ma H-I, Lee S-A, Kang D-Y, Ryu J-S, Lee JK. Usefulness of ictal 99mTc-ECD brain SPECT for presurgical evaluation in temporal lobe epilepsy. Epilepsia 1997;38:146-7.
- Kim YK, Lee DS, Lee SK, Chung CK, Chung JK, Lee MC. (18)F-FDG PET in localization of frontal lobe epilepsy: comparison of visual and SPM analysis. J Nucl Med 2002;43:1167-74.
- Lee SK, Lee SH, Kim SK, Lee DS, Kim H. The clinical usefulness of ictal SPECT in temporal lobe epilepsy: the lateralization of seizure focus and correlation with EEG. Epilepsia 2000;41:955-62.
- Lee DS, Lee KL, Kim YK, Lee SL, Cheon JC, Kang WK, et al. Superiority of HMPAO ictal SPECT to ECD ictal SPECT in localizing the epileptogenic zone. Epilepsia 2002;43:263-9.
- Lee JJ, Kang WJ, Lee DS, Lee JS, Hwang H, Kim KJ, et al. Diagnostic performance of 18F-FDG PET and ictal 99mTc-HMPAO SPECT in pediatric temporal lobe epilepsy: quantitative analysis by statistical parametric mapping, statistical probabilistic anatomical map, and subtraction ictal SPECT. Seizure 2005;14:213-20.
- Nelissen N, Van Paesschen W, Baete K, Van Laere K, Palmini A, Van Billoen H, et al. Correlations of interictal FDG-PET metabolism and ictal SPECT perfusion changes in human temporal lobe epilepsy with hippocampal sclerosis. Neuroimage 2006;32:684-95.
- Park SW, Chang KH, Kim HD, Song IC, Lee DS, Lee SK, et al. Lateralizing ability of single–voxel proton MR spectroscopy in hippocampal sclerosis: comparison with MR imaging and positron emission tomography. Am J Neuroradiol 2001;22:625-31.
- Sperli F, Spinelli L, Seeck M, Kurian M, Michel CM, Lantz G. EEG source imaging in pediatric epilepsy surgery: a new perspective in presurgical workup. Epilepsia 2006;47:981-90.
- Tatsch KR, Weil S, Linke R, Kerner MM, Winkler PA, Hahn K, et al. Ictal ECD SPECT reliably differentiates between temporal and extratemporal epilepsy: confirmation by postoperative seizure control. J Nucl Med 2000;41.
- Weil S, Noachtar S, Arnold S, Yousry TA, Winkler PA, Tatsch K. Ictal ECD-SPECT differentiates between temporal and extratemporal epilepsy: confirmation by excellent postoperative seizure control. Nucl Med Commun 2001;22:233-7.
- Uesugi H, Shimizu H, Oda M, Matsuda H, Arai N, Nakayama H, et al. Relation between imaging, pathologic features, and clinical factors in surgical cases of temporal lobe epilepsy. Epilepsia 1996;37:68-9.
- Uesugi H, Shimizu H, Arai N, Matsuda H, Nakayama H, Maehara T, et al. Relationship between imaging and pathological features and clinical factors in surgical cases of temporal lobe epilepsy. No To Shinkei 1998;50:253-7.
- Al-Semari A, Al-Dosari M, Girvin J, McLean DR, Siddiqui K. Outcome of epilepsy surgery in patients with normal MRI. Epilepsia 2005;46.
- Altay EE, Fessler JA, Vahle VP, Attarian HP, Gilliam FG. FDG-PET hypometabolism is associated with good surgical outcome in temporal lobe epilepsy with normal MRI. Epilepsia 2003;44.
- Alving J, Nielsen H, Andersen AR. The diagnostic value of interictal SPECT in pre-surgical diagnostic work-up of temporal lobe epilepsy. Epilepsia 1999;40.
- Avery RA, Spencer SS, Studholme C, Stokking R, Morano G, Corsi M, et al. Reproducibility of serial peri-ictal single-photon emission tomography difference images in epilepsy patients undergoing surgical resection. Eur J Nucl Med 2000;27:50-5.
- Barrington SF, Koutroumanidis M, Agathonikou A, Marsden PK, Binnie CD, Polkey CE, et al. Clinical value of ‘ictal’ FDG-positron emission tomography and the routine use of simultaneous scalp EEG studies in patients with intractable partial epilepsies. Epilepsia 1998;39:753-66.
- Bouilleret V, Valenti MP, Hirsch E, Semah F, Namer IJ. Correlation between PET and SISCOM in temporal lobe epilepsy. J Nucl Med 2002;43:991-8.
- Cascino GD, Cambier DM, O’Brien TJ, So EL, Mullan BP, Brinkmann BH, et al. Subtraction ictal SPECT coregistered to MRI (SISCOM) in patients undergoing repeat temporal lobe resections for partial epilepsy. Epilepsia 1999;40.
- Cendes F, Andermann F, Dubeau F, Matthews PM, Arnold DL. Normalization of neuronal metabolic dysfunction after surgery for temporal lobe epilepsy. Evidence from proton MR spectroscopic imaging. Neurology 1997;49:1525-33.
- Chernov MF, Ochiai T, Ono Y, Muragaki Y, Yamane F, Taira T, et al. Role of proton magnetic resonance spectroscopy in preoperative evaluation of patients with mesial temporal lobe epilepsy. J Neurol Sci 2009;285:212-19.
- Cunha I, Brissos S, Dinis M, Mendes I, Nobre A, Passao V. Comparison between the results of the Symptom Checklist–90 in two different populations with temporal lobe epilepsy. Epilepsy Behav 2003;4:733-9.
- Depositario–Cabacar Dewi F, Fasano R, Yaun A, Pearl P, Conry J, Tsuchida TN, et al. MEG findings and its concordance with video EEG and subdural EEG and surgical outcomes in children. Epilepsia 2009;50.
- Drzezga A, Arnold S, Noachtar S, Tolle TR, Weber W, Yousry T, et al. Comparison of F-18 FDG-PET and C-11 FMZ-PET in extratemporal epilepsy with MRI, EEG and postoperative analysis of resected brain tissue. J Nucl Med 1998;39.
- Dupont P, Van Paesschen W, Palmini A, Ambayi R, Van Loon J, Goffin J, et al. Ictal perfusion patterns associated with single MRI-visible focal dysplastic lesions: implications for the noninvasive delineation of the epileptogenic zone. Epilepsia 2006;47:1550-7.
- Dupont S, Croize AC, Semah F, Clemenceau S, Adam C, Hasboun D, et al. Selectivity of amygdalohippocampectomy in medial temporal lobe epilepsy: a FDG-PET study. Proceedings of the 23rd International Epilepsy Congress, Prague, Czech Republic, 12–17 September 1999. Epilepsia 1999;40.
- Eliashiv SD, Waxman A, Gupta P, Fried I, Engel J, Shewmon A. Localization of rapidly propagating seizures using ictal SPECT with early injection of ECD following seizure onset: surgical outcome and invasive electrode validation. J Nucl Med 1997;38.
- Eliashiv DS, Elsas SM, Squires K, Fried I, Engel J. Ictal magnetic source imaging as a localizing tool in partial epilepsy. Neurology 2002;59:1600-10.
- Fedi M, Reutens D, Okazawa H, Andermann F, Boling W, Dubeau F, et al. Localizing value of alpha-methyl-l-tryptophan PET in intractable epilepsy of neocortical origin. Neurology 2001;57:1629-36.
- Fukuda M, Masuda H, Honma J, Fujimoto A, Kameyama S, Oishi M, et al. Magnetic brain source imaging and ictal SPECT as pre–surgical evaluation for extratemporal epilepsy patients. Epilepsia 2005;46:326-7.
- Genow A, Hummel C, Scheler G, Hopfengartner R, Kaltenhauser M, Buchfelder M, et al. Epilepsy surgery, resection volume and MSI localization in lesional frontal lobe epilepsy. Neuroimage 2004;21:444-9.
- Gross DW, Concha L, Beaulieu C. Extratemporal white matter abnormalities in mesial temporal lobe epilepsy demonstrated with diffusion tensor imaging. Epilepsia 2006;47:1360-3.
- Gupta A, Raja S, Kotagal P, Lachhwani D, Wyllie E, Bingaman WB. Ictal SPECT in children with partial epilepsy due to focal cortical dysplasia. Pediatr Neurol 2004;31:89-95.
- Guye M, Ranjeva JP, Bartolomei F, Confort-Gouny S, McGonigal A, Regis J, et al. What is the significance of interictal water diffusion changes in frontal lobe epilepsies?. Neuroimage 2007;35:28-37.
- Hammers A, Koepp MJ, Brooks DJ, Duncan JS. Periventricular white matter flumazenil binding and postoperative outcome in hippocampal sclerosis. Epilepsia 2005;46:944-8.
- Holmes MD, Tucker DM, Quiring JM, Hakimian S, Miller JW, Ojemann JG. Comparing noninvasive dense array and intracranial electroencephalography for localization of seizures. Neurosurgery 2010;66:354-62.
- Iida K, Otsubo H, Matsumoto Y, Ochi A, Oishi M, Holowka S, et al. Characterizing magnetic spike sources by using magnetoencephalography-guided neuronavigation in epilepsy surgery in pediatric patients. J Neurosurg 2005;102:187-96.
- Iwasaki M, Nakasato N, Shamoto H, Nagamatsu K-I, Kanno A, Hatanaka K, et al. Surgical implications of neuromagnetic spike localization in temporal lobe epilepsy. Epilepsia 2002;43:415-24.
- Juhasz C, Chugani DC, Muzik O, Shah A, Shah J, Watson C, et al. Relationship of flumazenil and glucose PET abnormalities to neocortical epilepsy surgery outcome. Neurology 2001;56:1650-8.
- Kakisaka Y, Haginoya K, Ishitobi M, Togashi N, Kitamura T, Wakusawa K, et al. Utility of subtraction ictal SPECT images in detecting focal leading activity and understanding the pathophysiology of spasms in patients with West syndrome. Epilepsy Res 2009;83:177-83.
- Kamimura T, Tohyama J, Oishi M, Akasaka N, Kanazawa O, Sasagawa M, et al. Magnetoencephalography in patients with tuberous sclerosis and localization–related epilepsy. Epilepsia 2006;47:991-7.
- Kim SK, Lee DS, Lee SK, Kim YK, Kang KW, Chung CK, et al. Diagnostic performance of [18F]FDG-PET and ictal [99mTc]-HMPAO SPECT in occipital lobe epilepsy. Epilepsia 2001;42:1531-40.
- Kitamura Y, Uohashi T, Ishizu S, Kiriyama H, Agari T, Takahashi K, et al. Usefulness of MEG spike analysis by multiple signal classification algorithm in surgical treatment for epilepsy. Epilepsia 2003;44.
- Knowlton RC, Lawn ND, Mountz JM, Buddhiwardhan O, Miller S, Burneo JG, et al. Ictal single–photon emission computed tomography imaging in extra temporal lobe epilepsy using statistical parametric mapping. J Neuroimaging 2004;14:324-30.
- Knowlton R, Rowley H, Wong S, Roberts T, Laxer K. Registration of magnetoencephalography and high-resolution positron emission tomography with magnetic-resonance-imaging in the evaluation of non-lesional partial epilepsy. Epilepsia 1995;36.
- Knowlton R, Kuzniecky R, Palmer C, Abou-Khalil B, Viikinsalo M, Sawrie S. Hippocampal 1H-MRSI, FDG–PET and surgical histopathology in mesial temporal lobe epilepsy. Annual Meeting of the American Epilepsy Society, Los Angeles, CA, USA, December 01–06, 2000. Epilepsia 2000;41.
- Koo CW, Devinsky O, Hari K, Balasny J, Noz ME, Kramer EL. Epilepsia 2003;44:379-86.
- Krsek P, Hajek M, Dezortova M, Jiru F, Skoch A, Marusic P, et al. H–1 MR spectroscopic imaging in patients with MRI-negative extratemporal epilepsy: correlation with ictal onset zone and histopathology. Eur Radiol 2007;17:2126-35.
- Lambert MV, Brierley B, Al-Sarraj S, Shaw P, Polkey CE, Chandler C, et al. Quantitative magnetic resonance imaging of the amygdala in temporal lobe epilepsy–clinico–pathological correlations (a pilot study). Epilepsy Res 2003;53:39-46.
- Lamusuo S, Pitkanen A, Jutila L, Ylinen A, Partanen K, Kalviainen R, et al. [11C]Flumazenil binding in the medial temporal lobe in patients with temporal lobe epilepsy: correlation with hippocampal MR volumetry, T2 relaxometry, and neuropathology. Neurology 2000;54:2252-60.
- Lamusuo S, Jutila L, Ylinen A, Kalviainen R, Mervaala E, Haaparanta M, et al. [18F]FDG-PET reveals temporal hypometabolism in patients with temporal lobe epilepsy even when quantitative MRI and histopathological analysis show only mild hippocampal damage. Arch Neurol 2001;58:933-9.
- Lantz G, Grave de Peralta R, Spinelli L, Seeck M, Michel CM. Epileptic source localization with high density EEG: how many electrodes are needed?. Clin Neurophysiol 2003;114:63-9.
- Lantz G, Seeck M, Lazeyras F. Extent of preoperative abnormalities and focus lateralization predict postoperative normalization of contralateral 1H-magnetic resonance spectroscopy metabolite levels in patients with temporal lobe epilepsy. Am J Neuroradiol 2006;27:1766-9.
- Lassen NA, Andersen AR, Dam M. Technetium-99m-HMPAO and xenon-133 SPECT for presurgical evaluation of temporal lobe epilepsy. 36th Annual Meeting of the Society for Nuclear Medicine, St. Louis, MO, USA, 13–16 June 1989. J Nucl Med 1989;30:896-7.
- Liew CJ, Lim Y-M, Bonwetsch R, Shamim S, Sato S, Reeves-Tyer P, et al. 18F-FCWAY and 18F-FDG PET in MRI-negative temporal lobe epilepsy. Epilepsia 2009;50:234-9.
- Lynch BJ, O’Tuama LA, Treves ST, Mikati M, Holmes GL. Correlation of 99mTc-HMPAO SPECT with EEG monitoring: prognostic value for outcome of epilepsy surgery in children. Brain Dev 1995;17:409-17.
- MacDougall KW, Steven DA, Parrent AG, Burneo JG. Supplementary implantation of intracranial electrodes in the evaluation for epilepsy surgery. Epilepsy Res 2009;87:95-101.
- Maehara T, Tanaka Y, Nariai T, Ishii K, Ishiwata K, Ohno K. Multimodal navigation–guided temporal lobe epilepsy surgery using focus detection by F-18 fluorodeoxyglucose PET. Epilepsia 2005;46.
- Mayer CL, Miller JW, Lewellen B, Cross DJ, Minoshima S. Predicting outcome of temporal lobe resection in medically refractory epilepsy: statistical mapping analysis of FDG-PET. Epilepsia 2003;44:80-1.
- Mayer CL, Miller JW, Lewellen BL, Ojemann GA, Cross DJ, Minoshima S. Prognostic value of FDG PET in predicting outcome following temporal lobectomy in medically refractory epilepsy patients. J Nucl Med 2003;44.
- Menzel C, Steidele S, Grunwald F, Hufnagel A, Pavics L, Elger CE, et al. Evaluation of technetium-99m-ECD in childhood epilepsy. J Nucl Med 1996;37:1106-12.
- Minassian BA, Otsubo H, Weiss S, Elliott I, Rutka JT, Snead OC. Magnetoencephalographic localization in pediatric epilepsy surgery: comparison with invasive intracranial electroencephalography. Ann Neurol 1999;46:627-33.
- Mohamed A, Eberl S, Fulham MJ, Kassiou M, Zaman A, Henderson D, et al. Sequential 123I-iododexetimide scans in temporal lobe epilepsy: comparison with neuroimaging scans (MR imaging and 18F-FDG PET imaging). Eur J Nucl Med Mol Imaging 2005;32:180-5.
- Oishi M, Otsubo H, Iida K, Suyama Y, Ochi A, Weiss SK, et al. Preoperative simulation of intracerebral epileptiform discharges: synthetic aperture magnetometry virtual sensor analysis of interictal magnetoencephalography data. J Neurosurg 2006;105:41-9.
- O’Tuama LA, Holmes GL, Black PM, Lombroso CT, Ulanski J, Davis RT, et al. HMPAO SPECT versus EEG localization of foci in childhood-onset epilepsy clinical correlations and relationship to outcome. J Nucl Med 1991;32:912-13.
- Otsubo H, Ochi A, Elliott I, Chuang SH, Rutka JT, Jay V, et al. MEG predicts epileptic zone in lesional extrahippocampal epilepsy: 12 pediatric surgery cases. Epilepsia 2001;42:1523-30.
- Ozkara C, Hanodlu L, Dincer A, Keskinkylyc C, Etkazan E, Ozyurt E. Concordance of 1H MR spectroscopic imaging (MRSI) and neuropsychological tests before and after selective amygdalohippocampectomy (SAH) in mesial temporal lobe epilepsy. Epilepsia 1999;40.
- Paetau R, Kajola M, Karhu J, Nousiainen U, Partanen J, Tiihonen J, et al. Magnetoencephalographic localization of epileptic cortex: impact on surgical treatment. Ann Neurol 1992;32:106-9.
- Pataraia E, Lindinger G, Deecke L, Mayer D, Baumgartner C. Combined MEG/EEG analysis of the interictal spike complex in mesial temporal lobe epilepsy. Neuroimage 2005;24:607-14.
- Pu Y, Mintun MA, Trevathan NE, Gilliam FG, Moerlein SM, Ojemann JG. [11C] Flumazenil PET in the localization of the focus of epileptogenicity and surgical decision-making in intractable extra-limbic epilepsy. Radiology 2002;225.
- Sasaki M, Koga H, Nakagawa M, Kaneko K, Hayashi K, Kuwabara Y, et al. Presurgical identification of an epileptogenic area of temporal lobe epilepsy by 123I–iomazenil SPECT: a comparison with IMP-SPECT and FDG-PET. J Nucl Med 2003;44.
- Shah J, Zhai H, Fuerst D, Watson C. Hypersalivation in temporal lobe epilepsy. Epilepsia 2006;47:644-51.
- Shukla G, Bhatia M, Padma Srivastava MV, Tripathi M, Srivastava A, Singh VP, et al. Unidirectional whole body turning: a new lateralising sign in complex partial seizures. J Neurol Neurosurg Psychiatry 2005;76:1726-9.
- Stefan H, Schneider S, Feistel H, Pawlik G, Schuler P, Abraham-Fuchs K, et al. Ictal and interictal activity in partial epilepsy recorded with multichannel magnetoelectroencephalography – correlation of electroencephalography electrocorticography, magnetic- resonance-imaging, single photon emission computed tomography, and positron emission tomography findings. Epilepsia 1992;33:874-87.
- Suhy J, Laxer KD, Capizzano AA, Vermathen P, Matson GB, Barbaro NM, et al. 1H MRSI predicts surgical outcome in MRI-negative temporal lobe epilepsy. Neurology 2002;58:821-3.
- Szaflarski JP, Holland SK, Schmithorst VJ, Shear PK, Cahill WT, Privitera MD, et al. Can fMRI at 3 tesla predict epilepsy surgery outcome?. Epilepsia 2003;44:88-9.
- Szaflarski JP, Holland SK, Schmithorst VJ, Dunn RS, Privitera MD. High-resolution functional MRI at 3T in healthy and epilepsy subjects: hippocampal activation with picture encoding task. Epilepsy Behav 2004;5:244-52.
- Tanaka F, Yonekura Y, Ikeda A, Terada K, Mikuni N, Nishizawa S, et al. Presurgical identification of epileptic foci with iodine–123 iomazenil SPET: comparison with brain perfusion SPET and FDG PET. Eur J Nucl Med 1997;24:27-34.
- Tanaka F, Otsubo H, Gaetz WC, Chuang S, Houle S, Rutka JT, et al. FDG PET and MEG evaluation of focal cortical dysplasia: comparison with the results of intracranial invasive EEG and epilepsy surgery. J Nucl Med 2000;41.
- Tatum WO, Sperling MR, O’Connor MR, Jacobstein JG. Interictal SPECT as a predictor of post-operative seizure relief in temporal lobe epilepsy. Annual Meeting of the American Epilepsy Society, New Orleans, LA, USA, 2–8 December 1994. Epilepsia 1994;35.
- Tepmongkol S, Locharernkul C, Limotai C, Loplumlert J. How FDG–PET helps making decision for surgery in various difficult subgroups of temporal lobe epilepsy? n.d.
- Umeoka S, Matsuda K, Baba K, Usui N, Tottori T, Terada K, et al. Usefulness of 123I-iomazenil single-photon emission computed tomography in discriminating between mesial and lateral temporal lobe epilepsy in patients in whom magnetic resonance imaging demonstrates normal findings. J Neurosurg 2007;107:352-63.
- Vitikainen AM, Lioumis P, Paetau R, Salli E, Komssi S, Metsahonkala L, et al. Combined use of non-invasive techniques for improved functional localization for a selected group of epilepsy surgery candidates. Neuroimage 2009;45:342-8.
- Watson C, Nielson S, Cobb C, Burgerman R, Hsia R. Postoperative volumetric MRI, pathology, and surgical outcome in temporal lobe epilepsy (TLE). Annual Meeting of the American Epilepsy Society, New Orleans, LA, USA, 2–8 December 1994. Epilepsia 1994;35.
- Watson C, Moore GJ. Comparison of preoperative vs. postoperative 1H MR spectroscopic imaging findings in the contralateral temporal lobe following ipsilateral resection in adult temporal lobe epilepsy patients. Neurology 1998;50.
- Eliashiv SD, Waxman AD. Relative sensitivity of ictal SPECT versus FDG-PET in intractable partial epilepsy patients with invasive electrode and outcome confirmation. J Nucl Med 1998;39.
- Wichert-Ana L, Garzon E, Bustamante VCT, Velasco TR, Leite JP, Iazigi N, et al. Role of serial ictal SPECTS in the presurgical evaluation of intractable epilepsies. Epilepsia 1998;39.
- Wichert-Ana L, de Azevedo-Marques PM, Oliveira LF, Fernandes RMF, Velasco TR, Santos AC, et al. Ictal technetium-99 m ethyl cysteinate dimer single-photon emission tomographic findings in epileptic patients with polymicrogyria syndromes: a subtraction of ictal-interictal SPECT coregistered to MRI study. Eur J Nucl Med Mol Imaging 2008;35:1159-70.
- Wissmeyer M, Schindler K, Wiest R, Ritter P, Lovblad K, Weder B, et al. Ictal and interictal subtraction CBFSPECT fused with high resolution brain MRI in the presurgical evaluation of epilepsy: preliminary results. Eur J Nucl Med 2001;28.
- Wissmeyer M, Schindler K, Loevblad KO, Luescher D, Krause T, Weder B. Subtraction ictal SPECT coregistrated to high resolution MRI (SISCOM) in the presurgical evaluation of temporal lobe epilepsy (TLE). Radiology 2002;225.
- Wong CY, Geller EB, Chen EQ, MacIntyre WJ, Morris HH, Raja S, et al. Outcome of temporal lobe epilepsy surgery predicted by statistical parametric PET imaging. J Nucl Med 1996;37:1094-100.
- Wu JY, Sutherling WW, Koh S, Salamon N, Jonas R, Yudovin S, et al. Magnetic source imaging localizes epileptogenic zone in children with tuberous sclerosis complex. Neurology 2006;66:1270-2.
- Yeh H-S, Pretorius HT, Ficker D, Privitera M, Cahill W, Strawsberg R. FDG-SPECT: comparable to PET for presurgical evaluation of temporal lobe epilepsy. Epilepsia 1998;39:104-5.
- Biersack HJ, Grunwald F, Elger CE, Hufnagel A, Durwen HF, Bockisch A, et al. Localization of epileptic foci by HMPAO brain SPECT correlation with the site of temporal lobectomy. J Nucl Med 1990;31.
- Akamatsu N, Yamano M, Tsuji S, Tomoda Y. Usefulness of interictal 123I-iomazenil-SPECT for localising epileptogenic focus compared with 18F-FDG-PET and 99mTC-ECD-SPECT as presurgical evaluation for intractable epilepsy. Epilepsia 2007;48.
- Antar MA, McIntyre W. Can F-18FDG PET help in the diagnosis of bitemporal epilepsy? Correlation with the invasive gold standard ictal depth EEG and surgical outcome. Eur J Nucl Med Mol Imaging 2006;33.
- Antel SB, Li LM, Cendes F, Olivier A, Andermann F, Dubeau F, et al. An MR-based Bayes classifier for the prediction of surgical outcome in temporal lobe epilepsy patients. Epilepsia 2000;41.
- Eliashiv D, Mullin P, D’Agnolo A, Waxman A. Relative sensitivity of ictal SPECT versus FDG-PET in the evaluation of medically refractory partial epilepsy. J Nucl Med 2002;43.
- Fujiwara H, Rose DF, Hemasilpin N, Lee KH, Mangano FT, Seo JH, et al. MEG source localization with multiple algorithms in pediatric epilepsy surgery candidates with tuberous sclerosis complex. Epilepsia 2009;50:178-9.
- Hetherington HP, Kuzniecky RI, Spencer DD, Vives K, Pan JW. Predicting surgical outcome following temporal lobectomy using a neural network. Epilepsia 2007;48.
- Lee G, Pan J, Spencer D, Kuzniecky R, Hetherington H. Anterior hippocampal dysfunction predicts post-surgical seizure outcomes in medial temporal lobe epilepsy: an MR spectroscopic study. Epilepsia 2006;47.
- Lee DS, Lee SK. Predictive values of F-18 FDG PET and ictal SPECT to find epileptogenic zones in cryptogenic neocortical epilepsies. J Nucl Med 1997;38.
- Radtke RA, Lee N, Hanson MW, Tien RD, Foldvary N, Van Landingham KE, et al. Comparison of volumetric hippocampal MRI and FDG-PET: correlation with surgical outcome in mesial TLE. Annual Meeting of the American Epilepsy Society, Baltimore, MD, USA, 1–6 December 1995. Epilepsia 1995;36.
- Sundaram S, Chugani D, Govindan RM, Asano E, Juhasz C, Chakraborty P, et al. Alpha-methyl-l-tryptophan positron emission tomography (AMT-PET) predicts surgical outcome in children with intractable neocortical epilepsy. Ann Neurol 2006;60.
- Tan KM, Britton J, Buchhalter J, Worrell G, Cascino G, Lagerlund T, et al. Influence of SISCOM on decision-making in epilepsy surgery. Neurology 2008;70.
- Tripathi M, Chandra S, Madakasira P, Singh M, Shukla G, Gaikwad S, et al. Concordance of interictal EEG, long-term Ictal video EEG, MRI, and interictal SPECT and histopathology with outcome in patients operated on for intractable epilepsy. Epilepsia 2004;45.
- Tripathi M, Chandra S, Shukla G, Gaikwad S, Bal C, Sarkar C, et al. Concordance of interictal EEG, long term ictal video EEG, MRI and interictal SPECT and histopathology with outcome in patients operated on due to intractable epilepsy. Eur J Neurol 2004;11.
- Van Huffelen AC, Uijl SG, Leijten FSS, Arends JBA, Parra J, Moons KGM. Diagnostic value of non-invasive testing in the decision to perform temporal lobe epilepsy surgery. Epilepsia 2004;45.
- Vanisselt JW, Vanhuffelen AC, Vanrijk PP. The Value of I–123 Iomazenil SPECT in the presurgical work-up of patients with medically intractable focal epilepsy. J Nucl Med 1993;34.
- von Oertzen J, Reichmann K, Koenig R, Lengler U, Urbach H, Biersack HJ, et al. Value of SISCOM (subtraction Ictal SPECT Co-Registered to MRI) in Presurgical Evaluation of Epilepsy: A Prospective Study n.d.
- Vorgia P, Kahane P, Hirsch E, Bourgeois M, Ryvlin P, Sainte-Rose CH, et al. A French multicentre and multidisciplinary network for epilepsy surgery in children. Epilepsia 2005;46.
- Zuchun H, Xiaoping W. Studies on predictors of the postsurgical outcome in epilepsy. Epilepsia 2009;50:261-2.
- Di Gennaro G, Quarato PP, Mascia A, Picardi A, Sparano A, Grammaldo LG, et al. Temporal lobe epilepsy surgery: different surgical strategies after a non-invasive diagnostic protocol. Boll Lega It Epil 2005:109-12.
- Leroy RF, Mickey B, Devous M. EEG concordance versus surgical outcome: determining the ‘gold standard’ for evaluating SPECT in epilepsy surgery. Epilepsia 1992;33.
- Mohamed A, Fulham MJ, Bleasal AF, Somerville ES, Vignaendra V, Walsh JC. Role of FDG-PET in work-up of refractory epilepsy patients for surgery in two epilepsy centres in Sydney. Annual Meeting of the American Epilepsy Society, Los Angeles, CA, USA, 1–6 December 2000. Epilepsia 2000;41:63-4.
- O’Brien TJ, So EL, Cascino GG, Mullan BP, Hauser MF, Marsh R, et al. Subtraction SPECT coregistered to MRI provides sensitive and specific localization of the epileptogenic zone in medically refractory epilepsy associated with disorders of cortical development. Neurology 2001;56.
- Oh J-B, Lee SK, Yeo JS, Kim S-K, Chung C-K. Fluorodeoxyglucose (FDG)-positron emission tomography and surgical outcome of temporal lobe epilepsy: Severity and extent of hypometabolism quantified by probabilistic atlas of brain. Annual Meeting of the American Epilepsy Society, Philadelphia, PA, USA, 30 November to 5 December 2001. Epilepsia 2001;42.
- Politsky JM, Murro AM, Park YD, Lee KH, Lee MR, Pillai JJ, et al. The impact of SISCOM data regarding surgical planning and understanding the spread of neuronal activity in intractable epilepsy patients. Epilepsia 2003;44:143-4.
- Thadani VM, Siegel AM, Darcey TM, Gilbert KL, Williamson PD, Roberts DW, et al. Correlation of ictal SPECT, depth EEG, and epilepsy surgery. Epilepsia 1999;40:154-5.
- Willmore LJ, Wheless JW, Breier JI, Kataki M, Smith JR, King DW, et al. A comparison of magnetoencephalography (MEG), MRI, and V-EEG in patients evaluated for epilepsy surgery. Annual Meeting of the American Epilepsy Society, San Diego, CA, USA, 6–9 December 1998. Epilepsia 1998;39:64-5.
- Dupont S, Semah F, Hasboun D, Clemenceau S, Baulac M, Samson Y. Accurate prediction of surgical outcome in mesial temporal lobe epilepsy (MTLE): a FDG PET and MRI study. Neurology 1997;48.
- Braakman H. IMAGINE Study (imaging Study in Epilepsy) Is There a Neuronal Correlate for Cognitive Impairment and Response to Antiepileptic Drugs in Children With Frontal Lobe Epilepsy? 2009. www.trialregister.nl/trialreg/admin/rctview.asp?TC%20=%201749 (accessed July 2010).
- Charron M. Combined Role of Position Emission Tomography (PET) and Magnetoencephalography (MEG) in Nonlesional Epilepsy. Combined Role of PET and MEG in Nonlesional Epilepsy in Pediatric Population 2008. clinicaltrials.gov/show/NCT00741559 (accessed July 2010).
- Gavaret M. Localizing the Epileptogenic Zone With High Resolution Electroencephalography in Presurgical Evaluation of Partial Epilepsies 2009. clinicaltrials.gov/show/NCT01090934 (accessed July 2010).
- Bourgeois M, Di Rocco F, Roujeau T, Boddaert N, Lelouch-Tubiana A, Varlet P, et al. Epilepsy and focal lesions in children. Surgical management. Neurochirurgie 2008;54:362-5.
- Devaux B, Marnet D, Landre E, Turak B, Rodrigo S, Varlet P, et al. Surgical resection of focal cortical dysplasias in eloquent areas. Epilepsies 2009;21:45-53.
- He H-J, Shen T-Z, Chen X-R, Feng X-Y, Jiang C-C, Gao X. Prognostic value of MRI and 1H MRS in patients with temporal lobe epilepsy. Chin Med J 2003;83:1586-91.
- He H, Shen T, Chen X. Comparison of (1)H MRS, PET and EEG in lateralization of temporal lobe epilepsy. Chin Med J 2001;81:1242-5.
- Maehara T. Epilepsy surgery and focus detection utilizing imaging of preoperative multi-modal examinations. No Shinkei Geka 2007;35:1053-65.
- Matsuda K, Mihara T, Tottori T, Ohtsubo T, Baba K, Matsuyama N, et al. Neuroimaging and electrophysiological study in epilepsy. Rinsho Byori 2001;49:29-38.
- Papathanassiou D, Domange-Testard A, Thiriaux A, Bruna-Muraille C, Cuif-Job A, Motte J, et al. FDG PET role in the management of refractory epilepsy. Medecine Nucleaire 2009;33:352-62.
- Setoain X, Carreno M, Bargallo N, Rumia J, Donaire A, Fuster D, et al. The utility of interictal SPECT in temporal lobe epilepsy. Neurologia 2006;21:226-31.
- Stefan H, Eberhardt KE, Pauli E, Schafer I, Paulus W, Kasper B, et al. Diagnostic imaging in refractory temporal lobe epilepsy. A comparison of MR volumetry and multivoxel-MR-spectroscopy for assessment of postoperative prognosis. Nervenarzt 2001;72:130-5.
- van Veelen CW, van Rijen PC, Debets RM, van Wijk-Leenaars PW, van Emde Boas W. Dutch Collaborative Epilepsy Surgery Program: reduction of seizures, operative complications and tapering of medication in 338 patients, 1973–1998. Ned Tijdschr Geneeskd 2001;145:2223-8.
- Wu T, Zhang R, Liu HY, Yang L, Chen QQ. The clinical value of magnetoencephalography in preoperative localization of refractory epilepsy. Chin J Contemp Neurol Neurosurg 2009;9:361-5.
- Yu T, Wang YP, Li YJ, Zhang GJ, Du W. Localization of epileptogenic zones for frontal lobe epilepsy. Zhonghua Shen Jing Ge Za Zhi 2004;37:484-6.
- Citterio A, Colombo N, Mai R, Tassi L, Galli C, Villani F, et al. MRI in temporal epilepsy: correlation of neuroradiological and anatomopathological characteristics in 100 surgically treated patients. Riv Neuroradiol 2003;16:221-2.
- Engel J. Multimodal approaches in the evaluation of epilepsy patients for surgery. Electroencephalogr Clin Neurophysiol Suppl 1999;50:40-52.
- Huijin H, Tianzhen S, Zingrong C. Comparison of MRI, MRS, PET and EEG in the diagnosis of temporal lobe epilepsy. Chin Med J 2001;114:70-9.
- Hwang PA, Dilday DL, Adams C. SPECT studies in epilepsy: application to epilepsy surgery in children. J Epilepsy 1990;1:83-92.
- Lee MC, Chung JK, Lee DS, Jeong JM, Lee SK, Yeo JS, et al. Comparison of diagnostic performance between interictal F-18-FDG PET and ictal Tc-99m-HMPAO SPECT in occipital lobe epilepsy. Korean J Nucl Med 1999;33:262-72.
- Manych M. Better identification of patients for epilepsy surgery by means of automated PET scans. Rofo Fortschr Geb Rontgenstr 2007;179.
- Ogden ML. A Comparison of Methods to Localize the Epileptic Focus: Determining the Most Effective Predictors of Seizure Laterality 1998.
- Pinard JM, Bobain O, Lorti A, Dulac O, Delalande O. Epilepsy and cortical dysplasia in children: histologic and radiologic findings. Annual Meeting of the American Epilepsy Society, Miami, FL, USA, 5–8 December 1993. Epilepsia 1993;34:38-9.
- Sencer S, Kinay D, Gurses C, Kurt BB, Poyanli A, Gokyigit A, et al. Clinical and magnetic resonance imaging findings in patients with surgically treated mesial temporal sclerosis. Tani Girisim Radyol 2003;9:171-5.
- Vatti G, Mariottini A, Pucci B, Marino D, Santarnecchi E, Bernardi A, et al. Results of epilepsy surgery in patients selected with non invasive diagnostic work up. The experience of epilepsy centre of Siena. Boll Lega It Epil 2008;138/139:79-81.
- Xu R-X, Liu Z-L, Zhang X-W, Zhou G-L, Ke Y-Q, Peng P, et al. Temporal lobe epilepsy surgery and preoperative factors predictive of postoperative outcome: retrospective analysis of 143 cases. Di Yi Jun Yi Da Xue Xue Bao 2003;23:663-7.
- Cascino GD, Jack CR, Parisi JE, Sharbrough FW, Hirschorn KA, Meyer FB, et al. Magnetic resonance imaging-based volume studies in temporal lobe epilepsy: pathological correlations. Ann Neurol 1991;30:31-6.
- Knowlton RC, Razdan SN, Limdi N, Elgavish RA, Killen J, Blount J, et al. Effect of epilepsy magnetic source imaging on intracranial electrode placement. Ann Neurol 2009;65:716-23.
- Knowlton RC, Elgavish R, Howell J, Blount J, Burneo JG, Faught E, et al. Magnetic source imaging versus intracranial electroencephalogram in epilepsy surgery: a prospective study. Ann Neurol 2006;59:835-42.
- Pan JW, Cavus I, Kim J, Hetherington HP, Spencer DD. Hippocampal extracellular GABA correlates with metabolism in human epilepsy. Metab Brain Dis 2008;23:457-68.
- Goncalves Pereira PM, Oliveira E, Rosado P. Relative localizing value of amygdalo-hippocampal MR biometry in temporal lobe epilepsy. Epilepsy Res 2006;69:147-64.
Appendix 1 Literature search strategies
MEDLINE/PREMEDLINE
Interface used: Ovid MEDLINE(R) In-Process & Other Non-Indexed Citations and Ovid MEDLINE(R) – 1950 to present
-
Search date: 23 July 2010.
-
Records identified: 1936.
-
Restrictions: 2003 to present.
-
exp Epilepsy/ (110,555)
-
epilep$.ti,ab. (75,417)
-
1 or 2 (123,219)
Line 3 captures terms for epilepsy
-
exp Magnetic Resonance Imaging/ (232,901)
-
exp Magnetic Resonance Spectroscopy/ (152,076)
-
Magnetoencephalography/ (4460)
-
(magnetic resonance tomograph$ or magnetic resonance scan$ or magnetic resonance imag$ or magnetic resonance spectroscop$ or magnetoencephalograph$ or magnetic source imag$ or high density scalp eeg or high density scalp electroencephalogra$ or high density video eeg or high density video electroencephalogra$ or high density eeg or high density electroencephalogra$).ti,ab. (110,579)
-
(mr tomograph$ or mr scan$ or mr imag$ or mr spectroscop$).ti,ab. (38,154)
-
(fmri or mri or nmr or mrs or mrsi or hmrs or “(1)hmrs” or 1hmrs or ihmrs or hmrsi or meg or msi or hd-eeg or hd eeg or hdeeg).ti,ab. (194,728)
-
exp Tomography, Emission-Computed/ (59,345)
-
(spect or single photon emission computed tomograph$).ti,ab. (18,581)
-
(pet or positron emission tomograph$ or subtraction ictal spect or subtraction ictal single photon emission computed tomograph$ or siscom).ti,ab. (43,592)
-
volumetric exam$.ti,ab. (7)
-
quantitative t2 measure$.ti,ab. (8)
-
voxel based morphomet$.ti,ab. (1214)
-
(diffusion weight$ imag$ or dwi).ti,ab. (3867)
-
(diffusion tensor imag$ or dti).ti,ab. (3280)
-
(fluid attenuated inversion recovery or flair).ti,ab. (1896)
-
or/4-18 (503,707)
Line 19 captures terms for imaging technology
-
3 and 19 (13,231)
Line 20 captures records containing both epilepsy terms and imaging technology terms
-
Neurosurgical Procedures/ (11,010)
-
Anterior Temporal Lobectomy/ (390)
-
exp Cerebral Decortication/ (951)
-
exp Craniotomy/ (8031)
-
Split-Brain Procedure/ (17)
-
(surger$ or surgic$ or neurosurger$ or neurosurgic$ or lobectom$ or cortical resection or hemispherotom$ or hemispherectom$ or callosotom$ or callotom$).ti,ab. (992,393)
-
or/21-26 (1,000,015)
Line 27 captures surgery terms
-
20 and 27 (3751)
Line 28 captures records containing epilepsy terms and imaging technology terms and surgery terms
-
exp Animals/ (14,917,330)
-
Humans/ and exp Animals/ (11,394,975)
-
29 not 30 (3,522,355)
-
28 not 31 (3734)
Line 32 discards records which are about animals
-
(2003$ or 2004$ or 2005$ or 2006$ or 2007$ or 2008$ or 2009$ or 2010$).ed. (5,599,605)
Line 33 retrieves records which were added to MEDLINE (i.e. entry date) between 2003 and 2010
-
32 and 33 (1936)
Key:
-
/ = indexing term (MeSH heading)
-
exp = exploded MeSH heading
-
$ = truncation
-
.ti,ab. = terms in either title or abstract fields
Notes:
The appropriate MeSH heading for HD-EEG is Electroencephalography. However, this heading covers a lot of material irrelevant to this search, so it was discarded in this strategy.
The MeSH heading Tomography, Emission-Computed/ includes the headings Tomography, Emission-Computed, Single-Photon and Positron Emission Tomography.
BIOSIS Previews
Interface used: FILE 55: Biosis Previews(R) 1993–2010/August W5 (c) 2010 The Thomson Corporation
-
Search date: 7 September 2010.
-
Records identified: 623.
-
Restrictions: 2008 to present.
-
66890 EPILEP?/TI,AB,DE
-
133147 (MAGNETIC(W)RESONANCE(W)TOMOGRAPH? OR MAGNETIC(W)RESONANCE(W)SCAN? OR MAGNETIC(W)RESONANCE(W)IMAG? OR MAGNETIC(W)RESONANCE(W)SPECTROSCOP? OR MAGNETOENCEPHALOGRAPH? OR MAGNETIC(W)SOURCE(W)IMAG? OR HIGH(W)DENSITY(W)SCALP(W)EEG OR HIGH(W)DENSITY(W)SCALP(W)ELECTROENCEPHALOGRA? OR HIGH(W)DENSITY(W)VIDEO(W)EEG OR HIGH(W)DENSITY(W)VIDEO(W)ELECTROENCEPHALOGRA? OR HIGH(W)DENSITY(W)EEG OR HIGH(W)DENSITY(W)ELECTROENCEPHALOGRA?)/TI,AB,DE
-
16171 (MR(W)TOMOGRAPH? OR MR(W)SCAN? OR MR(W)IMAG? OR MR(W)SPECTROSCOP?)/TI,AB,DE
-
168752 (FMRI OR MRI OR NMR OR MRS OR MRSI OR HMRS OR “(1)HMRS” OR 1HMRS OR IHMRS OR HMRSI OR MEG OR MSI OR HD-EEG OR HD(W)EEG OR HDEEG)/TI,AB,DE
-
18879 (SPECT OR SINGLE(W)PHOTON(W)EMISSION(W)COMPUTED(W)TOMOGRAPH?)/TI,AB,DE
-
43855 (PET OR POSITRON(W)EMISSION(W)TOMOGRAPH? OR SUBTRACTION(W)ICTAL(W)SPECT OR SUBTRACTION(W)ICTAL(W)SINGLE(W)PHOTON(W)EMISSION(W)COMPUTED(W)TOMOGRAPH? OR SISCOM)/TI,AB,DE
-
3 VOLUMETRIC(W)EXAM?/TI,AB,DE
-
3 QUANTITATIVE(W)T2(W)MEASURE?/TI,AB,DE
-
1344 VOXEL(W)BASED(W)MORPHOMET?/TI,AB,DE
-
2319 (DIFFUSION(W)WEIGHT?(W)IMAG? OR DWI)/TI,AB,DE
-
2854 (DIFFUSION(W)TENSOR(W)IMAG? OR DTI)/TI,AB,DE
-
907 (FLUID(W)ATTENTUATED(W)INVERSION(W)RECOVERY OR FLAIR)/TI,AB,DE
-
279723 S2:S12
-
9942 S1 AND S13
-
630376 (SURGER? OR SURGIC? OR NEUROSURGER? OR NEUROSURGIC? OR LOBECTOM? OR CORTICAL(W)RESECTION OR HEMISPHEROTOM? OR HEMISPHERECTOM? OR CALLOSOTOM? OR CALLOTOM?)/TI,AB,DE
-
3241 S14 AND S15
-
1589884 PY = 2008:2011
-
623 S16 AND S17
Key:
-
? = truncation
-
/TI,AB,DE = terms in title, abstract, or descriptor fields
-
(W) = terms adjacent to each other (same order)
-
PY = publication year
-
: = range e.g. PY = 2008:2011 means year = 2008 OR 2009 OR 2010 OR 2011
Interface used: BIOSIS Previews 1969–2008 (via Web of Knowledge)
-
Search date: 7 September 2010.
-
Records identified: 1164.
-
Restrictions: 2003–8.
-
# 11 1,164 #10 AND #9
-
# 10 > 100,000 TS = (surger* OR surgic* OR neurosurger* OR neurosurgic* OR lobectom* OR hemispherotom* OR hemispherectom* OR callosotom* OR callotom*) OR TS = (“cortical resection*”)
-
# 9 9,168 #8 AND #1
-
# 8 > 100,000 #7 OR #6 OR #5 OR #4 OR #3 OR #2
-
# 7 5,297 TS = (“volumetric exam*”) OR TS = (“quantitative t2 measure*”) OR TS = (“voxel based morphomet*”) OR TS = (“diffusion weight* imag*”) OR TS = (dwi) OR TS = (“diffusion tensor imag*”) OR TS = (dti) OR TS = (“fluid attenuated inversion recovery*”) OR TS = (flair) # 6 45,284 TS = (pet) OR TS = (“positron emission tomograph*”) OR TS = (“subtraction ictal spect”) OR TS = (“subtraction ictal single photon emission computed tomograph*”) OR TS = (siscom)
-
# 5 22,822 TS = (spect) OR TS = (“single photon emission computed tomograph*”)
-
# 4 > 100,000 TS = (fmri OR mri OR nmr OR mrs OR mrsi OR hmrs OR 1hmrs OR ihmrs OR hmrsi OR meg OR msi OR hdeeg) OR TS = (“(1)hmrs”) OR TS = (“hd-eeg”) OR TS = (“hd eeg”)
-
# 3 18,462 TS = (“mr tomograph*”) OR TS = (“mr scan*”) OR TS = (“mr imag*”) OR TS = (“mr spectroscop*”)
-
# 2 > 100,000 TS = (“magnetic resonance tomograph*”) OR TS = (“magnetic resonance scan*”) OR TS = (“magnetic resonance imag*”) OR TS = (“magnetic resonance spectroscop*”) OR TS = (magnetoencephalogra*”) OR TS = (“magnetic source imag*”) OR TS = (“high density scalp eeg”) OR TS = (“high density scalp electroencephalogra*”) OR TS = (“high density video eeg”) OR TS = (“high density electroencephalogra*”) OR TS = (“high density eeg”) OR TS = (high density electroencephalogra*”)
-
# 1 86,724 TS = epilep*
Key:
-
TS = topic tag; searches terms in Title, Abstract, Author Keywords and Keywords Plus fields
-
* = truncation
-
? = embedded truncation
-
“ “ = phrase search
-
near/1 = terms within one word of each other (any order)
-
near/2 = terms within two words of each other (any order)
CDSR (Cochrane Database of Systematic Reviews)
See The Cochrane Library.
CENTRAL (Cochrane Central Register of Controlled Trials)
See The Cochrane Library.
ClinicalTrials.gov
-
Interface used: www.clinicaltrials.gov/.
-
Search date: 31 August 2010.
-
Records identified: 17.
-
surgery OR surgical OR neurosurgery OR neurosurgical
-
AND
-
“Epilepsy”
-
AND
-
magnetic OR magnetoencephalography OR density OR tomography OR electroencephalography OR scan OR scanning OR image OR spect OR pet OR fmri OR mri OR nmr OR mrs OR mrsi OR hmrs OR 1hmrs OR ihmrs OR hmrsi OR meg OR msi OR hd-eeg OR dwi OR dti OR siscom
The Cochrane Library
Interface used: www.thecochranelibrary.com/ Wiley, The Cochrane Library website Issue 7 of 12, July 2010
-
Search date: 30 July 2010.
-
Records identified: 22.
-
Restrictions: 2003 to present.
This search covered the following databases:
-
CENTRAL (Cochrane Central Register of Controlled Trials)
-
CDSR (Cochrane Database of Systematic Reviews)
-
DARE (Database of Abstracts of Reviews of Effectiveness)
-
HTA (Health Technology Assessment database)
-
NHS-EED (NHS Economic Evaluation Database)
-
#1 MeSH descriptor Epilepsy explode all trees (1975)
-
#2 epilep*:ti,ab (3106)
-
#3 (#1 OR #2) (3787)
-
#4 MeSH descriptor Magnetic Resonance Imaging explode all trees (3817)
-
#5 MeSH descriptor Magnetic Resonance Spectroscopy explode all trees (367)
-
#6 MeSH descriptor Magnetoencephalography, this term only (113)
-
#7 ((magnetic NEXT resonance NEXT tomograph*) OR (magnetic NEXT resonance NEXT scan*) OR (magnetic NEXT resonance NEXT imag*) OR (magnetic NEXT resonance NEXT spectroscop*) OR magnetoencephalograph* OR (magnetic NEXT source NEXT imag*) OR (high NEXT density NEXT scalp NEXT eeg) OR (high NEXT density NEXT scalp NEXT electroencephalogra*) OR (high NEXT density NEXT video NEXT eeg) OR (high NEXT density NEXT video NEXT electroencephalogra*) OR (high NEXT density NEXT eeg) OR (high NEXT density NEXT electroencephalogra*)):ti,ab (2734)
-
#8 ((mr NEXT tomograph*) OR (mr NEXT scan*) OR (mr NEXT imag*) OR (mr NEXT spectroscop*)):ti,ab (705)
-
#9 ((fmri OR mri OR mrs OR mrsi OR hmrs OR ihmrs OR hmrsi OR meg OR msi OR hdeeg)):ti,ab (3115)
-
#10 1hmrs:ti,ab (0)
-
#11 “(1)hmrs”:ti,ab (1)
-
#12 “hd-eeg”:ti,ab (0)
-
#13 (hd NEXT eeg):ti,ab (0 )
-
#14 MeSH descriptor Tomography, Emission-Computed explode all trees (2067)
-
#15 (spect OR (single NEXT photon NEXT emission NEXT computed NEXT tomograph*)):ti,ab (948)
-
#16 (pet OR (positron NEXT emission NEXT tomograph*) OR (subtraction NEXT ictal NEXT spect) OR (subtraction NEXT ictal NEXT single NEXT photon NEXT emission NEXT computed NEXT tomography*) OR siscom):ti,ab (1772)
-
#17 (volumetric NEXT exam*):ti,ab (0)
-
#18 (quantitative NEXT t2 NEXT measure*):ti,ab (0)
-
#19 (voxel NEXT based NEXT morphomet*):ti,ab (33)
-
#20 ((diffusion NEXT weight* NEXT imag*) OR dwi):ti,ab (107)
-
#21 ((diffusion NEXT tensor NEXT imag*) OR dti):ti,ab (73)
-
#22 ((fluid NEXT attenuated NEXT inversion NEXT recovery) or flair):ti,ab (29)
-
#23 (#4 OR #5 OR #6 OR #7 OR #8 OR #9 OR #10 OR #11 OR #12 OR #13 OR #14 OR #15 OR #16 OR #17 OR #18 OR #19 OR #20 OR #21 OR #22) (8804)
-
#24 (#3 AND #23) (159)
-
#25 MeSH descriptor Neurosurgical Procedures, this term only (284)
-
#26 MeSH descriptor Anterior Temporal Lobectomy, this term only (14)
-
#27 MeSH descriptor Cerebral Decortication explode all trees (4)
-
#28 MeSH descriptor Craniotomy explode all trees (268)
-
#29 MeSH descriptor Split-Brain Procedure, this term only (1)
-
#30 (surger* OR surgic* OR neurosurger* OR neurosurgic* OR lobectom* OR cortical resection OR hemispherotom* OR hemispherectom* OR callosotom* OR callotom*):ti,ab (59107)
-
#31 (#25 OR #26 OR #27 OR #28 OR #29 OR #30) (59,269)
-
#32 (#24 AND #31), from 2003 to 2010 (22)
Key:
-
MeSH descriptor = indexing term (MeSH heading)
-
* = truncation
-
“ “ = phrase search
-
:ti,ab = terms in either title or abstract fields
-
near/1 = terms within one word of each other (any order)
-
near/2 = terms within two words of each other (any order)
-
next = terms are next to each other.
Current Controlled Trials [metaRegister of Current Controlled Trials (mRCT)]
-
Interface used: www.controlled-trials.com/.
-
Search date: 7 September 2010.
-
Records identified: 96.
-
epilep*
-
AND
-
(magnetic OR magnetoencephalography OR density OR tomography OR electroencephalography OR scan OR scanning OR image)
-
AND
-
(surger* OR surgic*)
-
96 results
DARE (Database of Abstracts of Reviews of Effects)
See The Cochrane Library.
EMBASE
Interface used: Ovid EMBASE – 1980 to 2010 week 28
-
Search date: 23 July 2010.
-
Records identified: 3015.
-
Restrictions: 2003 to present.
-
exp Epilepsy/ (124,629)
-
epilep$.ti,ab. (95,124)
-
1 or 2 (139620)
-
exp Nuclear Magnetic Resonance Imaging/ (339,416)
-
exp Nuclear Magnetic Resonance Spectroscopy/ (74,276)
-
Magnetoencephalography/ (5369)
-
(magnetic resonance tomograph$ or magnetic resonance scan$ or magnetic resonance imag$ or magnetic resonance spectroscop$ or magnetoencephalograph$ or magnetic source imag$ or high density scalp eeg or high density scalp electroencephalogra$ or high density video eeg or high density video electroencephalogra$ or high density eeg or high density electroencephalogra$).ti,ab. (128608)
-
(mr tomograph$ or mr scan$ or mr imag$ or mr spectroscop$).ti,ab. (44,000)
-
(fmri or mri or nmr or mrs or mrsi or hmrs or “(1)hmrs” or 1hmrs or ihmrs or hmrsi or meg or msi or hd-eeg or hd eeg or hdeeg).ti,ab. (252,652)
-
Computer Assisted Emission Tomography/ (9435)
-
Positron Emission Tomography/ (54,025)
-
(spect or single photon emission computed tomograph$).ti,ab. (23,752)
-
(pet or positron emission tomograph$ or subtraction ictal spect or subtraction ictal single photon emission computed tomograph$ or siscom).ti,ab. (57,202)
-
volumetric exam$.ti,ab. (11)
-
quantitative t2 measure$.ti,ab. (11)
-
voxel based morphomet$.ti,ab. (1543)
-
(diffusion weight$ imag$ or dwi).ti,ab. (5092)
-
(diffusion tensor imag$ or dti).ti,ab. (4233)
-
(fluid attenuated inversion recovery or flair).ti,ab. (2634)
-
or/4-19 (599,154)
-
3 and 20 (18,633)
-
Neurosurgery/ (32,497)
-
Temporal Lobectomy/ (2193)
-
Decortication/ (1356)
-
Hemispherectomy/ (825)
-
Craniotomy/ (13,211)
-
Split-Brain/ (286)
-
(surger$ or surgic$ or neurosurger$ or neurosurgic$ or lobectom$ or cortical resection or hemispherotom$ or hemispherectom$ or callosotom$ or callotom$).ti,ab. (1,168,161)
-
or/22-28 (1,189,172)
-
21 and 29 (5147)
-
exp Animal/ not (Human/ and exp Animal/) (1,235,605)
-
30 not 31 (5145)
-
limit 32 to yr = “2003 -Current” (3015)
Key:
-
/ = indexing term (EMTREE heading)
-
exp = exploded EMTREE heading
-
$ = truncation
-
? = embedded truncation
-
.ti,ab. = terms in either title or abstract fields.
Conversion of thesaurus headings from MeSH to EMTREE
MEDLINE | EMBASE |
exp Magnetic Resonance Imaging/ | exp Nuclear Magnetic Resonance Imaging/ |
exp Magnetic Resonance Spectroscopy/ | exp Nuclear Magnetic Resonance Spectroscopy/ |
exp Tomography, Emission-Computed/ | exp Computer Assisted Emission Tomography/ |
[Captured within Tomography, Emission –Computed in MEDLINE] | Positron Emission Tomography/ |
Neurosurgical Procedures/ | Neurosurgery/ |
Anterior Temporal Lobectomy/ | Temporal Lobectomy/ |
exp Cerebral Decortication/ | exp Decortication/ |
[Captured within Cerebral Decortication in MEDLINE] | Hemispherectomy/ |
Craniotomy | Craniotomy |
Split-Brain Procedure/ | Split-Brain/ |
HTA (Health Technology Assessment) database
See The Cochrane Library.
Inside Conferences
Interface used: DialogClassic Web FILE 65: Inside Conferences –1993–2010/September 2006 (c) 2010 BLDSC all rts reserv.
-
Search date: 7 September 2010.
-
Records identified: 15.
-
Restrictions: 2003 to present.
-
6064 EPILEP?/TI,AB,DE
-
4100 (MAGNETIC(W)RESONANCE(W)TOMOGRAPH? OR MAGNETIC(W)RESONANCE(W)SCAN? OR MAGNETIC(W)RESONANCE(W)IMAG? OR MAGNETIC(W)RESONANCE(W)SPECTROSCOP? OR MAGNETOENCEPHALOGRAPH? OR MAGNETIC(W)SOURCE(W)IMAG? OR HIGH(W)DENSITY(W)SCALP(W)EEG OR HIGH(W)DENSITY(W)SCALP(W)ELECTROENCEPHALOGRA? OR HIGH(W)DENSITY(W)VIDEO(W)EEG OR HIGH(W)DENSITY(W)VIDEO(W)ELECTROENCEPHALOGRA? OR HIGH(W)DENSITY(W)EEG OR HIGH(W)DENSITY(W)ELECTROENCEPHALOGRA?)/TI,AB,DE
-
1918 (MR(W)TOMOGRAPH? OR MR(W)SCAN? OR MR(W)IMAG? OR MR(W)SPECTROSCOP?)/TI,AB,DE
-
58296 (FMRI OR MRI OR NMR OR MRS OR MRSI OR HMRS OR “(1)HMRS” OR 1HMRS OR IHMRS OR HMRSI OR MEG OR MSI OR HD-EEG OR HD(W)EEG OR HDEEG)/TI,AB,DE
-
2185 (SPECT OR SINGLE(W)PHOTON(W)EMISSION(W)COMPUTED(W)TOMOGRAPH?)/TI,AB,DE
-
8857 (PET OR POSITRON(W)EMISSION(W)TOMOGRAPH? OR SUBTRACTION(W)ICTAL(W)SPECT OR SUBTRACTION(W)ICTAL(W)SINGLE(W)PHOTON(W)EMISSION(W)COMPUTED(W)TOMOGRAPH? OR SISCOM)/TI,AB,DE
-
0 VOLUMETRIC(W)EXAM?/TI,AB,DE
-
0 QUANTITATIVE(W)T2(W)MEASURE?/TI,AB,DE
-
15 VOXEL(W)BASED(W)MORPHOMET?/TI,AB,DE
-
236 (DIFFUSION(W)WEIGHT?(W)IMAG? OR DWI)/TI,AB,DE
-
463 (DIFFUSION(W)TENSOR(W)IMAG? OR DTI)/TI,AB,DE
-
161 (FLUID(W)ATTENTUATED(W)INVERSION(W)RECOVERY OR FLAIR)/TI,AB,DE
-
74927 S2:S12
-
489 S1 AND S13
-
65503 (SURGER? OR SURGIC? OR NEUROSURGER? OR NEUROSURGIC? OR LOBECTOM? OR CORTICAL(W)RESECTION OR HEMISPHEROTOM? OR HEMISPHERECTOM? OR CALLOSOTOM? OR CALLOTOM?)/TI,AB,DE
-
58 S14 AND S15
-
2658053 PY = 2003:2011
-
15 S16 AND S17
Key:
-
? = truncation
-
/TI,AB,DE = terms in title, abstract, or descriptor fields
-
(W) = terms adjacent to each other (same order)
-
PY = publication year
-
: = range e.g. PY = 2008:2011 means year = 2008 OR 2009 OR 2010 OR 2011.
LILACS (Latin American and Caribbean Health Sciences Literature)
-
Interface used: http://tinyurl.com/39hn6e.
-
Search date: 30 July 2010.
-
Records identified: 66.
(“MAGNETIC RESONANCE spectroscopy” ) or (“MAGNETIC RESONANCE IMAGING”) or (“magnetoencephalography” ) or (“computerized emission TOMOGRAPHY”) or (“positron-emission TOMOGRAPHY”)
AND
“Epilepsy”
Medion (systematic reviews of diagnostic studies database)
-
Interface used: www.mediondatabase.nl/.
-
Search date: 30 July 2010.
-
Records identified: 4.
-
Epilepsy in title or abstract (4).
NHS EED (NHS Economic Evaluations Database)
See The Cochrane Library.
PASCAL
-
Interface used: DialogClassic Web FILE 144: Pascal_1973-2010/Aug W5 (c) 2010 INIST/CNRS.
-
Search date: 7 September 2010.
-
Records identified: 669.
-
Restrictions: 2003 to present.
-
45951 EPILEP?/TI,AB,DE
-
111046 (MAGNETIC(W)RESONANCE(W)TOMOGRAPH? OR MAGNETIC(W)RESONANCE(W)SCAN? OR MAGNETIC(W)RESONANCE(W)IMAG? OR MAGNETIC(W)RESONANCE(W)SPECTROSCOP? OR MAGNETOENCEPHALOGRAPH? OR MAGNETIC(W)SOURCE(W)IMAG? OR HIGH(W)DENSITY(W)SCALP(W)EEG OR HIGH(W)DENSITY(W)SCALP(W)ELECTROENCEPHALOGRA? OR HIGH(W)DENSITY(W)VIDEO(W)EEG OR HIGH(W)DENSITY(W)VIDEO(W)ELECTROENCEPHALOGRA? OR HIGH(W)DENSITY(W)EEG OR HIGH(W)DENSITY(W)ELECTROENCEPHALOGRA?)/TI,AB,DE
-
20020 (MR(W)TOMOGRAPH? OR MR(W)SCAN? OR MR(W)IMAG? OR MR(W)SPECTROSCOP?)/TI,AB,DE
-
256479 (FMRI OR MRI OR NMR OR MRS OR MRSI OR HMRS OR “(1)HMRS” OR 1HMRS OR IHMRS OR HMRSI OR MEG OR MSI OR HD-EEG OR HD(W)EEG OR HDEEG)/TI,AB,DE
-
9203 (SPECT OR SINGLE(W)PHOTON(W)EMISSION(W)COMPUTED(W)TOMOGRAPH?)/TI,AB,DE
-
34417 (PET OR POSITRON(W)EMISSION(W)TOMOGRAPH? OR SUBTRACTION(W)ICTAL(W)SPECT OR SUBTRACTION(W)ICTAL(W)SINGLE(W)PHOTON(W)EMISSION(W)COMPUTED(W)TOMOGRAPH? OR SISCOM)/TI,AB,DE
-
2 VOLUMETRIC(W)EXAM?/TI,AB,DE
-
2 QUANTITATIVE(W)T2(W)MEASURE?/TI,AB,DE
-
646 VOXEL(W)BASED(W)MORPHOMET?/TI,AB,DE
-
1876 (DIFFUSION(W)WEIGHT?(W)IMAG? OR
-
DWI)/TI,AB,DE
-
1545 (DIFFUSION(W)TENSOR(W)IMAG? OR
-
DTI)/TI,AB,DE
-
862 (FLUID(W)ATTENTUATED(W)INVERSION(W)RECOVERY
-
OR FLAIR)/TI,AB,DE
-
359738 S2:S12
-
4720 S1 AND S13
-
753052 (SURGER? OR SURGIC? OR NEUROSURGER? OR NEUROSURGIC? OR LOBECTOM? OR CORTICAL(W)RESECTION OR HEMISPHEROTOM? OR HEMISPHERECTOM? OR CALLOSOTOM? OR CALLOTOM?)/TI,AB,DE
-
1563 S14 AND S15
-
3673318 PY = 2003:2011
-
669 S16 AND S17
Key:
-
? = truncation
-
/TI,AB,DE = terms in title, abstract, or descriptor fields
-
(W) = terms adjacent to each other (same order)
-
PY = publication year
-
: = range e.g. PY = 2008:2011 means year = 2008 OR 2009 OR 2010 OR 2011.
SCI (Science Citation Index)
-
Interface used: Web of Knowledge: Science Citation Index Expanded (SCIE).
-
Search date: 30 July 2010.
-
Records identified: 1786.
-
Restrictions: 2003 to present.
-
# 11 (1,786) #10 AND #9
-
# 10 (> 100,000) TS = (surger* OR surgic* OR neurosurger* OR neurosurgic* OR lobectom* OR hemispherotom* OR hemispherectom* OR callosotom* OR callotom*) OR TS = (“cortical resection*”)
-
# 9 (5,616) #8 AND #1
-
# 8 (> 100,000) #7 OR #6 OR #5 OR #4 OR #3 OR #2
-
# 7 (9,684) TS = (“volumetric exam*”) OR TS = (“quantitative t2 measure*”) OR TS = (“voxel based morphomet*”) OR TS = (“diffusion weight* imag*”) OR TS = (dwi) OR TS = (“diffusion tensor imag*”) OR TS = (dti) OR TS = (“fluid attenuated inversion recovery*”) OR TS = (flair) # 6 (47,612) TS = (pet) OR TS = (“positron emission tomograph*”) OR TS = (“subtraction ictal spect”) OR TS = (“subtraction ictal single photon emission computed tomograph*”) OR TS = (siscom)
-
# 5 (11,163) TS = (spect) OR TS = (“single photon emission computed tomograph*”)
-
# 4 > 100,000 TS = (fmri OR mri OR nmr OR mrs OR mrsi OR hmrs OR 1hmrs OR ihmrs OR hmrsi OR meg OR msi OR hdeeg) OR TS = (“(1)hmrs”) OR TS = (“hd-eeg”) OR TS = (“hd eeg”)
-
# 3 (18,585) TS = (“mr tomograph*”) OR TS = (“mr scan*”) OR TS = (“mr imag*”) OR TS = (“mr spectroscop*”)
-
# 2 (66,305) TS = (“magnetic resonance tomograph*”) OR TS = (“magnetic resonance scan*”) OR TS = (“magnetic resonance imag*”) OR TS = (“magnetic resonance spectroscop*”) OR TS = (magnetoencephalogra*”) OR TS = (“magnetic source imag*”) OR TS = (“high density scalp eeg”) OR TS = (“high density scalp electroencephalogra*”) OR TS = (“high density video eeg”) OR TS = (“high density electroencephalogra*”) OR TS = (“high density eeg”) OR TS = (high density electroencephalogra*”)
-
# 1 (41,584) TS = epilep*
Key:
-
TS = topic tag; searches terms in Title, Abstract, Author Keywords and Keywords Plus fields
-
* = truncation
-
? = embedded truncation
-
“ “ = phrase search.
World Health Organization ICTRP (International Clinical Trials Registry Platform)
Interface used: http://apps.who.int/trialsearch/AdvSearch.aspx
-
Search date: 7 September 2010.
-
Records identified: 7.
-
Epilepsy [in condition field]
-
AND
-
(magnetic OR magnetoencephalography OR density OR tomography OR electroencephalography OR scan OR scanning OR image) [in intervention field]
Appendix 2 Results of, and guidelines for, the quality assessment
Author/year | Criterion | |||||||||||||||
---|---|---|---|---|---|---|---|---|---|---|---|---|---|---|---|---|
1. Patient spectrum representative of clinical practice? | 2a. Selection criteria described clearly? | 2b. Prognostic factors described clearly and in full? | 3a. Index test described in sufficient detail to permit replication? | 3b. Reference test(s)/surgical procedure described in sufficient detail to permit replication? | 4a. Index test interpreted independently? | 4b. Reference standard test(s) interpreted/site of surgery decided independently? | 5. Same clinical data available? | 6. Outcome assessment blinded to exposure status? | 7. Duration of follow-up adequate? | 8. Uninterpretable/intermediate results reported? | 9. Withdrawals reported/explained? | 10. Dropouts similar across groups? | 11. Test reliably evaluated? | 12. Groups comparable on all important confounding factors? | 13. Adequate adjustment for confounding factors? | |
Achten (1997)55 | N | Y | N/A | Y | Y | Y | Y | UC | UC | N | Y | Y | N/A | N/A | N/A | N/A |
Antel (2002)60 | N | N/A | N | Y | N | UC | UC | UC | UC | N | UC | Y | Y | Y | UC | N |
Assaf (2004)63 | N | Y | N/A | Y | UC | UC | UC | UC | UC | Y | UC | Y | N/A | N/A | N/A | N/A |
Didelot (2008)58 | N | Y | N/A | Y | Y | Y | UC | N | UC | N | Y | Y | N/A | N/A | N/A | N/A |
Dupont (2000)70 | N | N/A | N | Y | Y | UC | N | UC | UC | Y | Y | Y | Y | Y | UC | N |
Kaiboriboon (2010)64 | N | Y | N/A | N | Y | UC | Y | UC | UC | Y | N | Y | N/A | N/A | N/A | N/A |
Knowlton (1997)56 | N | Y | N | Y | Y | Y/N | Y | Y/N | UC | Y | Y | Y | UC | Y | UC | N |
Knowlton (2008)49 | N | Y | N | Y | Y | N | N | UC | Y | Y | UC | Y | UC | Y | UC | N |
Lee (2002) (first SPECT)57 | N | Y | N/A | Y | N | N | UC | N | UC | Y | Y | Y | N/A | N/A | N/A | N/A |
Lee (2002) (second SPECT)57 | N | Y | N/A | Y | N | Y | UC | N | UC | Y | Y | Y | N/A | N/A | N/A | N/A |
Lee (2005)65 | Y | Y | N/A | Y | Y | UC | UC | UC | UC | Y | UC | Y | N/A | N/A | N/A | N/A |
Lewis (1998)61 | Y | N | N/A | Y | Y | UC | UC | N | UC | Y | Y | Y | N/A | N/A | N/A | N/A |
O’Brien (1999)67 | N | N/A | N | Y | N | Y | Y | N | UC | Y | UC | Y | UC | Y | UC | N |
O’Brien (2001)62 | N | N/A | N | Y | N | Y | N | N | UC | N | UC | Y | UC | Y | UC | N |
Smith (1995)69 | UC | N | N/A | Y | N | UC | N | UC | UC | N | Y | Y | N/A | N/A | N/A | N/A |
Theodore (1997)68 | N | Y | N | Y | Y | Y | Y | N | UC | Y | UC | Y | UC | Y | UC | N |
Uijl (2007)59 | N | Y | N/A | Y | Y | Y | N | Y | UC | Y | Y | Y | Y | N/A | N/A | N/A |
Wheless (1999)42 | N | Y | N/A | Y | Y | UC | N | UC | UC | N | Y | Y | N/A | N/A | N/A | N/A |
Wu (2010)66 | N | Y | N/A | N | N | UC | N | Y | UC | Y | Y | Y | N/A | N/A | N/A | N/A |
Guidelines for completing the quality assessment
1. Was the spectrum of patients representative of the full range of patients who will receive the test in practice? Yes: Unselected prospective patients with refractory epilepsy being considered for surgery; no restrictions in terms of age, gender or type of epilepsy No: All other patient spectra including a retrospectively selected patient population Unclear: Insufficient details were provided to make a judgement as to whether the patient spectrum was representative |
2a. Were selection criteria clearly described in diagnostic accuracy/decision papers? 2b. Were there sufficient description of the groups and the distribution of prognostic factors in the outcome prediction papers? Yes: Enough details were provided of how patients were selected so that the selection process could be replicated No: Insufficient details were presented to allow replication of the selection process Not applicable: 2a, outcome prediction papers; 2b, diagnostic accuracy/decision papers |
3a. Was the execution of the index test described in sufficient detail to permit replication of the test? 3b. Was the execution of the comparator tests/surgical procedure described in sufficient detail to permit its replication? Yes: Sufficient details of the execution of the test were reported to allow replication No: Insufficient details reported to allow replication |
4a. Were index tests results interpreted without knowledge of the results of the reference standard? 4b. Were the comparator test results interpreted/site of surgery decided without knowledge of the results of the index test? Yes: The index test was assessed without knowledge of the results of the combination of tests used in the decision for surgery or the site at which surgery was to be conducted, and vice versa No: The person evaluating the index test knew the result of the tests used for decision for surgery, the site where surgery was to be conducted or the outcome following surgery, and vice versa Unclear: No information was provided regarding whether tests were interpreted blindly |
5. Were the same clinical data available when test results were interpreted as would be available when the test is used in practice? Yes: The interpreter of the index test had access to the results of any clinical information other than the MRI and EEG No: Such information was not available Unclear: No information was provided regarding whether the results of these tests were available |
6. Was outcome assessment blind to exposure status? Yes: Outcome following surgery was assessed blinded to the results of the test evaluations No: Outcome assessment was not blinded Unclear: The authors did not report on blinding |
7. Was follow-up long enough for the outcome to occur? Yes: Duration of post-surgical follow-up was > 12 months in all patients No: Duration of follow-up was < 12 months in at least some patients Unclear: Duration of follow-up following surgery was not reported |
8. Were uninterpretable/intermediate results reported? Yes: Details were provided on uninterpretable/intermediate test results No: Details were not provided on uninterpretable/intermediate test results Unclear: It was not clear whether there were any uninterpretable/intermediate test results |
9. Were withdrawals from the study reported/explained? Yes: All patients recruited into the study were accounted for No: There appeared to be patients who were recruited who were not accounted for Unclear: It was not clear whether any withdrawals occurred |
10. Were dropout rate and reasons for dropout similar across exposure groups? Yes: Dropout rates/reasons for withdrawal similar across exposure groups No: Dropout rates/reasons for withdrawal not similar across exposure groups Unclear: Dropout rates/reasons for withdrawal were not reported |
11. Was the test reliably evaluated? Yes: Details of test execution were reported, definition of an exposed (positive) results defined, and images were assessed blind to outcome following surgery No: One or more of the above was not fulfilled Unclear: It was unclear whether images were assessed blind to outcome following surgery but test details and definition of a positive result were reported Not applicable: This criterion was applied to the outcome prediction studies only |
12. Were the groups comparable on all important confounding factors? Yes: The studies reported on: febrile seizures, mesial temporal sclerosis, EEG/MRI concordance and extent of surgical excision, and groups were comparable with respect to these factors No: Groups were not comparable with respect to one or more of the potential confounding factors above Unclear: The distribution of these factors between groups was not reported Not applicable: This criterion was applied to the outcome prediction studies only |
13. Was there adequate adjustment for the effects of all important confounding factors? Yes: All confounding factors listed above were adjusted for in the analysis No: One or more of the factors were not considered in the analysis Unclear: It was unclear whether these factors were included in the analysis Not applicable: This criterion was applied to the outcome prediction studies only |
Appendix 3 Data extraction tables
Diagnostic accuracy studies
Study details
Study | Population | Test details | Surgery details |
---|---|---|---|
Achten (1997) 55 Country/region: Western Europe Direction of data collection: Prospective Was IPD reported? Yes For how many patients? 29 (100%) |
No. recruited (no. male): 29 (11) Mean age: 30.2 years (range: 14–53 years) Adults or children? Both Mean duration of epilepsy: NR Type of epilepsy: HS: 17 Mesotemporal lobe epilepsy: 10 Hippocampal atrophy: 1 Hippocampal damage: 1 Patients with or without a focus identified on MRI/EEG? Both |
Reference standard(s): Decision to go to surgery Outcome following surgery Definition(s) of a good surgical outcome: Engel I and II No. who underwent iEEG: 2 No. refusing iEEG: NR Index test(s) details: MRS (timing unclear) NAA/(Cho + Cr) MRI tesla strength: 1.5 T vMRI (timing unclear) MRI tesla strength: 1.5 T Interictal FDG-PET No. of patients with test-related adverse events: NR |
Eligibility for surgery: Eligible for surgery: 20 Underwent surgery: 17 Refused surgery: 1 Ineligible for surgery: 9 Unaccounted for: 0 Type of surgery performed: Temporal lobectomy (specific structures resected NR):17 Two patients awaiting surgery Postoperative follow-up: Range < 6 months to 36 months |
Assaf (2004) 63 Country/region: USA/Canada Direction of data collection: Prospective Was IPD reported? No |
No. recruited (no. male): 26 (NR) Mean age: NR Adults or children? NR Mean duration of epilepsy: NR Type of epilepsy: MTS: 19 Temporal (undefined): 7 Patients with or without a focus identified on MRI/EEG? Both |
Reference standard(s): Outcome following surgery Definition(s) of a good surgical outcome: SF No. who underwent iEEG: 7 No. refusing iEEG: NR Index test(s) details: Interictal MEG No. of patients with test-related adverse events: NR |
Eligibility for surgery: Eligible for surgery: 22 Underwent surgery: 22 Refused surgery: 1 Ineligible for surgery: 3 Unaccounted for: 0 Type of surgery performed: ATL: 14 SAH: 5 Lateral temporal neocortical resection: 3 Postoperative follow-up: 2 years |
Didelot (2008) 58 Country/region: Western Europe Direction of data collection: Prospective Was IPD reported? Yes For how many patients? 42 (100%) |
No. recruited (no. male): 42 (21) Mean age: 35.4 years (range: 17–61 years) Adults or children? Both Mean duration of epilepsy: 21 years (SD: 9.5 years; range: 5–54.5 years) Type of epilepsy: MTS: 32 (HS: 25) Temporal (undefined): 10 Patients with or without a focus identified on MRI/EEG? Both |
Reference standard(s): Decision to go to surgery Outcome following surgery Definition(s) of a good surgical outcome: Engel IA Engel I Engel I and II No. who underwent iEEG: 18 No. refusing iEEG: 2 Index test(s) details: FDG-PET (timing unclear) No. of patients with test-related adverse events: NR |
Eligibility for surgery: Eligible for surgery: 37 Underwent surgery: 27 Refused surgery: 10 Ineligible for surgery: 4 Unaccounted for: 1 Type of surgery performed: ATL: 22 SAH: 1 Selective corticectomy: 4 Postoperative follow-up: Mean 25 months (range 7–48 months) |
Kaiboriboon (2010) 64 Country/region: USA/Canada Direction of data collection: Prospective Was IPD reported? Yes For how many patients? 22 (88%) |
No. recruited (no. male): 25 (NR) Mean age: 42.8 years (range: 24–68 years) Adults or children? Adults Mean duration of epilepsy: NR Type of epilepsy: MTS: 25 Patients with or without a focus identified on MRI/EEG? Both |
Reference standard(s): Outcome following surgery Definition(s) of a good surgical outcome: Engel IA Engel I Engel I and II No. who underwent iEEG: NR No. refusing iEEG: NR Index test(s) details: MSI (timing unclear) MRI tesla strength: NR No. of patients with test-related adverse events: NR |
Eligibility for surgery: Eligible for surgery: 22 Underwent surgery: 22 Refused surgery: 0 Ineligible for surgery: Unclear Unaccounted for: 0 Three patients had unreliable spike detection and localisation and were therefore excluded from further analyses; it is unclear whether or not they were eligible for surgery Type of surgery performed: ATL: 22 Postoperative follow-up: 1 year |
Knowlton (1997) 56 Country/region: USA/Canada Direction of data collection: Prospective Was IPD reported? Yes For how many patients? 23 (92%) |
No. recruited (no. male): 25 (12) Mean age: 38 years (range: 14–56 years) Adults or children? Both Mean duration of epilepsy: NR Type of epilepsy: Temporal (undefined): 10 Hippocampal atrophy: 15 Patients with or without a focus identified on MRI/EEG? Both |
Reference standard(s): Outcome following surgery Definition(s) of a good surgical outcome: Engel I Engel I and II No. who underwent iEEG: 10 No. refusing iEEG: NR The 10 patients undergoing iEEG are from the original population of 36 patients; 12 patients were subsequently excluded due to tumours, vascular malformations or dysembryoplastic lesions, or did not receive all investigations Index test(s) details: FDG-PET (timing unclear) MRS (timing unclear) NAA/(Cho + Cr) MRI tesla strength: 1.5 T No. of patients with test-related adverse events: NR |
Eligibility for surgery: Eligible for surgery: 24 Underwent surgery: 24 Refused surgery: 0 Ineligible for surgery: 1 Unaccounted for: 0 Type of surgery performed: ATL: 24 SAH: 24 Postoperative follow-up: Mean 23 months (range 18–31 months) |
Knowlton (2008) 49 Country/region: USA/Canada Direction of data collection: Prospective Was IPD reported? No |
No. recruited (no. male): 62 (33) A total of 72 patients were in the cohort, but 10 patients were not localised using iEEG and were therefore not included in the analysis Mean age: 26 years (range 1–60 years) Adults or children? Both Mean duration of epilepsy: NR Type of epilepsy: MTS: 21 Lateral temporal (undefined): 9 Extratemporal (undefined): 29 Not localised: 3 Patients with or without a focus identified on MRI/EEG? Both |
Reference standard(s): Outcome following surgery Definition(s) of a good surgical outcome: Engel I No. who underwent iEEG: 62 No. refusing iEEG: 0 Index test(s) details: MSI (timing unclear) MRI tesla strength: NR Ictal HMPAO-SPECT FDG- PET (timing unclear) Tests results also combined, but only in the multivariate analysis; data for 2 x 4 contingency tables were not reported for consensus decisions MSI + PET MSI + SPECT MSI + PET + SPECT No. of patients with test-related adverse events: NR |
Eligibility for surgery: Eligible for surgery: 62 Underwent surgery: 62 Refused surgery: 0 Ineligible for surgery: 0 Unaccounted for: 0 Type of surgery performed: NR Postoperative follow-up: Mean 3.4 years, minimum 1 year; upper range NR |
Lee (2002) 57 Country/region: Asia Direction of data collection: Prospective Was IPD reported? Yes For how many patients? 24 (100%) |
No. recruited (no. male): 24 (9) Mean age: 19.2 years (range 5–35 years) Adults or children? Both Mean duration of epilepsy: Approximately 10 years (range < 1 year to 27 years) Type of epilepsy: MTS: 2 Frontal: 8 Parietal: 3 Occipital: 1 Temporal occipital: 1 Lateral temporal: 1 Multifocal: 8 Patients with or without a focus identified on MRI/EEG? Both |
Reference standard(s): Decision to go to surgery Outcome following surgery Definition(s) of a good surgical outcome: Engel I and II No. who underwent iEEG: No. refusing iEEG: Index test(s) details: Repeated ictal HMPAO-SPECT Results were extracted for the first and second SPECT scans separately then the first and second SPECT combined Insufficient numbers of patients had a third and fourth scan for data to be extracted No. of patients with test-related adverse events: NR |
Eligibility for surgery: Eligible for surgery: 18 Underwent surgery: 18 Refused surgery: 0 Ineligible for surgery: 6 Unaccounted for: 0 Type of surgery performed: ATL: 2 Frontal: 7 Parietal: 3 Occipital: 1 Temporal occipital: 1 Temporal frontal parietal: 1 Temporal parietal: 1 ATL + temporal neocortical: 1 ATL + frontal resection:1 Postoperative follow-up: Range 20–81 months |
Lee (2005) 65 Country/region: Asia Direction of data collection: Prospective Was IPD reported? No |
No. recruited (no. male): 84 (NR) Mean age: NR Adults or children? NR Mean duration of epilepsy: NR Type of epilepsy: MTS: 41 (HS: 41) Temporal (undefined): 22 Frontal: 13 Parietal: 3 Occipital: 5 Three were excluded from the neocortical group (unclear if excluded from 46 or 43 patients – surgery reported for 43) Unclassified in paper 2 (or 5 if the three were excluded to produce a group of 43) Patients with or without a focus identified on MRI/EEG? Both |
Reference standard(s): Outcome following surgery Definition(s) of a good surgical outcome: SF No. who underwent iEEG: 28 No. refusing iEEG: NR iEEG used for all patients with a negative MRI Index test(s) details: MRS (timing unclear) NAA/Cho MRI tesla strength: 1.5 T No. of patients with test-related adverse events: NR |
Eligibility for surgery: Eligible for surgery: 84 Underwent surgery: 84 Refused surgery: 0 Ineligible for surgery: 0 Unaccounted for: 0 Type of surgery performed: ATL: 84 Postoperative follow-up: At least 2 years (range NR) |
Lewis (1998) 61 Country/region: USA/Canada Direction of data collection: Prospective Was IPD reported? Yes For how many patients? 35 (100%) |
No. recruited (no. male): 35 (18) Mean age: 31 years (range 14–69 years) Adults or children? Both Mean duration of epilepsy: NR Type of epilepsy: NR Patients with or without a focus identified on MRI/EEG? Both |
Reference standard(s): Decision to go to surgery Outcome following surgery Definition(s) of a good surgical outcome: SF SF/significant improvement/worthwhile improvement No. who underwent iEEG: 0 No. refusing iEEG: 0 Index test(s) details: Interictal HMPAO (17 patients) or ECD (18 patients) SPECT No. of patients with test-related adverse events: NR |
Eligibility for surgery: Eligible for surgery: 23 Underwent surgery: 23 Refused surgery: 0 Ineligible for surgery: 11 Unaccounted for: 1 (lost to follow-up) Two patients had surgery but outcome was not available Five patients were excluded by CRD as they had tumours or other structural lesions Type of surgery performed: Temporal lobe: 15 Temporal occipital lobe: 1 Frontal lobe: 6 Corpus callosotomy: 1 MM: 11 Postoperative follow-up: Range 12 months to 3 years |
Smith (1995) 69 Country/region: USA/Canada Direction of data collection: Prospective Was IPD reported? No |
No. recruited (no. male): 50 (NR) Mean age: NR Adults or children? NR Mean duration of epilepsy: NR Type of epilepsy: MTS: 18 Lateral neocortical: 20 Orbitofrontal: 3 Orbitofrontal anteromesial temporal: 1 Not localised: 8 Patients with or without a focus identified on MRI/EEG? Both |
Reference standard(s): Outcome following surgery Definition(s) of a good surgical outcome: SF SF/rare DSs Over 90% reduction in seizures No. who underwent iEEG: 13 No. refusing iEEG: NR Index test(s) details: Interictal MEG No. of patients with test-related adverse events: NR |
Eligibility for surgery: Eligible for surgery: NR Underwent surgery: 32 Refused surgery: NR Ineligible for surgery: 8 Unaccounted for: 10 Type of surgery performed: ATL: 15 Orbitofrontal with or without ATL: 4 Convexity: 13 Of 18 with no surgical results reported, eight had insufficient localising information (therefore presumed to be ineligible for surgery). The reason for surgery not being undertaken in the other 10 is difficult to determine Postoperative follow-up: Mean 12.2 months (range 3–23 months) |
Theodore (1997) 68 Country/region: USA/Canada Direction of data collection: Prospective Was IPD reported? No |
No. recruited (no. male): 46 (NR) Mean age: NR Adults or children? NR Mean duration of epilepsy: NR Type of epilepsy: TLE: 26 Frontal: 1 Frontal temporal: 5 PET negative and focus NR: 14 Patients with or without a focus identified on MRI/EEG? Without |
Reference standard(s): Decision to go to surgery Outcome following surgery Definition(s) of a good surgical outcome: SF No. who underwent iEEG: 46 No. refusing iEEG: 0 Index test(s) details: FDG-PET (timing unclear) No. of patients with test-related adverse events: NR |
Eligibility for surgery: Eligible for surgery: 34 Underwent surgery: 34 Refused surgery: 0 Ineligible for surgery: 12 Unaccounted for: 0 Type of surgery performed: Temporal lobectomy, including resection of the lateral and mesial structures: 34 Postoperative follow-up: Mean 67.7 months (range 12–113 months) |
Wheless (1999) 42 Country/region: USA/Canada Direction of data collection: Prospective Was IPD reported? No |
No. recruited (no. male): 58 (23) The 58 patients are from a prospective study of 115 patients who underwent surgery; the other 57 either did not have all of the tests or did not undergo surgery Mean age: 26.7 years (range 7–55 years) Adults or children? Both Mean duration of epilepsy: 17.1 years (range 2–43 years) Type of epilepsy: MTS: 27 Extratemporal (undefined): 17 Posterior lateral temporal: 6 Mesial and lateral temporal: 2 Mixed temporal and extratemporal focus: 6 Patients with or without a focus identified on MRI/EEG? With |
Reference standard(s): Outcome following surgery Definition(s) of a good surgical outcome: SF or rare seizures Good surgical outcome (not defined further) No. who underwent iEEG: 45 No. refusing iEEG: NR Index test(s) details: MEG (timing unclear) No. of patients with test-related adverse events: NR |
Eligibility for surgery: Eligible for surgery: 58 Underwent surgery: 58 Refused surgery: 0 Ineligible for surgery: 0 Unaccounted for: 0 Type of surgery performed: NR Postoperative follow-up: Range 6–46 months |
Wu (2010) 66 Country/region: USA/Canada Direction of data collection: Prospective Was IPD reported? Yes For how many patients? 28 (100%) |
No. recruited (no. male): 28 (14) Mean age: 5.5 years (range 9 months to 17 years) Adults or children? Children Mean duration of epilepsy: (range 0.8–15.8 years) Type of epilepsy: The type of epilepsy suspected was not reported – only whether or not a focus was localised and the actual site of resection Patients with or without a focus identified on MRI/EEG? Both Two children had prior resection |
Reference standard(s): Decision to go to surgery Outcome following surgery Definition(s) of a good surgical outcome: SF No data were available for contingency table for outcome following surgery; only decision to go to surgery extracted No. who underwent iEEG: NR No. refusing iEEG: NR Index test(s) details: FDG-PET coregistered to MRI (timing unclear) MRI tesla strength: 1.5 T No. of patients with test-related adverse events: NR |
Eligibility for surgery: Eligible for surgery: 19 Underwent surgery: 18 Refused surgery: 1 Ineligible for surgery: 9 Unaccounted for: 0 Type of surgery performed: Hemispherectomy: 1 Temporal parietal occipital: 2 Temporal parietal: 4 Frontal central: 2 Temporal (unspecified): 5 Parietal: 3 Frontal: 1 Postoperative follow-up: Mean 4.1 years (SD 1.4 years; range 1.75–8.50 years) |
Results
Decision to go to surgery
Study | With or without focus on MRI/EEG? | Test timing | Tesla strength | Categories from Table 2a | Test not conducted | |||||||
---|---|---|---|---|---|---|---|---|---|---|---|---|
A | B | C | D | E | F | G | H | |||||
MRS: NAA/(Cho + Cr) | ||||||||||||
Achten (1997)55 | Both | Unclear/NR | 1.5 T | 10 | 6 | 0 | 1 | 10 | 1 | 0 | 1 | 0 |
FDG-PET | ||||||||||||
Achten (1997)55 | Both | Unclear/NR | N/A | 13 | 3 | 4 | 2 | 3 | 1 | 0 | 3 | 0 |
Didelot (2008)58 | 22 | 9 | 2 | 2 | 2 | 1 | 0 | 0 | 4 | |||
Theodore (1997)68 | Without | Unclear/NR | N/A | 23 | 2 | 7 | 7 | 5 | 1 | 0 | 1 | 0 |
FDG-PET coregistered to MRI | ||||||||||||
Wu (2010)66 | Both | Unclear/NR | 1.5 T | 2 | 4 | 7 | 5 | 7 | 0 | 3 | 0 | 0 |
HMPAO-SPECT | ||||||||||||
Lee (2002)57 | Both | First ictal | N/A | 2 | 3 | 11 | 1 | 4 | 2 | 1 | 0 | 0 |
Lee (2002)57 | Second ictal | 4 | 2 | 4 | 0 | 5 | 3 | 5 | 1 | 0 | ||
Lee (2002)57 | First and second ictal | 6 | 5 | 4 | 0 | 5 | 1 | 3 | 0 | 0 | ||
HMPAO or ECD-SPECT | ||||||||||||
Lewis (1998)61 | Both | Interictal | N/A | 9 | 4 | 0 | 0 | 9 | 4 | 1 | 1 | 0 |
vMRI | ||||||||||||
Achten (1997)55 | Both | Unclear/NR | 1.5 T | 19 | 4 | 0 | 1 | 1 | 3 | 0 | 1 | 0 |
Classification of the partially concordant and discordant tests when there was a decision to go to surgery, in terms of whether the site of surgery was based on the results of the comparator tests or the index test
Study | Partially concordant | Discordant | |
---|---|---|---|
MRS: NAA/(Cho + Cr) | |||
Achten (1997)55 |
10 Based on EEG localisation |
0 | |
FDG-PET | |||
Achten (1997)55 |
3 Based on EEG localisation |
0 | |
Didelot (2008)58 |
2 Based on comparator tests |
1 Based on comparator tests |
|
Theodore (1997)68 |
5 Based on iEEG localisation |
0 | |
FDG-PET coregistered to MRI | |||
Wu (2010)66 |
7 Difficult to determine which tests contributed most to the decision; authors state that decisions were based on all tests combined |
3 One based on the index test; two based on the comparator tests |
|
HMPAO-SPECT | |||
Lee (2002)57 | First SPECT |
4 Two seem to be based on comparator tests, but index test may have contributed to the decision; one based on SPECT; one could not be classified |
1 Based on comparator tests |
Second SPECT |
5 One based on the comparator tests; two seem to be based on comparator tests, but index test may have contributed to the decision; one based on first SPECT; one could not be classified |
5 Four based on comparator tests; one based on first SPECT |
|
First and second |
5 Four based on comparator tests; one based on first SPECT |
3 Based on comparator tests |
|
HMPAO or ECD-SPECT | |||
Lewis (1998)61 |
9 Eight based on comparator tests, one based on index test |
1 Based on comparator tests |
|
vMRI | |||
Achten (1997)55 |
1 Based on EEG localisation |
0 |
Detailed methods used to extract the data for the decision to go to surgery
As described in Chapter 3 (see Data extraction strategy), data were extracted into the following 2 × 4 contingency table:
Decision for surgery | Decision against surgery | |
---|---|---|
Concordant | A | B |
Non-localising | C | D |
Partially concordant | E | F |
Discordant | G | H |
Concordant, the focus identified by the comparator tests was the same as that identified by the index test. Discordant, the focus identified by the comparator tests was a different focus to that identified by the index test. Non-localising, the comparator tests were localising, but the index test was non-localising. Partially concordant, the focus identified by the comparator tests overlapped, but was not identical to, the focus identified by the index test | ||
Study | Method | |
Achten (1997)55 |
Data were extracted from table 2, p. 5. The site of surgery was based on the results of the EEG. Therefore, for those who underwent surgery, the concordance of the index test could be compared with either the site of surgery or the EEG localisation. When surgery was not undertaken, concordance of the index tests was determined using the EEG localisation Two patients (nos. 12 and 22) had non-lateralising EEG tests and bilateral foci identified on vMRI and MRS; these were considered concordant (i.e. none could identify a focus) with the decision not to go to surgery (cell B). In these same two patients, PET identified a focus in the left temporal lobe; these were classified as discordant (i.e. finding a focus when there was not one) with a decision not to go to surgery (cell H). It could be, of course, that the PET localisation was correct but there was insufficient confidence to risk undertaking excisional surgery, but there is no way to confirm this The categorisation was similar for patient no. 26; despite all three index tests identifying a left temporal focus, this was insufficient for a decision to undertake a temporal lobectomy, and the results for the three index tests were classified as discordant with the non-lateralising EEG (cell H) |
|
Didelot (2008)58 | The final decision on the lateralisation of the seizure focus is in table 1. The results of PET are reported in table 2, as ipsilateral, contralateral or non-localising. Therefore, all ipsilateral (unilateral) tests are concordant with the seizure focus. Where there are bilateral decreases, the PET scan is partially correct as it identified the seizure focus and an additional area. The remaining PET scans were either non-localising, or the results were not available. There were no PET scans that identified decreases on the contralateral side but not the ipsilateral side, therefore there were no discordant scans | |
Lee (2002)57 |
Table 1 gives the diagnosis, the final site of surgery if it was undertaken and the results of the ictal SPECT. Concordance of the SPECT was matched against the final diagnosis. Results for the first and second SPECT were extracted separately Patients who were difficult to classify and how this was done:A third set of data were extracted where the results of the first and second SPECT were combined to give a consensus decision. As examples, patients 4, 12 and 22 had a focus on the left on one scan, and on the right on the other, therefore the combined SPECT result for these patients would be bilateral foci. Where one SPECT scan was non-localising and the other localising, the localised focus was taken as the consensus decision Patients who were difficult to classify and how this was done:There were insufficient numbers of patients undergoing a third and fourth scan for data to be extracted |
|
Lewis (1998)61 |
Table 1 provides results of the MRI, interictal and ictal-surface EEGs and SPECT. Where surgery was undertaken, concordance of the index test was determined by the site of surgery, which can be seen as the consensus decision of the MRI, interictal and ictal-surface EEGs. Where medical treatment was the decision, there was no consensus decision from the MRI, interictal and ictal-surface EEGs; however, in these patients, the MRI, interictal and ictal-surface EEGs were either non-localising, or identified the same seizure focus, therefore the foci where identified was used as the consensus decision Patients who were difficult to classify: |
|
Theodore (1997)68 | Results for this study were extracted from the first two paragraphs of the results section on p. 82:
|
|
Wu (2010)66 |
Results were extracted from table 1 on p. 394. The results of the video-EEG are given only as non-lateralising, lateralising or localising, with the site of resection also provided rather than a preliminary decision as to the site of the seizure focus. Although MEG was one of our index tests of interest, there were insufficient numbers of patients who underwent this test to extract the data, and therefore the MEG result was used to supplement the reference standard in order to given a better indication as to the accuracy of the PET. This resulted in a complicated data extraction, with several of the patients being difficult to classify:Where surgery was not conducted, the results of the video-EEGs were used as the seizure focus to determine concordance Where neither the ictal nor interictal EEG showed an abnormality and the index test was non-localising, these were considered concordant and the decision was not to go to surgery (cell B) Where one or both of the EEGs either lateralised or localised, the non-localising index test was categorised as such (cell D) |
Results: outcome following surgery
Study | Definition of a good outcome | With or without focus on MRI/EEG? | Test timing | Tesla strength | Categories from Table 2a | Test not conducted | Test conducted but no surgery | |||||||
---|---|---|---|---|---|---|---|---|---|---|---|---|---|---|
A | B | C | D | E | F | G | H | |||||||
FDG-PET | ||||||||||||||
Achten (1997)55 | Engel Ia | Both | Interictal | N/A | 12 | 2 | 2 | 0 | 1 | 0 | 0 | 0 | 0 | 12 |
Didelot (2008)58 | Engel Ia | Both | Unclear/NR | N/A | 17 | 5 | 1 | 1 | 1 | 1 | 0 | 0 | 4 | 12 |
Theodore (1997)68 | SF (Engel Ia) | Without | Unclear/NR | N/A | 18 | 5 | 4 | 3 | 3 | 2 | 0 | 0 | 0 | 12 |
Knowlton (1997)56 | Engel I | Both | Unclear/NR | N/A | 15 | 4 | 1 | 2 | 0 | 0 | 0 | 0 | 1 | 1 |
One patient had no MRS and result for PET was not reported PET was coregistered to MRI in one patient to get localisation |
||||||||||||||
Didelot (2008)58 | Engel I | Both | Unclear/NR | N/A | 21 | 1 | 1 | 1 | 2 | 0 | 0 | 0 | 4 | 12 |
Two patients refused iEEG – unclear whether or not they were eligible for surgery | ||||||||||||||
Knowlton (2008)49 | Engel I | Both | Unclear/NR | N/A | Sensitivity 59% (95% CI 47.4% to 67.4%); specificity 79% (95% CI 58.7% to 92.5%); PPV 83% (95% CI 65.9% to 93.8%); NPV 54% (95% CI 39.8% to 62.7%) | 11 | 0 | |||||||
Didelot (2008)58 | Engel I and II | Both | Unclear/NR | N/A | 22 | 0 | 2 | 0 | 2 | 0 | 0 | 0 | 4 | 12 |
Two patients refused iEEG – unclear whether or not they were eligible for surgery | ||||||||||||||
Knowlton (1997)56 | Engel I and II | Both | Unclear/NR | N/A | 18 | 1 | 1 | 2 | 0 | 0 | 0 | 0 | 1 | 1 |
One patient had no MRS and result for PET was not reported PET was coregistered to MRI in one patient to get localisation |
||||||||||||||
Achten (1997)55 | Engel I and II | Both | Interictal | N/A | 12 | 2 | 2 | 0 | 1 | 0 | 0 | 0 | 0 | 12 |
HMPAO-SPECT | ||||||||||||||
Knowlton (2008)49 | Engel I | Both | Ictal | N/A | Sensitivity 50% (95% CI 29.9% to 67.0%); specificity 72% (95% CI 54.4% to 87.3%); PPV 62% (95% CI 36.8% to 82.4%); NPV 62% (95% CI 46.6% to 74.8%) | 28 | 0 | |||||||
Lee (2002)57 |
Engel I All patients with a good outcome had Engel I, therefore numbers the same for Engel I and II |
Both | First Ictal | N/A | 1 | 2 | 7 | 4 | 1 | 1 | 1 | 0 | 0 | 0 |
Patient no. 22 could not be classified as the side of temporal lobe surgery was NR | ||||||||||||||
Second Ictal | N/A | 2 | 2 | 2 | 2 | 2 | 1 | 4 | 2 | 0 | 0 | |||
Patient 22 could not be classified as the side of temporal lobe surgery was NR | ||||||||||||||
First and second Ictal | N/A | 2 | 2 | 2 | 2 | 3 | 4 | 3 | 0 | 0 | 0 | |||
HMPAO or ECD-SPECT | ||||||||||||||
Lewis (1998)61 | SF (Engel Ia) | Both | Interictal | N/A | 3 | 5 | 0 | 0 | 5 | 3 | 0 | 1 | 0 | 9 |
SF/significant or worthwhile improvement (Engle I–III) | Both | Interictal | N/A | 6 | 2 | 0 | 0 | 7 | 1 | 1 | 0 | 0 | 9 | |
MEG | ||||||||||||||
Assaf (2004)63 | SF (Engel Ia) | Both | Interictal | N/A | 21 | 1 | 0 | 0 | 0 | 0 | 0 | 0 | 0 | 4 |
Patient with poor outcome after SAH became SF after AMTL | ||||||||||||||
Smith (1995)69 | SF (Engel Ia) | Both | Interictal | N/A | 14 | 5 | 4 | 1 | 1 | 2 | 3 | 2 | 0 | 15 |
Smith (1995)69 | SF or rare seizures (Engel I and II) | Both | Interictal | N/A | 17 | 2 | 5 | 0 | 1 | 2 | 4 | 0 | 0 | 15 |
Wheless (1999)42 | Excellent outcome (SF or rare seizures; Engel I and II) | With | Unclear/NR | N/A | Sensitivity 52% overall; 57% for TLE; 44% for non-TLE | 0 | 0 | |||||||
Overall: Hits: 23; partial hits: 10; misses: 6; non-localising: 5 TLE: Hits: 16; partial hits: 6; misses: 4; non-localising: 2 Non-TLE: Hits: 7; partial hits: 4; misses: 2; non-localising: 3 |
||||||||||||||
Smith (1995)69 | Over 90% reduction in seizures | Both | Interictal | N/A | 19 | 0 | 5 | 0 | 1 | 2 | 4 | 1 | 0 | 15 |
Classification assuming the EEG focus indicated the site of surgery | ||||||||||||||
Wheless (1999)42 | Good surgical outcome (not defined) | With | Unclear/NR | N/A | Sensitivity 52% overall | 0 | 0 | |||||||
Five patients were not localised by MEG | ||||||||||||||
MRS: NAA/(Cho + Cr) | ||||||||||||||
Achten (1997)55 | Engel Ia | Both | Unclear/NR | 1.5T | 7 | 1 | 0 | 0 | 8 | 1 | 0 | 0 | 0 | 11 |
Knowlton (1997)56 | Engel I | Both | Unclear/NR | 1.5T | 10 | 2 | 5 | 4 | 0 | 0 | 1 | 0 | 1 | 1 |
One patient had no PET and result for MRS was not reported | ||||||||||||||
Achten (1997)55 | Engel I and II | Both | Unclear/NR | 1.5T | 7 | 1 | 0 | 0 | 8 | 1 | 0 | 0 | 0 | 11 |
Knowlton (1997)56 | Engel I and II | Both | Unclear/NR | 1.5T | 10 | 2 | 8 | 1 | 0 | 0 | 1 | 0 | 1 | 1 |
One patient had no PET and result for MRS was not reported | ||||||||||||||
MRS: NAA/Cho | ||||||||||||||
Lee (2005)65 | SF (Engel Ia) | Both | Unclear/NR | 1.5T | 13 | 1 | NR | NR | 6 | 5 | 0 | 0 | 0 | 0 |
MSI | ||||||||||||||
Kaiboriboon (2010)64 | Engel Ia | Both | Unclear/NR | Unclear/NR | 8 | 5 | 3 | 0 | 2 | 2 | 2 | 0 | 0 | NR |
Engel I | 9 | 4 | 3 | 0 | 3 | 1 | 2 | 0 | ||||||
Engel I and II | 13 | 0 | 3 | 0 | 3 | 1 | 2 | 0 | ||||||
Knowlton (2008)49 | Engel I | Both | Unclear/NR | Unclear/NR | Sensitivity 55% (95% CI 44.2% to 63.7%); specificity 75% (95% CI 57.4% to 88.4%); PPV 78% (95% CI 61.2% to 89.7%); NPV 51% (95% CI 39.4% to 60.6%) | 0 | 0 | |||||||
vMRI | ||||||||||||||
Achten (1997)55 | Engel Ia (SF) | Both | Unclear/NR | 1.5T | 14 | 2 | 0 | 0 | 1 | 0 | 0 | 0 | 0 | 11 |
MSI + FDG-PET | ||||||||||||||
Knowlton (2008)49 | Engel I | Both | Unclear/NR | Unclear/NR | Sensitivity 25% (95% CI 15.2% to 28.0%); specificity 95% (95% CI 78.2% to 99.7%); PPV 89% (95% CI 54.0% to 99.4%); NPV 43% (95% CI 35.4% to 45.1%) | 11 | 0 | |||||||
MSI + HMPAO-SPECT | ||||||||||||||
Knowlton (2008)49 | Engel I | Both | Unclear/NR | Unclear/NR | Sensitivity 19% (95% CI 5.5% to 32.0%); specificity 83% (95% CI 71.6% to 95.1%); PPV 50% (95% CI 14.7% to 85.2%); NPV 54% (95% CI 46.0% to 61.1%) | 28 | 0 | |||||||
MSI + FDG-PET + HMPAO-SPECT | ||||||||||||||
Knowlton (2008)49 | Engel I | Both | Unclear/NR | Unclear/NR | Sensitivity 8% (95% CI 0.4% to 15.0%); specificity 100%; PPV 100%; NPV 54% (95% CI 50.2% to 57.5%) | 35 | 0 |
Detailed methods used to extract the data for the outcome following surgery
As described in Chapter 3 (see Data extraction strategy), data were extracted into the following 2 × 4 contingency table:
Good outcome following surgery | Poor outcome following surgery | |
---|---|---|
Concordant | A | B |
Non-localising | C | D |
Partially concordant | E | F |
Discordant | G | H |
Study | Method |
---|---|
Achten (1997)55 | Table 1: Concordance was determined by comparing the localisation of the index test with consensus site of surgery |
Assaf (2004)63 | Table 1 was used to confirm concordance between all MEG tests and the iEEG and/or MRI. In row 1, the number of patients identified by MEG as requiring SAH, AMTL and cortical resection, correspond to the numbers identified by the other two tests in the rows below. From the text it appears that some MEG results were only partially concordant with the other results; however, all dipoles were located in the temporal lobe, and therefore from the point of view of the review, were fully concordant. This means that only cells A and B will contain patients. Of the 22 who underwent surgery, 21 were SF (cell A) and one was not (cell B) |
Didelot (2008)58 | The results of PET and the outcome following surgery are reported in Table 2. PET scans are either ipsilateral (all are unilateral and concordant with the seizure focus), contralateral or non-localising. Where there are bilateral decreases, the PET scan is partially correct as it identified the seizure focus (one temporal lobe) and an additional area (the opposite temporal lobe). The remaining PET scans were either non-localising or the results were not available. No PET scans the identified decreases on the contralateral side but not the ipsilateral side, therefore there were no discordant scans |
Kaiboriboon (2010)64 | Table 1 provides the site of surgery with which the results of the index test were compared with determine concordance, with the associated Engel outcome classification in the last column |
Knowlton (1997)56 |
Data were extracted from figure 2 on p. 834. From table 1 it can be seen that all lateralising PET scans were concordant with ictal EEG, and with the threshold of 12% used for MRS [NAA/(Cho + Cr)], one scan was discordant (top left of p. 833) In figure 2, the scans are classified as either lateralised (concordant) or non-lateralised, and the Engel classification is given in the circles. For the PET results, all are concordant, therefore, depending on whether they are lateralising or not, with a good outcome or not, cells A, B, C and D would contain patients For MRS, the patient with a discordant NAA/(Cho + Cr) MRS scan became SF (top right paragraph, p. 833). The others were all concordant and the results extracted from figure 2 as with PET One patient did not have PET, and another did not have MRS, therefore these patients are not presented in the figure. One patient (top line of right-hand figure) did not undergo surgery |
Lee (2002)57 |
Table 1 gives the final site of surgery and this was used to determine the concordance of the results of the SPECT. Results for the first and second SPECT were extracted separately. A third set of data were extracted where the results of the first and second SPECT were combined to give a consensus decision. For example, patient no. 22 had a focus on the left on one scan and on the right on the other, therefore the combined SPECT result for such patients would be bilateral foci There were insufficient numbers of patients undergoing third and fourth scans for data to be extracted |
Lee (2005)65 | Data for patients with TLE were extracted from table 8; there were no surgical outcome results reported for those with non-localising MRS, only those with concordant and partially concordant (bilateral) tests (patients with non-localising scans are reported in table 6) |
Lewis (1998)61 | Table 1: Concordance was determined by comparing the localisation of the index test with site of surgery in the outcome column |
Smith (1995)69 | Table 2 gives the concordance between MEG and EEG, and the outcome following surgery. From the text it seems that the final localisation is based on the EEG results in all cases, and in part on the MEG results in some patients. This may lead to incorporation bias |
Theodore (1997)68 | Data were extracted from the first two paragraphs of the results section:
|
Outcome prediction studies
Study details
Study | Population | Surgery details | Analyses |
---|---|---|---|
Antel (2002) 60 Country/region: USA/Canada Direction of data collection: Retrospective Was IPD reported? No |
No. recruited (no. male): 81 (31) Mean age: 35 years (SD 11.2 years) Adults/children? Unclear/NR Mean duration of epilepsy: (SD range) Type of epilepsy: Temporal (undefined): 81 |
Eligibility for surgery: Eligible for surgery: 81 Underwent surgery: 81 Refused surgery: 0 Ineligible for surgery: 0 Unaccounted for: 0 Type of surgery performed: ATL: 41 SAH: 40 Postoperative follow-up: Mean 38.1 months (SD range 9.2–78.2 months) |
Analysis used: Bayesian classifiers used to predict outcome based on conditional probability distributions Measure of association: Predictive values (%) Dependent variable(s): SF > 90% reduction in seizures Not SF No worthwhile improvement Index tests used as independent variable(s): MRI/MRS Other independent variable(s) in model: NR |
Dupont (2000) 70 Country/region: Western Europe Direction of data collection: Retrospective Was IPD reported? No |
No. recruited (no. male): 30 (13) Mean age: 29 years (range NR) Adults/children? NR Mean duration of epilepsy: NR Type of epilepsy: MTS: 30 (hippocampal atrophy: 27) |
No. who underwent iEEG: 6 Eligibility for surgery: Eligible for surgery: 30 Underwent surgery: 30 Refused surgery: 0 Ineligible for surgery: 0 Unaccounted for: 0 Type of surgery performed: ATL: 30 SAH: 30 Postoperative follow-up: Mean 3.5 years (SD range 2.1–5.3 years) |
Analysis used: Discriminant analysis using as multiple dependent variables the regional metabolic asymmetries Measure of association: Other Dependent variable(s): Class A (SF) vs class C (rare DSs or worthwhile improvement) Index tests used as independent variable(s): PET Other independent variable(s) in model: NR |
Knowlton (1997) 56 Country/region: USA/Canada Direction of data collection: Prospective Was IPD reported? Yes For how many patients? 23 (92%) |
No. recruited (no. male): 25 (12) Mean age: 38 years (range 14–56 years) Adults/children? Both Mean duration of epilepsy: NR Type of epilepsy: Hippocampal atrophy: 15 Temporal (undefined): 10 |
No. who underwent iEEG: 10 Eligibility for surgery: Eligible for surgery: 24 Underwent surgery: 24 Refused surgery: 0 Ineligible for surgery: 1 Unaccounted for: 0 Type of surgery performed: ATL: 24 SAH: 24 Postoperative follow-up: Mean 23 months (range 18–31 months) |
Analysis used: NR Measure of association: NR Dependent variable(s): SF Index tests used as independent variable(s): vMRI PET MRS Other independent variable(s) in model: NR |
Knowlton (2008) 49 Country/region: USA/Canada Direction of data collection: Prospective Was IPD reported? No |
No. recruited (no. male): 62 (33) Mean age: 26 years (range 1–60 years) Adults/children? Both Mean duration of epilepsy: NR Type of epilepsy: MTS: 21 Lateral TLE: 9 Extratemporal (undefined): 29 Not localised: 3 |
No. who underwent iEEG: 62 Eligibility for surgery: Eligible for surgery: 62 Underwent surgery: 62 Refused surgery: 0 Ineligible for surgery: 0 Unaccounted for: 0 Type of surgery performed: NR Postoperative follow-up: Mean 3.4 years, minimum 1 year; maximum NR |
Analysis used: Logistic regression Measure of association: Odds ratio Dependent variable(s): Good outcome (Engel I only) Index tests used as independent variable(s): MSI: in patients undergoing MSI only MSI: in patients undergoing MSI and PET MSI: in patients undergoing MSI and SPECT MSI: in patients undergoing MSI, PET and SPECT SPECT: in patients undergoing MSI and SPECT SPECT: in patients undergoing MSI, PET and SPECT PET: in patients undergoing MSI and PET PET: in patients undergoing MSI, PET and SPECT Other independent variable(s) in model: Age; location of resection; routine MRI; video-EEG |
O’Brien (2001) 62 Country/region: Australia/New Zealand Direction of data collection: Unclear Was IPD reported? No |
No. recruited (no. male): 55 (31) Mean age: 34 years (range 16–63 years) Adults/children? Both Mean duration of epilepsy: NR Type of epilepsy: Temporal (undefined): 41 Frontal: 2 Frontoparietal: 4 Temporoparietal: 1 Parietal: 1 Not localised extratemporal focus: 6 |
Eligibility for surgery: Eligible for surgery: 40 Underwent surgery: 24 Refused surgery: 9 Ineligible for surgery: 15 Unaccounted for: 0 Type of surgery performed: Temporal (unspecified): 22 Extratemporal: 2 Awaiting surgery or have < 6 months’ follow-up: 7 Postoperative follow-up: Median 17 months (range 6–42 months) |
Analysis used: Linear regression Measure of association: Beta coefficients Dependent variable(s): Engel classes I–IV Index tests used as independent variable(s): PET Other independent variable(s) in model: Routine MRI |
O’Brien (1999) 67 Country/region: USA/Canada Direction of data collection: Retrospective Was IPD reported? No |
No. recruited (no. male): 35 (18) Mean age: Median 26 years (range 2–69 years) Adults/children? Both Mean duration of epilepsy: NR Type of epilepsy: Temporal (undefined): 15 Extratemporal (undefined): 15 Unlocalised: 5 |
Eligibility for surgery: Eligible for surgery: 35 Underwent surgery: 35 Refused surgery: 0 Ineligible for surgery: 0 Unaccounted for: 0 Type of surgery performed: ATL with amygdalohippocampectomy: 15 ATL with amygdalohippocampectomy-frontal: 1 Frontal: 5 Frontal parietal: 1 Temporal parietal: 1 Postoperative follow-up: Median 27 months (range 12–42 months) |
Analysis used: Logistic regression Measure of association: Beta coefficients Dependent variable(s): Excellent outcome: A seizure score of 4 or less (SF, with or without AEDs, or non-disabling nocturnal seizures only) Index tests used as independent variable(s): SPECT (hypoperfusion SISCOM) SPECT (combined SISCOM) Other independent variable(s) in model: Location of resection; routine MRI |
Theodore (1997) 68 Country/region: USA/Canada Direction of data collection: Prospective Was IPD reported? No |
No. recruited (no. male): 46 (NR) Mean age: NR Adults/children? NR Mean duration of epilepsy: NR Type of epilepsy: Temporal (undefined): 26 Frontal: 1 Frontal temporal: 5 PET negative and focus NR: 14 |
No. who underwent iEEG: 46 Eligibility for surgery: Eligible for surgery: 34 Underwent surgery: 34 Refused surgery: 0 Ineligible for surgery: 12 Unaccounted for: 0 Type of surgery performed: Temporal lobectomy, including resection of the lateral and mesial structures: 34 Postoperative follow-up: Mean 67.7 months (range 12–113 months) |
Analysis used: Logistic regression Measure of association: NR Dependent variable(s): SF Index tests used as independent variable(s): PET Other independent variable(s) in model: Constant; iEEG; routine MRI |
Decision study
Study details
Study | Population | Test details | Surgery details |
---|---|---|---|
Uijl (2007) 59 Country/region: Western Europe Direction of data collection: Retrospective Was IPD reported? No |
No. recruited (no. male): 469 (NR) Mean age: NR Adults/children? NR Mean duration of epilepsy: NR Type of epilepsy: Temporal (undefined): 469 Patients with or without a focus after EEG/MRI: Both |
Test(s) on which the preliminary decision was made: Routine MRI and surface video-EEG Tests on which final decision was based: Tests used for preliminary decision plus PET Definition(s) of a good surgical outcome: SF (Engel Ia) No. who underwent iEEG: 14 were reported but more may have been investigated invasively No. refusing iEEG: NR Index test(s) details: FDG-PET (timing unclear) Of the 469 patients, 359 (77%) did not receive PET; of these, 215 underwent surgery, 58 did not undergo surgery, and in 86 the surgical decision remained uncertain No. of patients with test-related adverse events: NR |
Eligibility for surgery: Eligible for surgery: 302 Underwent surgery: 302 Refused surgery: NR Ineligible for surgery: 167 (all presumed ineligible but some may have refused surgery) Unaccounted for: 0 Type of surgery performed: Temporal lobe surgery (specific structures excised NR): 302 Postoperative follow-up: Mean 4.2 years (range 1–10 years) |
Appendix 4 Excluded studies with rationale
A total of 283 studies were excluded because they were either retrospective or the direction of data collection was unclear, and they did not report the impact of an index test of interest on the decision to undertake surgery (were not a potential ‘Decision study’) or report the results of a multivariate analysis when an index test of interest was an independent variable (not a potential ‘Outcome prediction study’); details available from the authors.
Other exclusion criteria
Wrong population
Bell 2009;98 Berg 2003;99 DeBets 1997;100 Duncan 1993;101 Feucht 2004;102 Gallen 1997;103 Goncalves 2006;104 Hessen 2008;105 Jayakar 2008;106 Jeong 2005;107 Joo 2006;108 Kim 2009;109 Kim 1999;110 Kumar 2009;111 Lee 2004;112 Leiderman 1992;113 Mastin 1996;114 Matsuda 2001;115 Matsuda 2001;116 O’Brien;117 Pan 2009;118 Pondal-Sordo 2007;119 Rathore 2011;73 Rossi 1994;120 Rowe 1989;121 Seki 1998;122 Shih 2003;123 Stefan 1994;124 Tan 2008;125 Thadani 2004;126 Theodore 1992;127 Tripathi 2010;128 Uijl 2008;129 Velasco 2011;74 von Oertzen 2006;130 von Oertzen 2002;131 Wang 2008;132 Weitemeyer 2005;133 Wieser 2003;87 Yasuda 2007;134 and Zijlmans 2007. 135
Index test of interest not evaluated
Ahmad 2007;136 Ansari 2010;137 Asadi-Pooya 2008;138 Azevedo 2009;139 Berg 2007;140 Beleza 2010;141 Boon 2002;142 Boshuisen 2009;143 Brooks 1990;144 Chaudhry 2010;145 Clusmann 2004;146 Costello 2009;147 Cukiert 2009;148 Fauser 2008;149 Feucht 2004;102 Foldvary 2000;150 Gilliam 2000;151 Jackson 1990;152 Jacobs 2010;153 Kuzniecky 1993;154 Mihara 2004;155 Mikati 2004;156 Moritake 2009;157 Paglioli 2004;158 Quarato 2005;159 Roberti 2007;160 Salmenpera 2002;161 Selwa 2003;162 Spooner 2006;163 Stefan 2009;164 Strandberg 2008;165 Tanriverdi 2008;166 Tanriverdi 2008;167 Tatum 2003;168 Tellez-Zenteno 2005;169 Tripathi 2010;128 Uijl 2008;170 Velasco 2006;171 and Yasuda 2006. 172
No decision for, or outcome following, surgery, or given for subset of < 20 patients
Antar 1991;173 Antar 1992;174 Aung 1995;175 Berg 1998;176 Bilir 1998;177 Blum 2003;178 Bohnen 1996;179 Bouvard 1998;180 Buchpiguel 1992;181 Cendes 1995;182 Cendes 1997;183 Chacon 2009;184 Chee 1991;185 Cook 1992;186 Cook 1994;187 Doi 1995;188 Eliashiv 1999;189 Eliashiv 2000;190 Fischer 2005;191 Garcia 2006;192 Gilliam 2008;193 Gram 1988;194 Harvey 1993;195 Kagawa 2005;196 Knake 2006;197 Knowlton 2003;198 Knowlton 2005;199 Knowlton 2005;200 Knowlton 2007;201 Knowlton 2008;51 Knowlton 1997;202 Knowlton 2009;203 Kumar 2009;204 Kuzniecky 1998;205 Lee 1996;206 Lee 2007;207 Medina 2005;208 Miller 2003;209 Newton 1992;210 Newton 1993;211 Newton 1994;212 Newton 1995;213 Ng 1994;214 O’Brien 1998;215 Okpaku 1999;216 Oommen 1997;217 Oommen (unpublished);218 Oommen 2004;219 Otsuki 2004;220 Pataraia 2004;221 Radhakrishnan 2009;222 Rowe 1988;223 Rowe 1988;224 Runge 1997;225 Ryvlin 1998;226 Seidenberg 2005;227 Smith 2003;228 So 1996;229 So 1997;230 Swartz 1992;231 Tecoma 1995;232 Thomsen. 2006;233 Tsimerinov and Eliashiv 2007;234 Tsimerinov 2007;235 Venz 1994;236 Vera 1999;237 Watanabe 2002;238 Xiang 2009;239 Yu 1995;240 and Zhou 1994. 241
Magnetic resonance imaging was acquired using a magnet of < 1.5 T
Assadi 1997;242 Boundy 1996;86 Debets 1990;243 Duncan 1993;101 Hong 2002;244 Jabbari 1991;245 Kim 2004;246 Kun Lee 2005;247 Kuzniecky 1991;248 Lee 2005;249 Lee 2008;250 Oliveira 1999;251 Rowe 1991;252 Shukla 2003;253 Son 1999;254 Sperling 1986;255 Sutherling 2008;72 Theodore 1990;256 Won 1999;80 and Zaknun 2008. 257
Study was restricted to patients with a good or bad outcome following surgery
Eliashiv 1998;258 Fukuda 2006;259 Heinz 1994;260 Hong 2000;261 Kang 1997;262 Kim 2002;263 Kim 1999;110 Lee 2000;264 Lee 2002;265 Lee 2005;266 Nelissen 2006;267 Park 2001;268 Sperli 2006;269 Tatsch 2000;270 Weil 2001;271 Uesugi 1996;272 and Uesugi 1998. 273
The study/relevant subgroup had < 20 patients, or the number of eligible patients (i.e. no tumour, vascular malformation, cyst, etc.) for whom results were reported was < 20 (< 30 patients for the decision question)
Al-Semari 2005;274 Altay 2003;275 Alving 1999;276 Avery 2000;277 Barrington 1998;278 Bouilleret 2002;279 Cascino 1999;280 Cendes 1997;281 Chernov 2009;282 Cunha 2003;283 Depositario-Cabacar Dewi 2009;284 Drzezga 1998;285 Dupont 2006;286 Dupont 1999;287 Eliashiv 1997;288 Eliashiv 2002;289 Eliashiv and Fedi 2001;290 Fukuda 2005;291 Fukuda 2006;259 Gallen 1997;103 Genow 2004;292 Gross 2006;293 Gupta 2004;294 Guye 2007;295 Hammers 2005;296 Holmes 2010;297 Iida 2005;298 Iwasaki 2002;299 Juhasz 2001;300 Kakisaka 2009;301 Kamimura 2006;302 Kim 2001;303 Kitamura 2003;304 Knowlton 2004;305 Knowlton 1995;306 Knowlton 2000;307 Koo 2003;308 Krsek 2007;309 Lambert 2003;310 Lamusuo 2000;311 Lamusuo 2001;312 Lantz 2003;313 Lantz 2006;314 Lassen 1989;315 Liew 2009;316 Lynch 1995;317 MacDougall 2009;318 Maehara 2005;319 Mayer 2003;320 Mayer 2003;321 Menzel 1996;322 Minassian 1999;323 Mohamed 2005;324 Nelissen 2006;267 Oishi 2006;325 O’Tuama 1991;326 Otsubo 2001;327 Ozkara 1999;328 Paetau 1992;329 Pan 2009;118 Pataraia 2005;330 Pu 2002;331 Rathore 2011;73 Rowe 1989;121 Sasaki 2003;332 Schuler 1994;124 Seki 1998;122 Shah 2006;333 Shukla 2005;334 Stefan 1992;335 Suhy 2002;336 Szaflarski 2003;337 Szaflarski 2004;338 Tanaka 1997;339 Tanaka 2000;340 Tatum 1994;341 Tatum 2003;168 Tepmongkol 2007;342 Thornton 2007;18 Umeoka 2007;343 Vitikainen 2009;344 Watson 1994;345 Watson and Moore 1998;346 Waxman 1998;347 Wichert-Ana 1998;348 Wichert-Ana 2008;349 Wissmeyer 2001;350 Wissmeyer 2002;351 Wong 1996;352 Wu 2006;353 and Yeh 1998. 354
Only the decision to go to surgery was reported, and there was no invasive electroencephalography/magnetic resonance imaging
Biersack 1990. 355
Abstract with insufficient information to extract and no full paper
Akamatsu 2007;356 Antar and McIntyre 2006;357 Antel 2000;358 Eliashiv 2002;359 Fujiwara 2009;360 Hetherington 2007;361 Hugg 1998;29 Lee 2006;362 Lee and Lee 1997;363 Radtke 1995;364 Sundaram 2006;365 Tan 2008;366 Tripathi 2004;367 Tripathi 2004;368 Van Huffelen 2004;369 Vanisselt 1993;370 von Oertzen 2002;371 Vorgia 2005;372 and, Zuchun and Xiaoping 2009. 373
Abstract with insufficient information, linked to an excluded full paper
Di Gennaro 2005;374 Leroy 1992;375 Mohamed 2000;376 O’Brien 2001;377 Oh 2001;378 Politsky 2003;379 Tan 2008;366 Thadani 1999;380 and Willmore 1998. 381
Abstract with insufficient information, linked to an included full paper
Dupont 1997382 (linked to Dupont 200070) and Hoo 199730 (linked to Knowlton 199756).
Non-English
Bourgeois 2008;386 Devaux 2009;387 He 2003;388 He 2001;389 Maehara 2007;390 Matsuda 2001;391 Papathanassiou 2009;392 Setoain 2006;393 Stefan 2001;394 van Veelen 2001;395 Wu 2009;396 and Yu 2004. 397
Appendix 5 Quality of the cost-effectiveness studies
Quality of the O’Brien et al. 78 paper was assessed according to a checklist based on that developed by Drummond et al. 76 All items are graded as follows: ‘yes’ (items adequately addressed), ‘no’ (items not adequately addressed), ‘?’ (unclear or not enough information), ‘N/A’ (not applicable) or ‘NS’ (not stated).
Study question | Answer | Comment |
---|---|---|
1. Costs and effects examined | Yes | |
2. Alternatives compared | Yes | |
3. The viewpoint(s)/perspectives of the analysis is clearly stated (e.g. NHS, society) | Yes | |
Selection of alternatives | ||
4. All relevant alternatives are compared (including do nothing if applicable) | No | Several alternative visualisation technologies and combinations are not included |
5. The alternatives being compared are clearly described (who did what to whom, where and how often) | Yes | |
6. The rationale for choosing the alternative programmes or interventions compared is stated | No | |
Form of evaluation | ||
7. The choice of form of economic evaluation is justified in relation to the questions addressed | Yes | |
8. If a cost-minimising design is chosen, have equivalent outcomes been adequately demonstrated? | N/A | |
Effectiveness data | ||
9. The source(s) of effectiveness estimates used are stated (e.g. single study, selection of studies, systematic reviews, expert opinions) | Yes | |
10. Effectiveness data from RCT or review of RCTs | No | |
11. Potential biases identified (especially if data not from RCTs) | No | |
12. Details of the method of synthesis or meta-analysis of estimates are given (if based on an overview of a number of effectiveness studies) | N/A | |
Costs | ||
13. All of the important and relevant resource use included | ? | It is not clear how long-term cost savings were estimated or applied in the analysis |
14. All of the important and relevant resource use measured accurately (with methodology) | Yes | |
15. Appropriate unit costs estimated (with methodology) | Yes | |
16. Unit costs reported separately from resource-use data | No | |
17. Productivity costs treated separately from other costs | N/A | |
18. The year and country to which unit costs apply is stated with appropriate adjustments for inflation and/or currency conversion | Yes | |
Benefits measurement and valuation | ||
19. The primary outcome measure(s) for the economic evaluation are clearly stated (cases detected, life-years, QALYs, etc.) | Yes | |
20. Methods to value health states and other benefits are states (e.g. time trade off) | N/A | |
21. Details of the individuals from whom valuations were obtained are given (patients, members of the public, health-care professionals, etc.) | N/A | |
Decision modelling | ||
22. Details of any decision model used are given (e.g. decision tree, Markov model) | Yes | |
23. The choice of model used and the key input parameters on which it is based are adequately detailed and justified | Yes | |
24. All model outputs described adequately | No | |
Discounting | ||
25. Discount rate used for both costs and benefits | ? | Unclear as long-term costs are provided as single cost-saving figure, with no mention of discounting |
26. Do discount rates accord with NHS guidelines | N/A | |
Allowance for uncertainty | ||
Stochastic analysis of patient-level data | ||
27. Details of statistical tests and CIs given for stochastic data | No | |
28. Uncertainty around cost-effectiveness expressed (e.g. CI around ICER, CEACs) | No | |
29. Sensitivity analysis used to assess uncertainty in non-stochastic variables (e.g. unit costs, discount rates) and analytic decisions (e.g. methods to handle missing data) | Yes | |
Stochastic analysis of decision models | ||
30. Are all appropriate input parameters included with uncertainty? | No | |
31. Is second-order uncertainty (uncertainty in means) included rather than first-order (uncertainty between patients)? | No | |
32. Are the probability distributions adequately detailed and appropriate? | No | |
Deterministic analysis | ||
33. The approach to sensitivity analysis is given (univariate, threshold analysis, etc.) | Yes | |
34. The choice of variables for sensitivity analysis is justified | No | |
35. The ranges over which the variables are varied are stated | Yes | |
Presentation of results | ||
36. Incremental analysis is reported using appropriate decision rules | ? | It is unclear what decision rules are used to determine the incremental analysis |
37. Major outcomes are presented in a disaggregated as well as aggregated form | Yes | |
38. Applicable to NHS setting | ? | It is not clear due to a lack of clarity in a number of key parameters |
Appendix 6 Protocol
Technology Assessment Report commissioned by the NIHR HTA Programme – Protocol
Title of the project:
The clinical and cost-effectiveness of technologies used to visualise the seizure focus in people with refractory epilepsy being considered for surgery: a systematic review and decision analytical model.
Name of TAR team and ‘lead’
CRD/CHE Technology Assessment Group (Centre for Reviews and Dissemination/Centre for Health Economics), University of York.
-
Dr Jane Burch
-
Research Fellow
-
Centre for Reviews and Dissemination,
-
University of York, Heslington, York
-
YO10 5DD
-
Stephen Palmer
-
Senior Research Fellow
-
Centre for Health Economics
-
University of York, Heslington, York
-
YO10 5DD
Plain English Summary
Epilepsy is the most common serious neurological condition; approximately 456,000 people in the UK have a diagnosis of epilepsy. Epilepsy is usually controlled using antiepileptic drugs (AEDs), however, approximately 20-30% of people continue to have fits (seizures) despite drug treatment. For people who do not respond to drug treatment, surgical removal of the part of the brain (resection) where the epileptic seizures originate (the epileptic focus) may be considered, as this can result in the person becoming seizure-free after the operation. The epileptic focus may be located anywhere in the cerebral cortex (the grey matter) of the brain.
Before surgery can take place, the epileptic focus must be identified. To do this a range of diagnostic tests can be used. In the UK NHS, usual practice is to obtain details of the person’s medical history, undertake a clinical examination, and perform an electroencephalogram (EEG) with electrodes applied to the scalp and a routine magnetic resonance imaging (MRI) scan. Individuals will usually go on to have continuous video and EEG recording with electrodes applied to the scalp in order to record seizures and identify the likely seizure focus. If these are unsuccessful in locating the epileptic focus three broad alternatives will need to be considered. Firstly, if there is a clear indication as to the site of the seizure focus the person may be admitted to hospital for continuous invasive EEG monitoring with electrodes inserted into, or across the surface of, the brain. Secondly, if there is no clear indication as to the site of the seizure focus, and it is considered unlikely that the patient will benefit from surgery, a decision might be made not to continue with the pre-surgical evaluation. Thirdly, and the most common, would be a decision to proceed with further, non-invasive, investigations such as specialised imaging scans or magnetoencephalography (MEG). The main aim of these investigations is to provide further information about the likely site of the seizure focus so that a decision can be made as to whether to proceed with invasive EEG monitoring, proceed to surgery, or to halt the pre-surgical evaluation.
We will undertake a systematic review to determine the added value of these non-invasive technologies in this decision making process and investigate the cost-effectiveness of these technologies. The cost of conducting further research will also be investigated.
Background
Epilepsy
Epilepsy is the most common serious neurological condition, with a prevalence of between 0.5% and 1% in developed countries, and a cumulative incidence of up to 3% by the age of 75 years. 1,2 In a recent review of epidemiological data from European countries, prevalence ranged from 0.32% to 0.78%, depending on the country and population studied. 3 The only UK study included in the review of prevalence, reported a prevalence of 0.43% in children aged 6 to 14 years in England. 4 With approximately 456,000 people in the UK having a diagnosis of epilepsy,5–7 overall prevalence can be estimated at around 0.8%. For most people seizure control is good, but up to 30% continue to have seizures despite ongoing treatment with one or more AED. 8
Classification of epilepsies
Epilepsy is not a single condition; epilepsies are a large heterogeneous group of disorders, including those that are genetically determined (idiopathic, tending to present in childhood and adolescence), to those that are symptomatic of a brain injury (for example stroke or head injury). The epilepsies are classified into syndromes according to seizure types, aetiology, age of onset, EEG changes and MRI findings. One of the major classifications is the localization related epilepsies (affect a limited area of the brain, i.e. focal), which result in focal seizures which can be simple (no associated impairment of consciousness) or complex (impairment of consciousness), or secondarily generalised. These epilepsies are sub-classified according to the site of the focus (frontal, temporal, parietal, occipital lobes) and then further divided according to aetiology:
-
Symptomatic epilepsy Where there is evidence of a lesion or damage to the brain (for example mesial temporal lobe sclerosis, cortical dysplasia, tumour, vascular malformation, haemorrhage, infarct, infection and trauma).
-
Cryptogenic epilepsy Where there are symptoms to suggest brain damage, but there is no evidence of a lesion or damage to the brain.
-
Idiopathic epilepsy: assumed genetic aetiology.
Of the 20-30% of people who continue to have seizures despite drug treatment, the majority have symptomatic or cryptogenic localization related epilepsy. A review conducted in 2001 reported response rates in patients with refractory localisation related epilepsy to adjunctive therapies (adjusted for placebo response rates) of between 12% and 29% for antiepileptic drugs (drugs evaluated were: gabapentin; lamotrigine; levetiracetam; oxcarbazepine; tiagabine; topiramate; zonisamide), and 12% for adjunctive vagal nerve stimulation. 9 For patients who do not respond to adjunctive therapy, surgical resection of the epileptic focus may be considered, and can result in the patient becoming seizure-free.
Surgery for epilepsy
The main aim of surgery is to remove the seizure focus, leaving the patient free from seizures without causing other disability. The epileptic focus may be located anywhere in the cerebral cortex, and there are a number of potential aetiologies. Most epilepsy surgery programmes focus on temporal lobe surgery (temporal lobectomy); excision of the temporal lobe has a higher rate of success and a lower chance of causing harm, than resection of extra-temporal lobe foci. Surgical procedures other than lobectomy are also sometimes preformed, for example, hemispherectomy, corpus callosotomy, multiple subpial transaction and vagal nerve stimulation. The first RCT evaluating epilepsy surgery randomised eighty patients with drug-refractory temporal lobe epilepsy to either surgery or continued AED treatment. At 12 months, 58% of the surgical group and 8% of the AED group were free of complex partial seizures, giving a number needed to treat of 2 (95% CI 1.3 to 3). 10
Burden of illness
According to Hospital Episode Statistics (HES), in 2005/6 epilepsy was responsible for 50,112 consultant episodes and 39,871 admissions (34,226 emergency, 3,431 waiting list and 1,662 day cases), which accounted for 173,885 bed days. In 2007/8, there were 51,864 episodes of admitted patient care accounting for 151,007 occupied bed days. 6 In 2008/9, 330 operations were conducted where major excision of brain tissue was the main operation, and 3,744 where excision of a lesion of brain tissue was the main operation. 6 The 1999 Department of Health Commissioned report from the Clinical Standards Advisory Group (CSAG) on services for patients with epilepsy2 uses estimated that between 5% and 10% of patients with refractory focal epilepsy might benefit from epilepsy surgery. Using this estimate they highlight that between 5000 and 10,000 UK patients might benefit from epilepsy surgery whilst between 750 and 1500 cases are added to this cohort each year. A study using the UK National General Practice Study of Epilepsy estimated the number of patients with newly diagnosed patients who may eventually require surgery, based on an estimate of 30,000 incident cases in the UK, to be around 1.5% (95% CI 0.5 to 2.5), or 450 patients a year. 11
In a 199812 study, the direct cost of epilepsy was estimated at £1,568 per patient per annum. 8 When seizure frequency was taken into account, the total cost of care for patients having more than one seizure per month was eight times that of patients who were seizure free (£3,508 versus £443). Drug cost accounted for 23% of the cost, 43% of which was spent on the then new AEDs, vigabatrin and lamotrigine, which were prescribed for only 6% of patients. Since this study, several drugs, used either as monotherapy and/or adjunctive therapy, have obtained licenses in the UK, including levetiracetam (2000), oxcarbazepine (2000), pregabalin (2004), zonisamide (2005) rufinamide (2007), and lacosamide (2008). 13,14 A 2007 review estimated the cost of epilepsy in European countries at 2004 pricing levels. 3 The total cost (direct and indirect) ranged from 2,000 Euros(Estonia) to 11,500 Euros (Switzerland); approximately 18% was direct healthcare costs. 3 The total cost in the UK was estimated at approximately 8,250 Euros. 3
Work-up for epilepsy surgery
The work-up for epilepsy surgery aims to isolate the seizure focus and identify the underlying aetiology. 15 In the UK, all patients being considered for surgery will have had a detailed history taken, a clinical examination, psychometric testing (testing of memory and IQ for evidence of focal cognitive dysfunction), routine interictal and ictal EEG with surface (scalp) electrodes and routine MRI (T1, T2 with or without FLAIR). The Wada test, where a short acting barbiturate (sodium amytal) is injected into one carotid artery to anaesthetise part of one hemisphere following which memory and language are tested in the opposite hemisphere, may also be conducted.
After conducting routine surface EEG and routine MRI, several potential courses of action may become available:
-
A seizure focus, features of mesial temporal sclerosis, or a lesion such as a tumour, vascular malformation, developmental malformation, or post-trauma or infection malformation may be apparent. In these cases, surgery can proceed, or where surgery is not possible, further evaluation can be halted and an alternative strategy to best control seizures decided.
-
There may be a clear indication as to the site of the seizure focus the person may be admitted to hospital for continuous invasive EEG monitoring with electrodes inserted into, or across the surface of, the brain. During this monitoring, patients usually have their AED medication reduced to increase the likelihood of seizures; this is associated with a small risk of status epilepticus and death.
-
There may be no clear indication as to the site of the seizure focus, and it is considered unlikely that the patient will benefit from surgery, a decision might be made not to continue with the pre-surgical evaluation.
-
None of the above apply: a decision is made to proceed with further, non-invasive, investigations such as specialised imaging scans or MEG.
The main aim of the non-invasive investigations is to provide further information about the likely site of the seizure focus before invasive testing is undertaken. Results of non-invasive investigations can provide information to inform the scope and positioning of any invasive EEG investigations. The results may inform the decisions as to whether to proceed with invasive EEG monitoring, proceed to surgery, or to halt the pre-surgical evaluation. Patients may undergo some or all of the following investigations:
-
High density scalp EEG (HD-EEG): Uses surface electrodes as in routine EEG, but differs from routine EEG by using more electrode contacts and sophisticated data analysis strategies.
-
Magnetoencephalography (MEG): Measures the magnetic fields produced by electrical activity in the brain using sensitive devices such as superconducting quantum interference devices (SQUIDs).
-
Magnetic source imaging (MSI): MEG co-registered with MRI. The two sets of data are combined by measuring the location of a common set of points of reference; these are marked during MRI with lipid markers, and with electrified coils of wire that give off magnetic fields during MEG.
-
Neuroimaging: Can provide information about structural and/or functional abnormalities. Some imaging technologies, such as functional MRI (fMRI), identify where important functions are located so that they can be avoided during surgery. Although these are important in the work-up for surgery, only those imaging technologies that are used to identify the location of potential foci are being considered in this review. Structural imaging technologies include routine (T1, T2 and FLAIR; used in the UK prior to invasive EEG techniques) and volumetric MRI, quantitative T2 measurements and magnetisation transfer imaging (MTI). Functional imaging measure physiological activities such as blood flow and metabolism; changes in these activities can provide information about the site of seizure onset. Functional technologies include single photon emission computerised tomography (SPECT), positron emission tomography (PET), and magnetic resonance spectroscopy (MRS). Some technologies can give information on both structure and function, such as diffuser tensor imaging (DTI or diffusion MRI).
-
Volumetric MRI examination: Here, stereological techniques are used to estimate the volume of brain structures, most commonly the hippocampus, amygdala and temporal lobe.3 Differences in volume (usually a reduction) when compared with normative data suggest focal pathology which may be the site of the seizure onset. Patients may have normal volumes, a unilateral abnormality or bilateral abnormalities, which should be taken into account when assessing this technology. In addition, volumetric information is usually interpreted in conjunction with quantitative T2 data.
-
Quantitative T2 measurements: This technique is usually applied to the hippocampus where mesial temporal lobe sclerosis is associated with high signal on T2 weighted images. This change can be measured quantitatively by measuring the T2 relaxation time15 and making comparisons with normative data. As with volumetric examinations, abnormalities may be absent, unilateral or bilateral.
-
MTI: High-resolution imaging (with and without surface coils) with post processing methods such as voxel-based morphometry, fractal analysis and curvilinear reconstruction of 3D magnetic resonance imaging, are used to detect subtle abnormalities. They were usually applied on T1-weighted images.
-
SPECT: Uses a radiolabelled compound that binds preferentially to certain areas of the brain, depending on the compounds properties. The most commonly used methods during epilepsy surgery work up are 99Technecium labelled compounds (hexamethylpropylenamine oxime (HMPAO)) and ethyl cysteinate dimer (ECD). Once the tracer has been given there is a maximum 6-hour window in which to do the scan. Scans can be conducted inter-ictally (not during a seizure), but more reliable information about the site of seizure onset is provided by injecting the radiolabelled compound at the start of a seizure (ictal) or just after it (post-ictal). Scans show an area of increased uptake at the site of seizure activity. Patients require simultaneous video-EEG monitoring and the presence of a member of staff who can give the radiolabelled compound as soon as the seizure starts.
-
Subtraction ictal SPECT co-registered with MRI (SISCOM): Subtracts the results of ictal and interictal SPECT images, and overlays the result on an MRI image.
-
PET: Uses radiolabelled tracers; 15O labelled water is used to assess blood flow (inter-ictal only), [18F]fluorodeoxyglucose (18F-FDG) for to assess glucose metabolism (ictal and post-ictal), and flumazenil to visualize the distribution of GABAA receptors in the brain. PET provides better spatial resolution than SPECT. FDG-PET is thought to provide more reliable results and provide better spatial resolution and is thus more commonly used in selection for surgery.
-
MRS: An MR technology used inter-ictally to measure the relative concentration of certain molecules in an attempt to find focal abnormalities consistent with the seizure focus. Proton spectroscopy can provide information about N-acetylaspartate, creatine lactate and choline containing compounds whilst 31phosphorus spectroscopy provides information about phosphorus containing compounds such as adenosine triphosphate.
-
DTI: An MR technique quantitatively measures the magnitude and directionality of diffusion in a three dimensional space.
In the majority of cases confirmation of the focus by means of invasive EEG is considered necessary. The main invasive EEG options are:
-
Depth electrodes: Wires are inserted into the brain via a small hole drilled into the skull.
-
Subdural strips: A strip of electrodes is placed over an area of the cerebral cortex, usually inserted via a burr-hole.
-
Subdural mats: A craniotomy is performed and a grid of electrodes placed over an area of the cerebral cortex.
-
Foramen ovale (FO) electrodes: Wires are passed through the FO to lie under the mesial temporal lobe. These are rarely used in current practice, but given that a number of studies are expected to be restricted to patients with temporal lobe epilepsy, they are included in the list of suitable reference standards to maximise the available data in this patient population.
Invasive methods of EEG monitoring are associated with a small risk of infection and haemorrhage.
The cost of these diagnostic technologies can be high, for example, £218 for MRI of one area with contrast (RA02Z),16 £215 for SPECT (RA37Z, Nuclear medicine category 3),16 and £700 to £1000 for PET. 9,17 However, a recent study has shown that if seizure control is achieved after surgery, epilepsy-related costs are reduced in the two years after surgery, from approximately $2,068 to $2,094 (in patients with persisting seizures whether they undergo surgery or not) to $582 in seizure-free patients. 15 Therefore the cost of surgery, and the surgical work-up, may be offset by savings post-surgery in those who attain a seizure-free status.
These diagnostic technologies were reviewed in a previous broad ranging HTA systematic review18 which drew a number of conclusions:
-
Test performance was more promising in studies restricted to patients with temporal lobe epilepsy.
-
Ictal SPECT generally had more correctly localising (70–100%), and fewer non-localising (0–7%), scans in patients with temporal lobe epilepsy than other techniques evaluated.
-
CT and interictal SPECT were relatively poor at localising the seizure focus.
-
Of the 33 evaluations providing data on the association of a localised scan with outcome following surgery, 24 suggested that patients with a correctly or partially localised scan had a better outcome following surgery than those with an incorrectly localised or non-localised scan. However, only three studies showed a significant association between having a localised scan and outcome following surgery.
-
In studies which used multivariate analysis to investigate the association of various imaging techniques with the outcome following surgery, there was a trend for positive localisation of abnormalities to be associated with a beneficial outcome
However, given the limitations of the included studies, the overall conclusion of that review was that the results did little to inform clinical practice. In addition, there was no decision modelling component to the previous review. Therefore, the present work will not attempt to update that review but instead will attempt to address a valuable clinical question outlined in the decision problem below.
Decision problem
In relation to the use of specialised, non-invasive, imaging or MEG technologies in the surgical work-up of epilepsy patients, the decision problem in clinical practice is: ‘What, if any, further diagnostic procedures should be undertaken in individuals for whom there is a reasonable hypothesis for the site of the seizure focus, but in whom that focus has not been identified with surface EEG and MRI?’ The choice will be between:
-
No further investigation and a decision taken not to proceed to surgery; a course of action that may be requested by some patients. This is included in the decision analytical model as a theoretical option for the clinical decision maker.
-
Further investigations before the decision is taken on whether to proceed to surgery:
-
– One of a range of non-invasive tests.
-
– A combination or sequence of non-invasive tests or diagnostic algorithms involving non-invasive technologies.
-
– One of a range of non-invasive tests followed by invasive EEG.
-
– A combination or sequence of non-invasive tests or diagnostic algorithms involving non-invasive technologies, followed by invasive EEG.
-
The measure used to indicate which of these options is most effective will be the long-term outcome for the patient in terms of seizure control and any treatment sequelae.
Objectives
The main aim of the project is to determine the clinical and cost-effectiveness of further non-invasive technologies such as HD-EEG, non-standard MRI, SPECT, PET and MEG over and above routine surface EEG/MRI, to identify people with refractory partial epilepsy who are likely to benefit from surgery and who require confirmation of their focus using invasive EEG methods. A decision analytical model will be developed to investigate the cost-effectiveness of non-invasive techniques, and where possible combinations and sequences of these technologies, to confirm the epileptic focus in people with indeterminate routine surface EEG/MRI results. The proposed structure of the decision analytical model to be developed is given in Figure 1. A review of clinical evidence will be conducted to identify which non-invasive technologies, combination and sequences or technologies, or diagnostic algorithms involving non-invasive technologies can be explored in the model. In addition the cost of conducting further research in terms of expected value of perfect information (EVPI) for the overall decision problem and key parameters will be estimated, which will give the value of eliminating all uncertainty, and uncertainties in particular subsets of parameters.
Methods of synthesising evidence of clinical effectiveness
A systematic review of the relevant evidence for the use of non-invasive diagnostic technologies in the work-up for epilepsy surgery will be undertaken systematically following the general principles recommended in CRD Guidance for Undertaking Systematic Reviews19 and the PRISMA statement. 20
Inclusion and exclusion criteria
Population
People with refractory partial epilepsy being considered for surgery. Studies of patients with generalised epilepsy being considered for surgery will be excluded; surgery in this population is palliative.
Interventions
HD-EEG, any relevant specialist MR technology (volumetric, functional, MRS), SPECT, PET, MEG, SISCOM (SPECT and MRI co-registered), and MSI (MRI and MEG co-registered). All studies using MR technology must employ at least a 1.5 Tesla magnet to be included.
Reference standard
The review will consider two reference standards:
-
The decision to undertake surgery. This must be established using at least an invasive EEG technique (depth electrodes, subdural strips or mats, or foramen ovale electrodes in patients with suspected mesial temporal lobe epilepsy) plus routine MRI. Where a routine MRI was not conducted in addition to invasive EEG, routine MRI must have been evaluated as an index test within the same study (this will allow an assessment of the heterogeneity caused by including patients in the assessment who may have had an epileptic focus identified using routine MRI (and therefore in the UK may not have gone on to receive any of the technologies being investigated). Studies using ‘site of surgery’ as the reference standard must specify that the site of surgery was selected based on the results of an invasive EEG plus MRI (or MRI as an index test). Studies using video EEG as the reference standard must make clear that this was undertaken in combination with invasive EEG electrodes, not surface electrodes. Studies using surface EEG and/or alternative imaging technique(s) without an invasive EEG to make the decision to undertake surgery, will be excluded. Where only a proportion of the population received invasive EEG, results for this subgroup must be reported separately.
-
Outcome following surgery. Studies restricted to patients who had a good (or bad) outcome after surgery will be excluded.
Outcomes
Studies must report sufficient data to construct a contingency table, where the numbers of patients who did, and did not, undergo surgery, and/or the outcome following surgery, can be established for each cell of the contingency table (see Table 1). A good outcome following surgery will be defined as Engel Class I and II or seizure-freedom. Studies reporting standard diagnostic accuracy outcomes, but not the subsequent number of patient who progress to surgery, or the post surgical outcomes, will be excluded.
TP | FP | FN | TN | Partially localised | Wrongly localised | |
---|---|---|---|---|---|---|
Decision: surgery or Good surgical outcome |
A | C | E | G | I | K |
Decision: no surgery or Bad surgical outcome |
B | D | F | H | J | L |
Study designs
Randomised controlled trials (RCTs) directly comparing two or more diagnostic tests of interest that report either the number in each arm that progressed to surgery and/or surgical outcome, will be eligible for inclusion. Prospective diagnostic accuracy studies recruiting at least 20 patients that are of a cohort design will also be eligible for inclusion. Where the direction of data collection is unclear, the studies will be included and their impact on the results investigated. As the decision to go to surgery and/or the outcome following surgery are required outcomes, studies that compare test results in patients suffering epilepsy (cases) with test results in healthy people (controls) in order to determine diagnostic accuracy will be excluded, unless the required outcomes are reported separately for the cases. Where studies report the results of a subgroup of patients that meet the inclusion criteria, or results for the cases of a case-control study, there must be at least 20 patients in the subgroup/20 cases.
Search strategy
Studies will be identified by searching the major medical databases such as MEDLINE, EMBASE, BIOSIS, Pascal, Science Citation Index and LILACS, with no language restriction. In addition, information on studies in progress, unpublished research or research reported in the grey literature was sought by searching a range of relevant databases, including Inside Conferences, metaRegister of Controlled Trials, and NTIS. These searches will be conducted from 2003 to present, with studies conducted prior to these dates identified from the previous HTA monograph. 18 The MEDLINE search strategy is provided in Appendix 1.
Two reviewers will independently screen all titles and abstracts. Full paper manuscripts that may be relevant will be obtained where possible and the relevance of each study assessed independently by two reviewers according to the criteria below. Discrepancies will be resolved by discussion, or by referral to a third reviewer when necessary.
Data extraction strategy
Data relating to study design and quality will be extracted by one reviewer using a standardised data extraction form and checked by a second reviewer. Discrepancies will be resolved by discussion, with involvement of a third reviewer when necessary. If time constraints allow, attempts will be made to contact authors for missing data. Where multiple publications are identified, data from the same study will be extracted and reported as a single study. Where applicable and available, extraction will include data on: study details (e.g. study identifier/EndNote ID, author, country and date conducted, direction of data collection, etc.), patient characteristics (e.g. age, gender, ethnicity, epilepsy classification etc.), details of intervention (specific technology used, for example the Tesla strength of MRI magnets, contrast agent used etc.), study quality, and reported outcomes as specified above.
Quality assessment
The quality of the individual studies will be assessed by one reviewer, and independently checked by a second reviewer. Any disagreements will be resolved by consensus and if necessary a third reviewer will be consulted. The quality of included studies will be assessed using QUADAS;21 the criteria will be adapted as necessary to be review specific.
Methods of synthesis
Key study characteristics, patient outcomes and study quality will be summarised in a narrative and tables. Where appropriate, meta-analysis will be employed to estimate a summary estimate of diagnostic outcomes. Each index test will be analysed separately. Heterogeneity will be investigated using the X2 and I2 tests. Potential sources of heterogeneity will explored, such as differences in the reference standards, patient populations, and the specific technology employed, and study quality. Subgroups of potential interest will be investigated if sufficient data are available; these will include: adults; children; if a surface EEG and/or MRI were performed in addition to the invasive EEG, whether these were localising or not; and whether a lesion was detected on MRI or not. Where applicable, sensitivity analyses will be conducted using only studies that report the use of the technologies in accordance with current UK NHS clinical practices.
Methods of synthesising evidence of cost-effectiveness
A systematic review of existing cost-effectiveness evidence on the cost-effectiveness of neuroimaging assessments will be undertaken and critically appraised using conventional c checklists. Subject to available data, a de novo cost-effectiveness model will also be developed.
Identifying and systematically reviewing published cost-effectiveness studies
A systematic review of existing published studies reporting the cost-effectiveness of neuroimaging assessments in people with refractory epilepsy being considered for surgery. Systematic searches will be undertaken using the following databases will be searched: MEDLINE, EMBASE, CENTRAL and EconLit. In addition, searches of NHS EED will be conducted, along with a search of the Economics Working Papers archive (IDEAS). A broad range of studies will be considered in the assessment of cost-effectiveness including economic evaluations conducted alongside trials, modelling studies and analyses of administrative databases. Only full economic evaluations that compare two or more options and consider both costs and consequences (including cost-effectiveness, cost-utility and cost-benefit analyses) will be included in the review of economic literature.
The quality of the cost-effectiveness studies will be assessed according to a checklist updated from that developed by Drummond et al. (2005)22 and Philips et al. (2002). 2 This checklist will reflect the criteria for economic evaluation detailed in the methodological guidance developed by the National Institute for Health and Clinical Excellence. This information will be tabulated and summarised within the text of the report. In particular information will be extracted on the comparators, study population, main analytic approaches (e.g. patient-level analysis/decision-analytic modelling), primary outcome specified for the economic analysis, details of adjustment for quality-of life, direct costs (medical and non-medical) and productivity costs, estimates of incremental cost-effectiveness and approaches to quantifying decision uncertainty (e.g. deterministic/probabilistic sensitivity analysis).
The review will examine existing decision-analytic models in detail, with the aim of identifying important structural assumptions, highlighting key areas of uncertainty and outlining the potential issues of generalising from the results of existing models. This review will be used to identify the central issues associated with adapting existing decision models to address the specific research question posed and to assist in the development of a new decision model drawing on the issues identified in the clinical and cost-effectiveness review. The presence of any data gaps (e.g. resource use data) that may need to be filled during the development of the model will be identified and additional searches may be required.
Development of a new decision-analytic model
Subject to the availability of appropriate data, a decision-analytic model will be developed to estimate the cost-effectiveness of alternative approaches incorporating neuroimaging assessments. The decision problem will focus on alternative approaches to managing patients in whom surface EEG and standard MRI do not identify a focus, or there is some question as to the accuracy of the focus identified. The cost-effectiveness model will evaluate the cost effectiveness of SPECT, MEG, PET and other new forms of enhanced MR imaging compared with invasive EEG and also a strategy of medical therapy alone for this population (Figure 1). Where suitable data exist, alternative diagnostic strategies will be included as single strategies or as part of a potential sequence e.g. use of PET alone or use of PET plus invasive EEG versus invasive EEG or medical therapy alone. The final strategies included in the model will be based on the findings of the clinical effectiveness review and wider clinical input.
The specific objectives of the cost-effectiveness analysis are:
-
To structure an appropriate decision model to evaluate the diagnostic accuracy for site localisation and subsequent impact on surgical decision making and outcome after epilepsy surgery of the alternative diagnostic algorithms.
-
To populate this model using the most appropriate data identified systematically from published literature and routine data sources.
-
To relate intermediate outcomes from the clinical effectiveness review (e.g. site localisation accuracy, proportion of patients considered eligible for surgery and seizure outcomes) to final health outcomes, expressed in terms of quality-adjusted life years (QALYs). This is necessary in order to provide decision makers with an indication of the health gain achieved by each intervention, relative to its additional cost, in units which permit comparison with other uses of health service resources.
-
To estimate the mean cost-effectiveness of alternative diagnostic algorithms compared with invasive EEG or medical therapy alone, in subjects with refractory epilepsy being considered for surgery and whose routine surface EEG and standard MRI do not identify a focus, or there is some question as to the accuracy of the focus identified. This will be based on an assessment of NHS and Personal Social Service costs and QALYs.
-
To characterise the uncertainty in the data used to populate the model and to present the uncertainty in these results to decision makers. A probabilistic model will be developed which requires that each input in the model is entered as an uncertain, rather than a fixed, parameter. Using Monte Carlo simulation, this parameter uncertainty, is translated into uncertainty in the overall results. This ultimately helps decision makers understand the probability that, in choosing to fund an intervention, they are making the wrong decision – that is, decision uncertainty. This is presented using cost-effectiveness acceptability curves which show the probability that each intervention is cost-effective conditional on a range of possible threshold values which NHS decision makers attach to an additional QALY.
The model structure will be developed during the review period. However, it is anticipated that the model will comprise an initial short-term decision tree to reflect the diagnostic accuracy and subsequent impact on surgical decision making and short term seizure outcomes (surgical and non-surgical) and a separate Markov model to capture the longer term impact of these outcomes in terms of associated resource utilisation and quality of life. A basic schematic of the proposed model structure is given in Figure 1.
It is anticipated that additional systematic searches will be necessary to populate specific parameter inputs and assumptions applied in the longer-term Markov model. In order to estimate QALYs required for the cost-effectiveness analysis, it will be necessary to systematically search for appropriate published utility or preference scores related to different surgical outcomes. Additional evidence may also be needed to supplement the proposed clinical effectiveness review to appropriately model longer-term seizure outcomes. This additional evidence will be sought from previously published meta-analyses of long-term seizure outcomes following epilepsy surgery (e.g. Tellez-Zenteno et al. 2005,23 200724).
Resource utilisation will reflect the inputs associated with the diagnostic tests themselves, surgery, medication and epilepsy-related events. Resource-use data will be informed from the clinical and cost-effectiveness reviews, meta-analyses of long term seizure outcomes and expert clinical opinion where necessary. These data will be combined with national sources of cost data (e.g. NHS Reference Costs, British National Formulary, etc.) in order to estimate the total costs associated with each strategy considered.
To consider future research priorities in the NHS, the model will also be used to undertake analyses of the expected value of information. The expected value of perfect information (EVPI) will be estimated for the overall decision problem and for key parameters. EVPI represents the expected costs of decision uncertainty since perfect information would eliminate the possibility of making the wrong decision. Hence, EVPI for the overall decision problem represents the value of eliminating all uncertainty and EVPI for key parameters (termed partial EVPI) represents the value of eliminating uncertainties in particular subsets of parameters. Separate analyses will be undertaken to reflect the variability considered in the decision model itself. Per patient EVPI estimates will be scaled up to reflect the relevant UK population size and will adopt an appropriate time-horizon.
EVPI also represents the maximum amount that a decision-maker should be willing to pay for additional evidence to inform this decision in the future. EVPI provides an upper bound on the value of additional research. This valuation provides an initial hurdle, acting as a necessary requirement for determining the potential efficiency of further primary research. Applying this decision rule, additional research should only be considered if the EVPI exceeds the expected cost of the research. In addition to providing a global estimate of the total cost of uncertainty related to all inputs in the model, EVPI can also be estimated for individual parameters (and groups of parameters) contained in the model. The objective of this analysis (termed partial EVPI) is to identify the model parameters where it would be most worthwhile obtaining more precise estimates.
The results from the clinical effectiveness review and the EVPI results will be used to identify future research recommendations.
TAR Centre
The Technology Assessment Review team at the University of York is drawn from two specialist centres: the Centre for Reviews and Dissemination (CRD) and the Centre for Health Economics (CHE).
CRD undertakes reviews of research about the effects of interventions used in health and social care (www.york.ac.uk/inst/crd). The centre maintains various databases, provides an enquiry service and disseminates results of research to NHS decision makers.
CHE undertakes research and training in all areas of health economics (www.york.ac.uk/inst/che). The bulk of the input into the TARs comes from the programme for economic evaluation and health technology assessment which specialises in decision analysis and Bayesian methods in economic evaluations (see http://www.york.ac.uk/inst/che/teehta.htm).
Recent TARs undertaken by CRD/CHE at York relate to aldosterone treatment for post-MI heart failure, treatments for bipolar disorder, sugammadex for the reversal of muscle relaxation in general anaesthesia, and photodynamic therapy in the treatment of specified cancer sites.
Expertise in the TAR team and team contributions
Jane Burch, Research Fellow, Centre for Reviews and Dissemination (jb67@york.ac.uk). Over six years experience in systematic reviews and systematic review methodology. Has worked on systematic reviews for NICE, the HTA programme and the NHS Cancer Screening Programmes. Responsible for writing the protocol, all aspects of the clinical effectiveness review and co-ordinating the production of the final report.
Stephen Palmer, Senior Research Fellow, Centre for Health Economics (sjp21@york.ac.uk). Over 15 years experience in economic evaluation and health technology assessment. Stephen has particular expertise in the use of decision-analytic and value of information approaches. He has recently undertaken an evaluation of the clinical and cost-effectiveness of novel biomarkers in coronary heart disease for the NIHR-HTA programme. Responsible for writing the protocol, managing the cost-effectiveness review and development of the cost-effectiveness model.
Junior health economist: To be decided.
Junior reviewer: To be decided.
Professor Tony Marson, Consultant Neurologist, University of Liverpool (a.g.marson@liverpool.ac.uk). Neurologist with a particular clinical and research interest in epilepsy and leads the epilepsy research team at the University of Liverpool. Also the coordinating editor of the Cochrane Epilepsy Group, lead for the UK Epilepsy Research Network, and deputy director of MRC North West Hub for Trial Methodology Research. Will be providing clinical advice throughout the review, and providing comments on the draft protocol and report.
Dr Udo Wieshmann, Consultant Neurologist, Walton Centre, Liverpool (udo.wieshmann@thewaltoncentre.nhs.uk). Neurologist with an interest in epilepsy and neuroimaging, and part of the multidisciplinary epilepsy surgery team at the Walton Centre which evaluates patients for epilepsy surgery. Will be providing clinical advice throughout the review, and providing comments on the draft protocol and report.
Professor Mark Richardson, Consultant Neurologist specialising in epilepsy, University of Liverpool (mark.richardson@kcl.ac.uk). Neurologist with a particular clinical and research interest in epilepsy and specialist knowledge of structural and functional neuroimaging, transcranial magnetic brain stimulation and EEG. Will be providing clinical advice throughout the review, and providing comments on the draft protocol and report.
Dr Simon Hughes, Consultant in Radiology and Nuclear Medicine, Royal Victoria Hospital, Belfast (simon.hughes@belfasttrust.hscni.net). Extensive experience in neuroimaging with a particular expertise in PET. Will be providing clinical advice throughout the review, and providing comments on the draft protocol and report.
Ms Kath Wright, Information Service Manager, Centre for Reviews and Dissemination (kew5@york.ac.uk). Over 10 years experience as an information specialist, conducting literature searching for systematic reviews, including searches for a number of health technology assessments, and providing support for research staff. Responsible for devising the search strategy, conducting the literature searches and maintaining the literature database.
Nerys Woolacott, Senior Research Fellow, Centre for Reviews and Dissemination (nw11@york.ac.uk). Nine years’ experience in health technology assessment, systematic reviews and review methodology. She has produced and managed a number of technology assessments and systematic reviews for HTA, NICE, Department of Health and others. She has contributed to the development of the protocol and will provide input at all stages of the project, management of the clinical evidence review and will contribute to and comment on various drafts of the report.
Competing interests of authors
None of the authors have any conflicts of interest.
Timetable/milestones
Submission of: | |
---|---|
Team submit final protocol | February 2010 |
Expected date for HTA to send comments on draft protocol | By June 2010 |
Team submit final protocol to HTA | 2 weeks after receiving comments |
Team submit assessment report to HTA | 6 months after final protocol approval |
References
- Epilepsy Foundation n.d. URL: http://www.epilepsyfoundation.org/.
- Philips Z, Ginnelly L, Sculpher M, Claxton K, Golder S, Riemsma R, et al. Review of guidelines for good practice in decision-analytic modelling in health technology assessment. Health Technol Assess 2002;8:1-158.
- Pugliatti M, Beghi E, Forsgren L, Ekman M, Sobocki P. Estimating the cost of epilepsy in Europe: a review with economic modeling. Epilepsia 2007;48:2224-33.
- Tidman L, Saravanan K, Gibbs J. Epilepsy in mainstream and special educational primary school settings. Seizure 2003;12:47-51.
- National Society for Epilepsy n.d. URL: http://www.epilepsysociety.org.uk/Homepage.
- HESonline n.d. URL: http://www.hesonline.nhs.uk/Ease/servlet/ContentServer?siteID=1937.
- NHS Choices . Epilepsy n.d. URL: http://www.nhs.uk/epilepsy.
- Jacoby A, Buck D, Baker G, McNamee P, Graham-Jones TS, Chadwick D. Uptake and costs of care for epilepsy: findings from a UK regional study. Epilepsia 1998;39:776-86.
- Facey K, Bradbury I, Laking G, Payne E. Overview of the clinical effectiveness of positron emission tomography imaging in selected cancers. Health Technol Assess 2007;11. URL: http://www.hta.ac.uk/fullmono/mon1144.pdf.
- Wiebe S, Blume WT, Irvin JPG, Eliasziw M. A randomized controlled trial of surgery for temporal-lobe epilepsy. N Engl J Med 2001;345:311-18.
- Lhatoo SD, Solomon JK, McEvoy AW, Kitchen ND, Shorvon SD, Sander JW. A prospective study of the requirement for and the provision of epilepsy surgery in the United Kingdom. Epilepsia 2003;44:673-6.
- Yogarajah M, Powell HWR, Heaney D, Smith SJM, Duncan JS, Sisodiya SM. Long term monitoring in refractory epilepsy: the Gowers Unit experience. J Neurol Neurosurg Psychiatry 2009;80:305-10.
- Epilepsy Action . Anti-Epileptic Medication Available in the United Kingdom 2009. URL: http://www.epilepsy.org.uk/info/drugslist.html.
- Epilepsy Action n.d. URL: http://www.epilepsy.org.uk/.
- Langfitt JT, Holloway RG, McDermott MP, Messing S, Sarosky K, Berg AT, et al. Health care costs decline after successful epilepsy surgery. Neurology 2007;68:1290-8.
- Department of Health . Reference Costs 2007 8 (dh 098948) n.d. URL: http://www.dh.gov.uk/en/Publicationsandstatistics/Publications/PublicationsPolicyAndGuidance/DH_082571.
- Cancer Research UK . PET Scan n.d. URL: http://www.cancerhelp.org.uk/about-cancer/tests/pet-scan2#cost.
- Whiting P, Gupta R, Burch J, Mujica Mota RE, Wright K, Marson A, et al. A systematic review of the effectiveness and cost-effectiveness of neuroimaging assessments used to visualise the seizure focus in people with refractory epilepsy being considered for surgery. Health Technol Assess 2006;10.
- Centre for Reviews and Dissemination . Systematic reviews. CRD’s Guidance for Undertaking Reviews in Health Care 2009.
- Liberati A, Altman DG, Tetzlaff J, Mulrow C, Gøtzsche PC, Ioannidis JP, et al. The PRISMA Statement for Reporting Systematic Reviews and Meta-Analyses of studies that evaluate health care interventions: explanation and elaboration. PLoS Med 2009;6:1-28.
- Whiting P, Rutjes AWS, Reitsma JB, Bossuyt PMM, Kleijnen J. The development of QUADAS: a tool for the quality assessment of studies of diagnostic accuracy included in systematic reviews. BMC Med Res Methodol 2003;3.
- Drummond M, Sculpher M, Torrence G, O’Brien B, Stoddart G. Methods for the economic evaluation of health care programmes. Oxford: Oxford University Press; 2005.
- Tellez-Zenteno J, Dhar R, Wiebe S. Long-term seizure outcomes following epilepsy surgery: a systematic review and meta-analysis. Brain 2005;128:1188-98.
- Tellez-Zenteno J, Dhar R, Hernandez-Ronquillo L, Wiebe S. Long-term outcomes in epilepsy surgery: antiepileptic drugs, mortality, cognitive and psychosocial aspects. Brain 2007;130:334-45.
Appendix 1: Example MEDLINE search strategy
Database: Ovid MEDLINE(R) <1996 to November Week 3 2009>
Search strategy:
-
exp Epilepsy/
-
epilep$.ti,ab.
-
1 or 2
-
exp Magnetic Resonance Imaging/
-
exp Magnetic Resonance Spectroscopy/
-
Magnetoencephalography/
-
(magnetic resonance tomograph$ or magnetic resonance scan$ or magnetic resonance imag$ or magnetic resonance spectroscop$ or magnetoencephalograph$ or magnetic source imag$).ti,ab.
-
(mr tomograph$ or mr scan$ or mr imag$ or mr spectroscop$).ti,ab.
-
(fmri or mri or nmr or mrs or meg or msi).ti,ab.
-
exp Tomography, Emission-Computed, Single-Photon/
-
(spect or single photon emission computed tomograph$).ti,ab.
-
volumetric exam$.ti,ab.
-
quantitative t2 measure$.ti,ab.
-
voxel based morphomet$.ti,ab.
-
diffusion weight$ imag$.ti,ab.
-
(diffusion weight$ imag$ or dwi).ti,ab.
-
(diffusion tensor imag$ or dti).ti,ab.
-
(fluid attentuated inversion recovery or flair).ti,ab.
-
4 or 5 or 6 or 7 or 8 or 9 or 10 or 11 or 12 or 13 or 14 or 15 or 16 or 17 or 18
-
3 and 19
-
limit 20 to humans
List of abbreviations
- 18F-FDG
- [18F]-2-fluorodeoxy-d-glucose
- AED
- antiepileptic drug
- ATL
- anterior temporal lobectomy
- CEAC
- cost-effectiveness acceptability curve
- CHE
- Centre for Health Economics
- Cho
- choline
- CI
- confidence interval
- Cr
- creatine
- CRD
- Centre for Reviews and Dissemination
- DS
- disabling seizure
- ECD
- 99mTc-ethyl cysteinate dimer
- EEG
- electroencephalography
- EQ-5D
- European Quality of Life-5 Dimensions
- EVPI
- expected value of information
- FDG-PET
- fluorodeoxyglucose positron emission tomography
- fMRI
- functional magnetic resonance imaging
- GABAA
- ionotropic gamma-aminobutyric acid A
- HD-EEG
- high-density electroencephalography
- HMPAO
- hexamethylpropyleneamine oxime
- HRQoL
- health-related quality of life
- HS
- hippocampal sclerosis
- HTA
- health technology assessment
- ICER
- incremental cost-effectiveness ratio
- iEEG
- invasive/intracranial electroencephalography
- IPD
- individual patient data
- MEG
- magnetoencephalography
- MeSH
- medical subject headings in the MEDLINE thesaurus
- MM
- medical management
- MRI
- magnetic resonance imaging
- MRS
- magnetic resonance spectroscopy
- MSI
- magnetic source imaging
- MTI
- magnetisation transfer imaging
- MTS
- mesial (medial) temporal sclerosis
- N/A
- not applicable
- NAA
- N-acetylaspartate
- NICE
- National Institute for Health and Clinical Excellence
- NIRS
- near-infrared spectroscopy
- NR
- not reported
- OR
- odds ratio
- pAED
- proportion of patients each year who discontinue AED treatment after ≥ 3 years of being seizure free
- PET
- positron emission tomography
- post-ictal
- period after a seizure has ceased
- PRISMA
- Preferred Reporting Items for Systematic Reviews and Meta-Analyses
- PSA
- probabilistic sensitivity analysis
- PSS
- Personal Social Services
- QALY
- quality-adjusted life-year
- QoL
- quality of life
- QUADAS
- Quality Assessment of Diagnostic Accuracy Studies
- RCT
- randomised controlled trial
- SAH
- selective amygdalohippocampectomy
- SD
- standard deviation
- SE
- standard error
- SF
- seizure free
- SISCOM
- subtraction ictal SPECT coregistered with magnetic resonance imaging
- SPECT
- single-photon emission computed tomography
- SQUID
- superconducting quantum interference device
- TLE
- temporal lobe epilepsy
- TP
- transition probability
- vMRI
- volumetric magnetic resonance imaging
All abbreviations that have been used in this report are listed here unless the abbreviation is well known (e.g. NHS), or it has only been used once, or it is a non-standard abbreviation used only in figures/tables/appendices in which case the abbreviation is defined in the figure or table legend.
Notes
Health Technology Assessment programme
-
Director, NIHR HTA programme, Professor of Clinical Pharmacology, Department of Pharmacology and Therapeutics, University of Liverpool
-
Professor of Dermato-Epidemiology, Centre of Evidence-Based Dermatology, University of Nottingham
Prioritisation Group
-
Director, NIHR HTA programme, Professor of Clinical Pharmacology, Department of Pharmacology and Therapeutics, University of Liverpool
-
Professor Imti Choonara, Professor in Child Health, Academic Division of Child Health, University of Nottingham
Chair – Pharmaceuticals Panel
-
Dr Bob Coates, Consultant Advisor – Disease Prevention Panel
-
Dr Andrew Cook, Consultant Advisor – Intervention Procedures Panel
-
Dr Peter Davidson, Director of NETSCC, Health Technology Assessment
-
Dr Nick Hicks, Consultant Adviser – Diagnostic Technologies and Screening Panel, Consultant Advisor–Psychological and Community Therapies Panel
-
Ms Susan Hird, Consultant Advisor, External Devices and Physical Therapies Panel
-
Professor Sallie Lamb, Director, Warwick Clinical Trials Unit, Warwick Medical School, University of Warwick
Chair – HTA Clinical Evaluation and Trials Board
-
Professor Jonathan Michaels, Professor of Vascular Surgery, Sheffield Vascular Institute, University of Sheffield
Chair – Interventional Procedures Panel
-
Professor Ruairidh Milne, Director – External Relations
-
Dr John Pounsford, Consultant Physician, Directorate of Medical Services, North Bristol NHS Trust
Chair – External Devices and Physical Therapies Panel
-
Dr Vaughan Thomas, Consultant Advisor – Pharmaceuticals Panel, Clinical
Lead – Clinical Evaluation Trials Prioritisation Group
-
Professor Margaret Thorogood, Professor of Epidemiology, Health Sciences Research Institute, University of Warwick
Chair – Disease Prevention Panel
-
Professor Lindsay Turnbull, Professor of Radiology, Centre for the MR Investigations, University of Hull
Chair – Diagnostic Technologies and Screening Panel
-
Professor Scott Weich, Professor of Psychiatry, Health Sciences Research Institute, University of Warwick
Chair – Psychological and Community Therapies Panel
-
Professor Hywel Williams, Director of Nottingham Clinical Trials Unit, Centre of Evidence-Based Dermatology, University of Nottingham
Chair – HTA Commissioning Board
Deputy HTA Programme Director
HTA Commissioning Board
-
Professor of Dermato-Epidemiology, Centre of Evidence-Based Dermatology, University of Nottingham
-
Professor of Bio-Statistics, Department of Public Health and Epidemiology, University of Birmingham
-
Professor of Clinical Pharmacology, Director, NIHR HTA programme, Department of Pharmacology and Therapeutics, University of Liverpool
-
Professor Zarko Alfirevic, Head of Department for Women’s and Children’s Health, Institute of Translational Medicine, University of Liverpool
-
Professor Judith Bliss, Director of ICR-Clinical Trials and Statistics Unit, The Institute of Cancer Research
-
Professor David Fitzmaurice, Professor of Primary Care Research, Department of Primary Care Clinical Sciences, University of Birmingham
-
Professor John W Gregory, Professor in Paediatric Endocrinology, Department of Child Health, Wales School of Medicine, Cardiff University
-
Professor Steve Halligan, Professor of Gastrointestinal Radiology, Department of Specialist Radiology, University College Hospital, London
-
Professor Angela Harden, Professor of Community and Family Health, Institute for Health and Human Development, University of East London
-
Dr Joanne Lord, Reader, Health Economics Research Group, Brunel University
-
Professor Stephen Morris, Professor of Health Economics, University College London, Research Department of Epidemiology and Public Health, University College London
-
Professor Dion Morton, Professor of Surgery, Academic Department of Surgery, University of Birmingham
-
Professor Gail Mountain, Professor of Health Services Research, Rehabilitation and Assistive Technologies Group, University of Sheffield
-
Professor Irwin Nazareth, Professor of Primary Care and Head of Department, Department of Primary Care and Population Sciences, University College London
-
Professor E Andrea Nelson, Professor of Wound Healing and Director of Research, School of Healthcare, University of Leeds
-
Professor John David Norrie, Director, Centre for Healthcare Randomised Trials, Health Services Research Unit, University of Aberdeen
-
Professor Barney Reeves, Professorial Research Fellow in Health Services Research, Department of Clinical Science, University of Bristol
-
Professor Peter Tyrer, Professor of Community Psychiatry, Centre for Mental Health, Imperial College London
-
Professor Martin Underwood, Professor of Primary Care Research, Warwick Medical School, University of Warwick
-
Professor Caroline Watkins, Professor of Stroke and Older People’s Care, Chair of UK Forum for Stroke Training, Stroke Practice Research Unit, University of Central Lancashire
-
Dr Duncan Young, Senior Clinical Lecturer and Consultant, Nuffield Department of Anaesthetics, University of Oxford
-
Dr Tom Foulks, Medical Research Council
-
Dr Kay Pattison, Senior NIHR Programme Manager, Department of Health
HTA Clinical Evaluation and Trials Board
-
Director, Warwick Clinical Trials Unit, Warwick Medical School, University of Warwick and Professor of Rehabilitation, Nuffield Department of Orthopaedic, Rheumatology and Musculoskeletal Sciences, University of Oxford
-
Professor of the Psychology of Health Care, Leeds Institute of Health Sciences, University of Leeds
-
Director, NIHR HTA programme, Professor of Clinical Pharmacology, University of Liverpool
-
Professor Keith Abrams, Professor of Medical Statistics, Department of Health Sciences, University of Leicester
-
Professor Martin Bland, Professor of Health Statistics, Department of Health Sciences, University of York
-
Professor Jane Blazeby, Professor of Surgery and Consultant Upper GI Surgeon, Department of Social Medicine, University of Bristol
-
Professor Julia M Brown, Director, Clinical Trials Research Unit, University of Leeds
-
Professor Alistair Burns, Professor of Old Age Psychiatry, Psychiatry Research Group, School of Community-Based Medicine, The University of Manchester & National Clinical Director for Dementia, Department of Health
-
Dr Jennifer Burr, Director, Centre for Healthcare Randomised trials (CHART), University of Aberdeen
-
Professor Linda Davies, Professor of Health Economics, Health Sciences Research Group, University of Manchester
-
Professor Simon Gilbody, Prof of Psych Medicine and Health Services Research, Department of Health Sciences, University of York
-
Professor Steven Goodacre, Professor and Consultant in Emergency Medicine, School of Health and Related Research, University of Sheffield
-
Professor Dyfrig Hughes, Professor of Pharmacoeconomics, Centre for Economics and Policy in Health, Institute of Medical and Social Care Research, Bangor University
-
Professor Paul Jones, Professor of Respiratory Medicine, Department of Cardiac and Vascular Science, St George‘s Hospital Medical School, University of London
-
Professor Khalid Khan, Professor of Women’s Health and Clinical Epidemiology, Barts and the London School of Medicine, Queen Mary, University of London
-
Professor Richard J McManus, Professor of Primary Care Cardiovascular Research, Primary Care Clinical Sciences Building, University of Birmingham
-
Professor Helen Rodgers, Professor of Stroke Care, Institute for Ageing and Health, Newcastle University
-
Professor Ken Stein, Professor of Public Health, Peninsula Technology Assessment Group, Peninsula College of Medicine and Dentistry, Universities of Exeter and Plymouth
-
Professor Jonathan Sterne, Professor of Medical Statistics and Epidemiology, Department of Social Medicine, University of Bristol
-
Mr Andy Vail, Senior Lecturer, Health Sciences Research Group, University of Manchester
-
Professor Clare Wilkinson, Professor of General Practice and Director of Research North Wales Clinical School, Department of Primary Care and Public Health, Cardiff University
-
Dr Ian B Wilkinson, Senior Lecturer and Honorary Consultant, Clinical Pharmacology Unit, Department of Medicine, University of Cambridge
-
Ms Kate Law, Director of Clinical Trials, Cancer Research UK
-
Dr Morven Roberts, Clinical Trials Manager, Health Services and Public Health Services Board, Medical Research Council
Diagnostic Technologies and Screening Panel
-
Scientific Director of the Centre for Magnetic Resonance Investigations and YCR Professor of Radiology, Hull Royal Infirmary
-
Professor Judith E Adams, Consultant Radiologist, Manchester Royal Infirmary, Central Manchester & Manchester Children’s University Hospitals NHS Trust, and Professor of Diagnostic Radiology, University of Manchester
-
Mr Angus S Arunkalaivanan, Honorary Senior Lecturer, University of Birmingham and Consultant Urogynaecologist and Obstetrician, City Hospital, Birmingham
-
Dr Diana Baralle, Consultant and Senior Lecturer in Clinical Genetics, University of Southampton
-
Dr Stephanie Dancer, Consultant Microbiologist, Hairmyres Hospital, East Kilbride
-
Dr Diane Eccles, Professor of Cancer Genetics, Wessex Clinical Genetics Service, Princess Anne Hospital
-
Dr Trevor Friedman, Consultant Liason Psychiatrist, Brandon Unit, Leicester General Hospital
-
Dr Ron Gray, Consultant, National Perinatal Epidemiology Unit, Institute of Health Sciences, University of Oxford
-
Professor Paul D Griffiths, Professor of Radiology, Academic Unit of Radiology, University of Sheffield
-
Mr Martin Hooper, Public contributor
-
Professor Anthony Robert Kendrick, Associate Dean for Clinical Research and Professor of Primary Medical Care, University of Southampton
-
Dr Nicola Lennard, Senior Medical Officer, MHRA
-
Dr Anne Mackie, Director of Programmes, UK National Screening Committee, London
-
Mr David Mathew, Public contributor
-
Dr Michael Millar, Consultant Senior Lecturer in Microbiology, Department of Pathology & Microbiology, Barts and The London NHS Trust, Royal London Hospital
-
Mrs Una Rennard, Public contributor
-
Dr Stuart Smellie, Consultant in Clinical Pathology, Bishop Auckland General Hospital
-
Ms Jane Smith, Consultant Ultrasound Practitioner, Leeds Teaching Hospital NHS Trust, Leeds
-
Dr Allison Streetly, Programme Director, NHS Sickle Cell and Thalassaemia Screening Programme, King’s College School of Medicine
-
Dr Matthew Thompson, Senior Clinical Scientist and GP, Department of Primary Health Care, University of Oxford
-
Dr Alan J Williams, Consultant Physician, General and Respiratory Medicine, The Royal Bournemouth Hospital
-
Dr Tim Elliott, Team Leader, Cancer Screening, Department of Health
-
Dr Joanna Jenkinson, Board Secretary, Neurosciences and Mental Health Board (NMHB), Medical Research Council
-
Professor Julietta Patrick, Director, NHS Cancer Screening Programme, Sheffield
-
Dr Kay Pattison, Senior NIHR Programme Manager, Department of Health
-
Professor Tom Walley, CBE, Director, NIHR HTA programme, Professor of Clinical Pharmacology, University of Liverpool
-
Dr Ursula Wells, Principal Research Officer, Policy Research Programme, Department of Health
Disease Prevention Panel
-
Professor of Epidemiology, University of Warwick Medical School, Coventry
-
Dr Robert Cook, Clinical Programmes Director, Bazian Ltd, London
-
Dr Colin Greaves, Senior Research Fellow, Peninsula Medical School (Primary Care)
-
Mr Michael Head, Public contributor
-
Professor Cathy Jackson, Professor of Primary Care Medicine, Bute Medical School, University of St Andrews
-
Dr Russell Jago, Senior Lecturer in Exercise, Nutrition and Health, Centre for Sport, Exercise and Health, University of Bristol
-
Dr Julie Mytton, Consultant in Child Public Health, NHS Bristol
-
Professor Irwin Nazareth, Professor of Primary Care and Director, Department of Primary Care and Population Sciences, University College London
-
Dr Richard Richards, Assistant Director of Public Health, Derbyshire County Primary Care Trust
-
Professor Ian Roberts, Professor of Epidemiology and Public Health, London School of Hygiene & Tropical Medicine
-
Dr Kenneth Robertson, Consultant Paediatrician, Royal Hospital for Sick Children, Glasgow
-
Dr Catherine Swann, Associate Director, Centre for Public Health Excellence, NICE
-
Mrs Jean Thurston, Public contributor
-
Professor David Weller, Head, School of Clinical Science and Community Health, University of Edinburgh
-
Ms Christine McGuire, Research & Development, Department of Health
-
Dr Kay Pattison, Senior NIHR Programme Manager, Department of Health
-
Professor Tom Walley, CBE, Director, NIHR HTA programme, Professor of Clinical Pharmacology, University of Liverpool
External Devices and Physical Therapies Panel
-
Consultant Physician North Bristol NHS Trust
-
Reader in Wound Healing and Director of Research, University of Leeds
-
Professor Bipin Bhakta, Charterhouse Professor in Rehabilitation Medicine, University of Leeds
-
Mrs Penny Calder, Public contributor
-
Dr Dawn Carnes, Senior Research Fellow, Barts and the London School of Medicine and Dentistry
-
Dr Emma Clark, Clinician Scientist Fellow & Cons. Rheumatologist, University of Bristol
-
Mrs Anthea De Barton-Watson, Public contributor
-
Professor Nadine Foster, Professor of Musculoskeletal Health in Primary Care Arthritis Research, Keele University
-
Dr Shaheen Hamdy, Clinical Senior Lecturer and Consultant Physician, University of Manchester
-
Professor Christine Norton, Professor of Clinical Nursing Innovation, Bucks New University and Imperial College Healthcare NHS Trust
-
Dr Lorraine Pinnigton, Associate Professor in Rehabilitation, University of Nottingham
-
Dr Kate Radford, Senior Lecturer (Research), University of Central Lancashire
-
Mr Jim Reece, Public contributor
-
Professor Maria Stokes, Professor of Neuromusculoskeletal Rehabilitation, University of Southampton
-
Dr Pippa Tyrrell, Senior Lecturer/Consultant, Salford Royal Foundation Hospitals’ Trust and University of Manchester
-
Dr Nefyn Williams, Clinical Senior Lecturer, Cardiff University
-
Dr Kay Pattison, Senior NIHR Programme Manager, Department of Health
-
Dr Morven Roberts, Clinical Trials Manager, Health Services and Public Health Services Board, Medical Research Council
-
Professor Tom Walley, CBE, Director, NIHR HTA programme, Professor of Clinical Pharmacology, University of Liverpool
-
Dr Ursula Wells, Principal Research Officer, Policy Research Programme, Department of Health
Interventional Procedures Panel
-
Professor of Vascular Surgery, University of Sheffield
-
Consultant Colorectal Surgeon, Bristol Royal Infirmary
-
Mrs Isabel Boyer, Public contributor
-
Mr Sankaran Chandra Sekharan, Consultant Surgeon, Breast Surgery, Colchester Hospital University NHS Foundation Trust
-
Professor Nicholas Clarke, Consultant Orthopaedic Surgeon, Southampton University Hospitals NHS Trust
-
Ms Leonie Cooke, Public contributor
-
Mr Seumas Eckford, Consultant in Obstetrics & Gynaecology, North Devon District Hospital
-
Professor Sam Eljamel, Consultant Neurosurgeon, Ninewells Hospital and Medical School, Dundee
-
Dr Adele Fielding, Senior Lecturer and Honorary Consultant in Haematology, University College London Medical School
-
Dr Matthew Hatton, Consultant in Clinical Oncology, Sheffield Teaching Hospital Foundation Trust
-
Dr John Holden, General Practitioner, Garswood Surgery, Wigan
-
Dr Fiona Lecky, Senior Lecturer/Honorary Consultant in Emergency Medicine, University of Manchester/Salford Royal Hospitals NHS Foundation Trust
-
Dr Nadim Malik, Consultant Cardiologist/Honorary Lecturer, University of Manchester
-
Mr Hisham Mehanna, Consultant & Honorary Associate Professor, University Hospitals Coventry & Warwickshire NHS Trust
-
Dr Jane Montgomery, Consultant in Anaesthetics and Critical Care, South Devon Healthcare NHS Foundation Trust
-
Professor Jon Moss, Consultant Interventional Radiologist, North Glasgow Hospitals University NHS Trust
-
Dr Simon Padley, Consultant Radiologist, Chelsea & Westminster Hospital
-
Dr Ashish Paul, Medical Director, Bedfordshire PCT
-
Dr Sarah Purdy, Consultant Senior Lecturer, University of Bristol
-
Dr Matthew Wilson, Consultant Anaesthetist, Sheffield Teaching Hospitals NHS Foundation Trust
-
Professor Yit Chiun Yang, Consultant Ophthalmologist, Royal Wolverhampton Hospitals NHS Trust
-
Dr Kay Pattison, Senior NIHR Programme Manager, Department of Health
-
Dr Morven Roberts, Clinical Trials Manager, Health Services and Public Health Services Board, Medical Research Council
-
Professor Tom Walley, CBE, Director, NIHR HTA programme, Professor of Clinical Pharmacology, University of Liverpool
-
Dr Ursula Wells, Principal Research Officer, Policy Research Programme, Department of Health
Pharmaceuticals Panel
-
Professor in Child Health, University of Nottingham
-
Senior Lecturer in Clinical Pharmacology, University of East Anglia
-
Dr Martin Ashton-Key, Medical Advisor, National Commissioning Group, NHS London
-
Dr Peter Elton, Director of Public Health, Bury Primary Care Trust
-
Dr Ben Goldacre, Research Fellow, Division of Psychological Medicine and Psychiatry, King’s College London
-
Dr James Gray, Consultant Microbiologist, Department of Microbiology, Birmingham Children’s Hospital NHS Foundation Trust
-
Dr Jurjees Hasan, Consultant in Medical Oncology, The Christie, Manchester
-
Dr Carl Heneghan, Deputy Director Centre for Evidence-Based Medicine and Clinical Lecturer, Department of Primary Health Care, University of Oxford
-
Dr Dyfrig Hughes, Reader in Pharmacoeconomics and Deputy Director, Centre for Economics and Policy in Health, IMSCaR, Bangor University
-
Dr Maria Kouimtzi, Pharmacy and Informatics Director, Global Clinical Solutions, Wiley-Blackwell
-
Professor Femi Oyebode, Consultant Psychiatrist and Head of Department, University of Birmingham
-
Dr Andrew Prentice, Senior Lecturer and Consultant Obstetrician and Gynaecologist, The Rosie Hospital, University of Cambridge
-
Ms Amanda Roberts, Public contributor
-
Dr Gillian Shepherd, Director, Health and Clinical Excellence, Merck Serono Ltd
-
Mrs Katrina Simister, Assistant Director New Medicines, National Prescribing Centre, Liverpool
-
Professor Donald Singer, Professor of Clinical Pharmacology and Therapeutics, Clinical Sciences Research Institute, CSB, University of Warwick Medical School
-
Mr David Symes, Public contributor
-
Dr Arnold Zermansky, General Practitioner, Senior Research Fellow, Pharmacy Practice and Medicines Management Group, Leeds University
-
Dr Kay Pattison, Senior NIHR Programme Manager, Department of Health
-
Mr Simon Reeve, Head of Clinical and Cost-Effectiveness, Medicines, Pharmacy and Industry Group, Department of Health
-
Dr Heike Weber, Programme Manager, Medical Research Council
-
Professor Tom Walley, CBE, Director, NIHR HTA programme, Professor of Clinical Pharmacology, University of Liverpool
-
Dr Ursula Wells, Principal Research Officer, Policy Research Programme, Department of Health
Psychological and Community Therapies Panel
-
Professor of Psychiatry, University of Warwick, Coventry
-
Consultant & University Lecturer in Psychiatry, University of Cambridge
-
Professor Jane Barlow, Professor of Public Health in the Early Years, Health Sciences Research Institute, Warwick Medical School
-
Dr Sabyasachi Bhaumik, Consultant Psychiatrist, Leicestershire Partnership NHS Trust
-
Mrs Val Carlill, Public contributor
-
Dr Steve Cunningham, Consultant Respiratory Paediatrician, Lothian Health Board
-
Dr Anne Hesketh, Senior Clinical Lecturer in Speech and Language Therapy, University of Manchester
-
Dr Peter Langdon, Senior Clinical Lecturer, School of Medicine, Health Policy and Practice, University of East Anglia
-
Dr Yann Lefeuvre, GP Partner, Burrage Road Surgery, London
-
Dr Jeremy J Murphy, Consultant Physician and Cardiologist, County Durham and Darlington Foundation Trust
-
Dr Richard Neal, Clinical Senior Lecturer in General Practice, Cardiff University
-
Mr John Needham, Public contributor
-
Ms Mary Nettle, Mental Health User Consultant
-
Professor John Potter, Professor of Ageing and Stroke Medicine, University of East Anglia
-
Dr Greta Rait, Senior Clinical Lecturer and General Practitioner, University College London
-
Dr Paul Ramchandani, Senior Research Fellow/Cons. Child Psychiatrist, University of Oxford
-
Dr Karen Roberts, Nurse/Consultant, Dunston Hill Hospital, Tyne and Wear
-
Dr Karim Saad, Consultant in Old Age Psychiatry, Coventry and Warwickshire Partnership Trust
-
Dr Lesley Stockton, Lecturer, School of Health Sciences, University of Liverpool
-
Dr Simon Wright, GP Partner, Walkden Medical Centre, Manchester
-
Dr Kay Pattison, Senior NIHR Programme Manager, Department of Health
-
Dr Morven Roberts, Clinical Trials Manager, Health Services and Public Health Services Board, Medical Research Council
-
Professor Tom Walley, CBE, Director, NIHR HTA programme, Professor of Clinical Pharmacology, University of Liverpool
-
Dr Ursula Wells, Principal Research Officer, Policy Research Programme, Department of Health