Notes
Article history
The research reported in this issue of the journal was funded by the HTA programme as project number 11/36/09. The contractual start date was in October 2012. The draft report began editorial review in October 2013 and was accepted for publication in January 2015. The authors have been wholly responsible for all data collection, analysis and interpretation, and for writing up their work. The HTA editors and publisher have tried to ensure the accuracy of the authors’ report and would like to thank the reviewers for their constructive comments on the draft document. However, they do not accept liability for damages or losses arising from material published in this report.
Declared competing interests of authors
none
Permissions
Copyright statement
© Queen’s Printer and Controller of HMSO 2015. This work was produced by Pandor et al. under the terms of a commissioning contract issued by the Secretary of State for Health. This issue may be freely reproduced for the purposes of private research and study and extracts (or indeed, the full report) may be included in professional journals provided that suitable acknowledgement is made and the reproduction is not associated with any form of advertising. Applications for commercial reproduction should be addressed to: NIHR Journals Library, National Institute for Health Research, Evaluation, Trials and Studies Coordinating Centre, Alpha House, University of Southampton Science Park, Southampton SO16 7NS, UK.
Chapter 1 Background
Description of the health problem
Respiratory failure occurs when disease of the heart or lungs leads to failure to maintain adequate blood oxygen levels (hypoxia) or increased blood carbon dioxide levels (hypercapnia). By definition, hypoxaemic respiratory failure is characterised by an arterial oxygen tension (PaO2) of < 8 kPa (60 mmHg) with normal or low arterial carbon dioxide tension (PaCO2). 1 In contrast, hypercapnic respiratory failure is the presence of a PaCO2 > 6 kPa (45 mmHg) and PaO2 < 8 kPa. Respiratory failure can be acute (develops within minutes or hours in patients with no or minor evidence of pre-existing respiratory disease), acute on chronic (an acute deterioration in an individual with pre-existing respiratory failure) or chronic (develops over several days or longer in patients with existing respiratory disease). 1
Acute respiratory failure is a common but life-threatening medical emergency, especially in elderly patients (aged ≥ 65 years) with respiratory and cardiac diseases. 2–4 As patients with acute respiratory failure constitute a highly heterogeneous group, epidemiological data are sparse. Nevertheless, pneumonia, chronic obstructive pulmonary disease (COPD), acute lower respiratory infection and heart failure are the main causes of acute respiratory failure and were together responsible for 379,731 hospital admissions in England in 2009–10. Some 53,608 (14%) of these patients died within 30 days of admission,5 typically after developing acute respiratory failure. With an ageing population, coupled with improved survival following an episode of acute respiratory failure, the burden of acute respiratory failure on the NHS is likely to continue to increase.
The definitive treatment of acute respiratory failure depends on the underlying cause, but patients often require treatment in the ambulance while en route to hospital (pre-hospital treatment). At this point it is difficult to accurately determine the underlying cause, so pre-hospital treatment of acute respiratory failure often follows a common pathway rather than being specific to the underlying cause. Around 10% of medical admissions to hospital via emergency ambulance arrive at hospital with hypoxia (peripheral oxygen saturation below 92%) despite pre-hospital oxygen therapy [Goodacre S. Unpublished data from the DAVROS study (Development And Validation of Risk-adjusted Outcomes for Systems of Emergency Care) 2006–2011. 2013]. The risk of death in patients with respiratory problems increases markedly with distance travelled to hospital, from 10% at distances below 10 km to 20% at distances over 20 km. 6 This may be because many hospital treatments for acute hypoxaemic respiratory failure, particularly those involving respiratory support, are not routinely available in the pre-hospital setting.
Acute non-invasive ventilation (NIV) involves providing respiratory support through a tight-fitting mask, which is usually applied around the patient’s mouth and nose. It may take the form of continuous positive airway pressure (CPAP) or bilevel inspiratory positive airway pressure (BiPAP). Acute NIV is usually used in hospital but can be administered en route to hospital. CPAP is simpler to use and thus more suitable for pre-hospital care. Acute respiratory failure is often associated with elevated carbon dioxide levels and acidosis, in addition to hypoxia. In patients with chronic respiratory disease, oxygen therapy may reduce respiratory drive and worsen hypercapnia and thus outcome. BiPAP can improve gas exchange and outcome in these circumstances.
Current service provision
Pre-hospital care is provided by ambulance services in the UK in accordance with clinical practice guidelines from the Joint Royal Colleges Ambulance Liaison Committee (JRCALC). 7 Treatment pathways for the management of acute respiratory failure follow a standardised and structured approach to initial assessment often referred to as the ABCDE (airway, breathing, circulation, disability, exposure) approach. This allows the treating clinician to rapidly assess and treat any immediately life-threatening problems before progressing to a more detailed assessment of the underlying cause of respiratory failure. The JRCALC guidelines7 provide general guidance for the treatment of patients with dyspnoea and specific guidance for the treatment of asthma and COPD. General management options include patient positioning, assisted ventilation and supplemental oxygen. Specific management options for patients with suspected asthma or COPD include nebulised salbutamol and ipratropium bromide, intramuscular adrenaline and intravenous steroids.
On arrival at hospital a more detailed assessment can take place, involving a detailed clinical history and examination, followed by investigations such as chest radiography and arterial blood gas analysis. This allows the clinician to initiate treatments that are tailored to the underlying condition, while continuing with general management measures. In-hospital NIV is widely used in the NHS to treat acute respiratory failure that is refractory to initial medical therapy. 1,8–15 Treatment is delivered predominantly in the emergency department, acute medical/respiratory ward and critical care units. A common pathway of care, based on NIV application in the hospital setting, is summarised in Figure 1.
FIGURE 1.
Simplified in-hospital care pathway for management of patients with acute respiratory failure. ABCDE, airway, breathing, circulation, disability, exposure; ACPO, acute cardiogenic pulmonary oedema.
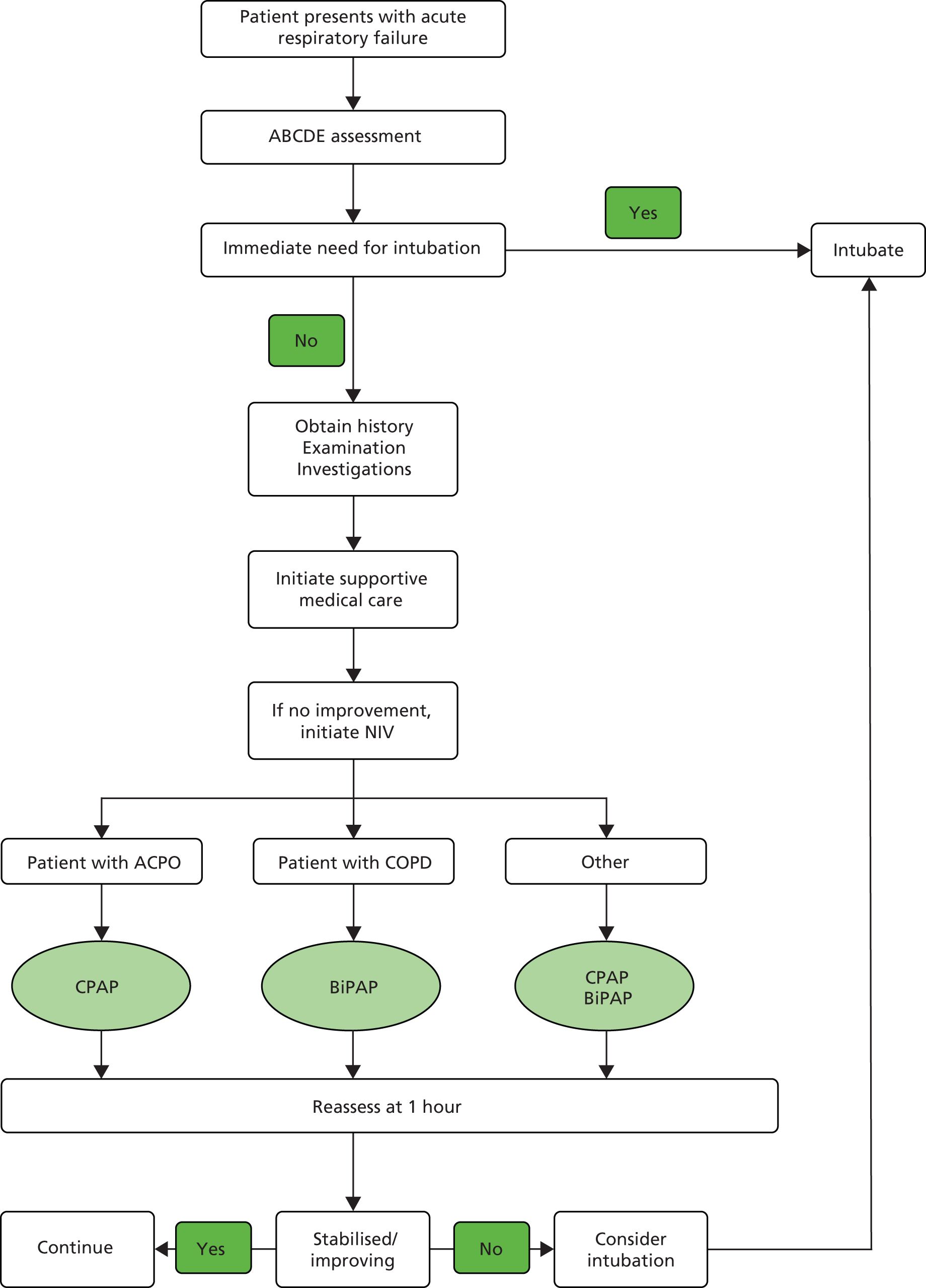
If NIV is contraindicated or fails to reverse acute respiratory failure, then intubation may be required. However, thresholds for intubation are not typically the same as those for providing NIV. Intubation requires sedation and neuromuscular paralysis followed by admission to the intensive care unit. This is likely to result in recovery with worthwhile quality of life only if the patient’s health and functional status were reasonable before the acute illness. Patients presenting with acute respiratory failure who have severe underlying disease and multiple comorbidities may benefit less from intubation and invasive ventilation. NIV, however, does not require sedation or neuromuscular paralysis and can be appropriately used in patients with relatively severe underlying disease.
The use of NIV is included in several national clinical practice guidelines for acute respiratory failure. For patients presenting to hospital with an acute exacerbation of COPD, the National Institute for Health and Care Excellence (NICE) guidelines16 recommend NIV as the treatment of choice for persistent hypercapnic ventilatory failure during an acute exacerbation. It is recommended that treatment is restricted to those not responding to standard medical therapy (controlled oxygen therapy, nebulisers and corticosteroids). The European Society of Cardiology recommends that NIV may be considered for use in dyspnoeic patients with pulmonary oedema and a respiratory rate > 20 breaths/minute to improve breathlessness and reduce hypercapnia and acidosis. 17 By contrast, the British Thoracic Society advises caution about the use of NIV in patients presenting with respiratory failure secondary to pneumonia or asthma. 1 If NIV is provided in these situations it should be done so in the setting of a critical care unit, where rapid access to invasive ventilation is immediately available in the event of treatment failure.
Although pre-hospital NIV is used in several European countries, it is not used routinely by UK NHS ambulance services or recommended in JRCALC guidance. However, the recent UK Ambulance Services Clinical Practice Guidelines 20137 recommended (for the first time) the use of CPAP in the pre-hospital environment on the basis of expert consensus.
Conceptually, the use of pre-hospital NIV is attractive as it would allow treatment to be initiated earlier. However, the pre-hospital setting differs from the in-hospital setting in a number of ways, which means that the results from in-hospital trials18–25 cannot be directly translated. Specifically, the initial assessment of patients is limited by the difficulty in conducting a full clinical examination and by the absence of diagnostic investigations, which creates less certainty about the underlying diagnosis. During pre-hospital treatment and transfer to hospital, the paramedic is isolated from the critical care support services that are immediately available in an acute hospital in the event of deterioration. The equipment available is likely to be limited by space constraints in the ambulance vehicle. These factors raise uncertainty about the effectiveness of pre-hospital NIV in the UK NHS.
The potential delivery of pre-hospital NIV across the UK is associated with considerable variation and uncertainty. This may partly be due to changing service configuration within the health-care setting as well as available relevant medical personnel, expertise and NIV equipment. In addition, as clinical management options can be adopted outside the UK Ambulance Clinical Practice Guidelines 20137 (depending on the priorities of an ambulance service clinical group), this may lead to variations in uptake, training, equipment and outcomes.
Description of the technology under assessment
Non-invasive ventilation is a form of positive-pressure respiratory support delivered to a spontaneously breathing patient who does not require the use of an invasive and artificial airway, for example an endotracheal tube or laryngeal mask. 1 NIV differs from invasive techniques because it does not bypass the upper respiratory tract. 1 The potential advantages of NIV over conventional management in selected patients include reduced breathlessness, improved arterial blood gases, and decreased intubation rates, mortality, morbidity and in-hospital length of stay. 20,23–25 Additionally, NIV is associated with less difficulty in weaning from invasive mechanical ventilation. 26 This is because NIV allows voluntary coughing, reduces the need for sedation and muscle relaxants and supports self-feeding and communication. 27,28 Commonly reported complications of NIV include aspiration pneumonia, gastric distension, vomiting, pressure lesions or sores, interface device leaks, intolerance to NIV and ventilator asynchrony. 29
Non-invasive ventilation modes or modalities have been described in different ways. 30 The ventilatory mode may be defined according to the method of gas flow administration. Commonly used NIV modes include CPAP and BiPAP ventilation.
The administration of CPAP involves the application of constant positive airway pressure during inspiration and expiration using a pressure compressor or a flow generator. 29,31 This mode is suitable for patients who are breathing spontaneously because it can only provide support to an underlying respiratory drive. 29,31,32 CPAP improves ventilation–perfusion matching and thereby improves oxygenation. 29 Other physiological effects of this mode of NIV include a reduction in venous return and a decrease in left ventricular wall stress; both effects result in an improvement in cardiac output. 29 This is particularly important in patients with acute cardiogenic pulmonary oedema (ACPO). There is no recommended initial pressure setting for CPAP; however, this may be determined by the patient’s age and the nature and severity of underlying disease. 29
Bilevel inspiratory positive airway pressure or non-invasive pressure-support ventilation involves the application of preset inspiratory and expiratory pressures which may be time-triggered by preset controls (controlled ventilation) or flow-triggered by the patient’s airway pressure (assisted ventilation). 29 The recommended initial inspiratory positive airway pressure (IPAP) is 10 cmH2O, and IPAP is increased in steps of 2–5 cmH2O or at a rate of approximately 5 cmH2O every 10 minutes. It is advised that an expiratory positive airway pressure (EPAP) of 4–5 cmH2O should be applied concurrently. 15 A pressure support level at 20 cmH2O is eventually maintained during BiPAP application.
For the available NIV modes, a variety of interface devices, for example helmets, full-face (facial) masks, nasal masks, oronasal masks and mouthpieces, can be used to provide a connection for transport of pressurised gas between the ventilator tubing and the patient’s upper airway. 31 Despite this broad variety, Schönhofer and Sortor-Leger33 found that the use of facial masks (≈ 70%) predominated, followed by nasal masks (≈ 25%) and nasal pillows (≈ 5%), in the administration of NIV in patients with acute respiratory failure. In the paediatric population, nasal pillows are commonly used. 29
Extensive research has evaluated the in-hospital role of NIV for various causes of acute respiratory failure. Meta-analysis of in-hospital trials for COPD21 has shown that NIV in conjunction with usual care, compared with usual care alone, is associated with reduced mortality [n = 7 studies; relative risk 0.41, 95% confidence interval (CI) 0.26 to 0.64] and need for intubation (n = 8 studies, relative risk 0.42, 95% CI 0.31 to 0.59). A systematic review of in-hospital trials of NIV for pneumonia found equivocal effects, especially in patients without COPD. 20 Several meta-analyses of NIV in ACPO have found reduced mortality and intubation rates. 19,22,23 The Three Interventions in Cardiogenic Pulmonary Oedema (3CPO) trial, which was published after the meta-analyses, found that NIV improved physiological parameters and symptoms of breathlessness in ACPO but did not reduce mortality or intubation rates. 18
It has been argued that NIV is more likely to be effective if used early in the course of respiratory failure, before fatigue develops. 34 This raises the possibility that pre-hospital NIV could be more effective than in-hospital NIV. Less research has been undertaken evaluating the pre-hospital use of NIV, but a number of recent reviews have indicated that pre-hospital NIV is feasible and beneficial in selected patients with acute respiratory failure. 35,36
A number of issues need to be considered in relation to the provision of pre-hospital NIV. The equipment needs to be suitable for pre-hospital use. Features of NIV devices for use in the pre-hospital setting include a compact size, portability, robust construction, ability to work with oxygen only rather than requiring compressed air, as well as compatibility with a range of available power sources. 32 Few, if any, of the existing BiPAP technologies meet these stringent requirements. As a result of these factors, and the available technologies, it is considered that, in pre-hospital care in the UK, it is currently more feasible to deliver CPAP than other forms of NIV.
Pre-hospital NIV is generally administered by paramedics or emergency medical teams, which may include a physician, nurse or respiratory technician or therapist. To our knowledge, pre-hospital use of NIV is currently limited in the UK to critical care paramedics in a few specific settings, such as the South East Coast Ambulance Service. However, interest in providing NIV is growing. In the USA, the National Association of Emergency Medical Service Physicians stated that NIV is an important treatment modality for the pre-hospital management of acute dyspnoea. 37 In the UK, it was identified among research priorities by a recent 999 emergency medical services research forum. 38
With around 16,000 paramedics and 5500 ambulance vehicles in England, widespread adoption of NIV into paramedic practice would require substantial resources of training and equipment. Widespread provision of pre-hospital NIV will require substantial resources, training and reorganisation. It is currently not clear if existing evidence justifies widespread use of pre-hospital NIV. It is also not clear what further evidence would be required to reduce uncertainty and help decision-making.
Pre-hospital treatment of acute respiratory failure also has substantial knock-on costs for the health service. Patients with life-threatening respiratory illness often require prolonged hospital stay and/or critical care involvement owing to the requirement for ventilatory support. Inadequate or inappropriate initial management can result in the need for respiratory support and critical care admission. Conversely, the appropriate use of early intervention can reduce the need for intubation and ventilation, thus reducing critical care costs.
Chapter 2 Research questions
Rationale for the study
Pre-hospital NIV has the potential to reduce mortality from acute respiratory failure, but widespread provision of pre-hospital NIV will require substantial resources, training and reorganisation. It is currently not clear if existing evidence justifies widespread use of pre-hospital NIV. In-hospital studies of NIV suggest benefit in some conditions and uncertainty in others. Arguments can be made for pre-hospital NIV being either more effective or less effective than in-hospital NIV, so findings from in-hospital studies cannot be automatically extrapolated to the pre-hospital setting. A number of trials of pre-hospital NIV have been undertaken but they have not been subject to comprehensive systematic reviews and their findings have not been synthesised using the best current methods. A systematic review and meta-analysis is therefore required to determine whether or not the currently available evidence supports pre-hospital use of NIV.
Even if there was reliable evidence of effectiveness, this would not necessarily justify widespread implementation of pre-hospital NIV. The costs of such implementation could be substantial and could represent poor value for health-care resources if pre-hospital NIV were applied to a small number of patients only or associated with a small health benefit. An economic analysis is therefore required to determine the cost-effectiveness of pre-hospital NIV compared with standard usual care for acute respiratory failure. Issues of practicality, available equipment and training mean that pre-hospital CPAP is the most likely form of pre-hospital NIV to be widely implemented in the NHS, so economic analysis needs to focus on pre-hospital CPAP rather than BiPAP.
Finally, it is not clear what further evidence would be required to reduce uncertainty and help decision-making. Undertaking a large randomised trial of pre-hospital NIV would reduce uncertainty, but it is not clear whether or not the current evidence base justifies such a substantial undertaking. Expected value of information analysis is therefore required to determine whether or not further research into pre-hospital NIV would represent a cost-effective use of health-care resources and identify where future research would be best focused.
Overall aims and objectives of assessment
The overall aim was to determine the clinical effectiveness and cost-effectiveness of pre-hospital NIV for acute respiratory failure and identify priorities for future research. More specifically the objectives were:
-
to undertake a systematic review [including individual patient-level data (IPD) meta-analysis, if appropriate data were available] to determine the effectiveness of pre-hospital NIV in patients with acute respiratory failure
-
to develop an economic model to (a) estimate the incremental cost per quality-adjusted life-year (QALY) gained by providing pre-hospital NIV (specifically pre-hospital CPAP) instead of standard care; (b) estimate the additional costs incurred by establishing and providing pre-hospital CPAP, and the lives saved and QALYs gained across the population served by a typical ambulance service; and (c) estimate the expected value of information associated with reducing uncertainty around key parameters.
Chapter 3 Assessment of clinical effectiveness
We carried out a systematic review of the literature and a network meta-analysis (NMA) to evaluate the clinical effectiveness of pre-hospital NIV in patients with acute respiratory failure.
The review of the evidence was carried out in accordance with the general principles recommended in the Preferred Reporting Items for Systematic Reviews and Meta-Analyses statement. 39
Methods for reviewing effectiveness
Identification of studies
Electronic databases
Studies were identified by searching the following electronic databases and research registers:
-
MEDLINE In-Process & Other Non-Indexed Citations and MEDLINE (via OvidSP) from 1948 to August 2013
-
EMBASE (via OvidSP) from 1980 to August 2013
-
Cumulative Index to Nursing and Allied Health Literature (via EBSCOhost) from 1982 to August 2013
-
Cochrane Database of Systematic Reviews (via Wiley Online) from 1996 to August 2013
-
Cochrane Central Register of Controlled Trials (via Wiley Online) from 1898 to August 2013
-
Health Technology Assessment Database (via Wiley Online) from 1995 to August 2013
-
Database of Abstracts of Review of Effects (via Wiley Online) from 1995 to August 2013
-
Bioscience Information Service (BIOSIS) Previews (via ISI Web of Knowledge) from 1969 to August 2013
-
Science Citation Index Expanded (via Web of Science) from 1899 to August 2013
-
Conference Proceedings Citation Index – Science (via Web of Science) from 1990 to August 2013
-
UK Clinical Research Network Portfolio Database [National Institute for Health Research (NIHR)] from 2001 to October 2012
-
National Research Register Archive (NIHR) from 2000 to September 2007
-
Current Controlled Trials from 2000 to October 2012
-
ClinicalTrials.gov (USA National Institutes of Health) from 2000 to October 2012.
Sensitive keyword strategies were developed using free text and, where available, thesaurus terms using Boolean operators and database-specific syntax to search the electronic databases. Synonyms relating to the setting (e.g. pre-hospital) were combined with terms for NIV. No language or date restrictions were used on any database. All resources were initially searched from inception to October 2012. With the exception of the four research registers, updated searches to August 2013 were conducted on the remaining electronic databases. An example of the MEDLINE search strategy is provided in Appendix 1.
Other resources
To identify additional published, unpublished and ongoing studies, the reference lists of all relevant studies were checked and a citation search of relevant articles (using the Web of Science, Science Citation Index Expanded and Conference Proceedings Citation Index – Science) was undertaken to identify articles that cite the relevant articles. In addition, systematic keyword searches of the internet were undertaken using the Google search engine (Google Inc., Mountain View, CA, USA) and key experts in the field were contacted.
All identified citations from the electronic searches and other resources were imported into and managed using the Reference Manager bibliographic software version 12.0 (Thomson Reuters, Philadelphia, PA, USA).
Inclusion and exclusion criteria
The inclusion of potentially relevant articles was undertaken using a two-step process. First, all titles were examined for inclusion by one reviewer. Any citations that clearly did not meet the inclusion criteria (e.g. non-human, unrelated to acute respiratory failure) were excluded. Second, all abstracts and full-text articles were examined independently by two reviewers. Any disagreements in the selection process were resolved through discussion. The relevance of each article for the systematic review was assessed in accordance with the criteria below.
Study design
All randomised (individual or cluster) or quasi-randomised controlled trials that evaluated pre-hospital NIV (as part of acute treatment by the emergency care system) in patients with acute respiratory failure were included. Non-randomised observational studies were not included in the formal systematic review but were retained and reported descriptively as additional evidence and, if appropriate, used to develop the economic model. In addition, all trials in progress (identified via trial registers) were recorded but not included in the analysis.
The following publication types were excluded from the review: animal models; pre-clinical and biological studies; narrative reviews, editorials, opinions; non-English-language papers; and reports published as meeting abstracts only, where insufficient methodological details are reported to allow critical appraisal of study quality.
Population
All studies of adults (defined as > 18 years of age) presenting to the emergency services with acute (hypoxaemic or hypercapnic) respiratory failure from any cause or no specified cause were included.
Interventions
Pre-hospital NIV (defined as ventilatory support provided before arrival at hospital and delivered to a spontaneously breathing individual without airway intervention) requiring BiPAP or CPAP interventions were included. Head-to-head studies that compared different applications of NIV (e.g. CPAP vs. BiPAP) or different interfaces (e.g. NIV with a face mask vs. NIV with a helmet) were excluded.
Relevant comparators
The relevant comparator was considered to be usual care. This consisted of any alternative treatment to pre-hospital NIV, including standard oxygen therapy, standard medical therapy, delayed NIV or in-hospital NIV.
Outcomes
The outcomes of the review included the need for intubation, mortality (within 30 days), measures of breathlessness or respiratory function and patient-relevant outcomes.
Data abstraction strategy
Data abstraction was performed by one reviewer into a standardised data extraction form and independently checked for accuracy by a second reviewer. Discrepancies were resolved by discussion between the two reviewers and, if agreement could not be reached, a third reviewer was consulted. Where multiple publications of the same study were identified, data were extracted and reported as a single study.
The following information was extracted for all studies when reported: study characteristics (e.g. author, year of publication, country, study design, setting, duration of follow-up, funding), participant details (e.g. age, sex, diagnosis, comorbidities, baseline physiology), intervention [e.g. system used, pressure(s) used, duration of treatment, practitioners providing intervention] and comparator (e.g. any use of NIV, supplemental oxygen) details, including information on any specified co-treatments, and outcomes (including definitions). Where applicable, the authors of all included randomised trials were contacted to clarify details, obtain missing data and request IPD for meta-analysis.
Quality assessment strategy
The methodological quality of each included study was assessed by one reviewer and independently checked by another. Disagreements were resolved by discussion between the two reviewers and if agreement could not be reached, a third reviewer was consulted. The study quality characteristics were assessed according to (adapted) criteria based on those proposed by Verhagen et al. 40 for randomised controlled trials (RCTs). Further details are provided in Appendix 2.
Methods of data synthesis
The extracted data and quality assessment variables were presented for each study, both in structured tables and as a narrative description. For each outcome of interest (mortality and the need for intubation), a NMA was performed in two separate analyses using (1) aggregate data from all studies and (2) IPD from authors who provided relevant data and aggregate data for studies where IPD were not available. A NMA allows a comprehensive comparison of all interventions that are linked with respect to at least one common intervention without breaking the randomisation within studies. The summary statistics that were analysed were the numbers of patients who had an event. Potential treatment effect modifiers (age, sex, provider, primary diagnosis, severity of acute respiratory failure and pre-hospital time delay) were explored using a NMA combining both IPD and aggregate data. Where possible, univariate regression analyses of the IPD from individual studies were performed to identify potential treatment effect modifiers and the plausibility of conducting a full NMA. A one-stage NMA of the most likely treatment effect modifiers was then performed separately for each covariate. Any missing covariates in the IPD were assumed to be missing completely at random and were imputed using multiple imputation by giving them a prior distribution.
All models were analysed using Markov chain Monte Carlo techniques using a random-effects model (to allow for heterogeneity in treatment effects across studies) implemented using the WinBUGS Version 1.4.3 (MRC Biostatistics Unit, Cambridge, UK)41,42 and OpenBUGS43 Version 3.2.3 (www.openbugs.net/w/FrontPage) software package. Further details of the aggregate and combined IPD and aggregate data models are presented in Appendices 3 and 4.
Convergence of the model to its posterior distribution was assessed using the Gelman–Rubin convergence statistic (as modified by Brooks and Gelman). 44 In each aggregate data NMA, convergence occurred within 10,000 iterations, so the final analysis used a burn-in of 10,000. In each combined IPD and aggregate data NMA, convergence occurred within 50,000 iterations so the final analysis used a burn-in of 50,000. There was some suggestion of moderate autocorrelation between successive iterations of the Markov chains; to compensate for this, the Markov chains were thinned every five iterations. Parameter estimates were estimated based on 10,000 iterations of the Markov chains. The total residual deviance was used to formally assess whether or not the statistical model provided a reasonable representation of the sample data. The total residual deviance is the mean of the deviance under the current model minus the deviance for the saturated model, so that each data point should contribute about 1 to the deviance.
When competing models were used in the analysis, then the deviance information criterion (DIC)45 was used to assess the goodness of fit. The DIC compares models based on a trade-off between the fit of the data and the complexity of the fitted model, where the complexity of the model is measured by estimating the effective number of model parameters. Lower DIC values indicate a better model choice.
Results of the NMA were reported in terms of odds ratios (ORs) and 95% credible intervals (CrIs) relative to the baseline intervention (i.e. usual care). The 95% CrIs represent the 95% probability that the true underlying effect lies in the interval specified. The posterior median of the between-study standard deviation together with the 95% CrIs was also presented. To account for potential heterogeneity in intervention effects between studies, the posterior predictive distribution for the OR from a hypothetical new study was also presented.
Results
Quantity and quality of research available
Number of studies identified/included
The literature searches identified 2284 citations. Of these, eight RCTs46–53 and two quasi-randomised trials54,55 met the inclusion criteria. A flow chart describing the process of identifying relevant literature can be found in Figure 2.
Number and type of studies excluded
A total of 55 full-text articles were excluded, as they did not meet all the prespecified inclusion criteria. The majority of the articles were excluded primarily on the basis of inappropriate study design (non-RCT), inappropriate setting (in-hospital NIV) or unsuitable publication type (reviews, commentaries or editorials). One of the excluded studies (the VeNIS BPCO trial),56 which was identified on a trials register, was a planned open-label RCT that was designed to evaluate the effectiveness of pre-hospital NIV compared with standard medical treatment in reducing intubation rates during acute respiratory failure in people with COPD. With an estimated enrolment of 398 adult patients from France, the study was due for completion (final data collection) in December 2014. However, at the time of writing, the study was not yet open for participant recruitment. A full list of excluded studies with reasons for exclusion is presented in Appendix 5.
Assessment of effectiveness
Description of included studies (design and patient characteristics)
The design and patient characteristics of the 10 included studies46–55 that evaluated the effectiveness of pre-hospital NIV for adults with acute respiratory failure are summarised in Tables 1–3.
Author, year, country | Design | Intervention | Comparator | Primary outcomes | Prespecified intubation criteria | Duration of follow-up | Funding |
---|---|---|---|---|---|---|---|
Studies evaluating CPAP | |||||||
Austin and Wills 2012;46 Australia (abstract) | RCT (n = 50) | CPAP (no details provided) (n = 24) | CMT (including oxygen) with bag–valve–mask ventilation (n = 26) | Mortality (pre-hospital or in-hospital) | NR | NR | NR |
Provider: NR | In-hospital NIV use: NR | ||||||
Ducros et al. 2011;47 France | RCT (n = 207) | CPAP; 7.5–10 cmH2O, FiO2 0.3–1.0 by face mask (n = 107) | CMT including oxygen at 15 l/minute (n = 100) | Composite end point of death, need for intubation, persistence of all ACPO symptoms or circulatory failure at 2 hours or reappearance after 2 hours | Refractory hypoxaemia (SpO2 < 85%) after 30 minutes of supplementary oxygen at 15 l/minute (control group) or maximal FiO2 60% (CPAP group), respiratory arrest or pauses with loss of consciousness, agitation, increased dyspnoea and haemodynamic instability | Until time of hospital discharge or death (duration NR) | French Ministry of Health, France |
Provider: physician | In-hospital NIV use: prohibited | ||||||
Frontin et al. 2011;48 France | RCT (n = 122)a | CPAP; 10 cmH2O by face mask for 1 hour (n = 60) | CMT including oxygen at 15 l/minute by face mask (n = 62) | Treatment successb | Worsening SpO2 or clinical condition despite effective treatment, loss of airway protective reflexes, deteriorating consciousness, haemodynamic instability, intolerance/poor fit of face mask (CPAP group only) | 30 days | University Hospital of Toulouse, France |
Provider: physician | In-hospital NIV use: allowed | ||||||
Plaisance et al. 2007;50 France | RCT (n = 124) | CPAP (early CPAP) was applied for the first 30 minutes at 7.5 cmH2O, FiO2 0.33–0.37 by face mask. Subsequent administration of CMT and oxygen therapy only for the remaining 15 minutes of the study period (n = 63) | CPAP (late CPAP) included CMT with oxygen administered for the initial 15 minutes, followed by CPAP (7.5 cmH2O) for another 15 minutes. CPAP was then discontinued. Only CMT was maintained for the remaining 15 minutes of the study period (n = 61) | Effect of early CPAP on dyspnoea scorec and arterial blood gases | Refractory hypoxaemia (SpO2 < 85%), respiratory arrest or cessations, loss of consciousness, agitation requiring sedation, heart rate < 50 beats/minute and haemodynamic instability | Until time of hospital discharge or death (duration NR) | Hôpital Lariboisière, France |
Provider: physician | In-hospital NIV use: mandated | ||||||
Schmidbauer et al. 2011;52 Germany | RCT (n = 36) | CPAP; 5–30 cmH2O, FiO2 0.5–1.0 by face mask (n = 18) | SOT delivered by face mask (flow rate NR) (n = 18) | Intubation rate | NR (intubation performed according to the physicians discretion) | Until time of hospital discharge or death (duration NR) | Medical Service of the German Armed Forces, Germany |
Provider: physician | In-hospital NIV use: NR | ||||||
Thompson et al. 2008;53 Canada | RCT (n = 71)a | CPAP; 10 cmH2O by face mask (n = 36) | CMT with oxygen by face mask (i.e. SOT), bag–valve–mask ventilation or tracheal intubation (n = 35) | Intubation rate | Worsening SpO2 despite effective CPAP, loss of airway protective reflexes, impaired consciousness, evidence of cardiac ischaemia, haemodynamic instability or intolerance/poor fit of face mask | Until time of hospital discharge or death (duration NR) | Dalhousie University and Capital District Health Authority, Canada |
Provider: paramedic | In-hospital NIV use: allowed (n = 4) | ||||||
Studies evaluating BiPAP | |||||||
Mas et al. 2002;49 Spain (abstract) | RCT (n = 56) | BiPAP; EPAP 7 cmH2O, IPAP 19 cmH2O (n = 28) | Standard therapy, not specified (n = 28) | Intubation rate | NR | Until time of hospital discharge or death (duration NR) | The Fund for Health in Spain |
Provider: paramedic and physician | In-hospital NIV use: NR | ||||||
Roessler et al. 2012;51 Germany | RCT (n = 49)d | BiPAP;e 5–20 cmH2O, PEEP 5–15 cmH2O, FiO2 1.0 by face mask (n = 24) | CMT including supplementary oxygen (n = 25) | Treatment successf | Respiratory arrest or cessations, impaired consciousness, heart rate < 50 beats/minute, or haemodynamic instability | 30 days | University of Göttingen, Germany |
Provider: physician | In-hospital NIV use: allowed (n = 4) | ||||||
Craven et al. 2000;54 USA | Quasi-RCT (n = 62)g | BiPAP; by face mask (pressure level NR) (n = 37) | CMT with oxygen (no further details provided) (n = 25) | NR; however, outcomes included the need for intubation, mortality, out-of-hospital treatment time, changes in oxygen saturation and length of hospital stay | NR | NR | NR |
Provider: paramedich | In-hospital NIV use: NR | ||||||
Weitz et al. 2007;55 Germany | Quasi-RCT (n = 23) | BiPAP; 12 cmH2O, PEEP 5 cmH2O, FiO2 0.6 by face mask (n = 10) | CMT for acute heart failure with oxygen at 8 l/minute by face mask (n = 13) | Oxygen saturation | NR | Until time of hospital discharge or death (duration NR) | Dräger Medical, Lubeck, Germany |
Provider: physiciani | In-hospital NIV use: NR |
Variable | Author, year | |||||
---|---|---|---|---|---|---|
Austin and Wills, 201246 (abstract) | Ducros et al., 201147 | Frontin et al., 201148 | Plaisance et al., 200750 | Schmidbauer et al., 201152 | Thompson et al., 200853 | |
Population | Adults with presumed ACPO experiencing severe respiratory distress with insufficient respiratory effort | Adults with presumed ACPO [orthopnoea, diffuse crackles (Killip score of > III), RR > 25 breaths/minute, SpO2 < 90%] | Adults with presumed ACPO (orthopnoea, diffuse crackles without signs of pulmonary aspiration or infection, RR > 25 breaths/minute, SpO2 < 90%) | Adults with presumed ACPO (orthopnoea, diffuse crackles without signs of pulmonary aspiration or infection, SpO2 ≤ 90%) | Adults presenting with acute exacerbated COPD (acute dyspnoea, RR > 25 breaths/minute, SpO2 < 90%) | Adults presenting with severe respiratory distress (failing respiratory effort, accessory muscle use, RR > 25 breaths/minute, hypoxia) |
Age, mean (years) | 80 | 80 | 79 | 77 | NR | 68 |
Males (%) | 56 | 41 | 43 | 49 | NR | 51 |
Diagnosis (primary) | NR | ACPO, n = 207 | ACPO, n = 122 | ACPO, n = 124 | COPD exacerbation, n = 36 | Asthma, n = 10; CHF, n = 39; COPD, n = 18; pneumonia, n = 1; mixed diagnosis, n = 1; NR, n = 2 |
Co-treatments | Furosemide, nitrates | Furosemide, nitroglycerin, bumetanide, dobutamine | Furosemide, isosorbide dinitrate | Dobutamine | NR (additional therapy received in accordance with standard local operating procedures) | Furosemide, nitroglycerin, morphine, salbutamol, ipratropium bromide |
Baseline physiology (mean ± SD) | ||||||
pH | NR | 7.35 ± 0.08 | NR | 7.32 ± 0.09 | NR | NR |
RR (breaths/minute) | NR | 28.78 ± 7.68 | 35.16 ± 7.67 | 34.15 ± 7.25 | NR | 37.88 ± 7.07 |
HR (beats/minute) | NR | 91.38 ± 21.89 | 108.86 ± 24.78 | 104.51 ± 22.09 | NR | NR |
Systolic BP (mmHg) | NR | 152.18 ± 30.33 | 167.09 ± 37.63 | 175.07 ± 38.68 | NR | NR |
SpO2 (%) | NR | 96.64 ± 4.19 | 77.84 ± 11.36 | 85.99 ± 2.98 | NR | 75.52 ± 14.09 |
PaO2 (mmHg) | NR | 142.91 ± 92.08 | 98.62 ± 50.84 | 49.70 ± 5.86 | NR | NR |
PaCO2 (mmHg) | NR | 44.17 ± 11.45 | 48.63 ± 14.51 | 49.70 ± 9.12 | NR | NR |
Variable | Author, year | |||
---|---|---|---|---|
Craven et al., 200054a | Mas et al., 200249 | Roessler et al., 201251 | Weitz et al., 200755 | |
Population | Adults experiencing CHF with presumed ACPO (dyspnoea with increased RR, HR, sweating, peripheral oedema) | Adults presenting with ARF (RR > 28 breaths/minute, SpO2 < 92% or SpO2 < 90% at any RR) | Adults presenting with ARF owing to presumed COPD or pneumonia with signs of hypoxaemia (SpO2 < 90%) or ventilator failure (SpO2 < 90% with RR > 20 breaths/minute at rest) | Adults with presumed ACPO (severe dyspnoea; SpO2 < 90%) |
Age, mean (years) | NR (median, 75) | 78 | 74 | 77 |
Males (%) | 45 | NR | 53 | 52 |
Diagnosis (primary) | NR | Acute pulmonary oedema, n = 28; COPD exacerbation, n = 17; mixed diagnosis, n = 5; other, n = 6 | ACPO, n = 25; asthma, n = 1; COPD exacerbation, n = 17; pneumonia, n = 6 | Pulmonary oedema, n = 20; mixed diagnosis, n = 3 |
Co-treatments | Diuretics, nitrates, other (not specified) | NR | Furosemide, urapidil, reproterol, dexamethasone, opioids | Furosemide, nitroglycerin, morphine |
Baseline physiology (mean ± SD) | ||||
pH | NR | NR | 7.29 ± 0.11 | 7.31 ± 0.14 |
RR (breaths/minute) | NR | 36.25 ± 7.31 | 30.63 ± 6.47 | 29.47 ± 8.07 |
HR (beats/minute) | NR | 108.70 ± 25.96 | 116.04 ± 31.22 | 110.70 ± 22.75 |
Systolic BP (mmHg) | NR | 133.68 ± 21.26 | 164.27 ± 40.41 | 173.48 ± 36.13 |
SpO2 (%) | NR | 78.71 ± 10.05 | 77.24 ± 14.82 | 82.52 ± 6.44 |
PaO2 (mmHg) | NR | NR | 216.44 ± 73.53 | 72.91 ± 18.51 |
PaCO2 (mmHg) | NR | NR | 52.71 ± 16.83 | 49.03 ± 16.11 |
All studies were published between 2000 and 2012. Studies were undertaken in a variety of countries and settings including Australia,46 Europe (France,47,48,50 Germany51,52,55 and Spain49) and North America (Canada53 and the USA54). The duration of follow-up was not reported in two studies;46,54 however, the length of follow-up in the remaining studies ranged from 30 days48,51 to hospital discharge or death. 47,49,50,52,53,55 Of the 10 studies, only one study55 recieved funding from one or more commercial sponsors. The design of the included studies required the continuation of management of respiratory failure in the hospital setting and in-hospital management was generally at the discretion of the treating physicians.
As all studies included patients with acute respiratory failure without immediate need for intubation (i.e. spontaneously breathing patients), there was wide variation in terms of underlying conditions resulting in respiratory failure. Six studies46–48,50,54,55 included a selected population of patients with ACPO. Of these, two studies47,50 documented the exclusion of patients with COPD. Conversely, one study52 included patients with acute respiratory failure due to COPD. One study53 enrolled a diverse population, including patients with chronic heart failure, COPD, asthma, pneumonia and acute coronary syndrome with respiratory failure. The sample sizes of the included studies ranged from 23 patients55 to 207 patients,47 with the mean age of participants ranging from 68 years53 to 80 years. 46,47 The percentage of male participants ranged from 41%47 to 56%. 46
Continuous positive airway pressure was the most commonly used mode of NIV in the intervention arm of the included studies. While one study, that of Plaisance et al. ,50 compared the effectiveness of early CPAP (where patients had CPAP for the first 15 minutes after study inclusion, followed by CPAP with medical treatment for 15 minutes) with late CPAP (where patients received conventional medical treatment with supplementary oxygen for the first 15 minutes of study inclusion, followed by the addition of CPAP for another 15 minutes), five studies46–48,52,53 provided CPAP to patients in the intervention group while patients in the control group received conventional medical treatment only. Of the four studies49,51,54,55 that assessed the use of BiPAP versus standard usual care, two were quasi-randomised trials. 54,55 In the study by Roessler et al. ,51 NIV was initially started with CPAP; however, this was quickly changed to BiPAP, if CPAP was tolerated (22 of 24 patients in the intervention group).
The NIV intervention in the included studies, where reported, was provided either by paramedics49,53,54 or by an emergency physician. 47,48,50–52,55 However, in the study by Thompson et al. 53 the response to out-of-hospital emergency calls was the responsibility of an advanced life support team of paramedics, with ongoing online support provided by a physician, remotely. One study46 had no information relating to medical personnel administering pre-hospital NIV.
Although two studies46,49 did not provide details relating to the NIV interface or pressure support levels, eight studies47,48,50–55 used a face mask as the interface of choice for the administration of NIV. In RCTs evaluating CPAP, pressure levels were fixed at 7.5 cmH2O50 or 10 cmH2O. 48,53 Applied pressure levels used in other studies47,52 were determined by a titration method based on patient’s response to treatment and degree of comfort. In these studies, the pressure support levels ranged from 5 cmH2O to 30 cmH2052 and from 7.5 cmH2O to 10 cmH2O. 47 One study provided no details on pressure levels. 46 In studies evaluating BiPAP, a pressure of 12 cmH2O was applied in the study by Weitz et al. 55 [positive end-expiratory pressure (PEEP), 5 cmH2O; fraction of inspired oxygen (FiO2), 0.6], with pressure support titration to achieve a tidal volume of 7 ml/kg body weight or more in treated patients. Similarly, Roessler et al. 51 adjusted pressure support levels from 5 cmH2O to 20 cmH2O, depending on comfort (PEEP, 5–15 cmH2O). In contrast, BiPAP with a fixed airway pressure support (EPAP 7 cmH2O; IPAP 19 cmH2O) was administered in a study by Mas et al. 49 No information was available relating to pressure support levels in the study by Craven et al. 54 However, this was the only study that reported that NIV was applied following transfer of the patient into an ambulance equipped with the ventilation system.
Descriptions of the treatment schemes in the control groups were varied and included terminology such as ‘usual treatment’,54 ‘standard therapy’,49 ‘usual care’,46–48,53 ‘standard oxygen therapy’,52 ‘standard medical treatment’51,55 or delayed NIV. 50 As all control groups received conventional medical treatment together with supplementary oxygen for the management of acute respiratory failure or its underlying cause, treatment in the control groups is considered as usual care (standard oxygen therapy) throughout this report. The use of NIV in the control group varied between studies. In the study by Plaisance et al. ,50 which compared early CPAP with late CPAP, NIV use in the control group was regarded as mandatory. Two studies, those of Roessler et al. 51 and Thompson et al. ,53 reported that patients in the control groups were managed with NIV following admission (n = 4/25 and n = 4/35, respectively). NIV use in the control group of these studies was, therefore, regarded as allowed. Although no patients used NIV, Frontin et al. 48 reported that their study allowed the use of NIV in the control group. On the other hand, patients in the study conducted by Ducros et al. 47 presenting with intubation criteria could not receive any type of NIV support. These patients were intubated in the first instance. In this study NIV use in the control group was considered to be prohibited. For the remaining studies,46,49,52,54,55 the use of NIV in the control group was unclear.
Reporting of dosing regimens and number of patients in study groups receiving co-treatments varied across studies. However, the commonest interventions were diuretics46–48,51,53–55 and nitrates. 46,47,53–55
Quality characteristics
The overall methodological quality of the 10 included studies is summarised in Figure 3 and Table 4. Generally, six studies performed well,47–51,53 receiving a positive assessment on at least six out of nine methodological quality items.
FIGURE 3.
Methodological quality graph: review authors’ judgements about each methodological quality item as percentages across all included studies.

Author, year | Methodological assessment criteria | ||||||||
---|---|---|---|---|---|---|---|---|---|
1 | 2 | 3 | 4 | 5 | 6 | 7 | 8 | 9 | |
Austin and Wills, 201246 (abstract) | U | U | U | U | U | U | N | U | U |
Craven et al., 200054 | N | N | N | Y | N | Y | Y | N | U |
aDucros et al., 201147 | Y | Y | U | Y | Y | Y | Y | Y | N |
aFrontin 2011 et al., 201148 | Y | Y | Y | Y | Y | Y | Y | Y | Y |
aMas et al., 200249a (abstract) | Y | Y | N | Y | Y | Y | Y | Y | N |
aPlaisance et al., 200750 | Y | Y | N | Y | Y | Y | Y | Y | Y |
aRoessler et al., 201251 | Y | Y | N | Y | Y | Y | Y | Y | N |
Schmidbauer et al., 201152 | U | Y | U | Y | Y | Y | Y | U | U |
aThompson et al., 200853 | Y | Y | Y | Y | Y | Y | Y | Y | Y |
Weitz et al., 200755 | N | N | N | Y | N | Y | Y | Y | N |
Of the eight RCTs,46–53 only six studies reported the method of randomisation. 47–51,53 In seven studies,47–53 similar methods for concealing treatment allocation were used: a randomly generated sequence of treatment allocation concealed in sealed envelopes. Two studies were considered to be quasi-randomised trials. 54,55 In Weitz et al. 55 the study design was described by the authors as a prospective, randomised trial. However, the method of randomisation in this study was based on date of birth. This method of assignment (systematic allocation) is not considered as strictly random. 57 In Craven et al. 54 the study was described by the authors as a prospective, sequential, parallel controlled trial. However, no details were provided on the method of randomisation. Moreover, in this study, 10 emergency service units were divided into five matched pairs (based on similar patient demographics). Five units (one of each matched pair) were then equipped with a BiPAP ventilation system and five without. A convenience sample of adults presumed to have chronic heart failure was given BiPAP by the emergency team during transport and was compared with a control group that received usual care.
The potential sources of bias most frequently identified in studies concerned lack of blinding of outcome assessment and lack of adequate power to detect differences in the primary outcome. Lack of blinding may influence intubation rate (although in a pragmatic trial this may be acceptable) but is unlikely to influence mortality. Many of the studies had small sample sizes (< 100 patients)46,49,51–55 so it is likely they had inadequate statistical power to detect between-group differences, even if they were present. All of the included studies were conducted outside the UK, making generalisability of the findings to the UK setting uncertain.
Effects of interventions
Network meta-analysis using aggregate data
A NMA was undertaken to compare the effectiveness of pre-hospital NIV for adults with acute respiratory failure in terms of mortality and intubation. 58 Figure 4 presents the network of evidence. A total of 10 studies46–55 comparing BiPAP or CPAP against standard care provided information on at least one of the outcomes being analysed, although two studies did not provided information on intubation. 46,55 A summary of all the trials (data) included in the base-case NMA is presented in Appendix 6.
FIGURE 4.
Network diagram of different pre-hospital NIV interventions vs. standard oxygen therapy (usual care) for acute respiratory failure.
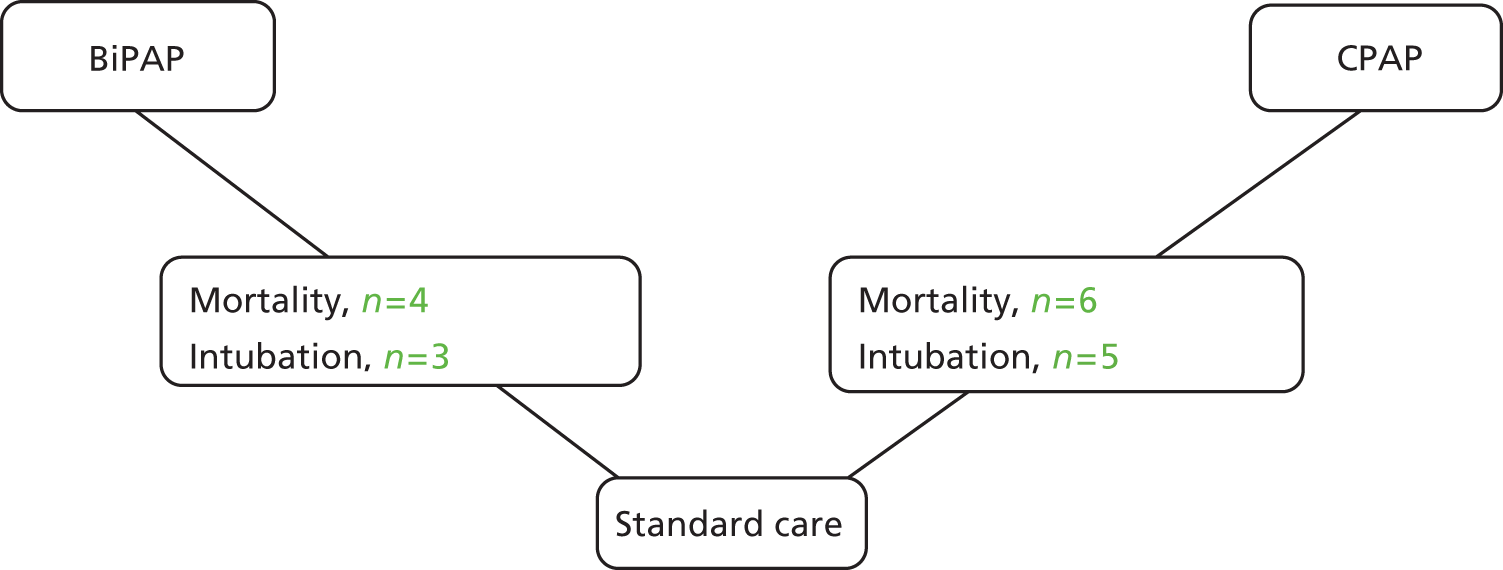
Craven et al. 54 performed a cluster randomised trial. However, we had no information on the intracluster correlation coefficient and we did not adjust the effective sample size of this study. Consequently, this study may contribute more information to the analysis than is appropriate. A sensitivity analysis was performed by excluding data from Plaisance et al. 50 because the control group received delayed pre-hospital CPAP rather than in-hospital CPAP, and by excluding data from Craven et al. 54 and Weitz et al. 55 because neither was genuinely randomised.
Data were available from 10 studies,46–55 including six comparing CPAP46–48,50,52,53 with usual care and four49,51,54,55 comparing BiPAP with usual care. However, there were no deaths in the Schmidbauer et al. 52 study, so this study provides no information about treatment effect. A summary of the results from the NMA is presented in Table 5.
Treatment | Random-effects mean | Predictive distribution | Probability most effective | ||
---|---|---|---|---|---|
OR | 95% CrI | OR | 95% CrI | ||
NIV | |||||
BiPAP | 1.94 | 0.65 to 6.14 | 1.93 | 0.50 to 7.98 | 0.008 |
CPAP | 0.41 | 0.20 to 0.77 | 0.41 | 0.14 to 1.16 | 0.989 |
Usual carea | |||||
Reference | Reference | Reference | Reference | Reference | 0.004 |
Between-study standard deviation | 0.29 | 0.02 to 0.85 | – | – | – |
The NMA model fitted the data reasonably well, with a residual deviance close to the total number of (non-zero) data points included in the analysis. The total residual deviance was 18.82, which compared favourably with the 18 non-zero data points being analysed. The between-study standard deviation was estimated to be 0.29 (95% CrI 0.02 to 0.85). This suggests that there was mild heterogeneity between studies in the intervention effects, although with some uncertainty about the true variability in intervention effects between studies.
There was evidence to suggest that CPAP is the most effective of the three interventions (probability = 0.989). The effect of CPAP relative to usual care was statistically significant at a conventional 5% level (OR 0.41, 95% CrI 0.20 to 0.77). The heterogeneity in the effect of CPAP between studies meant that the effect relative to usual care in a randomly chosen study varies according to the characteristics of the study (OR 0.41, 95% CrI 0.14 to 1.16). There was considerable uncertainty associated with the effect of BiPAP relative to usual care (OR 1.94, 95% CrI 0.65 to 6.14). The heterogeneity in the effect of BiPAP between studies meant that the effect relative to usual care in a randomly chosen study varies according to the characteristics of the study (OR 1.93, 95% CrI 0.50 to 7.98).
A sensitivity analysis was performed excluding the studies by Plaisance et al. ,50 Craven et al. 54 and Weitz et al. 55 A summary of the results from the NMA is presented in Table 6. There was little impact on the heterogeneity in intervention effects between studies. As before, the intervention that exhibited the greatest effect was CPAP (OR 0.45, 95% CrI 0.21 to 0.93), although the heterogeneity in the effect of NIV between studies means that the intervention effects in a randomly chosen study varies substantially depending on the characteristics of the study (OR 0.46, 95% CrI 0.13 to 1.41). The effect of BiPAP relative to standard care remained uncertain (OR 1.95, 95% CrI 0.43 to 9.46).
Treatment | Random-effects mean | Predictive distribution | Probability most effective | ||
---|---|---|---|---|---|
OR | 95% CrI | OR | 95% CrI | ||
NIV | |||||
BiPAP | 1.95 | 0.43 to 9.46 | 1.95 | 0.33 to 11.47 | 0.039 |
CPAP | 0.45 | 0.21 to 0.93 | 0.46 | 0.13 to 1.41 | 0.949 |
Usual carea | |||||
Reference | Reference | Reference | Reference | Reference | 0.012 |
Between-study standard deviation | 0.32 | 0.02 to 0.92 | – | – | – |
Data were available from eight studies,47–54 including five47,48,50,52,53 comparing CPAP with usual care and three studies49,51,54 comparing BiPAP with usual care. The analysis assumes that the lack of intubation data from the studies of Weitz et al. 55 and Austin and Wills46 are not related to the effects of the interventions in these studies. A summary of the results from the NMA is presented in Table 7.
Treatment | Random-effects mean | Predictive distribution | Probability most effective | ||
---|---|---|---|---|---|
OR | 95% CrI | OR | 95% CrI | ||
NIV | |||||
BiPAP | 0.40 | 0.14 to 1.16 | 0.40 | 0.12 to 1.39 | 0.361 |
CPAP | 0.32 | 0.17 to 0.62 | 0.32 | 0.13 to 0.82 | 0.639 |
Usual carea | |||||
Reference | Reference | Reference | Reference | Reference | 0.000 |
Between-study standard deviation | 0.21 | 0.01 to 0.73 | – | – | – |
The NMA model fitted the data well, with a residual deviance, 16.05, close to the total number of data points, 16, included in the analysis. The between-study standard deviation was estimated to be 0.21 (95% CrI 0.01 to 0.73). This indicated that there was mild heterogeneity between studies in the intervention effects, although with some uncertainty about the true variability in intervention effects between studies.
Both patients treated with BiPAP and those treated with CPAP were less likely to require intubation than those receiving usual care, although there was evidence to suggest that CPAP was the more effective intervention (probability = 0.639). The effect of CPAP relative to usual care was statistically significant at a conventional 5% level (OR 0.32, 95% CrI 0.17 to 0.62). The heterogeneity in the effect of CPAP between studies meant that the effect relative to usual care in a randomly chosen study varies according to the characteristics of the study (OR 0.32; 95% CrI 0.13 to 0.82). There was considerable uncertainty associated with the effect of BiPAP relative to usual care (OR 0.40, 95% CrI 0.14 to 1.16).
A sensitivity analysis was performed excluding the studies of Plaisance et al. ,50 Craven et al. 54 and Weitz et al. 55 A summary of the results from the NMA is presented in Table 8. There was a small increase in the between-trial standard deviation, although this is likely to be a consequence of there being fewer studies rather than a genuine increase in heterogeneity in intervention effects between studies. As before, the intervention that exhibited the greatest effect was CPAP (OR 0.34, 95% CrI 0.15 to 0.77), although the heterogeneity in the effect of NIV between studies means that the intervention effects in a randomly chosen study varies depending on the characteristics of the study (OR 0.34, 95% CrI 0.11 to 1.09). The effect of BiPAP relative to standard care remained uncertain (OR 0.53, 95% CrI 0.11 to 2.28).
Treatment | Random-effects mean | Predictive distribution | Probability most effective | ||
---|---|---|---|---|---|
OR | 95% CrI | OR | 95% CrI | ||
NIV | |||||
BiPAP | 0.53 | 0.11 to 2.28 | 0.53 | 0.09 to 2.87 | 0.306 |
CPAP | 0.34 | 0.15 to 0.77 | 0.34 | 0.11 to 1.09 | 0.692 |
Usual carea | |||||
Reference | Reference | Reference | Reference | Reference | 0.002 |
Between-study standard deviation | 0.27 | 0.02 to 0.86 | – | – | – |
Network meta-analysis using combined individual patient-level data and aggregate data
A NMA using combined IPD and aggregate data was undertaken to compare the comparative efficacy of pre-hospital NIV for adults with acute respiratory failure on mortality and intubation. Of the 10 included studies,46–55 IPD were available from only seven studies reporting a total of 650 patients. 47–51,53,55 Potential treatment effect modifiers were explored in separate analyses for age, sex, provider, primary diagnosis, severity of acute respiratory failure and pre-hospital time delay. Data on pre-hospital time delay were not well defined or reported and were not used in the analysis.
Despite the availability of IPD and aggregate data, a few discrepancies were noted. In the study by Ducros et al. 47 the number of events (i.e. death) reported in the intervention arm of the IPD set (n = 9) was higher than that reported for the aggregate data (n = 8). Similarly, in the study by Frontin et al. ,48 the number of events reported in the control arm of the IPD set (n = 8) was also higher than that reported for the aggregate data (n = 7).
The preliminary analysis of each study suggested that age, sex, primary diagnosis (ACPO, COPD) and respiratory rate could be the potential treatment effect modifiers (provider was not analysed because it was a study-level covariate). There was insufficient information on patients with a primary diagnosis of asthma and pneumonia to allow a meaningful estimate of treatment by diagnosis interaction; analyses were performed but results were extremely uncertain (result not provided). A summary of the results from the combined IPD and aggregate data NMA with covariates is presented in Tables 9 and 10.
Variable | Potential treatment effect modifiera | |
---|---|---|
Age | Respiratory rate | |
Data source | ||
IPD | Ducros et al.,47 Frontin et al.,48 Plaisance et al.,50 Roessler et al.,51 Thompson et al.,53 Mas et al.49 and Weitz et al.55 | Ducros et al.,47 Frontin et al.,48 Plaisance et al.,50 Roessler et al.,51 Thompson et al.,53 Mas et al.49 and Weitz et al.55 |
Aggregate data | Austin and Wills,46 and Craven et al.54 | – |
Coefficient of treatment effect modifier, OR (95% CrI) | ||
BiPAP | 1.04 (0.92 to 1.18) | 0.88 (0.70 to 1.04) |
CPAP | 1.02 (0.97 to 1.08) | 0.95 (0.88 to 1.03) |
Treatment effect at the average value of the treatment effect modifier, OR (95% CrI) | ||
BiPAP | 2.44 (0.76 to 8.71) | 2.66 (0.59 to 15.19) |
CPAP | 0.40 (0.19 to 0.77) | 0.62 (0.28 to 1.29) |
Between-study standard deviation (95% CrI) | 0.31 (0.02 to 0.87) | 0.30 (0.01 to 0.89) |
DIC (model with treatment effect modifier vs. model without treatment effect modifier) | 481.80 vs. 470.54 | 455.99 vs. 451.62 |
Variable | Potential treatment effect modifiera | |||
---|---|---|---|---|
Sex | ACPOb | COPDb | Provider | |
Data source | ||||
IPD | Ducros et al.,47 Frontin et al.,48 Plaisance et al.,50 Roessler et al.51 and Weitz et al.55 | Roessler et al.51 and Mas et al.49 | Roessler et al.,51 Thompson et al.53 and Mas et al.49 | – |
Aggregate data | Thompson et al.,53 Austin and Wills46 and Craven et al.54 | Ducros et al.,47 Frontin et al.,48 Plaisance et al.,50 Thompson et al.,53 Austin and Wills46 and Craven et al.54 | Ducros et al.,47 Frontin et al.,48 Plaisance et al.,50 Austin and Wills46 and Craven et al.54 | Ducros et al.,47 Frontin et al.,48 Plaisance et al.,50 Roessler et al.,51 Thompson et al.,53 Mas et al.,49 Weitz et al.,55 Austin and Wills46 and Craven et al.54 |
Coefficient of treatment effect modifier, OR (95% CrI) | ||||
BiPAP | 0.19 (0.01 to 2.44) | 1.45 (0.25 to 9.44) | 0.19 (0.01 to 1.70) | 0.57 (0.06 to 3.59) |
CPAP | 0.18 (0.04 to 0.74) | 1.30 (0.25 to 7.13) | 0.27 (0.03 to 1.92) | 1.43 (0.32 to 6.36) |
Treatment effect at the average value of the treatment effect modifier, OR (95% CrI) | ||||
BiPAP | Males: 0.55 (0.08 to 3.40) | Patients with ACPO: 2.07 (0.59 to 8.11) | Patients with COPD: 0.50 (0.04 to 4.34) | Physicians: 1.29 (0.19 to 7.45) |
Females: 2.92 (0.44 to 21.82) | Patients without ACPO: 1.41 (0.28 to 7.65) | Patients without COPD: 2.58 (0.82 to 9.51) | Paramedics: 2.31 (0.72 to 8.83) | |
CPAP | Males: 0.16 (0.05 to 0.44) | Patients with ACPO: 0.42 (0.20 to 0.81) | Patients with COPD: 0.12 (0.01 to 0.83) | Physicians: 0.55 (0.24 to 1.19) |
Females: 0.88 (0.34 to 2.20) | Patients without ACPO: 0.32 (0.06 to 1.60) | Patients without COPD: 0.45 (0.22 to 0.87) | Paramedics: 0.38 (0.10 to 1.41) | |
Between-study standard deviation (95% CrI) | 0.32 (0.01 to 0.89) | 0.31 (0.02 to 0.87) | 0.30 (0.01 to 0.86) | 0.25 (0.01 to 0.80) |
DIC (model with treatment effect modifier vs. model without treatment effect modifier) | 353.39 vs. 358.43 | 210.65 vs. 208.36 | 207.89 vs. 208.46 | 77.95 vs. 76.32 |
In general, the DIC for models with and without covariates were within 5 units, which is the range normally taken to mean that the models provide a similar fit to the data. However, when age was included as a covariate, the DIC increased from 470.54 to 481.80, suggesting that including age resulted in a worse-fitting model.
Combining the IPD and aggregate data in the NMA suggested that gender modifies the effect of CPAP relative to usual care [males : females OR 0.18, 95% CrI (0.04 to 0.74)] but there was no evidence that gender modifies the effect of BIPAP relative to usual care. After allowing for sex in the model, the effects of both CPAP and BiPAP relative to usual care for females were not statistically significant at a conventional 5% level for mortality (CPAP: OR 0.88, 95% CrI 0.34 to 2.20; BiPAP: OR 2.92, 95% CrI 0.44 to 21.82). The benefit of CPAP relative to usual care for males, in terms of reduced mortality, was statistically significant at a conventional 5% significance (OR 0.16, 95% CrI 0.05 to 0.44). However, the reduction in male mortality for BiPAP relative to usual care was not statistically significant at a conventional 5% significance level (OR 0.55, 95% CrI 0.08 to 3.40).
The preliminary analysis of each study suggested that sex, respiratory rate, oxygen saturation (SpO2), PaO2 and PaCO2 could be the potential treatment effect modifiers (provider was not analysed because it was a study-level covariate). Four studies were included in the analysis of whether or not PaO2 and PaCO2 were treatment effect modifiers: those of Ducros et al. 2011,47 Frontin et al. 2011,48 Plaisance et al. 200750 and Roessler et al. 2012. 51 Three out of these four studies (Ducros et al. 201147, Frontin et al. 201148 and Plaisance et al. 200750) compared CPAP with usual care, and only one study (Roessler et al. 201251) compared BiPAP with usual care. Hence there were not enough studies to estimate the coefficient of the treatment effect modifier for BiPAP, and whether or not PaO2 and PaCO2 were treatment modifiers was assessed only for CPAP. A summary of the results from the combined IPD and aggregate data NMA with covariates is presented in Tables 11 and 12.
Variable | Potential treatment effect modifiera | |||
---|---|---|---|---|
Respiratory rate | SpO2 | PaO2 | PaCO2 | |
Data source | ||||
IPD | Ducros et al.,47 Frontin et al.,48 Plaisance et al.,50 Roessler et al.51 and Mas et al.49 | Ducros et al.,47 Frontin et al.,48 Plaisance et al.,50 Roessler et al.51 and Mas et al.49 | Ducros et al.,47 Frontin et al.48 and Plaisance et al.50 | Ducros et al.,47 Frontin et al.48 and Plaisance et al.50 |
Aggregate data | Thompson et al.53 | Thompson et al.53 | – | – |
Coefficient of treatment effect modifier, OR (95% CrI) | ||||
BiPAP | 0.94 (0.77 to 1.12) | 1.02 (0.92 to 1.14) | – | – |
CPAP | 0.99 (0.90 to 1.10) | 1.02 (0.95 to 1.11) | 1.0 (0.99 to 1.02) | 1.03 (0.96 to 1.10) |
Treatment effect at the average value of the treatment effect modifier, OR (95% CrI) | ||||
BiPAP | 0.50 (0.10 to 2.33) | 0.57 (0.08 to 3.28) | – | – |
CPAP | 0.35 (0.15 to 0.83) | 0.34 (0.15 to 0.74) | 0.38 (0.14 to 0.97) | 0.32 (0.11 to 0.82) |
Between-study standard deviation (95% CrI) | 0.29 (0.01 to 0.91) | 0.26 (0.01 to 0.87) | 0.24 (0.01 to 0.81) | 0.24 (0.01 to 0.81) |
DIC (model with treatment effect modifier vs. model without treatment effect modifier) | 320.76 vs. 318.67 | 325.83 vs. 318.67 | 241.17 vs. 234.69 | 228.30 vs. 234.69 |
Variable | Potential treatment effect modifiera | |
---|---|---|
Sex | Provider | |
Data source | ||
IPD | Ducros et al.,47 Frontin et al.,48 Plaisance et al.50 and Roessler et al.51 | – |
Aggregate | Thompson et al.53 and Craven et al.54 | Ducros et al.,47 Frontin et al.,48 Plaisance et al.,50 Roessler et al.,51 Thompson et al.,53 Mas et al.,49 Weitz et al.,55 Austin and Wills46 and Craven et al.54 |
Coefficient of treatment effect modifier, OR (95% CrI) | ||
BiPAP | 3.42 (0.26 to 43.80) | 0.46 (0.04 to 2.81) |
CPAP | 3.61 (0.78 to 19.11) | 1.12 (0.26 to 4.59) |
Treatment effect at the average value of the treatment effect modifier, OR (95% CrI) | ||
BiPAP | Males: 0.37 (0.06 to 1.98) | Physicians: 0.23 (0.02 to 1.21) |
Females: 0.11 (0.02 to 0.63) | Paramedics: 0.51 (0.15 to 1.70) | |
CPAP | Males: 0.55 (0.21 to 1.43) | Physicians: 0.33 (0.15 to 0.70) |
Females: 0.16 (0.04 to 0.49) | Paramedics: 0.30 (0.09 to 1.00) | |
Between-study standard deviation (95% CrI) | 0.21 (0.01 to 0.74) | 0.23 (0.01 to 0.80) |
DIC (model with treatment effect modifier vs. model without treatment effect modifier) | 298.76 vs. 293.92 | 80.229 vs. 76.318 |
The DIC suggested that the models with covariate SpO2 and PaO2 were a poorer fit for the data than the model without these covariates. There is little to choose between models with covariates sex, respiratory rate and provider and the models without these covariates, as the DIC for models with and without these covariates were within 5 units. None of the coefficients of treatment effect modifiers was statistically significant at a conventional 5% significance level.
The model with covariate PaCO2 fitted the data better than the model without this covariate. However, PaCO2 was not a statistically significant treatment effect modifier at a conventional 5% significance level (coefficient for the CPAP arm: OR 1.03, 95% CrI 0.96 to 1.10).
Additional evidence
Supplementary evidence from 20 non-randomised observational studies (representing 21 articles)59–79 with relevant outcome data (namely intubation rates and mortality) from patients with acute respiratory failure following the application of NIV in the pre-hospital setting were identified and are reported here as additional evidence (i.e. data presented as structured tables with a narrative description, but without a formal quality assessment or analysis).
Eight studies60,64–67,72,74,77 described the use of pre-hospital NIV in patients with acute respiratory failure. A summary of the studies is presented in Table 13. Studies were published between 2005 and 2013, and were undertaken in France,65 Italy,72 the Netherlands77 and the USA. 60,64,66,67,74 Three studies64,72,74 collected data prospectively, while the remaining studies used a retrospective study design. 60,65–67,77
Author, year; country | Design | Population | Intervention | Comparator | Intubation rate | Mortality rate (within 30 days) | ||||
---|---|---|---|---|---|---|---|---|---|---|
Intervention | Control | p-value | Intervention | Control | p-value | |||||
Aguilar et al., 201159 (abstract) and 201360 USA (full text) | Retrospective cohort study (n = 410) | Patients with severe respiratory distress (CHF, COPD or asthma) | CPAP; 5–10 cmH2O by face maska (n = 175) | CMT including oxygen, nitrates, morphine and furosemide (n = 235) | 39/175 (22.3%) | 41/232 (17.7%) | 0.151 | 16/175 (9.1%) | 30/235 (12.8%) | 0.161 |
Mean age, years: NR but median 67 years | Provider: paramedic | |||||||||
Bultman et al., 2005;64 USA (abstract) | Prospective parallel cohort study (n = 467) | Patients in respiratory distress or suspected pulmonary oedema | CPAP (no further details provided) (n = 218) | Usual care (n = 249) | NR | NR | – | NR | NR | – |
Mean age, years: 73.8 | Provider: NR | |||||||||
Cuny et al., 2013;65 France (abstract) | Retrospective cohort study (n = 42) | COPD patients with respiratory failure | NIV (no further details provided) (n = 20) | No NIV (no further details provided) (n = 33) | NRb | NRb | – | NRb | NRb | – |
Mean age, years: 68.9 | Provider: NR | |||||||||
Derr et al., 2006;66 USA (abstract) | Retrospective cohort study (n = 128) | Patients with acute decompensated heart failure | CPAP (no further details provided) (n = 65) | Conventional treatment (no further details provided) (n = 63) | NR | NR | – | 9/65 (13.8%) | 11/63 (17.5%) | NR |
Mean age, years: NR | Provider: NR | |||||||||
Dib et al., 2012;67 USA | Retrospective cohort study (n = 387) | Patients with acute severe heart failure | CPAP; (fixed) 10 cmH2O (FiO2 30%) by face mask (n = 149) | CMT including oxygen, furosemide, nitrates, and morphine (n = 238) | 4/149 (2.7%) | 11/238 (4.6%) | < 0.01 | 0/149 (0%) | 0/238 (0%) | NR |
Mean age, years:c 74.8 | Provider: paramedic | |||||||||
Garuti et al., 2010;72 Italy | Prospective cohort study with a historical control (n = 206) | Patients with acute respiratory failure (owing to any cause, including ACPO, AECOPD and pneumonia) | Pre-hospital:CPAP; 5–10 cmH2O (FiO2, 30–50%) by helmet (n = 35) | In-hospital: CPAP; 5–15 cmH2O (FiO2 30–60%) by helmet (n = 46) | 0/35 (0%) | In-hospital: 0/46 (0%) | NR | 1/35 (2.9%) | In-hospital: 6/46 (13.0%) | Pre-hospital vs. control: 0.005 |
Mean age, years:c 77.7 | Provider: ambulance nurse | Historical control: CMT including oxygen (n = 125) | Historical control: 14/125 (11.2%) | Historical control: 30/125 (24.0%) | In-hospital vs. control: 0.03 | |||||
Pre-hospital vs. In-hospital: 0.0097 | ||||||||||
Hubble et al., 2006;74 USA | Prospective parallel cohort study (n = 215) | Patients with ACPO | CPAP; 10 cmH2O by face mask (n = 120) | CMT including oxygen, nitrates, furosemide and morphine (n = 95) | 10/120 (8.3%)d | 24/95 (25.3%) | 0.003 | 6/120 (5.0%) | 22/95 (23.2%) | < 0.001 |
Mean age, years:c 71.8 | Provider: paramedic | |||||||||
Spijker et al., 2013;77 the Netherlands | Retrospective cohort studye (n = 59) | Patients with ACPO | CPAP; 5 cmH2O by face mask (n = 16) | CMT including diuretics and vasodilators (n = 43) | 1/16 (6.3%) | 3/43 (7.0%) | NR | 2/16 (12.5%) | 4/43 (9.3%) | NR |
Mean age, years: NR, but median 84 years | Provider: paramedic |
While all studies included patients with acute respiratory distress there was wide variation in terms of underlying conditions resulting in respiratory failure. Moreover, in two studies,60,66 patients with acute decompensated heart failure and chronic heart failure were also eligible for inclusion. These conditions may be difficult to distinguish objectively from ACPO in the pre-hospital setting. The sample sizes of the studies ranged from 42 patients65 to 467 patients,64 with the mean age of participants ranging from 68.9 years65 to 77.7 years72 (no details of mean age were provided in three studies). 60,66,77
With the exception of one study65 (which provided limited data), all studies used CPAP as the mode of NIV in the intervention group. Although two studies64,66 did not provide details relating to the CPAP interface or pressure support level, four studies used a face mask60,67,74,77 and one used a helmet72 as the interface of choice for the administration of CPAP. NIV was administered by paramedics in four studies60,67,74,77 and by an ambulance nurse in one study. 72 Three studies64–66 provided no information on the category of medical personnel that administered pre-hospital NIV. Patients in the control groups were generally managed with conventional medical treatments (usual care) including oxygen for respiratory distress.
Comparison with non-randomised controls suggested lower intubation rates67,72,74,77 and mortality60,66,72,74,77 in patients receiving CPAP in addition to standard treatment. However, these non-randomised comparisons carry a high risk of bias and are unlikely to provide useful evidence of effectiveness when randomised comparisons are available. We did not therefore undertake further analysis of these data or attempt to draw any conclusions from them regarding effectiveness.
Data from non-randomised studies can provide some useful information about outcomes when interventions are used outside the trial setting. Mortality rates in the intervention groups ranged from 0%67 to 13.8%66 (median 7%), while intubation rates ranged from 0%72 to 22.3%60 (median 6%). These are similar to the mortality rates (range 0–21%, median 9%) and intubation rates (range 0–17%, median 8%) reported in the intervention groups of the randomised trials, suggesting that outcome rates reported in trials appear to be reproduced in more routine practice.
Twelve studies61–63,68–71,73,75,76,78,79 described the use of pre-hospital NIV in patients with acute respiratory failure. A summary of the studies is presented in Table 14. Studies were published between 2000 and 2013, and were undertaken in Finland,75 France,61,63,78 Greece,71,73 Italy,69 the Netherlands,68 Portugal,70 and the USA. 62,76,79 Two studies61,75 collected data retrospectively, while the remaining studies used a prospective study design. 62,63,68–71,73,76,78,79
Author, year; country | Design | Population | Intervention | Intubation rate | Mortality rate (within 30 days) |
---|---|---|---|---|---|
Berteloot et al., 2011;61 France (abstract) | Retrospective case series (n = 21) | Patients with severe respiratory distress (severe acute asthma, n = 8; and COPD, n = 13) | CPAP (no further details provided) | 5/21 (23.8%) | NR |
Mean age, years: severe acute asthma, 48 years; COPD, 68 years | Provider: NR | ||||
Bledsoe et al., 2012;62 USA | Prospective study (n = 340) | Patients with respiratory distress (acute pulmonary oedema/CHF, COPD, asthma and pneumonia) | CPAP; 10 cmH2O (FiO2 28–30%) by face mask | 19/340 (5.6%)a | NR |
Mean age, years: 67.7 | Provider: paramedic | ||||
Bruge et al., 2008;63 France | Prospective case series (n = 138) | Patients with severe respiratory failure (acute respiratory failure, CHF and COPD exacerbation) | BiPAP; 10–20 cmH2O (FiO2 adjusted to achieve an SpO2 > 95%; PEEP, 5 cmH2O) by face mask | 35/138 (25.4%) | NR |
Mean age, years: 75 | Provider: NR | ||||
Dieperink et al., 2009;68 the Netherlands | Prospective case series (n = 32) | Patients with severe acute respiratory distress (ACPO, COPD exacerbation and pneumonia) | CPAP; 2–8 cmH2O by face mask | NR | 11/32 (34.4%) |
Mean age, years: NR, but median 82 years | Provider: ambulance nurse | ||||
Foti et al., 2009;69 Italy | Prospective case series (two groups)b (n = 121) | Patients with presumed ACPO rescued by physician or nurse | CPAP; oxygen flow ≥ 30 l/minute; PEEP, 5–15 cmH2O (physician group) or 10 cmH2O (nurse group) by helmet | Physician group: 4/62 (6.5%) | Physician group: 11/62 (17.7%) |
Mean age, years:c 78.3 | Providers: physician or nurse | Nurse group: 5/59 (8.5%) | Nurse group: 9/59 (15.3%) | ||
Freitas et al., 2010;70 Portugal (abstract) | Prospective case series (n = 48) | Patients with ACPO | CPAP (no further details provided) | 2/48 (4.2%) | NR |
Mean age, years: 73.9 | Provider: NR | ||||
Fyntanidou et al., 2009;71 Greece (abstract) | Prospective study (n = 79) | Patients with ACPO | CPAP; 10 cmH2O (interface, NR) | 5/79 (6.3%) | 1/79 (1.3%) |
Mean age, years: 71.4 | Provider: physician | ||||
Grosomanidis et al., 2000;73 Greece (abstract) | Prospective study (n = 23) | Patients with pulmonary oedema (not specified) | CPAP; 5–7 cmH2O by face mask | 1/23 (4.3%) | NR |
Mean age, years: NR | Provider: physician | ||||
Kallio et al., 2003;75 Finland | Retrospective case series (n = 113)d | Patients with acute severe pulmonary oedema | CPAP; 5–12.5 cmH2O (FiO2 30–35%; PEEP 1 cmH2O per 10 kg body weight) by face mask | 6/113 (5.3%) | 9/113 (8.0%) |
Mean age, years: NR | Provider: physician | ||||
Kosowsky et al., 2001;76 USA | Prospective case series (n = 19) | Patients with acute respiratory failure owing to presumed ACPO | CPAP; 10 cmH2O (FiO2 35–95%) by face mask | 7/19 (36.8%) | 1/19 (5.3%) |
Mean age, years: 68.9 | Provider: paramedic | ||||
Templier et al., 2003;78 France | Prospective case series (n = 50) | Patients with presumed ACPO, COPD and hypoxaemic pulmonary disease | CPAP; 10 cmH2O with an EPAP level of 7 cmH2O by face mask | 10/50 (20.0%) | NR |
Mean age, years: 78 | Provider: physician | ||||
Warner, 2010;79 USA | Prospective study (n = 106)e | Patients with acute respiratory distress (underlying cause not specified) | CPAP; 7.5 cmH2O (interface, NR) | 0/106 (0%) | NR |
Mean age, years: NR | Provider: ALS team (no further details reported) |
While all studies included patients with acute respiratory distress, there was wide variation in terms of underlying conditions resulting in respiratory failure. The sample sizes of the studies ranged between 19 patients76 to 340 patients,62 with the mean age of participants ranging from 67.7 years62 to 78.3 years69 (no details of mean age were provided in four studies). 68,73,75,79, In one study,61 the mean age of patients was reported separately by underlying condition (asthma, 48 years and COPD, 68 years)
With the exception of one study (which used BiPAP via a single-use full-face mask),63 all studies used CPAP as the mode of NIV in the intervention group. Although four studies61,70,71,79 did not provide details relating to the CPAP interface, six studies used a face mask62,68,73,75,76,78 and one used a helmet69 as the interface of choice for the administration of CPAP. NIV was administered by paramedics in two studies,62,76 physicians in four studies,71,73,75,78 an ambulance nurse in one study,68 a physician or nurse in one study,69 and an emergency service team in one study. 79 Three studies61,63,70 provided no information on the category of medical personnel that administered pre-hospital NIV.
In non-randomised studies without a comparative group, intubation rates ranged from 0%79 to 36.8%. 76 Similarly, mortality rates ranged from 1.3%71 to 34.4%. 68 This wide variation in rates may be explained by differences in study populations and study methodologies in the included studies. Overall, intubation rates and mortality rates were generally higher in studies without a control group compared with studies with a control group.
Chapter 4 Assessment of cost-effectiveness
This chapter provides details on the methods and results of the health economic model constructed to evaluate the cost-effectiveness of pre-hospital NIV (specifically pre-hospital CPAP) for patients with acute respiratory failure. We developed a decision-analytic model to compare the costs and QALYs accrued by a theoretical population with acute respiratory failure attended by emergency ambulances providing pre-hospital CPAP to management by ambulances without pre-hospital NIV (standard care is assumed as hospital NIV).
Systematic review of existing cost-effectiveness evidence
The objective of this review was to identify and evaluate studies exploring the cost-effectiveness of pre-hospital NIV for patients with acute respiratory failure because of any cause or no specified cause.
Identification of studies
Electronic databases
Studies were identified by searching the following electronic databases and research registers:
-
MEDLINE(R) In-Process & Other Non-Indexed Citations and MEDLINE(R) (via OvidSP) from 1948 to August 2013
-
EMBASE (via OvidSP) from 1980 to August 2013
-
Cumulative Index to Nursing and Allied Health Literature (via EBSCOhost) from 1982 to August 2013
-
Cochrane Database of Systematic Reviews (via Wiley Online) from 1996 to August 2013
-
Cochrane Central Register of Controlled Trials (via Wiley Online) from 1898 to August 2013
-
Health Technology Assessment Database (via Wiley Online) from 1995 to August 2013
-
Database of Abstracts of Review of Effects (via Wiley Online) from 1995 to August 2013
-
NHS Economic Evaluation Database (via Wiley Online) from 1995 to August 2013
-
BIOSIS Previews (via ISI Web of Knowledge) from 1969 to August 2013
-
Science Citation Index Expanded (via Web of Science) from 1899 to August 2013
-
Conference Proceedings Index – Science (via Web of Science) from 1990 to August 2013
-
EconLit (via OvidSP) from 1961 to August 2013
-
UK Clinical Research Network Portfolio Database (NIHR) from 2001 to October 2012
-
National Research Register Archive (NIHR) from 2000 to September 2007
-
Current Controlled Trials from 2000 to October 2012
-
ClinicalTrials.gov (US National Institutes of Health) from 2000 to October 2012.
The keyword strategies developed in the review of clinical effectiveness (see Chapter 3, Methods for reviewing effectiveness, Identification of studies) were used with a sensitive economic evaluation (where applicable) or quality-of-life search filter aimed at restricting search results to economic and cost-related studies (used in the searches of MEDLINE, Cumulative Index to Nursing and Allied Health Literature and EMBASE). All resources were initially searched from inception to October 2012. With the exception of the four research registers, updated searches to August 2013 were conducted on the remaining electronic databases. An example of the MEDLINE search strategy is provided in Appendix 7.
Other resources
To identify additional published, unpublished and ongoing studies, the reference lists of all relevant studies (including existing systematic reviews) were checked and a citation search of relevant articles (using the Web of Science Citation Index Expanded and Conference Proceedings Citation Index – Science) was carried out to identify articles that cite the relevant articles. In addition, systematic keyword searches of the World Wide Web were undertaken using the Google search engine and key experts in the field were contacted.
All identified citations from the electronic searches and other resources were imported into and managed using the Reference Manager bibliographic software (version 12.0).
Inclusion and exclusion criteria
Studies were selected for inclusion according to predetermined inclusion and exclusion criteria. Studies were included if they reported an economic evaluation of pre-hospital NIV for patients with acute respiratory failure and estimated the benefits in terms of life-years gained or QALYs.
Studies that performed economic evaluations alongside trials were excluded if they did not extrapolate the outcomes beyond the trial duration, as these economic analyses are only valid for the trials under consideration. Studies that were considered to be methodologically unsound, that were not reported in sufficient detail to extract costs and outcome estimates (including abstracts) or did not report an estimate of cost-effectiveness (e.g. costing studies) were also excluded. Papers not published in the English language were also excluded.
The inclusion of potentially relevant articles was undertaken using a two-step process. First, all titles were examined for inclusion by one reviewer. Any citations that clearly did not meet the inclusion criteria were excluded. Second, all abstracts and full-text articles were examined independently by two reviewers and any disagreements in the selection process were resolved through discussion.
Results of the cost-effectiveness review
The electronic literature searches identified 214 potentially relevant publications. Of these, one study80 met the inclusion criteria. A flow chart describing the process of identifying relevant literature can be found in Figure 5. Further details of the included study including an assessment of its methodological quality are provided below.
Quality assessment strategy
The methodological quality of each included study was assessed using a combination of key components of the Drummond and Jefferson81 and Drummond et al. 82 guidelines for economic evaluations, together with the Eddy checklist for mathematical models used in technology assessments. 83 The use of the checklist ensured a consistent approach to assessing the quality of each economic evaluation.
Cost-effectiveness review summary
Hubble et al. 80 assessed the cost-effectiveness of pre-hospital CPAP compared with no CPAP in managing ACPO in a typical urban ambulance service. Using estimates from published reports on pre-hospital and emergency department CPAP, a cost-effectiveness model of implementing CPAP in a typical urban ambulance service was derived from the societal perspective as well as the perspective of the implementing service. The model used a 1-year time horizon. The theoretical service would be expected to use CPAP four times per 1000 patients and expected to save 0.75 additional lives per 1000 patients at a cost of US$490 per life saved. CPAP is also expected to eliminate the need for approximately one in six intubations and reduce hospitalisation costs by US$4075 per year for each application. The model was verified to be robust across a wide range of input variable assumptions.
Comments
The analysis was performed for patients with ACPO and the main outcomes measured were the reduction in hospitalisation costs and mortality. In order to evaluate the clinical effectiveness, a meta-analysis of six clinical trials of in-hospital CPAP was carried out. One of the limitations of this study is the use of data from studies of emergency departments owing to a lack of adequate numbers of pre-hospital studies. Most of the costs were presented in a detailed and systematic way. Initial capital costs of CPAP equipment purchasing were expensed over a projected useful lifespan of 5 years. Training costs of different staff groups and other resource costs were extracted from the trial data. One-way sensitivity analysis was performed, but the authors did not perform a probabilistic sensitivity analysis. The model did not estimate QALYs as the measure of effectiveness and thus, the cost-effectiveness analysis results are not applicable to the current decision problem. Furthermore, the model used only a 1-year time horizon, which does not take the full lifetime costs and outcomes into account. Thus, the validity of findings from this study is still uncertain.
Independent economic assessment methods
This section details the methods and assumptions of the de novo economic model constructed to evaluate the cost-effectiveness of pre-hospital NIV (specifically pre-hospital CPAP) compared with standard care for patients with acute respiratory failure.
Objectives
The objectives of the cost-effectiveness analysis were to:
-
estimate the cost-effectiveness of pre-hospital CPAP compared with standard care for patients with acute respiratory failure, in terms of the costs and QALYs gained by each strategy
-
identify the strategy that is most likely to be cost-effective for patients with acute respiratory failure, defined as the most cost-effective strategy at a willingness-to-pay threshold of £20,000–30,000 per QALY gained
-
identify the expected cost of pre-hospital CPAP and whether or not future research would be valuable by estimating the expected value of perfect information (EVPI)
-
identify the critical areas of uncertainty where future research would produce most benefit and recommend specific primary research designs to address the uncertainty around using expected value of sample information (EVSI) techniques.
The costs and benefits of pre-hospital continuous positive airway pressure
The treatment of acute respiratory failure depends on the underlying cause, but patients often require treatment in the ambulance while en route to hospital (pre-hospital treatment). The risk of death in patients with respiratory problems increases markedly with distance travelled to hospital, from 10% at distances below 10 km to 20% at distances over 20 km.
The main benefits of pre-hospital CPAP relate to the reduction of mortality and intubation rates for these patients. Reduced intubation rates translate into reduced requirement for intensive care and hence reduced health-care costs. Reduced short-term mortality translates into long-term health benefits in terms of QALYs accrued by additional survivors.
The direct costs of pre-hospital CPAP include the costs of delivering the CPAP in the ambulance and the subsequent costs of providing treatment in the hospital. Most of the costs of pre-hospital CPAP are set-up costs, with small additional costs per patient treated. In order to set up a service of pre-hospital CPAP, there are a number of initial costs such as equipment costs, staff training costs and service reconfiguration costs. This is a key determinant of cost-effectiveness as the unit cost of pre-hospital CPAP will involve dividing the total costs of providing CPAP by the number of patients treated and receiving benefit (i.e. the number of patients treated will determine cost-effectiveness).
The decision-analytic model structure
A de novo economic model was developed using R software (The R Foundation for Statistical Computing)84 to explore the costs and health outcomes associated with pre-hospital CPAP and standard care. The economic perspective of the model is the NHS in England and Wales with the structure of the model shown in Figure 6.
FIGURE 6.
Model structure.

The different interventions (pre-hospital CPAP and standard care) were applied to a hypothetical cohort of patients with acute respiratory failure, that is, all patients receive pre-hospital CPAP in the intervention group and standard care in the comparator group. The model assigned to each patient a probability of intubation or death depending on their characteristics and whether they received pre-hospital CPAP or standard care. The patients who survived accrued lifetime QALYs and health-care costs according to their life expectancy. Costs were also accrued through costs of the intervention (i.e. pre-hospital CPAP) and hospital treatment costs which depended on whether or not the patient needed intubation. Details of each of these processes are outlined below.
Model structure
A decision-analytic model was developed to estimate the costs and health outcomes associated with pre-hospital CPAP and standard care in a hypothetical cohort of patients with acute respiratory failure.
Population
The population consisted of a hypothetical cohort of patients with acute respiratory failure of any cause or no specified cause in a given ambulance service setting. Although this cohort can include patients with ACPO/heart failure, COPD or pneumonia, for the purpose of modelling they are treated as a single group. Using an incidence rate, the annual number of instances of patients with acute respiratory failure for a given ambulance service can be estimated based on the population served.
Intervention
There are multiple alternative specifications of the pre-hospital NIV approaches reported in the studies included within the systematic review; the interventions were classified and specified as reported in Chapter 3. These include (a) early CPAP provided by medical responders, (b) CPAP provided by paramedics, (c) CPAP provided by medical responders, (d) BiPAP provided by medical responders and (e) BiPAP provided by emergency physicians.
The clinical expert group decided that CPAP provided by paramedics was the approach most likely to be feasible in the UK, based on knowledge of currently available equipment, training requirements, anticipated costs, existing guidelines and the practicality of use in NHS ambulances. These factors all suggested that the model of pre-hospital NIV delivery most likely to be used in the NHS was CPAP delivered by paramedics. The meta-analysis would generate an estimate of treatment effect for the model by assuming a general effect for CPAP, regardless of whether it was provided by paramedics or physicians.
Comparator
Again, there are multiple alternative specifications of standard care reported in the studies included within the systematic review, which include (a) standard care without any NIV, (b) standard care with in-hospital NIV and (c) delayed pre-hospital NIV.
However, for the purposes of the economic model, in-hospital NIV is chosen as standard care. This assumption was deemed sensible by the clinical expert group, as any patient who received pre-hospital CPAP appropriately would have received in-hospital NIV if pre-hospital CPAP were not available.
Outcomes
The main outcomes in the model were QALYs, which are accrued by patients who survive their acute event (i.e. survive to 30 days).
Model perspective
The model took a lifetime horizon and the economic perspective of the model was the NHS in England and Wales.
Discount rate
Both the costs and QALYs were discounted at an annual discount rate of 3.5%, as recommended by NICE. 85
The key modelling methods together with the evidence sources and assumptions used to populate the model are discussed in detail in the following sections, Short-term outcomes, Effect of pre-hospital continuous positive airway pressure, Long-term health outcomes and Costs.
Short-term outcomes
Patients with acute respiratory failure are at increased risk of both fatal and non-fatal major adverse cardiovascular events. The main outcomes of interest were short-term mortality and risk of intubation. The model estimated the prognosis of each patient by using a 30-day probability of death and probabilities of intubation depending on the type of treatment. This subsection details the baseline risks of intubation and death (i.e. for patients in standard care) estimated using data from the literature and the effectiveness of pre-hospital NIV in reducing mortality and intubation risks from NMA.
Mortality risk
The primary outcome measure, mortality, was typically recorded as a binary outcome and results presented as the response rate at 30 days. This 30-day mortality includes deaths in the ambulance and in the hospital.
The mortality risk of emergency admissions with respiratory illness was modelled using a large cohort data set of 668 patients presented with ‘respiratory disease’ across four ambulance services over a 4-year period from 1997 to 2001. 6 These were the Royal Berkshire Ambulance Service, the Derbyshire Ambulance Service, the Essex Ambulance Service and the West Midlands Ambulance Service. These ambulance services were representative of the types of environment typically encountered in England and included urban, mixed urban and rural, and very rural areas. Patients with ‘respiratory symptoms’ (n = 59, one death) were excluded, as these symptoms seemed to be less severe. There were 79 deaths in 668 patients, which resulted in a mean mortality rate of 11.8%. This was similar to that reported in a systematic review of 15 trials of NIV in ACPO, which reported an average mortality of 10.7% (42 deaths in 389 patients). 22 Thus, in the economic model a mean mortality rate of 11.8% was used with a beta distribution as shown in Table 15.
Scenario | Mortality rate | Distribution |
---|---|---|
General population scenario | 0.118 | Beta(79,589) |
Rural scenario | 0.141 | Beta(18,109) |
Urban scenario | 0.110 | Beta(21,166) |
Scenarios for cost-effectiveness analysis
The distance from hospital of patients is an important factor when considering the optimal cost-effectiveness strategy because of the increase in mortality with increase in distance to hospital for the patient. More specifically, it is reasonable to assume that NIV is one of the interventions that, being currently available only in hospital, accounts for some of the association between distance and mortality. This effect of distance on mortality of emergency admissions for respiratory illness can be observed in the large cohort of 668 patients presenting with ‘respiratory disease’ across four ambulance services over a 4-year period from 1997 to 2001. 6 This relationship can be observed in the raw data, as shown in Figure 7, which show an increase in mortality with an increase in the distance to hospital. Appendix 8 shows mortality variation with distances, categorised as short (< 10 km), medium (10–20 km) and long (> 20 km), with longer distances associated with higher mortality.
FIGURE 7.
Variation in mortality with distance to hospital.

In addition, the average distance to hospital and the distribution of distance to hospital for a cohort of patients will vary according to the geographical setting of the ambulance service. For example, the distance to hospital is greater, on average, for patients in a rural setting (South Western Ambulance Service) than for patients in an urban setting (West Midlands Ambulance Service). Thus, with regard to pre-hospital CPAP for patients with acute respiratory failure, we tested the model in three different scenarios:
-
General population scenario: average distance to hospital and the distribution of distance to hospital is that of the general population in the UK. This scenario reflects the cost-effectiveness of different strategies at the national level.
-
Rural population scenario: average distance to hospital and the distribution of distance to hospital is that of a typical rural setting in the UK. This scenario reflects the cost-effectiveness of different strategies at the rural level; thus, the services in rural areas are able to decide if this scenario and the results best reflect their local practice.
-
Urban population scenario: average distance to hospital and the distribution of distance to hospital is that of a typical urban setting in the UK. This scenario reflects the cost-effectiveness of different strategies at the urban level; thus, the services in urban areas are able to decide if this scenario and the results best reflect their local practice.
This approach was taken because it was possible that different strategies may have different levels of cost-effectiveness in different settings. The decision to commission pre-hospital CPAP service is typically made at the ambulance service level and thus, the users of the results are able to decide which scenario best reflects their local practice and if pre-hospital CPAP is a cost-effective use of resources in their setting. For example, the cost-effectiveness of pre-hospital CPAP may be different in urban and rural areas, that is, it is possible that it might be more cost-effective in a rural setting, where the average distance to hospital is high, but less cost-effective in an urban setting, where the distance to hospital is not high.
The different scenarios are implemented in the model using three different baseline mortality risks: for the general population scenario, the rural population scenario and the urban population scenario. The mortality risk for the general population scenario is 11.8%, as described earlier in this section. The mortality rate for South Western Ambulance Service is used as the mortality risk for the rural population scenario and the mortality rate for West Midlands Ambulance Service is used as the mortality risk for the urban population scenario. The mortality rates used for the different scenarios are as shown in Table 15.
Risk of intubation
A key secondary outcome measure of interest is the intubation rate, which is recorded as a binary outcome. This risk of intubation for respiratory illness was modelled using the data from 3CPO study,18 a multicentre open prospective RCT of 1069 patients presenting with severe ACPO at 26 emergency departments in the UK. The 3CPO study reported a mean intubation rate of 2.9%. This is similar to the intubation rates of 2.7% reported in the British Thoracic Society’s National Respiratory Audit Programme Annual Report 2011/12. 86 The variance was estimated as 0.003% based on elicitation from the clinical experts. The mean value of 2.9% and the variance was then used to calculate the α and β parameters for a beta distribution and, thus, in the model, intubation rate is represented as a beta distribution with an alpha of 4.45 and a beta of 150.
Effect of pre-hospital continuous positive airway pressure
Log-ORs for mortality and intubations were used as effectiveness parameters in the model for pre-hospital CPAP. These effectiveness parameters are estimated from the meta-analysis reported in Chapter 3, Effects of interventions. All analyses were performed using an intention-to-treat analysis, that is, all patients and their outcomes were analysed in the groups to which they were allocated, regardless of whether or not they received the treatment. These log-ORs parameters are assumed to be independent of the patient’s distance from the hospital, that is, the same effectiveness parameters are applied to all patients irrespective of how far they are from the hospital.
Effectiveness parameters of pre-hospital continuous positive airway pressure used in the model
The ORs estimated from the NMA, as reported in Chapter 3, Effects of interventions, are used as estimates of effectiveness for pre-hospital CPAP. The probabilities of mortality and intubation for patients given pre-hospital CPAP were estimated by applying the log-ORs to the baseline parameters using the formulae below. If the baseline event rate is P, then µ is estimated as logit(P) = log[P/(1 – P)]. Then the absolute probabilities for the interventions (i.e. BiPAP and CPAP) are estimated as
where d is the log-OR for an intervention relative to standard care estimated from the NMA.
Effect on mortality
Pre-hospital CPAP can reduce the mortality of patients as they receive the NIV earlier in the ambulance than they would have if they had to wait to get to the hospital. The log-OR of pre-hospital CPAP for mortality reduction is estimated from the meta-analysis reported Chapter 3, Effects of interventions.
As shown in Table 15, there is an increase in mortality with increase in distance to hospital, with the mortality of patients > 20 km from the hospital twice as high as those < 10 km from the hospital. This suggests that, despite the constant mortality, the absolute benefit increases as the patient’s distance from the hospital increases, that is, patients farther from the hospital achieve more benefit from pre-hospital CPAP than those who are closer; for example, the absolute effectiveness of pre-hospital CPAP is higher in a rural setting than in an urban setting.
Effect on risk of intubation
The effect of pre-hospital CPAP on risk of intubation is modelled as log-OR estimated from the meta-analysis reported in Chapter 3, Effects of interventions. This log-OR parameter is assumed to be independent of the patient’s distance from the hospital, that is, the same risk reduction is applied to all patients irrespective of how far they are from the hospital.
The reduction in number of intubations leads to a reduction in the mean hospital length of stay because mean hospital length of stay for patients without intubation is 5.84 days, compared with 10.82 days for patients with intubation. Thus, the effect of pre-hospital CPAP on the length of hospitalisation is not modelled as an independent relative risk to avoid double counting; it was assumed that the reduction in the mean hospital length of stay is achieved only by reducing the number of intubations.
Long-term health outcomes
The patients who survived (i.e. who avoided the short-term mortality risk) accrued QALYs and these lifetime QALYs are estimated based on patients’ life expectancy and their utilities.
The life expectancy of patients with acute respiratory failure admitted to hospital was captured from the 3CPO trial,18 which reported that the mean life expectancy for patients, if they were alive at 6 months, was 3.505 years. In the 3CPO trial,18 75% of the patients were alive at 6 months and an average life expectancy of 2 months was assumed for the remaining 25% of patients, which resulted in the mean discounted life expectancy estimated at 2.67 years. In the model, life expectancy is parameterised as a normal distribution with a mean of 2.67 years and standard deviation of 0.16 years, after discussions with the clinical expert group. This is similar to the mean life expectancy reported for patients with COPD and acute respiratory failure treated with NIV.
There was no evidence that patients who survived after receiving pre-hospital NIV experienced better health-related quality of life than patients given standard care, so it was assumed that for a patient with given characteristics the health-related quality of life was the same in both strategies, that is, pre-hospital CPAP and standard care. The 3CPO study18 reported that the mean utility value was 0.6 and a variance of 0.0225% was estimated from the clinical expert group. A search for studies of survival and quality of life after admission with pneumonia or COPD also reported similar utility values. The mean utility value and variance were then used to calculate the α and β parameters for a beta distribution and, thus, the utility values in the model are represented as a beta distribution with an α of 640 and a β of 425.
The estimated QALYs for patients with acute respiratory failure were estimated by multiplying the life-years by the lifetime quality of life shown in Table 16. It was assumed that the lifetime QALYs were same for all survivors, irrespective of whether they were in the standard care or pre-hospital CPAP arm.
Parameter | Central estimate | Distribution |
---|---|---|
Mean lifetime years | 2.67 years | Normal(2.67,0.16) |
Mean lifetime quality of life | 0.6 | Beta(640,425) |
Costs
The costs included in the model are:
-
costs of pre-hospital CPAP
-
costs of standard care
-
intubation costs
-
hospitalisation costs
-
lifetime costs of care for patients.
Table 17 shows the average costs and their distributions included in the model. The details of how these costs are derived are presented in the following subsections.
Parameter | Cost (£) | Distribution |
---|---|---|
Pre-hospital CPAP | 1212 | £1500 – £1000 × beta(2,5) |
Hospitalisation costs | 2250 | Gamma(75,30) |
Intubation costs | 3500 | Gamma(70,50) |
Annual costs | 5300 | Gamma(53,100) |
Costs of standard care
The cost of standard care was assumed as £0. This simplification was made as the analysis is based on incremental costs, that is, it was assumed that all initial treatment costs are same, regardless of whether or not the patient gets pre-hospital CPAP. The zero costs for standard care relates only to pre-hospital and emergency department treatment and does not include hospitalisation costs, intubation costs or additional lifetime costs for survivors. This was deemed sensible by the expert clinical group as it was assumed that all patients would receive NIV in hospital, irrespective of whether or not patients received pre-hospital CPAP. This does not cause any bias even if there are different mortality rates in pre-hospital CPAP and standard-care patients because deaths typically occur during or after emergency department treatment, so it is reasonable to assume emergency department costs are the same regardless of mortality rate. Thus, the only difference in treatment costs between standard care and pre-hospital CPAP was the additional costs of pre-hospital CPAP.
Costs of pre-hospital continuous positive airway pressure
There are a number of costs involved in providing pre-hospital CPAP, such as initial equipment costs, implementation costs and ongoing maintenance costs. These costs were converted into a cost per patient based on a 5-year depreciation period (i.e. assuming new pre-hospital NIV equipment will be required in 5 years) and sharing the overall costs out among the number of patients that would benefit from the service over this time period.
Number of patients receiving pre-hospital continuous positive airway pressure in a typical ambulance service
The incidence of patients who will benefit from pre-hospital CPAP is one of the key parameters in the model, as the unit cost of pre-hospital CPAP is estimated by dividing the total costs of pre-hospital CPAP to the ambulance service by the number of patients treated. Estimates of this incidence vary between sources, as shown in Table 18, and the different values are synthesised to achieve a distribution for the costs of pre-hospital CPAP.
Source | Incidence of eligible patients per 100,000 | Annual number of eligible patients in an ambulance service | Unit cost (£) of pre-hospital CPAPa |
---|---|---|---|
Spijker et al.77 | 3.5 | 175 | 1346.76 |
Aguilar et al.60 | 7.3 | 365 | 744.58 |
Luhr et al.87 | 17.8 | 890 | 417.40 |
Hubble et al.80 | 34.2 | 1700 | 309.02 |
BTS audit86 | 36.1 | 1800 | 302.40 |
STH ED data | 40.8 | 2000 | 291.15 |
Spijker et al. 77 reported that 16 patients received pre-hospital CPAP over an 11-month period in an ambulance service covering a population of 500,000, which amounts to 3.5 potentially eligible cases per 100,000 per year. This study identified only patients with ACPO, and many eligible patients did not receive treatment (which admittedly may reflect real life), and hence this could be an underestimate of true incidence. Similarly, Aguilar et al. 60 reported that 175 patients received pre-hospital NIV across 22 months in an ambulance service covering a population of 1.3 million, which amounts to 7.3 potentially eligible cases per 100,000 per year.
Luhr et al. 87 estimated 77.6 cases of acute respiratory failure per 100,000 per year. Of these, 13.7% were a result of COPD and 9.2% were a result of ACPO (i.e. cases with potential to benefit from pre-hospital NIV). Thus, the incidence can be estimated as 17.8 potentially eligible cases per 100,000 per year. However, these are relatively old data and include patients who develop acute respiratory failure in hospital, and so may be an overestimate.
The British Thoracic Society’s National Respiratory Audit Programme Annual Report 2011/1286 reported that 130 hospitals submitted data on 2490 patients with NIV between 1 February 2012 and 31 March 2012 (i.e. 2 months). This amounts to 19.15 patients (2490/130) per hospital per 2 months, which in yearly terms equates to 115 patients per hospital per year. There are 168 acute hospitals in England, serving a population of 53.01 million, which gives an incidence of 36.4 eligible cases per 100,000 population. However, the details of the audit were not clear and may be subject to bias. Furthermore, it includes patients who develop acute respiratory failure in hospital and so may be an overestimate.
In the Sheffield Teaching Hospital emergency department, 255 sets of NIV equipment were used over 1 year. This hospital serves a population of 551,800, which equates to 46.2 potentially eligible cases per 100,000 per year. However, the equipment may not actually have been used for patient care, or multiple pieces of equipment may have been used for the same patient, so this is likely to be an overestimate.
Hubble et al. 80 estimate that 4 per 1000 patients transported by ambulance are eligible for NIV. In 2011–12 there were 4.53 million emergency ambulance transfers to a type 1 or 2 emergency department in England (population 53.01 million). If 4 per 1000 of these patients were eligible, this suggests an incidence of 34.2 eligible cases per 100,000 population.
Total costs of pre-hospital continuous positive airway pressure to the ambulance service
The costs were often missing from the studies included in the review and, thus, bottom-up costing methods were used to estimate the costs of pre-hospital CPAP. The breakdown of the costs for pre-hospital CPAP is shown in Table 19 and is split into three main components:
-
initial costs of the pre-hospital CPAP devices
-
set-up/implementation costs (i.e. staff training costs and service reconfiguration costs)
-
maintenance costs of the service (i.e. consumables, depreciation).
Device costs | |||||
---|---|---|---|---|---|
Category | Number of devices | Source | Unit cost (£) | Source | Total cost (£) |
Out-of-hospital CPAP device | Number of ambulances that need the CPAP device (420) | Expert advisory input | 513.49 | Vygon Ltd, UK: hospital CPAP kit | 513.49 × 420 |
Assuming 10% new CPAP devices over 5-year use (42) | Expert advisory input | 513.49 | Vygon Ltd, UK: hospital CPAP kit | 513.49 × 42 | |
Total cost of the device | 237,232 | ||||
Set-up/implementation costs | |||||
Category | Resource use | Source | Unit cost (£) | Source | Total cost (£) |
Initial training | 1500 paramedics for 2 days each | Expert advisory input | 150 per day | Expert advisory input | 450,000 |
Service reconfiguration | One-off cost for reconfiguration | Expert advisory input | 100,000 | ||
Total set-up/implementation costs | 550,000 | ||||
Maintenance costs | |||||
Category | Resource use | Source | Unit cost (£) | Source | Total cost (£) |
Consumables | Number of patients over 5 years = 5 × n | Expert advisory input | 189.93 per use | Vygon Ltd, UK: facial mask, oxygen tubing and valve | 189.93 × 5 × n |
Ongoing training | 1500 paramedics for 1 day each | Expert advisory input | 150 per day | Expert advisory input | 225,000 |
Total maintenance costs | 225,000 + 949.65 × n | ||||
Total costs | |||||
Total costs of out-of-hospital CPAP | 1,012,232 + 949.65 × n | ||||
Total number of patients [n patients per year × depreciation period of 5 years (i.e. assuming new out-of-hospital CPAP equipment will be required in 5 years)] | 5 × n | ||||
Cost of out-of-hospital CPAP per patient | 189.93 + 202,446/n |
The costs of the pre-hospital CPAP devices were elicited from the expert advisory group. The costs of implementation on provider organisations were estimated using bottom-up costing methods assuming a typical ambulance service. The maintenance costs were estimated using activity-based costing for the resources spent on consumables based on evidence from the literature.
The pre-hospital CPAP device can take different levels of complexity and the cost of the device is based on this complexity. For example, the costs are different for the non-invasive positive-pressure ventilation devices and CPAP/BiPAP devices. Furthermore, the costs are also dependent on whether the devices use a cylinder or are electrically/mechanically powered. The costs of the device were elicited from the expert advisory group, assuming a close-fitting face mask CPAP device with Boussignac CPAP system manufactured by Vygon Ltd, UK, as representative of a typical CPAP system. The Boussignac hospital CPAP kit costs £513.49 and contains the equipment required to deliver out-of-hospital CPAP. We assumed that each ambulance would have the equipment and 10% would need to be replaced over the 5-year period.
The costs of implementation on provider organisations was estimated using bottom-up costing methods assuming a typical ambulance service, based on the mean size of NHS ambulance services in the UK. It was assumed that a typical ambulance service would have an average capacity of 1500 paramedics, which was deemed sensible by the expert advisory group. The group also suggested that an average of 2 days per year should be allocated to paramedics’ training. The costs associated with training were estimated by multiplying this paramedic time by their daily rate according to Personal Social Services Research Unit in 2012. 88 The daily cost per working day was estimated as £150 assuming an average salary of £40,000 (including overheads if they are in band 6/7) at the suggestion of the clinical advisory group. Service reconfiguration costs were estimated as a one-off cost of £100,000, and this included the cost of developing new guidelines/pathways. Installation costs were assumed to be zero as the CPAP system under consideration is a disposable system.
The maintenance costs were estimated using costing for the resources spent on consumables based on information from the manufacturers that the facial mask, oxygen tubing and valve (costing £189.93) would need to be replaced after each use, while the rest of the equipment was reusable. The expert advisory group suggested that an average of 1 additional day half-way through the 5-year period will be required by paramedics to update their training.
Scenarios for costs of pre-hospital continuous positive airway pressure to the ambulance service
As seen above, estimates of incidence reported in different sources are substantially different; they are summarised in Table 18. A typical ambulance service caters for a population of around 5 million, which suggests a range from around 175 to 2000 patients per ambulance service in a year depending on the estimate of the incidence. Thus, scenario analysis was conducted by estimating the unit cost for providing pre-hospital CPAP for these different estimates of the eligible population. In addition, this information was synthesised into an expression for the pre-hospital CPAP costs as £1500 – £1000 × β(2,5). This was chosen because our clinical experts believed that most of the samples of costs will fall between £1400 and £800, with only a few instances when the costs are lower than £800.
Three different cost scenarios were analysed alongside the baseline analysis:
-
A high-cost scenario assumed 170 patients per year would be eligible for pre-hospital CPAP with a unit cost of £1400.
-
A low-cost scenario assumed 365 patients per year would be eligible for pre-hospital CPAP with a unit cost of £745.
-
A lower-cost scenario assumed 1700–2000 patients per year would be eligible for pre-hospital CPAP with a unit cost of £300.
Hospitalisation costs
A further main outcome included in the model is the cost of stay associated with hospitalisations. The hospitalisation stay is dependent on whether or not the patient needs intubation, because length of stay is longer for patients who undergo intubation. Hubble et al. 80 report that the mean hospital length of stay for patients without intubation is 5.84 days. This is similar to the mean length of stay for patients with respiratory failure in the UK, estimated as the weighted average of 8801 patients associated with DZ27D and DZ27E (respiratory failure without intubation with major complications and comorbidity, and respiratory failure without intubation with intermediate complications and comorbidity) reported in the NHS Reference Costs 2011–2012. 89 Thus, the mean inpatient admission cost for hospitalisations was calculated as the weighted average of the costs of patients with DZ27D and DZ27E, from the NHS Reference Costs 2011–2012. 89 The hospitalisation cost used in the model, with a mean cost of £2400, is represented as a gamma distribution with an α of 80 and a β of 30.
Intubation costs
The cost of intubation was estimated as a one-off cost. Although costs for the DZ27A and DZ27B (respiratory failure with intubation with major complications and comorbidity, and respiratory failure with intubation with intermediate complications and comorbidity) were reported in the NHS Reference Costs 2011–2012,89 they were based on a small sample size of 180 patients across the UK whose average total length of stay is 7 days, that is, an additional 1.2 days for intubation. The clinical expert group deemed this as not representative and suggested using the data from Hubble et al. 80 to estimate the costs of intubation. Hubble et al. 80 report a mean hospital length of stay of 10.82 days for patients with intubation (i.e. approximately an additional 5 days for intubation compared with patients without intubation) and it was assumed that 5 additional hospital days spent by the intubated patients will be in the intensive care unit, based on the suggestions by the clinical expert group.
Thus, the cost of intubation was estimated by multiplying intensive care unit costs of £700 per day80 by the average length of stay for intubation, assumed to be 5 days, which results in a mean cost of intubation of £3500. The cost of intubation used in the model is represented as a gamma distribution with an α of 70 and a β of 50.
Lifetime costs of care
Lifetime costs of survivors were estimated using the annual costs and the discounted life expectancy of patients captured from the 3CPO trial. 18 The 3CPO study reported that the mean cost in months 4–6 was £1341, which resulted in mean annual costs of £5300. In the model, the annual cost is parameterised as a gamma distribution with an α of 53 and a β of 100, after discussions with the clinical expert group. It was assumed that the lifetime costs were the same for all survivors, irrespective of whether they were in the standard care or pre-hospital CPAP arm.
Summary of modelling input parameters
The decision-analytic model assigned to each patient a baseline probability of death and intubation. The risks of death and intubation for pre-hospital CPAP were estimated by applying the ORs from the meta-analysis to the baseline risks of mortality and intubation. Each patient alive then accumulated costs and QALYs based on the cost parameters, life expectancy and utility values. A summary of the model parameters is provided in Table 20.
Parameter | Mean | Distribution | Source |
---|---|---|---|
Baseline risks | |||
Scenario analysis: distribution of 30-day mortality risk | |||
General population mean 30-day mortality probability | 0.118 | Beta(79,589) | Nicholl et al.6 |
Rural scenario mean 30-day mortality probability | 0.141 | Beta(18,109) | Nicholl et al.6 |
Urban scenario mean 30-day mortality probability | 0.110 | Beta(21,166) | Nicholl et al.6 |
Baseline risks | |||
Risk of intubation | 0.029 | Beta(4.45,150) | 3CPO,18 clinical opinion |
OR for pre-hospital CPAP | |||
Mortality OR | 0.43 | Samples | NMA |
Intubation OR | 0.32 | Samples | NMA |
Life expectancy of patients | |||
Lifetime years | 2.67 years | Normal(2.67,0.16) | 3CPO,18 clinical opinion |
Health-related quality of life | |||
Utility | 0.6 | Beta(640,425) | 3CPO,18 clinical opinion |
Costs (£) | |||
Pre-hospital CPAP | 1212 | 1500 – 1000 × beta(2,5) | Clinical input |
Hospitalisation | 2250 | Gamma(75,30) | NHS reference costs89 |
Intubation | 3500 | Gamma(70,50) | NHS reference costs89 |
Annual costs | 5300 | Gamma(53,100) | NHS reference costs89 |
Methods to estimate cost-effectiveness
The cost-effectiveness of the different interventions was estimated using both the incremental cost-effectiveness ratio (ICER) and the net benefit approaches. Uncertainty was incorporated in the modelling by performing probabilistic sensitivity analysis. Descriptions of these terms and approaches are provided in the following sections: Definitions of cost-effectiveness terms, Uncertainty analysis and Value of information analysis.
Definitions of cost-effectiveness terms
The ICER measures the relative value of two strategies and is calculated as the mean incremental cost divided by the mean incremental benefits. A strategy is dominated when another strategy accrues more QALYs for less cost. Extended dominance occurs when a combination of two alternative strategies can produce the same QALYs as a chosen strategy but at a lower cost. Strategies that are neither dominated nor extendedly dominated constitute the cost-effectiveness frontier, and the ICER is reported for these strategies compared with the next least effective strategy. The willingness-to-pay threshold is the amount of money that the decision-maker is willing to pay to gain one additional QALY. The usual threshold for decision-making at NICE is considered to be around £20,000–30,000 per QALY. The net monetary benefit is defined as the QALYs multiplied by a value for the QALYs (e.g. £20,000) minus the costs of obtaining them, that is, net monetary benefit = (QALYs × λ) – cost, where λ is the willingness-to-pay threshold. The net monetary benefit approach is simpler to calculate and gives equivalent findings (but requires an explicit assumption regarding the value of λ).
Uncertainty analysis
The results presented in the following section include the effects of accounting for uncertainty in the model parameters (the costs, utilities, risks and ORs for mortality and intubation), characterised as probability distributions. Probabilistic sensitivity analysis is undertaken whereby the model is rerun (1000 times), each time with a different value for the risks, ORs, costs and utilities, which are sampled from the probability distributions. The cost-effectiveness plane shows the incremental costs (y-axis) and incremental QALYs (x-axis) compared with usual care. In this chart, if a model run for a strategy had exactly the same costs and QALYs as usual care then the ‘sample’ for that model run would appear at the origin. Samples plotted to the right of the y-axis have more QALYs than usual care and samples plotted above the x-axis have more costs. Samples plotted to the right of a straight line with slope λ passing through the origin are cost-effective, whereas those plotted to the left are not. The cost-effectiveness acceptability curve (CEAC) shows the proportion of model runs for which each strategy is cost-effective over a range of potential willingness-to-pay thresholds (i.e. λ).
Another measure of uncertainty is the overall EVPI. This calculation is carried out based on the theory that the decision-maker will choose the most cost-effective option but could acquire additional evidence to reduce the uncertainties in the decision, for example, know exactly what the HRs for mortality and hospitalisations are for each treatment. In the EVPI calculation, it can be estimated how often making the decision based on current evidence could be wrong, and also how many QALYs (and costs) would be lost by choosing the strategy that is expected to be most cost-effective given current evidence, when in fact one of the other strategies is truly the most cost-effective. The monetary value lost by making a ‘wrong’ decision to choose a strategy based on current evidence can be estimated by valuing the QALYs using the willingness-to-pay threshold for this possible loss, that is, the net benefit lost on each of the occasions when another strategy would be optimum. This can be multiplied by the number of patients per year and the expected lifetime of the decision to estimate the population EVPI.
Value of information analysis
The interpretation of population EVPI is that, if one could fund research to eliminate the uncertainty in effectiveness for all of the parameters for each strategy (e.g. by a large or infinitely large clinical trial), then the value of eliminating the uncertainty through such research would be expected to be the population EVPI. This can be thought of as the maximum that the health-care system should be willing to pay for additional evidence to inform the decision in the future and, thus, is an upper bound on the value of conducting further research, that is, if the population EVPI exceeds the expected costs of additional research then it is potentially cost-effective to conduct further research. However, EVPI, defined as the maximum investment a decision-maker would be willing to pay to eliminate all parameter uncertainty from the decision problem, has the limitation that it assumes that all information can be determined with certainty.
Expected value of partial perfect information (EVPPI) is similar to EVPI, but instead of evaluating the uncertainty associated with all parameters it focuses on the uncertainty associated with a subset of one of more parameters, allowing the decision-makers to be able to conclude in which variables further research would be most beneficial. The computational time required for EVPPI is markedly more than for EVPI as the process essentially requires two iterations of probabilistic analyses, as standard probabilistic sensitivity analyses are undertaken for each sampled parameter value for the variable(s) under analysis. If the population EVPPI for a subset of parameters exceeds the expected costs of additional research, then it is potentially cost-effective to conduct further research to estimate those parameters.
Expected value of sample information addresses the limitation that values for the parameters can be ascertained without uncertainty, which effectively assumes an infinite trial size, and seeks to provide an optimal number of patients to study within a future trial. In addition, EVSI also allows the evaluation of marginal returns with an increased sample size formally taken into account (e.g. that an additional 100 patients, when only 500 have been recruited, would be likely to provide more value than when 20,000 have been recruited). Within EVSI the costs of the trial are compared with the benefits achieved in order to find the maximum expected net benefit of sampling, which would correspond with the recommended trial size. If the population EVSI of a proposed trial is greater than the costs of the trial, then it is cost-effective to conduct the trial to address the uncertainty. EVSI, similarly to EVPPI, requires two iterations of probabilistic analyses and, additionally, the updating of prior information with the simulated results of the future trial to form a posterior distribution.
Results of the independent economic assessment
This section details the results of the cost-effectiveness analyses estimated for a single patient as mean values of 1000 probabilistic sensitivity analysis runs, each run with a different estimate for the risks, ORs, costs and utilities sampled from the probability distributions reported in Table 20. The expected estimates of cost-effectiveness and the uncertainty around them are presented, along with the probability that each of the strategies, pre-hospital CPAP and standard care, is the most cost-effective. The EVPI, a measure of how valuable it would be to eliminate all of the existing uncertainty, is also provided.
Results of the base-case scenario
The results of the NMA suggested that pre-hospital CPAP is effective in terms of reducing mortality and intubations as the mean ORs (calculated as an average of the 1000 samples provided by the NMA for input into the model) are less than one. However, pre-hospital CPAP is also more expensive than standard care with mean additional costs of around £1200 for pre-hospital CPAP. Thus, it is necessary to estimate the incremental cost-effectiveness compared with the other interventions to answer the question, ‘Is the additional effect estimated for pre-hospital CPAP worth the additional costs of the strategy?’
The QALY results suggest that the lower OR for mortality would result in an estimated QALY gain for pre-hospital CPAP over standard care of 0.099 QALYs (mean QALYs = 1.513 for pre-hospital NIV compared with 1.414 QALYs for standard care). The expected costs over a lifetime also differ, with pre-hospital CPAP having higher costs (£16,895) than usual care (£14,863). The majority of this cost difference of £2032 was a result of the difference in the costs of treatment, that is, the cost of pre-hospital CPAP and the higher long-term costs, which were dependent on the number of people alive and their annual costs.
To assess whether or not the additional costs are worthwhile, the incremental cost per QALY gained is estimated. Comparing pre-hospital CPAP with standard care, the incremental cost per QALY gained is £2032/0.076 = £20,514 per QALY, which is just above the typical NICE threshold of £20,000 per QALY gained. Another way to present these results is to calculate the net monetary benefit of each strategy. The net monetary benefit of pre-hospital CPAP is (1.513 × £20,000) – £16,895 = £13,365. This approach takes away the need to calculate the ICER and simplifies the interpretation for decision-makers as the strategy with the highest expected incremental net monetary benefit is the most cost-effective. Using a threshold value of £20,000 per QALY, the estimated incremental net monetary benefit of pre-hospital CPAP compared with standard care is estimated to be £13,365 – £13,419 = –£54. Mathematically, as this difference is negative (i.e. < 0), the ICER must be > £20,000 (the ICER of pre-hospital CPAP compared with standard care is £20,514 per QALY).
As the model is rerun 1000 times, each time with a different value for the OR, costs and utilities sampled from the probability distribution, in some of the sampled model runs standard care could be more effective than pre-hospital CPAP because of the uncertainty in the probability distributions of ORs. In the cost-effectiveness plane shown in Figure 8, the samples to the right of the diagonal line through the origin would have an incremental cost per QALY compared with usual care of < £20,000 and so would be considered cost-effective compared with usual care. Figure 8 shows that the samples fall almost equally on either side of the diagonal line, suggesting that there is uncertainty in stating that pre-hospital CPAP has a chance of being cost-effective compared with standard care. The uncertainty in costs shown in Figure 8 is actually a function of the uncertainty in the mortality ORs (more or less time alive, during which there is a cost per year). The mean ICER, presented as the blue triangle in cost-effectiveness plane of Figure 8, is just above the £20,000 per QALY threshold line which is in line with our estimated mean ICER of £20,514 per QALY.
FIGURE 8.
Cost-effectiveness plane for the base-case economic analysis.
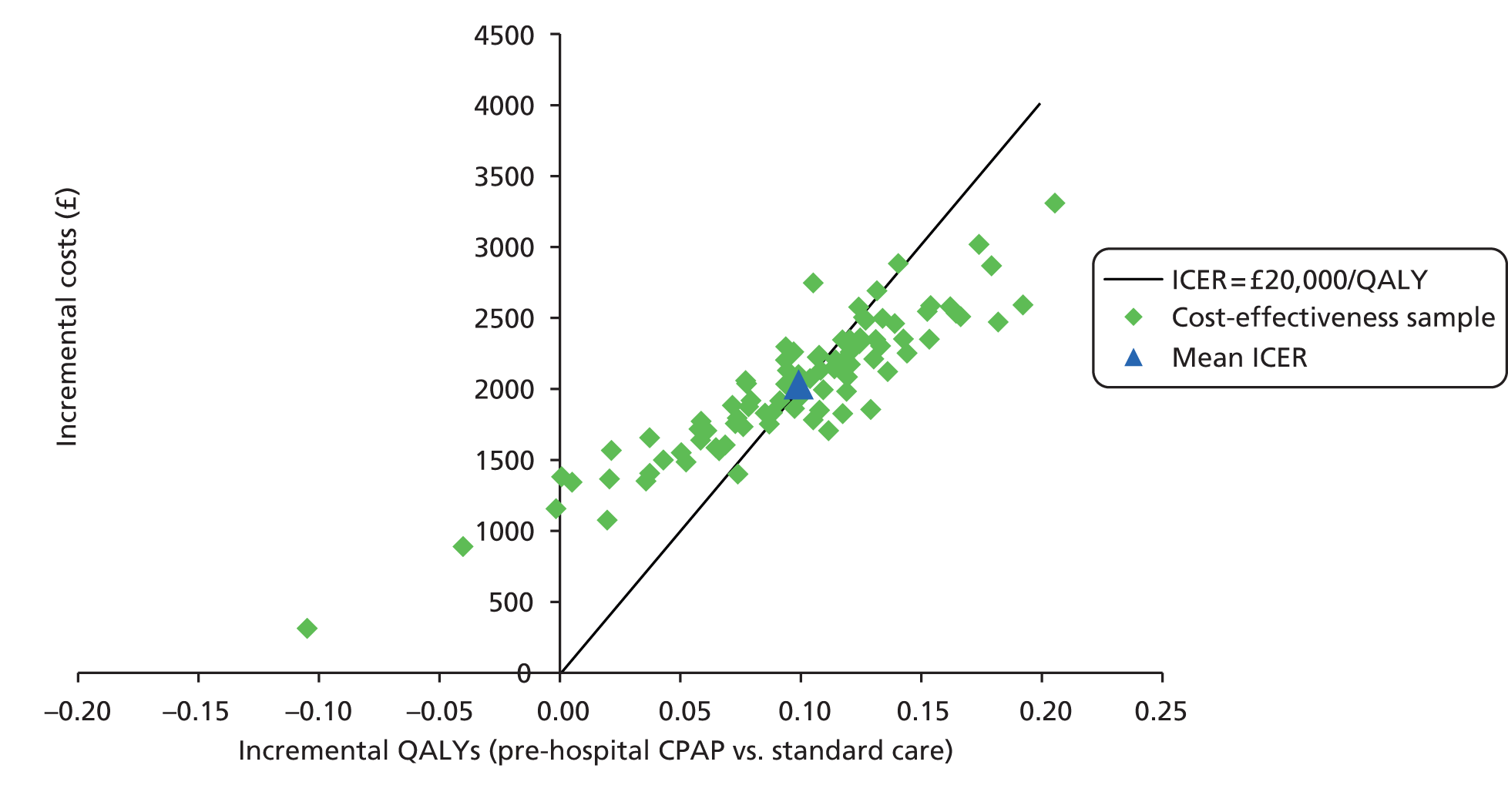
The CEAC in Figure 9 shows the proportion of model runs for which each strategy is cost-effective over a range of potential willingness-to-pay thresholds. The percentage of model runs in which pre-hospital CPAP was the most cost-effective strategy (at a £20,000 per QALY threshold) was 49.5%, with the percentage of model runs in which usual care was the most cost-effective being the rest, 50.5%. This was also observed in Figure 8, where the samples fall almost equally on either side of the diagonal line, that is, there is approximately a 50% chance of being cost-effective at a threshold of £20,000 per QALY. A CEAC in which the best strategy is cost-effective only half of the time indicates that there is uncertainty as to which strategy is optimum in terms of net benefit.
FIGURE 9.
Cost-effectiveness acceptability curve for the base-case economic analysis.

Results for different geographical scenarios
The results seen in the base-case scenario are for the UK general population scenario and reflect the cost-effectiveness of different strategies at the national level, that is, the average distance to hospital and the distribution of distance to hospital are those of the general population in the UK. However, the distance to hospital is greater, on average, for patients in a rural setting (e.g. South West Ambulance Service) than in an urban setting (e.g. West Midlands Ambulance Service) and, to this end, we tested the model in two other scenarios:
-
Rural population scenario: the average distance to hospital and the distribution of distance to hospital are those of a typical rural setting in the UK. This scenario reflects the cost-effectiveness of different strategies at the rural level; thus, the services in rural areas are able to decide if this scenario and the results best reflect their local practice.
-
Urban population scenario: the average distance to hospital and the distribution of distance to hospital are those of a typical urban setting in the UK. This scenario reflects the cost-effectiveness of different strategies at the urban level; thus, the services in urban areas are able to decide if this scenario and the results best reflect their local practice.
The mortality rates used for the different scenarios are shown in Table 15. This approach was taken because it is possible that different strategies may have different levels of cost-effectiveness in different settings. The decision to commission a pre-hospital CPAP service is typically made at the ambulance service level and, thus, the users of the results are able to decide which scenario best reflects their local practice and if pre-hospital CPAP is a cost-effective use of resources in their setting. A summary of the results for the two scenarios, compared with the base-case scenario, is presented in Table 21.
Scenario | Average usual care | Average pre-hospital CPAP | Pre-hospital CPAP vs. standard care | ICER (£) | Probability of being cost-effective | |||
---|---|---|---|---|---|---|---|---|
Total discounted costs (£) | Total discounted QALYs | Total discounted costs (£) | Total discounted QALYs | Difference in costs (£) | Difference in QALYs | |||
General population scenario | 14,863 | 1.414 | 16,895 | 1.513 | 2032 | 0.099 | 20,514 | 0.495 |
Rural population scenario | 14,540 | 1.377 | 16,724 | 1.494 | 2184 | 0.117 | 18,744 | 0.588 |
Urban population scenario | 14,971 | 1.427 | 16,950 | 1.520 | 1979 | 0.093 | 21,284 | 0.415 |
It can be seen that the cost-effectiveness of pre-hospital CPAP is different in urban and rural areas, that is, pre-hospital CPAP in a rural setting is more cost-effective as the average distance to hospital is high and pre-hospital CPAP can help save more lives but is not as cost-effective in an urban setting where the mortality is low as the distance to hospital is not high. The results are presented in more detail in the following sections Results of rural scenario and Results of urban scenario.
Results of rural scenario
The QALY results suggest that the higher baseline mortality in the rural setting combined with the same relative effectiveness of pre-hospital CPAP would result in a higher estimated QALY gain for pre-hospital CPAP over standard care of 0.117 QALYs (mean QALYs = 1.494 for pre-hospital CPAP compared with 1.377 QALYs for standard care). This is because of the higher baseline mortality risk in the rural scenario, which, combined with same mortality ORs of pre-hospital CPAP, results in more lives saved, which leads to more QALYs gained compared with the base-case scenario. The expected costs over a lifetime also differ, with pre-hospital CPAP having higher costs (£16,724) than usual care (£14,540). Comparing pre-hospital CPAP with standard care, the incremental cost per QALY gained is £2184/0.117 = £18,744 per QALY, which is below the typical NICE threshold of £20,000–30,000 per QALY gained.
This can also be observed in the mean ICER, presented as a blue triangle in the cost-effectiveness plane of Figure 10, as it is just below the £20,000 per QALY threshold line (which is in line with our estimated mean ICER of £18,744 per QALY). The cost-effectiveness plane in Figure 10 shows that the majority of the samples fall to the right of the diagonal line, suggesting that pre-hospital CPAP has a higher chance of being cost-effective than usual care.
FIGURE 10.
Cost-effectiveness plane for the rural population scenario.

The CEAC in Figure 11 shows the proportion of model runs for which each strategy is cost-effective over a range of potential willingness-to-pay thresholds. The percentage of model runs in which pre-hospital CPAP was the most cost-effective strategy (at a £20,000 per QALY threshold) was 58.8%, which again indicates less uncertainty as to which strategy is optimum in terms of net benefit.
FIGURE 11.
Cost-effectiveness acceptability curve for the rural population scenario.
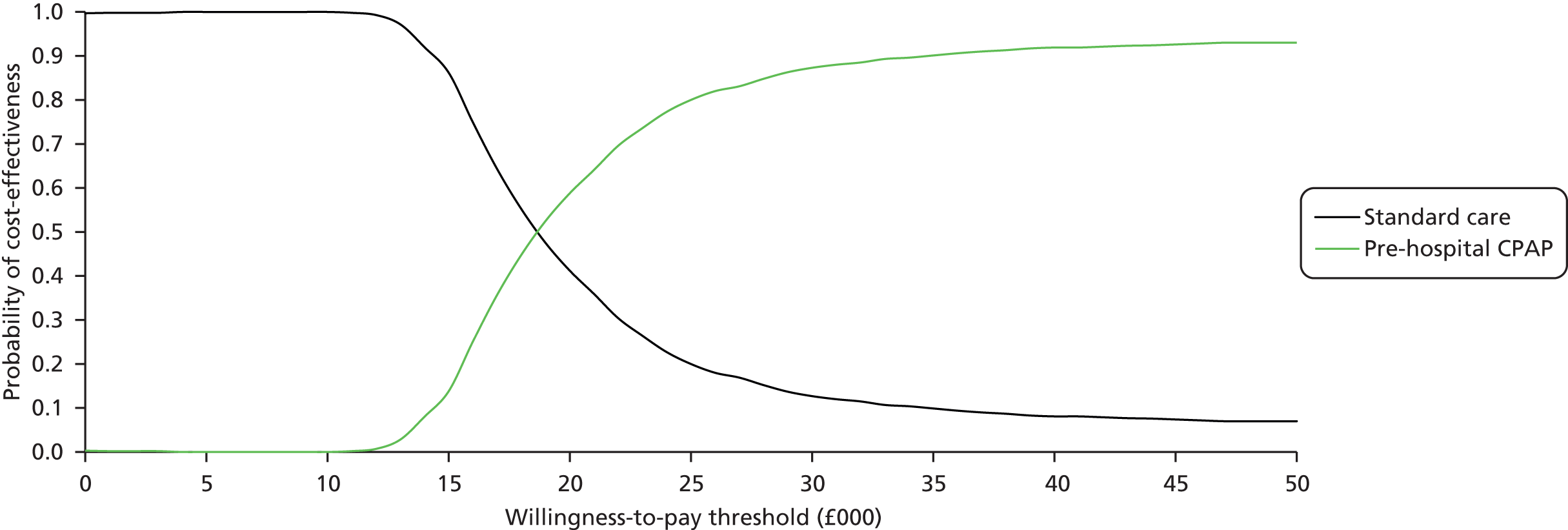
Results of urban scenario
The QALY results suggest that the higher baseline mortality in the urban setting, combined with the same relative effectiveness of pre-hospital CPAP, would result in a lower estimated QALY gain for pre-hospital CPAP over standard care of 0.093 QALYs (mean QALYs = 1.520 for pre-hospital NIV, compared with 1.427 QALYs for standard care). This is because of the lower baseline mortality risk in the urban scenario, which, combined with same mortality ORs of pre-hospital CPAP, results in fewer lives saved, which leads to fewer QALYs gained. The expected costs over a lifetime also differ, with pre-hospital CPAP having higher costs (£16,950) than usual care (£14,971). Comparing pre-hospital CPAP with standard care, the incremental cost per QALY gained is £1979/0.093 = £21,284 per QALY, which is above the typical NICE threshold of £20,000 per QALY gained.
This can also be observed in the mean ICER, presented as a blue triangle in the cost-effectiveness plane of Figure 12, as it is above the £20,000 per QALY threshold line (in line with our estimated mean ICER of £21,284 per QALY). The cost-effectiveness plane in Figure 12, shows that only a minority of the samples fall to the right of the diagonal line, suggesting that the chance of pre-hospital CPAP being cost-effective compared with usual care is lower than in the base-case scenario.
FIGURE 12.
Cost-effectiveness plane for the urban population scenario.

The CEAC in Figure 13 shows the proportion of model runs for which each strategy is cost-effective over a range of potential willingness-to-pay thresholds. The percentage of model runs in which pre-hospital CPAP was the most cost-effective strategy (at a £20,000 per QALY threshold) was 41.5%, which indicates greater uncertainty as to which strategy is optimum in terms of net benefit.
FIGURE 13.
Cost-effectiveness acceptability curve for the urban population scenario.

Results for different cost scenarios
Scenario analysis was also conducted for three different estimates of the unit (per patient) cost of performing pre-hospital CPAP (for different proportions of the eligible population): a high-cost scenario with a unit cost of £1400, a low-cost scenario with a unit cost of £745 and a lower-cost scenario with a unit cost of £300. These estimates relate to the capability of the ambulance services to deliver the pre-hospital CPAP service at these costs and the users of the results are able to decide which scenario best reflects their local practice and if pre-hospital CPAP is a cost-effective use of resources in their setting. A summary of the results for the three scenarios, compared with the base-case scenario, is presented in Table 22.
Scenario | Average usual care | Average pre-hospital CPAP | Pre-hospital CPAP vs. standard care | ICER (£) | Probability of being cost-effective | |||
---|---|---|---|---|---|---|---|---|
Total discounted costs (£) | Total discounted QALYs | Total discounted costs (£) | Total discounted QALYs | Difference in costs (£) | Difference in QALYs | |||
Base-case scenario | 14,863 | 1.414 | 16,895 | 1.513 | 2032 | 0.099 | 20,514 | 0.495 |
High-cost scenario, £1400 | 14,863 | 1.414 | 17,078 | 1.513 | 2216 | 0.099 | 22,368 | 0.354 |
Low-cost scenario, £745 | 14,863 | 1.414 | 16,421 | 1.513 | 1558 | 0.099 | 15,728 | 0.798 |
Lower-cost scenario, £300 | 14,863 | 1.414 | 15,977 | 1.513 | 1114 | 0.099 | 11,248 | 0.938 |
As expected, the cost-effectiveness of pre-hospital CPAP improves as the unit costs become lower. This is because the QALY gain for pre-hospital CPAP over standard care of 0.099 QALYs (mean QALYs = 1.513 for pre-hospital CPAP compared with 1.414 QALYs for standard care) remains the same while the expected costs over a lifetime go down (as a result of the lower costs of pre-hospital CPAP).
Results of high-cost scenario
The expected costs over a lifetime are higher in this scenario, with the cost of pre-hospital CPAP being higher, at £17,078, than that of standard care (£14,863), a cost difference of £2215. This is higher than the incremental costs in the base-case scenario, where the cost difference is £2032 (pre-hospital CPAP costs of £16,895, compared with a usual care cost of £14,863). This variation is due to the difference in the costs of pre-hospital CPAP in the high-cost scenario and the base-case scenario. In this high-cost scenario, the incremental cost per QALY gained is £2216/0.099 = £22,368 per QALY, which is above the typical NICE threshold of £20,000 per QALY gained, as shown by the blue triangle in the cost-effectiveness plane in Figure 14. The cost-effectiveness plane in Figure 14 shows that the only a minority of the samples fall to the right of the diagonal line, suggesting that pre-hospital CPAP has a low chance of being cost-effective compared with usual care.
FIGURE 14.
Cost-effectiveness plane for the high-cost scenario.

The CEAC in Figure 15 shows the proportion of model runs for which each strategy is cost-effective over a range of potential willingness-to-pay thresholds. The percentage of model runs in which pre-hospital CPAP was the most cost-effective strategy (at a £20,000 per QALY threshold) was 35.4%, which indicates increased uncertainty that pre-hospital CPAP is optimum in terms of net benefit. This was also observed in Figure 14, where most of the samples fall on left side of the diagonal line, that is, there is only a one-third chance of pre-hospital CPAP being cost-effective at a threshold of £20,000 per QALY.
FIGURE 15.
Cost-effectiveness acceptability curve for the high-cost scenario.

Results of low-cost scenario
The expected costs over a lifetime are lower than in the base-case scenario, with the cost of pre-hospital CPAP being £16,421, compared with £14,863 for usual care and, comparing pre-hospital CPAP with standard care, the incremental cost per QALY gained is £1558/0.099 = £15,728 per QALY, which is below the typical NICE threshold of £20,000 per QALY gained, suggesting that it is cost-effective. The cost-effectiveness plane for the high-cost scenario is shown in Figure 16.
FIGURE 16.
Cost-effectiveness plane for the low-cost scenario.
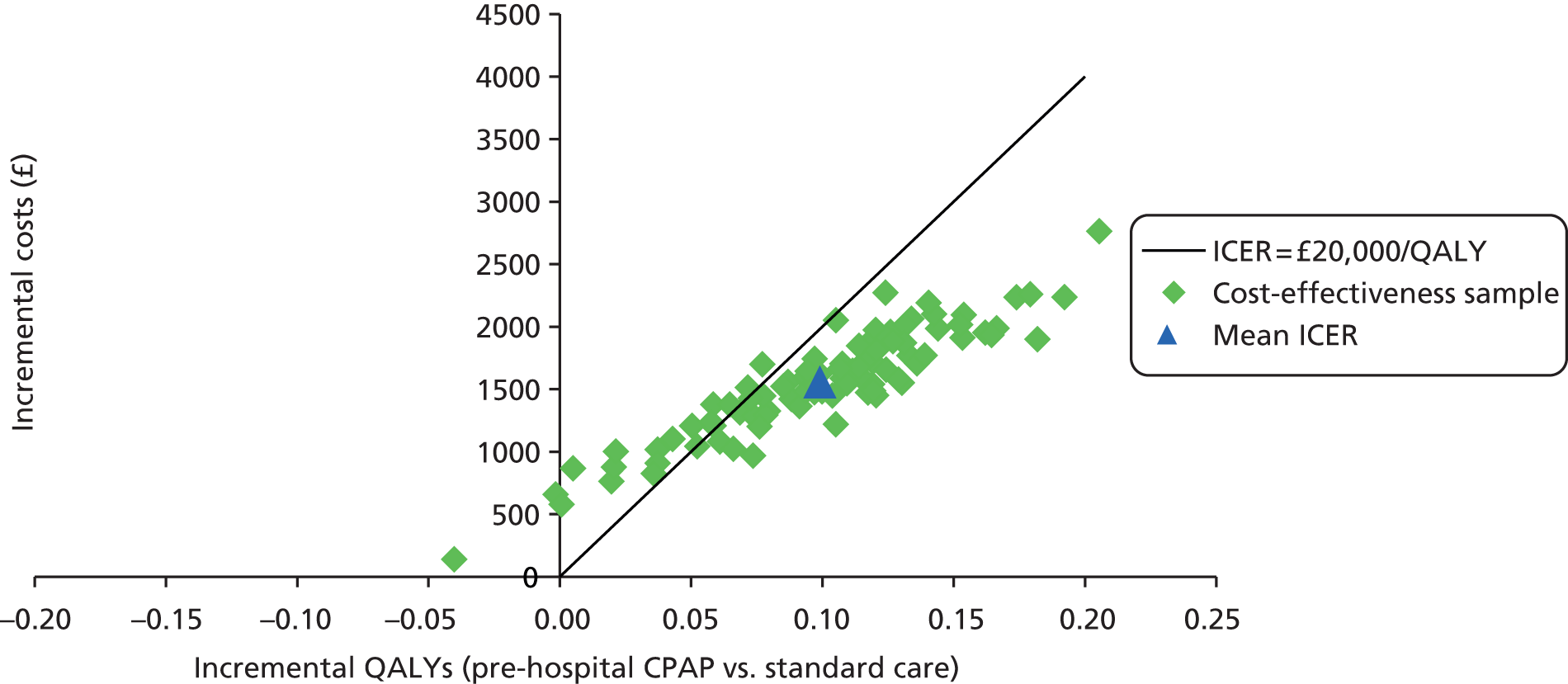
The CEAC in Figure 17 shows the proportion of model runs for which each strategy is cost-effective over a range of potential willingness-to-pay thresholds. The percentage of model runs in which pre-hospital CPAP was the most cost-effective strategy (at a £20,000 per QALY threshold) was 79.8%, which indicates greater confidence to state that pre-hospital CPAP is optimum in terms of net benefit.
FIGURE 17.
Cost-effectiveness acceptability curve for the low-cost scenario.
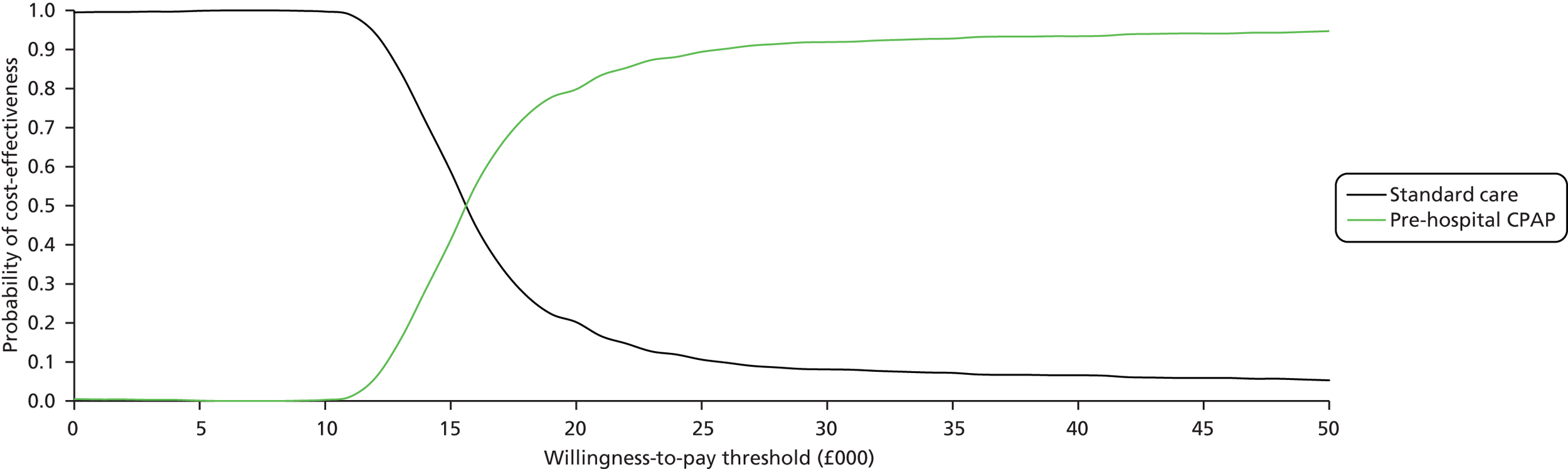
Results of lower-cost scenario
The expected costs over a lifetime for this scenario are even lower, with the costs of pre-hospital CPAP being £15,977, compared with £14,863 for standard care, that is, pre-hospital CPAP incurs additional costs of £1114 compared with standard care. This is much lower than the incremental cost in the base-case scenario, which is £2032 (pre-hospital CPAP costs of £16,895 compared with usual care costs of £14,863), and this variation is a result of the lower costs of pre-hospital CPAP in this scenario than in the base-case scenario.
The incremental cost per QALY gained is £1114/0.099 = £11,248 per QALY, which is much lower than the typical NICE threshold of £20,000 per QALY gained, suggesting that pre-hospital CPAP is cost-effective, as shown in the cost-effectiveness plane in Figure 18. The cost-effectiveness plane in Figure 18 shows that the majority of the samples fall to the right of the diagonal line, suggesting that pre-hospital CPAP has a high chance of being cost-effective compared with usual care.
FIGURE 18.
Cost-effectiveness plane for the lower-cost scenario.

The CEAC in Figure 19 shows the proportion of model runs for which each strategy is cost-effective over a range of potential willingness-to-pay thresholds. The percentage of model runs in which pre-hospital CPAP was the most cost-effective strategy (at a £20,000 per QALY threshold) was 93.8%, which indicates a much greater confidence to state that pre-hospital CPAP is optimum in terms of net benefit.
FIGURE 19.
Cost-effectiveness acceptability curve for the lower-cost scenario.
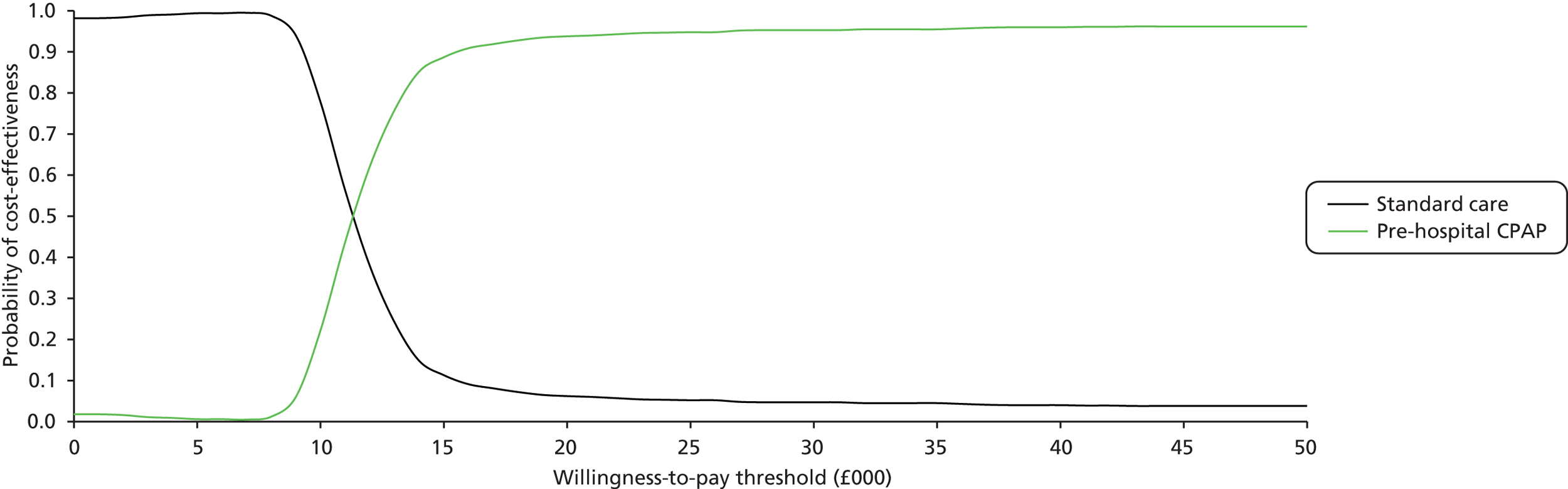
Costs and benefits for a typical NHS ambulance service
To present the results of the economic analysis in a way that may be more meaningful for decision-makers, we estimated the annual additional costs and lives saved that would be expected across a typical ambulance service if pre-hospital CPAP were implemented. These estimates were highly dependent on the estimated incidence of patients likely to benefit from pre-hospital CPAP, so they are presented in Table 23 according to the annual number of eligible patients expected by an ambulance service covering a population of 5 million.
Annual number of eligible patients | Annual cost of service (£) | Annual number of deaths with standard care | Annual number of deaths with pre-hospital CPAP | Annual number of lives saved |
---|---|---|---|---|
175 | 235,683 | 20.79 | 9.98 | 10.81 |
365 | 271,772 | 43.37 | 20.82 | 22.54 |
890 | 371,486 | 105.75 | 50.78 | 54.97 |
1700 | 525,334 | 201.99 | 96.99 | 105.00 |
1800 | 544,320 | 213.87 | 102.70 | 111.17 |
2000 | 582,300 | 237.63 | 114.11 | 123.52 |
The annual costs and lives saved obviously vary according to the number of eligible patients, but if a typical ambulance service treated 175 appropriate patients per year it could save 10.81 lives while incurring £235,683 additional costs, whereas if a typical ambulance service treated 2000 appropriate patients per year it could save 123.52 lives while incurring £582,300 additional costs.
Value of information analyses
The uncertainty in the base-case analysis can also be measured as the overall EVPI, which is the average of the net benefits lost by making the decision to choose pre-hospital CPAP. The individual patient EVPI for the base-case model is illustrated in Figure 20. At low thresholds for cost-effectiveness, additional information is unlikely to change that decision. The EVPI reaches maximum when there is most uncertainty about whether to adopt or reject the technology based on existing evidence, that is, at a threshold of around £20,000 per QALY. The EVPI for the base-case analysis at a threshold of £20,000 per QALY is £184 per patient for whom the decision is made. EVPI for the whole population can be estimated as EVPI per patient multiplied by the number of patients affected by the decision over the lifetime of the technology, that is, multiplying the EVPI by incidence over the lifetime of the technology. However, as reported in the previous section, Costs of pre-hospital continuous positive airway pressure, there is uncertainty regarding incidence as different estimates of incidence are reported in different sources. Thus, population EVPI was estimated at higher and lower values of incidence to reflect this uncertainty. The lifetime of the technology was assumed to be 5 years and the total population of England and Wales to be 60 million.
FIGURE 20.
Expected value of perfect information for the economic analysis.

Assuming an annual incidence of 3.5 per 100,000 population, the number of respiratory failure patients eligible for pre-hospital CPAP in England and Wales can be estimated as 2100 and, with a lifetime of 5 years for the technology, the population EVPI at the threshold of £20,000 per QALY is £184 × 2100 × 5 = £1.9M. If an annual incidence of 40.8 per 100,000 is assumed, then the population EVPI is £22.5M.
Expected value of partial perfect information
Partial EVPI provides the value of reducing the uncertainty surrounding particular input parameters in the decision model, and this can be used to identify the parameters for which more precise estimates would be most valuable to focus further research.
The EVPPIs associated with the parameters are illustrated in Figure 21. At the threshold of £20,000 per QALY, EVPPIs associated with ‘baseline mortality’, ‘pre-hospital CPAP mortality effectiveness’ and ‘total costs of pre-hospital CPAP’ are £14.85, £156.12 and £37.54, respectively. Other parameters do not come out of the analysis as substantial, as shown in Figure 21. In addition, the combined EVPPI for the three parameters (i.e. ‘baseline mortality’, ‘pre-hospital CPAP mortality effectiveness’ and ‘total costs of pre-hospital CPAP’) is £174.61, which is close to the overall EVPI; this suggests that most of the uncertainty in the model is due to the uncertainty in these three parameters.
FIGURE 21.
Individual patient EVPPI at £20,000 per QALY.

The high EVPPIs associated with the three parameters above suggest that further experimental research to estimate these parameters will potentially be cost-effective. EVPPI for the whole population can be estimated as EVPPI per patient multiplied by the number of patients affected by the decision over the lifetime of the technology. Assuming an annual incidence of 3.5 per 100,000 population, the undiscounted population EVPPI for the three parameters (i.e. ‘baseline mortality’, ‘pre-hospital CPAP mortality effectiveness’ and ‘total costs of pre-hospital CPAP’) at the threshold of £20,000 per QALY is £180 × 2100 × 5 = £1.83M. If an annual incidence of 40.8 per 100,000 is assumed, then the population EVPPI is £21.3M.
Expected value of sample information
Expected value of sample information seeks to provide an optimal number of patients to study within a future trial by comparing the costs of the trial with the benefits achieved in order to find the recommended trial size. For any given set of parameters, EVSI values are always lower than partial EVPPI values and hence EVSI analysis was conducted only for ‘baseline mortality’, ‘pre-hospital CPAP mortality effectiveness’ and ‘total costs of pre-hospital CPAP’, as the EVPPI values for other parameters are not significant (i.e. further experimental research to estimate other parameters may not be cost-effective). The EVSI values were estimated for a RCT conducted to estimate the ‘baseline mortality’ and ‘pre-hospital CPAP mortality effectiveness’ and the analysis was carried out for different sample sizes in order to estimate the optimal trial size.
It should also be noted that the trial has benefits other than EVSI as, for example, it also allows the estimation of the incidence of cases eligible for pre-hospital NIV, the costs of setting up and running the service, and determining whether or not a large trial would be feasible. This suggests that the EVSI will be higher than that reported in Table 24. If we assume that the trial will also address the uncertainty in the cost of pre-hospital CPAP, then an additional EVSI of £35.56 per patient can be added on top of the original EVSI.
Trial identifier | Number per arm | EVSI per patient | Population EVSI: low estimate (£) | Population EVSI: high estimate (£) |
---|---|---|---|---|
1 | 10 | 31.30 | 328,669 | 3,831,336 |
2 | 30 | 67.91 | 713,106 | 8,312,777 |
3 | 50 | 83.28 | 874,454 | 10,193,636 |
4 | 100 | 103.52 | 1,086,960 | 12,670,848 |
5 | 150 | 114.53 | 1,202,602 | 14,018,903 |
6 | 250 | 126.56 | 1,328,855 | 15,490,654 |
7 | 350 | 133.30 | 1,399,688 | 16,316,362 |
8 | 650 | 142.95 | 1,500,975 | 17,497,080 |
9 | 1000 | 148.25 | 1,556,625 | 18,145,800 |
10 | 2000 | 154.12 | 1,618,260 | 18,864,288 |
If the population EVSI of a proposed trial at a given sample size is greater than the costs of the trial, then it is cost-effective to conduct the trial to address the uncertainty. For example, it is cost-effective to do a trial with 100 patients in each arm (trial 4) if the overall cost is less than £1.08M or £12.67M, depending on which source of incidence is more believable. If an incidence of 40 patients per 100,000 population is to be believed, then there is a clear argument for conducting a trial, as the expected benefits of the trial outweigh the costs of conducting such a trial.
Chapter 5 Discussion
Statement of principal findings
Clinical effectiveness review
Pre-hospital CPAP appears to be an effective treatment for acute respiratory failure, with evidence that it reduces mortality (OR 0.41, 95% CrI 0.20 to 0.77) and intubation rate (OR 0.32, 95% CrI 0.17 to 0.62) compared with standard care. The effectiveness of pre-hospital BiPAP is uncertain, with estimates of the effect on mortality (OR 1.94, 95% CrI 0.65 to 6.14) and intubation rate (OR 0.40, 95% CrI 0.14 to 1.16) including the possibility of either worthwhile benefit or considerable harm. These findings suggest that pre-hospital CPAP may have a beneficial role in reducing mortality and intubation rates in acute respiratory failure, whereas there is currently insufficient evidence to determine the role of pre-hospital BiPAP.
The NMA using both IPD and aggregate data suggested that male sex was a significant treatment effect modifier of mortality, with CPAP being more effective in males. The pathological basis of these findings is not clear, so they should be interpreted with caution. We found no such associations in the analysis of intubation data.
Economic evaluation
The economic analysis showed that pre-hospital CPAP was more effective than standard care, with 0.099 QALYs gained per patient treated, but was more expensive, with an additional cost of £2032 per patient treated. The ICER for pre-hospital CPAP was £20,514 per QALY compared with standard care, with 49.5% probability of being cost-effective at the £20,000 per QALY threshold. These findings suggest that, even if the apparent effectiveness of pre-hospital CPAP suggested by our meta-analysis were confirmed, it is uncertain whether or not widespread implementation of pre-hospital CPAP would represent a worthwhile use of NHS resources.
Sensitivity analysis showed that, compared with the general population scenario, pre-hospital CPAP was more likely to be cost-effective in an ambulance service covering a rural population (ICER £18,744 per QALY, 58.8% probability of being cost-effective at the £20,000 per QALY threshold) and less likely to be cost-effective in an ambulance service covering an urban population (ICER £21,284 per QALY, 41.5% probability). So, although pre-hospital CPAP is most likely to be cost-effective in an ambulance service covering a rural population, there is substantial uncertainty regarding cost-effectiveness in each scenario.
While developing the economic model, we identified marked variation between estimates from different sources of the incidence of patients likely to benefit from pre-hospital CPAP. Sensitivity analysis also showed that this parameter was an important determinant of cost-effectiveness. The lower estimate of incidence resulted in a higher cost per patient (£1400), a high ICER (£22,368 per QALY) and a low probability of being cost-effective (35.4% at the £20,000 per QALY threshold). The higher estimate of incidence resulted in a lower cost per patient (£300), a lower ICER (£11,248 per QALY) and a high probability of being cost-effective (93.8% at the £20,000 per QALY threshold). Our analysis suggested that if a typical ambulance service treated 175 appropriate patients per year it could save 10.81 lives while incurring £235,683 additional costs, whereas if a typical ambulance service treated 2000 appropriate patients per year it could save 123.52 lives while incurring £582,300 additional costs.
The incidence of appropriate patients was also an important determinant of the expected value of information. The population EVPI is £1.9M at a low estimate of incidence and £22.5M at a higher incidence. EVPPI analysis suggested that ‘pre-hospital CPAP mortality effectiveness’, ‘total costs of pre-hospital CPAP’ and ‘baseline mortality’ are the key parameters with EVPPI values of £156.12, £37.54 and £14.85 per patient, respectively. Population EVPPI for the three parameters together at the threshold is estimated as £1.83M at low incidence and £21.3M at a higher incidence. Similarly, population EVSI value for a RCT with 100 patients in each arm to estimate ‘baseline mortality’ and ‘pre-hospital CPAP mortality effectiveness’ is estimated as £1.08M at low incidence and £12.67M at a higher incidence. If the population EVSI of a proposed trial at a given sample size is greater than the costs of the trial, then it is cost-effective to conduct the trial to address the uncertainty. A trial of pre-hospital CPAP would probably cost between £1.08M and £12.67M, so the value of undertaking a trial would depend on the anticipated incidence of appropriate patients. Feasibility of a trial would also, logically, depend on this parameter, so it appears that a reliable estimate of the incidence of eligible patients is an essential prerequisite to further randomised evaluation.
Strengths and limitations of the assessment
Clinical effectiveness review
Four previous systematic reviews35,36,38,90 have evaluated the role of pre-hospital NIV in treating ACPO or acute respiratory failure, but only one of these undertook a meta-analysis. 90 Williams et al. 90 evaluated the effectiveness of pre-hospital CPAP for acute respiratory failure and identified three randomised trials,47,48,53 a non-randomised comparative study74 and a retrospective comparative study. 67 The three randomised trials47,48,53 were all included in our meta-analysis. The two non-randomised studies67,74 were identified and included in our description of non-randomised studies but not included in our meta-analysis. We included two additional recent randomised trials in our meta-analysis. 46,52 We also included a trial of early versus late pre-hospital CPAP50 in our main analysis but excluded it from our sensitivity analysis. This trial was excluded by Williams et al. 90
The meta-analysis undertaken by Williams et al. 90 included randomised and non-randomised studies and reported that CPAP was associated with lower mortality (OR 0.41, 95% CI 0.19 to 0.87) and fewer intubations (OR 0.31, 95% CI 0.19 to 0.51). These ORs are very similar to ours (mortality OR 0.41, 95% CrI 0.20 to 0.77; intubation rate OR 0.32, 95% CrI 0.17 to 0.62), suggesting that the results are driven by the three trials47,48,53 that were included in both analyses, which together contributed 400 patients. The inclusion of non-randomised studies in the meta-analysis by Williams et al. 90 may be inappropriate, given their high risk of bias. Our decision to include quasi-randomised trials54,55 could also be criticised for similar reasons. Meanwhile, our decision to include the trial by Plaisance et al. 50 could be questioned, given that the control group in this trial received delayed pre-hospital NIV. However, our sensitivity analysis with these studies excluded produced very similar results to the main analysis.
Our review was more comprehensive than previous reviews and, by excluding non-randomised trials from meta-analysis, carried a lower risk of bias. Non-randomised trials were reported to describe outcomes only when pre-hospital NIV is used in non-trial settings. Although we are confident that we have identified and included all existing randomised trials, it is possible that unregistered trials exist and have not been reported. The validity of our findings is principally dependent on the validity of the primary data. The included studies were of reasonably high quality, the main threats to validity were the lack of blinding of outcome measurement and lack of adequate sample size. Lack of blinding is unlikely to have influenced mortality, but could have influenced intubation rates. A clinician might be more eager to initiate intubation in a patient is receiving no ventilator support than in one with similar respiratory parameters who is receiving NIV. However, from a pragmatic perspective, this apparent bias could be seen as part of the effect of NIV. If the provision of NIV results in less use of intubation then, pragmatically, it does not matter whether this is achieved by improving respiratory function or by encouraging the clinician to withhold intubation while medical treatment takes effect.
Meta-analysis is intended to overcome the lack of statistical power to detect potentially worthwhile differences in mortality and intubation rates in the primary studies. However, this is inevitably still limited by the sample sizes of the primary studies. We therefore cannot conclude that BiPAP is ineffective and the wide CIs suggest that we should not pay too much attention to the apparent direction of effect on mortality, with BiPAP appearing to increase mortality.
It is possible that the control groups in the trials did not all receive best alternative care and that this may have inflated the potential benefit of pre-hospital NIV. In-hospital NIV is widely available in most developed health services and one would expect that any patient eligible for pre-hospital NIV would receive in-hospital NIV if treatment were not available pre hospital. However, only one trial mandated the use of in-hospital NIV, while one prohibited its use, three allowed its use and five did not record this information.
Our analysis is also limited by a lack of generalisability, particularly to the UK. The trials were generally small (n = 23–207) and might represent selected patient groups. However, the trial reported mortality and intubation rates that were similar to those in non-randomised studies, while uncontrolled studies of pre-hospital NIV reported intubation and mortality rates that were, if anything, higher. This suggests that trials were not selecting patients at a higher risk of adverse outcome compared with non-randomised and observational studies.
None of the trials was undertaken in the UK. Pre-hospital systems vary substantially between countries and the trial settings were often very different from the UK. Pre-hospital NIV in the UK would probably be provided by paramedics working independently (i.e. without online medical control), whereas in the trials pre-hospital NIV was provided by specialist units which included physicians in six trials,47,48,50–52,55 paramedics in three49,53,54 and the provider was not reported in one. 46 Only two of the trials51,52 recruited patients with undifferentiated acute respiratory distress, while six46–48,50,54,55 recruited patients with presumed ACPO. It is not clear how ACPO would have been reliably diagnosed in the pre-hospital setting, although physicians may have more training and experience, to allow them to make this judgement, than paramedics. Finally, additional monitoring technology such as near-patient arterial blood gas analysis, which is not available to UK ambulance services, was used in five studies. 47,28,50,51,55
Generalising the findings from small trials of selected patients to routine practice can be potentially misleading. Meta-analysis of small studies of in-hospital NIV for ACPO22,23 concluded that treatment was effective and likely to reduce mortality and intubation rates. However, a subsequent large pragmatic trial of in-hospital NIV for ACPO18 found only modest improvements in breathlessness and acidosis, with no significant effect on mortality and intubation rates.
The combined IPD and aggregate data meta-analysis allowed an investigation of the potential for patient-level characteristics to be treatment effect modifiers. The use of IPD makes use of data from all patients rather than resorting to a meta-regression of aggregate data based on a limited number of studies.
Individual patient-level data were not available from all studies and we used a combination of IPD and aggregate data. The combined IPD and aggregate data meta-analyses were conducted separately for each potential treatment effect modifier. Ideally, we would incorporate all covariates into a single model but this was not possible because some studies did not provide data on all covariates. It is a limitation of the combined IPD and aggregate data meta-analyses that were conducted that the studies included in the analyses depended on the availability of data on each covariate in each study.
Economic evaluation
We identified only one previous economic evaluation80 of pre-hospital NIV for patients with acute respiratory failure that fulfilled our inclusion criteria. As described in Chapter 4, Cost-effectiveness review summary, this study had a number of limitations that prevented reliable conclusions being drawn regarding the cost-effectiveness of pre-hospital NIV in the NHS. It used in-hospital effectiveness data rather than pre-hospital data; outcomes were valued as lives saved rather than QALYs; the setting was the US health-care system and US cost estimates were used; the model only used a 1-year time horizon and probabilistic sensitivity analysis was not performed. We therefore undertook an analysis that addressed all these limitations.
Our analysis took the economic perspective of the NHS in England and Wales, was based on effectiveness estimates from our meta-analysis of pre-hospital CPAP for acute respiratory failure, valued outcomes as QALYs, used a lifetime horizon and included probabilistic sensitivity analysis. Other strengths included detailed costing at the level of the ambulance service and then estimated on a per-patient basis using a range of estimates for the incidence of eligible patients; use of relevant existing data sources to estimate key population, cost and outcome parameters; and scenario analysis involving urban and rural ambulance services.
Despite these strengths, our analysis had some limitations. As previously discussed, the estimates of effectiveness of pre-hospital NIV were derived from our meta-analysis of small trials, involving potentially selected study populations, that might not have compared pre-hospital CPAP to best alternative care and were undertaken in settings that differ markedly from the NHS. Meta-analysis suggested that pre-hospital CPAP reduces mortality and intubation rates, but if these findings are not reproduced in the NHS then pre-hospital CPAP will not be cost-effective.
The cost per patient of providing pre-hospital CPAP is calculated by dividing the total cost of setting up and running the service by the total number of patients treated. This means that the cost per patient is determined by the incidence of patients who are likely to benefit from pre-hospital CPAP. We identified a number of different sources for our estimate of this parameter but these estimates varied markedly. Sensitivity analysis showed that cost per patient is an important determinant of cost-effectiveness so an accurate estimate of the incidence of patients likely to benefit from pre-hospital CPAP is required to accurately estimate cost-effectiveness.
We assumed that all patients receiving pre-hospital CPAP would have this treatment continued in hospital and, that, in the absence of pre-hospital CPAP, all potentially treated patients would receive pre-hospital NIV. This assumption is unlikely to hold in practice. However, it is reasonable to assume that if pre-hospital CPAP is used in additional patients who are unlikely to really need it then this will only incur a small additional cost (since most of the costs of pre-hospital CPAP are accrued in setting up the service) and no significant additional benefit, so this will not markedly affect overall cost-effectiveness.
Uncertainties
Although our meta-analysis appears to show that pre-hospital CPAP is an effective treatment for acute respiratory failure, the reliability of this conclusion is limited by the issues outlined in the previous section, Strengths and limitations of the assessment. Evidence derived from synthesising multiple small trials should ideally be confirmed in a large pragmatic trial. None of the trials was undertaken in the UK, the intervention was delivered by physicians in most trials and the trial populations might have been a selected subset of all those presenting with acute respiratory failure. Further research is required to determine if pre-hospital NIV can be delivered by NHS paramedics and if the effectiveness of pre-hospital CPAP is confirmed in these circumstances.
Our estimates of the effectiveness of pre-hospital BiPAP were subject to substantial uncertainty and include the possibility of worthwhile benefit and significant harm. A large pragmatic trial would help to resolve this uncertainty but this is unlikely, unless a strong theoretical case can be made for favouring BiPAP over CPAP, since pre-hospital CPAP appears to be more feasible in the NHS and is supported by more promising data.
Economic analysis showed that the cost-effectiveness of pre-hospital CPAP is uncertain, with an ICER close to the £20,000-per-QALY threshold and 49.5% probability of being considered cost-effective at this threshold. Sensitivity analysis showed that these findings were dependent on the incidence of patients likely to benefit from pre-hospital CPAP and, to a lesser extent, whether the ambulance service covers a rural or urban population. Accurate estimation of the incidence of suitable patients is required to better estimate cost-effectiveness.
Expected value of information analysis was undertaken to explore uncertainty and determine the value of further research. It showed that the value of undertaking a trial depends on the estimated incidence of eligible patients. The maximum cost at which it would be cost-effective to carry out a trial with 100 patients in each arm is only £1.08M if there is a low estimated incidence of eligible patients, but is £12.67M if there is a high estimated incidence. A more precise estimate of the incidence of eligible patients is therefore required to determine the cost-effectiveness of a future trial of pre-hospital CPAP in the NHS.
Other relevant factors
Our meta-analysis suggests that pre-hospital CPAP is effective for acute respiratory failure but provides no evidence that BiPAP is effective. This should not be interpreted as providing evidence that pre-hospital CPAP works while pre-hospital BiPAP does not. A meta-analysis91 and a large trial92 comparing in-hospital CPAP to BiPAP for ACPO showed no significant differences in clinical outcomes. There was substantial uncertainty around the estimates of effectiveness of BiPAP and these include the possibility of worthwhile benefit. Our decision to use CPAP as the modality for pre-hospital NIV in the economic analysis was based on the practicalities of delivering pre-hospital NIV in a paramedic-based system rather than selection on the basis of effectiveness.
Assessment of factors relevant to the NHS and other parties
Acute respiratory failure is the common pathway for some of the most frequent causes of in-hospital death. Our analysis has shown that pre-hospital CPAP may reduce mortality from acute respiratory failure. However, this does not mean that large numbers of patients are eligible to be treated by pre-hospital CPAP. Its effectiveness is likely to be limited to selected patients with a reversible underlying cause who are transported to hospital by emergency ambulance and are severely ill, such that supplemental oxygen and medical therapy will be inadequate and the delay incurred prior to receiving in-hospital NIV could be critical.
We identified a number of sources to estimate the incidence of such cases, but none was ideal, and there was substantial variation between estimates. As noted earlier, this is important because the incidence of patients likely to benefit from CPAP has a powerful impact on cost-effectiveness. Perhaps more fundamentally, this parameter determines how important the whole issue of pre-hospital NIV is to the NHS.
The configuration of emergency medical care in the NHS is an important and related factor. Centralisation of services can improve outcomes for patients with myocardial infarction, stroke and major trauma, but may increase the distance that patients have to travel to hospital. This may increase the risk of death for some patient groups, particularly those with respiratory diseases and especially acute respiratory failure. We modelled the effect of increasing the distance travelled to hospital by comparing the general population scenario to an urban scenario with shorter distances and to a rural scenario with longer distances. Unsurprisingly, given the assumptions of our model, pre-hospital CPAP was more effective and thus more cost-effective in the rural scenario. However, variation in cost-effectiveness was modest and, in all three scenarios tested, the cost-effectiveness of pre-hospital CPAP compared with standard care remained uncertain with an ICER close to the £20,000 per QALY threshold. It therefore appears that, although any reconfiguration that increases the distance travelled to hospital could increase the potential need for pre-hospital CPAP, it is not likely to have a major impact on our estimates of cost-effectiveness.
As highlighted in Strengths and limitations of the assessment, all the trials of pre-hospital NIV were undertaken in pre-hospital systems that may differ markedly from the NHS. Most involved physicians delivering pre-hospital NIV, and those involving paramedics typically had some form of online support. Further research is required to determine the effectiveness of pre-hospital NIV delivered by independently working paramedics, which would represent typical practice in the NHS. In the economic analysis, we assumed that pre-hospital CPAP was more likely to be feasible in the NHS and would be delivered by widespread training of paramedics and equipping of ambulances. This approach is expensive but has the advantage of ensuring maximal coverage of the population. Alternative approaches could be used involving a smaller number of critical care paramedics using rapid response vehicles. This would be potentially cheaper but would require accurate targeting of patients eligible for pre-hospital NIV. There is currently no research available to determine whether or not this would be possible, so this approach carries a substantial risk that only a minority of patients who could benefit from pre-hospital CPAP would actually receive it.
If the model of delivering pre-hospital CPAP used in our analysis is adopted it will incur substantial up-front costs for training paramedics and equipping ambulances. Reducing hospital length of stay may offset some of these costs, and the overall additional costs may be justified by improved outcomes, but further research is required to determine this. Even if pre-hospital CPAP were shown to be cost-effective it would still require substantial up-front funding, which would involve either allocation of additional resources to ambulance services or reallocation from within the ambulance service budget.
Chapter 6 Conclusions
Implications for service provision
The available evidence suggests that pre-hospital CPAP is associated with reduced mortality and reduced intubation rates for patients with acute respiratory failure compared with standard care. However, this evidence has a number of limitations that may have led to overestimation of effectiveness, and the findings of the meta-analysis may not be generalisable to the NHS. Furthermore, pre-hospital CPAP can be effectively delivered only if ambulances are appropriately equipped and paramedics are properly trained. We estimated that setting up and running pre-hospital CPAP across an ambulance service covering a population of 5 million would cost between £235,683 and £582,300 per year. This means that any recommendation to implement pre-hospital CPAP needs to be based on evidence of cost-effectiveness as well as effectiveness.
Economic analysis suggested that cost-effectiveness is uncertain and is dependent on our estimates of effectiveness being realised in practice and that there is a sufficient pool of patients who would receive and benefit from pre-hospital CPAP. Current evidence is therefore insufficient to recommend the implementation of pre-hospital CPAP in the NHS. Further evidence of feasibility, effectiveness and cost-effectiveness in the NHS is required. It has been suggested that in the past pre-hospital and emergency medicine practitioners may have adopted new technologies before rigorous evaluation. 93 It may therefore be wise to recommend that use of pre-hospital CPAP in the NHS should be limited to the research setting.
Although BiPAP has theoretical benefits compared with CPAP, the available evidence is much weaker and does not currently support the use of pre-hospital BiPAP. The equipment and training required to deliver BiPAP may make this more difficult to deliver in the NHS. Further research into pre-hospital BiPAP is therefore desirable, but the practical advantages and more promising existing data for CPAP mean that future research efforts are likely to be better focused on pre-hospital CPAP.
Suggested research priorities
A large pragmatic trial with associated economic analysis could determine if pre-hospital CPAP is a clinically effective and cost-effective treatment for acute respiratory failure in the NHS. The trial would need to be large enough to detect a modest but potentially worthwhile difference in mortality. It would also need to compare pre-hospital CPAP with best alternative care, which in most cases would involve in-hospital NIV, and would need to be powered to explore effectiveness in subgroups with different transport times to hospital, such as rural and urban populations. However, our expected value of information analysis suggested that a trial costing several million pounds would only be cost-effective if the incidence of eligible patients was towards the higher end of our range of estimates. A more precise estimate of this parameter is therefore required before a trial can be recommended.
The incidence of eligible patients is also an important determinant of the feasibility of a trial. Estimates used in our economic analysis suggest that a typical paramedic may, on average, see an eligible patient less often than once per year. Such a low frequency may make reliable identification and recruitment difficult. Pre-hospital care is a challenging environment in which to recruit and randomise patients. 94 NHS paramedics currently have little experience of recruiting to trials or providing pre-hospital CPAP. A trial would be feasible only if there were sufficient eligible patients to support a reasonable recruitment rate and if these patients could be recruited and randomised in the challenging pre-hospital environment.
We therefore recommend a feasibility study of pre-hospital CPAP in one ambulance service to determine the incidence of patients transported by emergency ambulance who are eligible for pre-hospital CPAP. If randomised, this study could also determine the feasibility of a trial and explore barriers to recruitment. Updating our economic model with a more accurate estimate of the incidence of eligible patients would reduce the uncertainty surrounding our estimate of the cost-effectiveness of both a trial of pre-hospital CPAP and the cost-effectiveness of pre-hospital CPAP itself. If the feasibility study showed that pre-hospital CPAP could be appropriately delivered in the NHS with an incidence of use towards the higher end of our estimates, and showed that recruitment and randomisation were feasible, then a large pragmatic trial to determine clinical effectiveness and cost-effectiveness could be recommended.
Acknowledgements
We would like to thank Professor Matt Stevenson, School of Health and Related Research, University of Sheffield; Professor Simon Dixon, School of Health and Related Research, University of Sheffield; and Enid Hirst, Chairperson of Sheffield Emergency Care Forum, Sheffield, for their help and support with this research project.
We would also like to thank the following people for providing IPD from their trial for meta-analysis: Professor Vincent Bounes, Department of Emergency Medicine, Toulouse University Hospital, Toulouse, France; Dr Arantxa Mas, Intensive Care Department, Hospital de Sant Joan Despí Moisès Broggi, Barcelona, Spain; Professor Didier Payen, Department of Anaesthesiology and Critical Care, Lariboisière Hospital, Paris, France; Professor David Petrie, Department of Emergency Medicine, Dalhousie University, Nova Scotia, Canada; Dr Markus Soren Roessler, Department of Anaesthesiology, Emergency and Intensive Care Medicine, Georg-August-University, Goettingen, Germany; and Dr Gunther Weitz, University Hospital of Schleswig-Holstein, Lübeck, Germany.
Thanks also to Kathryn MacKellar for clerical assistance.
Contributions of authors
Abdullah Pandor (Senior Research Fellow) co-ordinated the review and was responsible for the acquisition of data, analysis and interpretation of data (for the systematic review) and drafting and revising the final report.
Praveen Thokala (Research Fellow) was responsible for the acquisition of data, analysis and interpretation of data and model construction (for the health economic evaluations) and drafting and revising of the final report.
Steve Goodacre (Professor of Emergency Medicine) was responsible for conception and design of the study, acquisition of data, analysis and interpretation of data (for the systematic reviews and health economic evaluations), and drafting and revising the final report.
Edith Poku (Research Associate) was responsible for the acquisition of data, analysis and interpretation of data (for the systematic reviews), and drafting and revising the final report.
John W Stevens (Senior Lecturer in Statistics) was responsible for the statistical analyses, interpretation of data and drafting and revising the final report.
Shijie Ren (Research Associate) was responsible for the statistical analyses, interpretation of data and drafting and revising the final report.
Anna Cantrell (Information Specialist) was responsible for the developing and undertaking the electronic literature searches.
Gavin D Perkins (Professor of Critical Care Medicine) contributed to the conception and design of the study, assisted with data collection and was responsible for the interpretation of data (for the systematic reviews and health economic evaluations), and drafting and revising the final report.
Matt Ward (Head of Clinical Practice) was responsible for the interpretation of data (for the systematic reviews and health economic evaluations), and drafting and revising the final report.
Jerry Penn-Ashman (General Manager Area Support) was responsible for the interpretation of data (for the systematic reviews and health economic evaluations), and drafting and revising the final report.
Disclaimers
This report presents independent research funded by the National Institute for Health Research (NIHR). The views and opinions expressed by authors in this publication are those of the authors and do not necessarily reflect those of the NHS, the NIHR, NETSCC, the HTA programme or the Department of Health. If there are verbatim quotations included in this publication the views and opinions expressed by the interviewees are those of the interviewees and do not necessarily reflect those of the authors, those of the NHS, the NIHR, NETSCC, the HTA programme or the Department of Health.
References
- Baudouin S, Blumenthal S, Cooper B, Davidson C, Davison A, Elliott M, et al. Non-invasive ventilation in acute respiratory failure – British Thoracic Society standards of care committee. Thorax 2002;57:192-211. http://dx.doi.org/10.1136/thorax.57.3.192.
- Delmere S, Ray P. Acute respiratory failure in the elderly: diagnosis and prognosis. Age Ageing 2008;37:251-7. http://dx.doi.org/10.1093/ageing/afn060.
- Aminzadeh F, Dalziel WB. Older adults in the emergency department: a systematic review of patterns of use, adverse outcomes, and effectiveness of interventions. Ann Emerg Med 2002;39:238-47. http://dx.doi.org/10.1067/mem.2002.121523.
- Ray P, Birolleau S, Lefort Y, Becquemin MH, Beigelman C, Isnard R, et al. Acute respiratory failure in the elderly: etiology, emergency diagnosis and prognosis. Crit Care 2006;10. http://dx.doi.org/10.1186/cc4926.
- The Health and Social Care Information Centre . Hospital Episodes Statistics for England: Linked ONS-HES Mortality Data, 2009–10 2010. www.hesonline.nhs.uk (accessed 19 December 2012).
- Nicholl J, West J, Goodacre S, Turner J. The relationship between distance to hospital and patient mortality in emergencies: an observational study. Emerg Med J 2007;24:665-8. http://dx.doi.org/10.1136/emj.2007.047654.
- UK Ambulance Services Clinical Practice Guidelines 2013. Basingstoke: Association of Ambulance Chief Executives; 2013.
- Berlac P, Hyldmo PK, Kongstad P, Kurola J, Nakstad AR, Sandberg M. Pre-hospital airway management: guidelines from a task force from the Scandinavian Society for Anaesthesiology and Intensive Care Medicine. Acta Anaesthesiol Scand 2008;52:897-90. http://dx.doi.org/10.1111/j.1399-6576.2008.01673.x.
- Fisher JD, Brown SN, Cooke MW. UK Ambulance Service Clinical Practice Guidelines (2006). London: Joint Royal College Ambulance Liaison Committee; 2006.
- Keenan SP, Sinuff T, Burns KEA, Muscedere J, Kutsogiannis J, Mehta S, et al. Clinical practice guidelines for the use of noninvasive positive-pressure ventilation and noninvasive continuous positive airway pressure in the acute care setting. CMAJ 2011;183:E195-214. http://dx.doi.org/10.1503/cmaj.100071.
- Kunze R. Standards and guidelines for prehospital ventilation management. J Anasth Intensivbehandlung 2007;15:61-5.
- Llorens P, Miro O, Martin-Sanchez FJ, Puente PH, Rodriguez JJ, Gil V, et al. Guidelines for emergency management of acute heart failure: consensus of the Acute Heart Failure Working Group of the Spanish Society of Emergency Medicine (ICA-SEMES) in 2011. Emergencias 2011;23:119-39.
- Roberts CM, Brown JL, Reinhardt AK, Kaul S, Scales K, Mikelsons C, et al. Non-invasive ventilation in chronic obstructive pulmonary disease: management of acute type 2 respiratory failure. Clin Med 2008;8:517-21. http://dx.doi.org/10.7861/clinmedicine.8-5-517.
- Schönhofer B, Kuhlen R, Neumann P, Westhoff M, Berndt C, Sitter H. Non-invasive ventilation as treatment for acute respiratory insufficiency. Essentials from the new S3 guidelines. Anaesthesist 2008;57:1091-102. http://dx.doi.org/10.1007/s00101-008-1449-0.
- Sinuff T, Keenan SP. Clinical practice guideline for the use of noninvasive positive pressure ventilation in COPD patients with acute respiratory failure. J Crit Care 2004;19:82-91. http://dx.doi.org/10.1016/j.jcrc.2004.04.006.
- Chronic Obstructive Pulmonary Disease: Management of Chronic Obstructive Pulmonary Disease in Adults In Primary and Secondary Care. London: National Clinical Guideline Centre; 2010.
- McMurray JJV, Adamopoulos S, Anker SD, Auricchio A, Bohm M, Dickstein K, et al. ESC Guidelines for the diagnosis and treatment of acute and chronic heart failure 2012. The Task Force for the Diagnosis and Treatment of Acute and Chronic Heart Failure 2012 of the European Society of Cardiology. Developed in collaboration with the Heart Failure Association (HFA) of the ESC. Eur Heart J 2012;33:1787-847. http://dx.doi.org/10.1093/eurheartj/ehs104.
- Gray AJ, Goodacre S, Newby DE, Masson MA, Sampson F, Dixon S, et al. A multicentre randomised controlled trial of the use of continuous positive airway pressure and non-invasive positive pressure ventilation in the early treatment of patients presenting to the emergency department with severe acute cardiogenic pulmonary oedema: the 3CPO trial. Health Technol Assess 2009;13. http://dx.doi.org/10.3310/hta13330.
- Ho K, Wong K. A comparison of continuous and bi-level positive airway pressure non-invasive ventilation in patients with acute cardiogenic pulmonary oedema: a meta-analysis. Crit Care 2006;10. http://dx.doi.org/10.1186/cc4861.
- Keenan SP, Sinuff T, Cook DJ, Hill NS. Does noninvasive positive pressure ventilation improve outcome in acute hypoxemic respiratory failure? A systematic review. Crit Care Med 2004;32:2516-23. http://dx.doi.org/10.1097/01.CCM.0000148011.51681.E2.
- Lightowler JV, Wedzicha JA, Elliott MW, Ram FSF. Non-invasive positive pressure ventilation to treat respiratory failure resulting from exacerbations of chronic obstructive pulmonary disease: Cochrane systematic review and meta-analysis. BMJ 2003;326:185-7. http://dx.doi.org/10.1136/bmj.326.7382.185.
- Masip J, Roque M, Sanchez B, Fernandez R, Subirana M, Exposito JA. Noninvasive ventilation in acute cardiogenic pulmonary edema: systematic review and meta-analysis. JAMA 2005;294:3124-30. http://dx.doi.org/10.1001/jama.294.24.3124.
- Peter JV, Moran JL, Phillips-Hughes J, Graham P, Bersten AD. Effect of non-invasive positive pressure ventilation (NIPPV) on mortality in patients with acute cardiogenic pulmonary oedema: a meta-analysis. Lancet 2006;367:1155-63. http://dx.doi.org/10.1016/S0140-6736(06)68506-1.
- Ram FS, Wellington S, Rowe B, Wedzicha JA. Non-invasive positive pressure ventilation for treatment of respiratory failure due to severe acute exacerbations of asthma. Cochrane Database Syst Rev 2005;3. http://dx.doi.org/10.1002/14651858.CD004360.pub2.
- Collins SP, Mielniczuk LM, Whittingham HA, Boseley ME, Schramm DR, Storrow AB. The use of noninvasive ventilation in emergency department patients with acute cardiogenic pulmonary edema: a systematic review. Ann Emerg Med 2006;48:260-9. http://dx.doi.org/10.1016/j.annemergmed.2006.01.038.
- Squadrone V, Coha M, Cerutti E, Schellino MM, Biolino P, Occella P, et al. Continuous positive airway pressure for treatment of postoperative hypoxemia: a randomized controlled trial. JAMA 2005;293:589-95. http://dx.doi.org/10.1001/jama.293.5.589.
- Devlin JW, Nava S, Fong JJ, Bahhady I, Hill NS. Survey of sedation practices during noninvasive positive-pressure ventilation to treat acute respiratory failure. Crit Care Med 2007;35:2298-302. http://dx.doi.org/10.1097/01.CCM.0000284512.21942.F8.
- Bercault N, Boulain T. Mortality rate attributable to ventilator-associated nosocomial pneumonia in an adult intensive care unit: a prospective case-control study. Crit Care Med 2001;29:2303-9. http://dx.doi.org/10.1097/00003246-200112000-00012.
- Baird JS, Ravindranath TM. Out-of-hospital noninvasive ventilation: epidemiology, technology and equipment. Paediatr Rep 2012;4. http://dx.doi.org/10.4081/pr.2012.e17.
- Rabec C, Rodenstein D, Leger P, Rouault S, Perrin C, Gonzalez-Bermejo J. Ventilator modes and settings during non-invasive ventilation: effects on respiratory events and implications for their identification. Thorax 2011;66:170-8. http://dx.doi.org/10.1136/thx.2010.142661.
- Penuelas O, Frutos-Vivar F, Esteban A, Ferguson ND, Raymondos K, Bin D, et al. Factor associated to failure and outcome of non-invasive positive pressure ventilation. Am J Respir Crit Care Med 2011;183. http://dx.doi.org/10.1164/ajrccm-conference.2011.183.1_MeetingAbstracts.A3741.
- Baptista FA, Moral GJ, del Pozo FJF. Management of acute respiratory failure with noninvasive ventilation in the emergency department. Emergencias 2009;21:189-202.
- Schönhofer B, Sortor-Leger S. Equipment needs for noninvasive mechanical ventilation. Eur Respir J 2002;20:1029-36. http://dx.doi.org/10.1183/09031936.02.00404202.
- Masip J, Mebazaa A, Filippatos G. Noninvasive ventilation in acute cardiogenic pulmonary edema. N Engl J Med 2008;359:2068-9. http://dx.doi.org/10.1056/NEJMc081649.
- Simpson PM, Bendall JC. Prehospital non-invasive ventilation for acute cardiogenic pulmonary oedema: an evidence-based review. Emerg Med J 2011;28:609-12. http://dx.doi.org/10.1136/emj.2010.092296.
- Williams B, Boyle M, Robertson N, Giddings C. When pressure is positive: a literature review of the prehospital use of continuous positive airway pressure. Prehosp Disaster Med 2013;28:52-60. http://dx.doi.org/10.1017/S1049023X12001562.
- Daily JC, Wang HE. Noninvasive positive pressure ventilation: resource document for the National Association of EMS Physicians position statement. Prehosp Emerg Care 2011;15:432-8. http://dx.doi.org/10.3109/10903127.2011.569851.
- Rees N. Prehospital continuous positive airway pressure ventilation in ACPO: part 1. J Paramedic Pract 2011;3:129-34. http://dx.doi.org/10.12968/jpar.2011.3.3.129.
- Moher D, Liberati A, Tetzlaff J, Altman DG. Preferred reporting items for systematic reviews and meta-analyses: the PRISMA statement. PLOS Med 2009;6. http://dx.doi.org/10.1371/journal.pmed.1000097.
- Verhagen AP, de Vet HC, de Bie RA, Kessels AG, Boers M, Bouter LM, et al. The Delphi list: a criteria list for quality assessment of randomized clinical trials for conducting systematic reviews developed by Delphi consensus. J Clin Epidemiol 1998;51:1235-41. http://dx.doi.org/10.1016/S0895-4356(98)00131-0.
- Lunn DJ, Thomas A, Best N, Spiegelhalter D. WinBUGS – a Bayesian modelling framework: concepts, structure, and extensibility. Stat Comput 2000;10:325-37. http://dx.doi.org/10.1023/A:1008929526011.
- McCullah P, Nelder J. Generalized Linear Models. London: Chapman & Hall; 1989.
- Lunn D, Spiegelhalter D, Thomas A, Best N. The BUGS project: evolution, critique and future directions. Stat Med 2009;28:3049-67. http://dx.doi.org/10.1002/sim.3680.
- Brooks S, Gelman A. Alternative methods for monitoring convergence of iterative simulations. J Comput Graphical Stat 1998;7:434-45.
- Spiegelhalter DJ, Best NG, Carlin BR, van der Linde A. Bayesian measures of model complexity and fit. J R Stat Soc B Stat Methodol 2002;64:583-639. http://dx.doi.org/10.1111/1467-9868.00353.
- Austin MA, Wills KE. Effect of continuous positive airway pressure on mortality in the treatment of acute cardiogenic pulmonary edema in the prehospital setting: randomized controlled trial. Acad Emerg Med 2012;19.
- Ducros L, Logeart D, Vicaut E, Henry P, Plaisance P, Collet JP, et al. CPAP for acute cardiogenic pulmonary oedema from out-of-hospital to cardiac intensive care unit: a randomised multicentre study. Intensive Care Med 2011;37:1501-9. http://dx.doi.org/10.1007/s00134-011-2311-4.
- Frontin P, Bounes V, Houze-Cerfon CH, Charpentier S, Houze-Cerfon V, Ducasse JL. Continuous positive airway pressure for cardiogenic pulmonary edema: a randomized study. Am J Emerg Med 2011;29:775-81. http://dx.doi.org/10.1016/j.ajem.2010.03.007.
- Mas A, Alonso G, Perez C, Saura P, Alcoverro JM, Guirado M. Non-invasive mechanical ventilation for acute dyspnea in out-of-hospital emergency care. Intensive Care Med 2002;28.
- Plaisance P, Pirracchio R, Berton C, Vicaut E, Payen D. A randomized study of out-of-hospital continuous positive airway pressure for acute cardiogenic pulmonary oedema: physiological and clinical effects. Eur Heart J 2007;28:2895-901. http://dx.doi.org/10.1093/eurheartj/ehm502.
- Roessler MS, Schmid DS, Michels P, Schmid O, Jung K, Stober J, et al. Early out-of-hospital non-invasive ventilation is superior to standard medical treatment in patients with acute respiratory failure: a pilot study. Emerg Med J 2012;29:409-14. http://dx.doi.org/10.1136/emj.2010.106393.
- Schmidbauer W, Ahlers O, Spies C, Dreyer A, Mager G, Kerner T. Early prehospital use of non-invasive ventilation improves acute respiratory failure in acute exacerbation of chronic obstructive pulmonary disease. Emerg Med J 2011;28:626-7. http://dx.doi.org/10.1136/emj.2009.089102.
- Thompson J, Petrie DA, Ackroyd-Stolarz S, Bardua DJ. Out-of-hospital continuous positive airway pressure ventilation versus usual care in acute respiratory failure: a randomized controlled trial. Ann Emerg Med 2008;52:232-41. http://dx.doi.org/10.1016/j.annemergmed.2008.01.006.
- Craven RA, Singletary N, Bosken L, Sewell E, Payne M, Lipsey R. Use of bilevel positive airway pressure in out-of-hospital patients. Acad Emerg Med 2000;7:1065-8. http://dx.doi.org/10.1111/j.1553-2712.2000.tb02102.x.
- Weitz G, Struck J, Zonak A, Balnus S, Perras B, Dodt C. Prehospital noninvasive pressure support ventilation for acute cardiogenic pulmonary edema. Eur J Emerg Med 2007;14:276-9. http://dx.doi.org/10.1097/MEJ.0b013e32826fb377.
- Fort PA. Multicenter National Randomized Controlled Open Label Study Assessing Interest of Non Invasive Ventilation in Out-of Hospital Setting During Acute Respiratory Failure in Chronic Obstructive Pulmonary Disease Patients. VeNIS BPCO 2012. clinicaltrials.gov/show/NCT01626937 (accessed 15 August 2013).
- Schulz KF, Grimes DA. Generation of allocation sequences in randomised trials: chance, not choice. Lancet 2002;359:515-19. http://dx.doi.org/10.1016/S0140-6736(02)07683-3.
- Dias S, Welton NJ, Sutton AJ, Ades AE. NICE DSU Technical Support Document 2: A Generalised Linear Modelling Framework for Pairwise and Network Meta-nalysis of Randomised Controlled Trials. Sheffield: Decision Support Unit, ScHARR, University of Sheffield; 2013.
- Aguilar SA, Castillo E, Choy J, Tuan B, Buono C, Serra J. Evaluation of prehospital continuous positive airway pressure ventilation for cases of respiratory distress. Acad Emerg Med 2011;18.
- Aguilar SA, Lee J, Castillo E, Lam B, Choy J, Patel E, et al. Assessment of the addition of prehospital continuous positive airway pressure (CPAP) to an urban emergency medical services (EMS) system in persons with severe respiratory distress. J Emerg Med 2013;45:210-19. http://dx.doi.org/10.1016/j.jemermed.2013.01.044.
- Berteloot C, Cuny J, Wiel E, Goldstein P. Assessment of the use of non invasive continuous positive airway pressure associated with nebulization in pre-hospital management. Intensive Care Med 2011;37.
- Bledsoe BE, Anderson E, Hodnick R, Johnson L, Johnson S, Dievendorf E. Low-fractional oxygen concentration continuous positive airway pressure is effective in the prehospital setting. Prehosp Emerg Care 2012;16:217-21. http://dx.doi.org/10.3109/10903127.2011.640765.
- Bruge P, Jabre P, Dru M, Jbeili C, Lecarpentier E, Khalid M, et al. An observational study of noninvasive positive pressure ventilation in an out-of-hospital setting. Am J Emerg Med 2008;26:165-9. http://dx.doi.org/10.1016/j.ajem.2007.04.022.
- Bultman LL, Mahoney B, Fringer R. Effectiveness of prehospital continuous positive airway pressure in treating adult respiratory distress. Ann Emerg Med 2005;46. http://dx.doi.org/10.1016/j.annemergmed.2005.06.416.
- Cuny J, Campagne G, Gosselin P, Goldstein P, Assez N, Wiel E. Analysis of management of non-invasive ventilation support in prehospital care for COPD patients and short-term outcome. Crit Care 2013;17.
- Derr C, O’Connor B, Klash A, Galwankar S, MacLeod S. The use of continuous positive airway pressure for the treatment of acute severe pulmonary edema in the prehospital setting. Ann Emerg Med 2006;48. http://dx.doi.org/10.1016/j.annemergmed.2006.07.765.
- Dib JE, Matin SA, Luckert A. Prehospital use of continuous positive airway pressure for acute severe congestive heart failure. J Emerg Med 2012;42:553-8. http://dx.doi.org/10.1016/j.jemermed.2011.06.002.
- Dieperink W, Weelink EE, van der Horst IC, de Vos R, Jaarsma T, Aarts LP, et al. Treatment of presumed acute cardiogenic pulmonary oedema in an ambulance system by nurses using Boussignac continuous positive airway pressure. Emerg Med J 2009;26:141-4. http://dx.doi.org/10.1136/emj.2007.055095.
- Foti G, Sangalli F, Berra L, Sironi S, Cazzaniga M, Rossi GP, et al. Is helmet CPAP first line pre-hospital treatment of presumed severe acute pulmonary edema?. Intensive Care Med 2009;35:656-62. http://dx.doi.org/10.1007/s00134-008-1354-7.
- Freitas P, Limpo B, Sá N, Camões A, Lufinha A. Prehospital boussignac continuous positive airway pressure: One year experience. Resuscitation 2010;81. http://dx.doi.org/10.1016/j.resuscitation.2010.09.233.
- Fyntanidou B, Amaniti E, Fortounis K, Grosomanidis V, Paulou K. Prehospital use of Boussignac continuous positive airway pressure system in acute cardiogenic pulmonary edema. Eur J Anaesthesiol 2009;26.
- Garuti G, Bandiera G, Cattaruzza MS, Gelati L, Osborn JF, Toscani S, et al. Out-of-hospital helmet CPAP in acute respiratory failure reduces mortality: a study led by nurses. Monaldi Arch Chest Dis 2010;73:145-51.
- Grosomanidis V, Karakoulas K, Matsikoudi C, Sofianos E, Ourailoglou V, Theodosiadis P, et al. Prehospital management of pulmonary edema by means of the Boussignac-Vygon (TM) CPAP system. 30th International Educational and Scientific Symposium, Society of Critical Care Medicine, February 2001, San Francisco, USA. Crit Care Med 2000;28.
- Hubble MW, Richards ME, Jarvis R, Millikan T, Young D. Effectiveness of prehospital continuous positive airway pressure in the management of acute pulmonary edema. Prehosp Emerg Care 2006;10:430-9. http://dx.doi.org/10.1080/10903120600884848.
- Kallio T, Kuisma M, Alaspaa A, Rosenberg PH. The use of prehospital continuous positive airway pressure treatment in presumed acute severe pulmonary edema. Prehosp Emerg Care 2003;7:209-13. http://dx.doi.org/10.1080/10903120390936798.
- Kosowsky JM, Stephanides SL, Branson RD, Sayre MR. Prehospital use of continuous positive airway pressure (CPAP) for presumed pulmonary edema: a preliminary case series. Prehosp Emerg Care 2001;5:190-6. http://dx.doi.org/10.1080/10903120190940119.
- Spijker EE, de Bont M, Bax M, Sandel M. Practical use, effects and complications of prehospital treatment of acute cardiogenic pulmonary edema using the Boussignac CPAP system. Int J Emerg Med 2013;6. http://dx.doi.org/10.1186/1865-1380-6-8.
- Templier F, Dolveck F, Baer M, Chauvin M, Fletcher D. ‘Boussignac’ continuous positive airway pressure system: practical use in a prehospital medical care unit. Eur J Emerg Med 2003;10:87-93. http://dx.doi.org/10.1097/00063110-200306000-00003.
- Warner GS. Evaluation of the effect of prehospital application of continuous positive airway pressure therapy in acute respiratory distress. Prehosp Disaster Med 2010;25:87-91.
- Hubble MW, Richards ME, Wilfong DA. Estimates of cost-effectiveness of prehospital continuous positive airway pressure in the management of acute pulmonary edema. Prehosp Emerg Care 2008;12:277-85. http://dx.doi.org/10.1080/10903120801949275.
- Drummond MF, Jefferson TO. Guidelines for authors and peer reviewers of economic submissions to the BMJ. BMJ 1996;313:275-83. http://dx.doi.org/10.1136/bmj.313.7052.275.
- Drummond MF, Sculpher MJ, Torrance GW, O’Brien BJ, Stoddart GL. Methods for the Economic Evaluation of Health Care Programmes. Oxford: Oxford University Press; 2005.
- Eddy DM. Assessing Medical Technology. Washington, DC: National Academy Press; 1985.
- R Development Core Team . R: A Language and Environment for Statistical Computing 2008.
- Guide To the Methods of Technology Appraisals. London: NICE; 2008.
- National Respiratory Audit Programme Annual Report 2011/12. London: British Thoracic Society; 2012.
- Luhr OR, Antonsen K, Karlsson M, Aardal S, Thorsteinsson A, Frostell CG, et al. Incidence and mortality after acute respiratory failure and acute respiratory distress syndrome in Sweden, Denmark, and Iceland. Am J Respir Crit Care Med 1999;159:1849-61. http://dx.doi.org/10.1164/ajrccm.159.6.9808136.
- Curtis L. Unit Costs of Health and Social Care 2012. Canterbury: Personal Social Services Research Unit, University of Kent; 2012.
- NHS Reference Costs 2011–2012. London: Department of Health; 2012.
- Williams TA, Finn J, Perkins GD, Jacobs IG. Prehospital continuous positive airway pressure for acute respiratory failure: a systematic review and meta-analysis. Prehosp Emerg Care 2013;17:261-73. http://dx.doi.org/10.3109/10903127.2012.749967.
- Hui L, Chunlin H, Jinming X, Xin L, Hongyan W, Xiaoyun Z, et al. A comparison of bilevel and continuous positive airway pressure noninvasive ventilation in acute cardiogenic pulmonary edema. Am J Emerg Med 2013;31:1322-7. http://dx.doi.org/10.1016/j.ajem.2013.05.043.
- Gray A, Goodacre S, Newby DE, Masson M, Sampson F, Nicholl J. Noninvasive ventilation in acute cardiogenic pulmonary edema. N Engl J Med 2008;359:142-51. http://dx.doi.org/10.1056/NEJMoa0707992.
- Woollard M, Mackway-Jones K. All that glistens is not gold. Emerg Med J 2008;25:251-2. http://dx.doi.org/10.1136/emj.2008.057711.
- Hargreaves K, Goodacre S, Mortimer P. Paramedic perceptions of the feasibility and practicalities of prehospital clinical trials: a questionnaire survey. Emerg Med J 2013;31:499-504. http://dx.doi.org/10.1136/emermed-2013-202346.
- Austin MA, Wills E, Kilpatrick D, Gibson M, Walters EH. Effect of continuous positive airway pressure (CPAP) on mortality in the treatment of acute cardiogenic pulmonary oedema (ACPO) in the pre-hospital setting: randomised controlled trial. European Medicines Agency 2013;25.
- Baker JP. Intermittent positive pressure breathing or compressor nebulizer therapy?. Ann Intern Med 1983;99:715-16. http://dx.doi.org/10.7326/0003-4819-99-5-715.
- Baker S. Use of continuous positive airway pressure for acute severe dyspnoea in the pre-hospital setting?. Crit Care Resusc 2005;7:50-2.
- Bohanske MS, Harrington LL, White LF, Dyer K. The effect of continuous positive airway pressure on patient care time in an urban EMS system. Ann Emerg Med 2010;6. http://dx.doi.org/10.1016/j.annemergmed.2010.06.252.
- Bott J, Carroll MP, Conway JH, Keilty SE, Ward EM, Brown AM, et al. Randomised controlled trial of nasal ventilation in acute ventilatory failure due to chronic obstructive airways disease. Lancet 1993;341:1555-7. http://dx.doi.org/10.1016/0140-6736(93)90696-E.
- Cheskes S, Thomson S, Turner L. Feasibility of continuous positive airway pressure by primary care paramedics. Prehosp Emerg Care 2012;16:535-40. http://dx.doi.org/10.3109/10903127.2012.689930.
- Confalonieri M, Potena A, Carbone G, Porta RD, Tolley EA, Umberto MG. Acute respiratory failure in patients with severe community-acquired pneumonia. A prospective randomized evaluation of noninvasive ventilation. Am J Respir Crit Care Med 1999;160:1585-91. http://dx.doi.org/10.1164/ajrccm.160.5.9903015.
- Crawford J, Otero R, Rivers EP, Lenoir T. How quick is soon? Early response to continuous positive airway pressure: a randomized controlled trial. Crit Care 2008;12. http://dx.doi.org/10.1186/cc6565.
- Ducros L, Logreart D, Collet JP, Vicaut E, Henry P, Montalescot G, et al. Continuous positive airway pressure from out-of-hospital setting to cardiac intensive care unit in acute heart failure: a multicenter randomized study. Circulation 2008;118.
- Gardtman M, Waagstein L, Karlsson T, Herlitz J. Has an intensified treatment in the ambulance of patients with acute severe left heart failure improved the outcome?. Eur J Emerg Med 2000;7:15-24. http://dx.doi.org/10.1097/00063110-200003000-00004.
- Gonzva J, Prunet B, Deniel C, Benner P, Toppin F, Brun PM. Early antidote use associated with noninvasive ventilation in prehospital treatment of methadone intoxication. Am J Emerg Med 2013;31:448-56. http://dx.doi.org/10.1016/j.ajem.2012.06.015.
- Goss JF. How non-invasive ventilation helps patients in respiratory failure. J Emerg Med Serv 2008;33:78-83. http://dx.doi.org/10.1016/S0197-2510(08)70050-0.
- Goss JF, Zygowiec J. Positive pressure: CPAP in the treatment of pulmonary edema & COPD. J Emerg Med Serv 2006;31:48-5. http://dx.doi.org/10.1016/S0197-2510(06)70558-7.
- Hastings D, Monahan J, Gray C, Pavlakovich D, Bartram P. CPAP. A supportive adjunct for congestive heart failure in the prehospital setting. J Emerg Med Serv 1998;23:58-65.
- Soo Hoo GW, Santiago S, Williams AJ. Nasal mechanical ventilation for hypercapnic respiratory-failure in chronic obstructive pulmonary-disease – determinants of success and failure. Crit Care Med 1994;22:1253-61. http://dx.doi.org/10.1097/00003246-199408000-00009.
- Kelly CA, Newby DE, McDonagh TA, Mackay TW, Barr J, Boon NA, et al. Randomised controlled trial of continuous positive airway pressure and standard oxygen therapy in acute pulmonary oedema; effects on plasma brain natriuretic peptide concentrations. Eur Heart J 2002;23:1379-86. http://dx.doi.org/10.1053/euhj.2001.3156.
- Klemen P, Golub M, Grmec S. Combination of quantitative capnometry, N-terminal pro-brain natriuretic peptide, and clinical assessment in differentiating acute heart failure from pulmonary disease as cause of acute dyspnea in pre-hospital emergency setting: study of diagnostic accuracy. Croat Med J 2009;50:133-42. http://dx.doi.org/10.3325/cmj.2009.50.133.
- Kosowsky JM, Zane R. Bilevel positive airway pressure for presumed pulmonary edema. Acad Emerg Med 2001;8:299-300. http://dx.doi.org/10.1111/j.1553-2712.2001.tb01314.x.
- Lightner L, Brywczynski J, McKinney J, Slovis CM. Shortness of breath: prehospital treatment of respiratory distress. JEMS 2010;35. http://dx.doi.org/10.1016/S0197-2510(10)70122-4.
- Lobato SD, Alises SM, Montiel G. Non-invasive mechanical ventilation in out-of-hospital emergency departments. Med Clin 2012;139. http://dx.doi.org/10.1016/j.medcli.2012.03.026.
- Maraffi T, Brambilla AM, Aliberti S, Pelosi P, Nava S, Tarsia P. A Multicenter Randomized Controlled Trial to Evaluate Continuous-Positive Airway Pressure (CPAP) Versus Conventional Oxygen in Patients With Acute Respiratory Failure (ARF) Due to Community-Acquired Pneumonia (CAP) n.d.
- Mattera CJ. Non-invasive pressure support ventilation. JEMS 1998;23:62-5.
- Moritz F, Benichou J, Vanheste M, Richard JC, Line S, Hellot MF, et al. Boussignac continuous positive airway pressure device in the emergency care of acute cardiogenic pulmonary oedema: a randomized pilot study. Eur J Emerg Med 2003;10:204-8. http://dx.doi.org/10.1097/00063110-200309000-00009.
- Navalesi P, Pollini A. Acute respiratory failure in patients with severe community-acquired pneumonia: a prospective randomized evaluation of noninvasive ventilation. Am J Respir Crit Care Med 2000;162:761-2. http://dx.doi.org/10.1164/ajrccm.162.2.16223c.
- Oliver CM, Narayanan M. Out-of-hospital CPAP in the treatment of cardiogenic pulmonary oedema. J Intensive Care Soc 2013;14:176-7. http://dx.doi.org/10.1177/175114371301400220.
- Roggla G, Moser B, Moser B. Non-invasive ventilation in prehospital settings. Intern Emerg Med 2013;8. http://dx.doi.org/10.1007/s11739-013-0909-y.
- Soma T, Hino M, Kida K, Kudoh S. A prospective and randomized study for improvement of acute asthma by non-invasive positive pressure ventilation (NPPV). Intern Med 2008;47:493-501. http://dx.doi.org/10.2169/internalmedicine.47.0429.
- Taylor DM, Bernard SA, Masci K, MacBean CE, Kennedy MP, Zalstein S. Prehospital noninvasive ventilation: A viable treatment option in the urban setting. Prehosp Emerg Care 2008;12:42-5. http://dx.doi.org/10.1080/10903120701710389.
- Templier F, Conchon G, Baer M, Chauvin M, Fletcher D. Non-invasive positive pressure ventilation for prehospital treatment of acute hypercapnic respiratory failure in a patient with chronic respiratory disease. JEUR 2002;15:104-8.
- Templier F, Kabayadondo MG, Thys F. The use of the Boussignac CPAP device during cardiogenic pulmonary edema (CPE): why add air to the oxygen gas source?. Intensive Care Med 2011;37:1555-7. http://dx.doi.org/10.1007/s00134-011-2278-1.
- Templier F, Labastire L, Pes P, Berthier F, Le CP, Thys F. Noninvasive ventilation use in French out-of-hospital settings: a preliminary national survey. Am J Emerg Med 2012;30:765-9. http://dx.doi.org/10.1016/j.ajem.2011.03.012.
- Thys F, Roeseler J, Reynaert M, Liistro G, Rodenstein DO. Noninvasive ventilation for acute respiratory failure: a prospective randomised placebo-controlled trial. Eur Respir J 2002;20:545-55. http://dx.doi.org/10.1183/09031936.02.00287402.
- Valipour A, Cozzarini W, Burghuber OC. Non-invasive pressure support ventilation in patients with respiratory failure due to severe acute cardiogenic pulmonary edema. Respiration 2004;71:144-51. http://dx.doi.org/10.1159/000076675.
Appendix 1 Literature search strategies for the review of clinical effectiveness: a MEDLINE example
Ovid MEDLINE(R) In-Process & Other Non-Indexed Citations and Ovid MEDLINE
Provider: OvidSP.
Date range searched: from 1948 to August 2013.
Date of search: August 2013.
-
non-invasive ventilation.ti,ab.
-
non invasive ventilation.ti,ab.
-
noninvasive ventilation.ti,ab.
-
NIV.ti,ab.
-
non-invasive positive pressure ventilation.ti,ab.
-
non invasive positive pressure ventilation.ti,ab.
-
noninvasive positive pressure ventilation.ti,ab.
-
NIPPV.ti,ab.
-
exp Positive-Pressure Respiration/
-
exp Continuous Positive Airway Pressure/
-
continuous positive airway pressure.ti,ab.
-
CPAP.ti,ab.
-
bi-level positive airway pressure.ti,ab.
-
bi level positive airway pressure.ti,ab.
-
bilevel positive airway pressure.ti,ab.
-
BIPAP.ti,ab.
-
non-invasive ventilatory support.ti,ab.
-
non invasive ventilatory support.ti,ab.
-
noninvasive ventilatory support.ti,ab.
-
bag-valve-mask ventilat$.ti,ab.
-
bag valve mask ventilat$.ti,ab.
-
bvm.ti,ab.
-
or/1-22
-
pre-hospital.ti,ab.
-
pre hospital.ti,ab.
-
prehospital.ti,ab.
-
exp Ambulances/
-
ambulance$.ti,ab.
-
community.ti,ab.
-
out of hospital.ti,ab.
-
before hospital.ti,ab.
-
(prior adj5 hospital).ti,ab.
-
paramedic$.ti,ab.
-
exp Emergency Medical Services/
-
emergency medical service$.ti,ab.
-
emergency care.ti,ab.
-
emergency health service$.ti,ab.
-
ems.ti,ab.
-
exp Ambulatory Care/
-
ambulatory care.ab,ti.
-
mobile intensive care unit$.ti,ab.
-
micu.ti,ab.
-
mobile intensive care ambulance$.ti,ab.
-
mica$.ti,ab.
-
Emergency Medical Technicians/
-
paramedic$.ab,ti.
-
(emergency adj3 technician$).ab,ti.
-
emt.ab,ti.
-
or/24-48
-
23 and 49
Appendix 2 Methodological assessment (adapted) criteria for randomised controlled trials
Criteria | Criteria met | Criteria defined (if applicable) | |
---|---|---|---|
1 | Was the method used to assign participants to the treatment groups really random? | Yes | Computer-generated random numbers, random number tables, random-permuted blocks, sealed assignment, sequentially numbered sealed opaque envelopes |
No | Use of alternation, case record numbers, date of birth or days of the week | ||
Unclear | Insufficient detail to make judgement | ||
2 | Was the allocation of treatment concealed? | Yes | Allocation to each group performed adequately (e.g. centrally, sequentially numbered, sealed opaque envelopes) |
No | Group assignment based on day of admission, case record numbers, date of birth or day of the week, open random number lists, non-opaque sealed envelopes | ||
Unclear | Insufficient detail to make judgement | ||
3 | Were the outcome assessors/data analysts blinded to the treatment allocations (it was not considered plausible that patients could be blinded to these types of interventions)? | Yes | Independent outcome assessors and data analysts were blinded to which group the patient belongs to |
No | Outcomes assessed and data analysed by those involved in the intervention, or those who are aware of group membership | ||
Unclear | Insufficient detail to make judgement | ||
4 | Were the eligibility criteria for study entry specified? | Yes | Eligibility criteria for study entry specified |
No | Eligibility criteria for study entry not specified | ||
Unclear | Insufficient detail to make judgement | ||
5 | Was baseline comparability achieved for the most important prognostic indicators? | Yes | The baseline characteristics of each study group (in particular age, diagnosis and/or physiological characteristics) were clearly outlined and any differences identified were accounted for |
No | The baseline characteristics (in particular age, diagnosis and/or physiological characteristics) of each study group were not outlined or accounted for | ||
Unclear | Insufficient detail to make judgement | ||
6 | Adequate follow-up of patients (at least 80%) | Yes | Proportion and characteristics of those participants lost to follow-up (≤ 20%) clearly reported for each group and outcome. A clear outline is provided as to how losses of participants were handled |
No | Proportion and characteristics of those participants lost to follow-up more than 20%. No clear outline is provided as to how losses of participants were handled | ||
Unclear | Insufficient detail to make judgement | ||
7 | Were the reasons for withdrawal stated? | Yes | Reasons for withdrawal were stated |
No | Reasons for withdrawal were not stated | ||
Unclear | Insufficient detail to make judgement | ||
8 | Was an intention-to-treat analysis included? | Yes | All patients assigned to one of the treatments are analysed together, regardless of whether or not they completed or received that treatment |
No | All patients assigned to one of the treatments are not analysed together, regardless of whether or not they completed or received that treatment (e.g. per protocol) | ||
Unclear | Insufficient detail to make judgement | ||
9 | Was the study powered to detect differences in outcomes? | Yes | A power calculation was performed and reported. The study was adequately powered to detect differences in outcomes |
No | A power calculation was not performed. A power calculation was performed and reported but the study was not adequately powered to detect differences in outcomes. A power calculation was performed but not reported, the study states it was adequately powered to detect differences in outcomes | ||
Unclear | Insufficient detail to make judgement |
Appendix 3 Network meta-analysis model and sensitivity analyses
We let rik be the number of events out of the total number of patients in each arm, nik, for arm k and trial i. We assume that the data follow a binomial distribution such that:
where pik represents the probability of an event in arm k of trial i.
We use the logit link function to map the probabilities on to the real line. We then define:
where
The μi are trial-specific baselines representing the log-odds of an event in the control treatment, and the δi,1k are the trial-specific log–ORs of an event in the treatment group relative to the control.
We assume that the trial-specific treatment effects of the treatment in arm k, relative to the control treatment (in arm 1), are drawn from a common random-effects distribution such that:
where dti1,tik represents the population effect of the treatment in arm k in trial i, tik, compared with the treatment in arm 1 of trial i, ti1, and τ2 represents the between-trial variance in treatment effects (heterogeneity).
The basic parameters for the treatment effects are d1t, the effect of treatment t (t = BiPAP, CPAP) relative to the reference treatment 1 (defined as usual care).
The model is completed by giving the trial-specific baselines, the basic parameters and the between-trial standard deviations prior distributions such that:
The prior distribution for τ has mean 0.32 and 95% CrI 0.01 to 0.91. This suggests that we believe, a priori, that it is most likely there is mild heterogeneity in intervention effects between trials, but that we allow for the possibility that there could be moderate to extreme heterogeneity in intervention effects between trials.
The common reference prior distribution that is used for the between-trials standard deviation, and the one that we used in our initial analysis, is a uniform (U) prior distribution on the interval 0 to 2, that is, U(0,2). This means that we believe a priori that there may be extreme heterogeneity in intervention effects between trials, and the range of plausible values means that the heterogeneity could be huge.
There appeared to be relatively little Bayesian updating of the prior distribution to the posterior distribution of the between-trials standard deviation (probably as a consequence of there being relatively few studies). Given that prior distributions should reflect genuine prior beliefs unless there is a reasonable number of sample data, or else posterior distributions will not reflect genuine posterior beliefs, we used a half-normal (HN) prior distribution [i.e. HN(0,0.16)] in our main analyses. Results of the analyses using a U(0,2) prior distribution are presented below.
Treatment | Random-effects mean | Predictive distribution | Probability most effective | ||
---|---|---|---|---|---|
OR | 95% CrI | OR | 95% CrI | ||
NIV | |||||
BiPAP | 1.89 | 0.48 to 7.18 | 1.91 | 0.21 to 17.15 | 0.000 |
CPAP | 0.38 | 0.13 to 0.91 | 0.39 | 0.05 to 2.51 | 1.000 |
Usual carea | |||||
Reference | Reference | Reference | Reference | Reference | 0.000 |
Between-study standard deviation | 0.61 | 0.04 to 1.71 | – | – | – |
Treatment | Random-effects mean | Predictive distribution | Probability most effective | ||
---|---|---|---|---|---|
OR | 95% CrI | OR | 95% CrI | ||
NIV | |||||
BiPAP | 0.42 | 0.12 to 1.51 | 0.42 | 0.06 to 2.97 | 0.352 |
CPAP | 0.32 | 0.14 to 0.77 | 0.32 | 0.06 to 1.82 | 0.647 |
Usual carea | |||||
Reference | Reference | Reference | Reference | Reference | 0.001 |
Between-study standard deviation | 0.43 | 0.03 to 1.62 | – | – | – |
Appendix 4 Network meta-analysis model including treatment effect modifiers
Let j be the study where j = 1,. . ., NSIPD, and NSIPD is the number of IPD studies included in the NMA. Let yijk = 1 if the ith patient in the jth study on treatment k experiences the event and yijk = 0 if the ith patient in the jth study on treatment k does not experience the event, where i = 1,. . . . , Nj, such that Nj is the number of patients in the jth study. Assume that the outcomes of patients yijk, are independent and identically distributed as yijk∼ Bernoulli (pjk), where pjk is the probability of an event for a patient in the jth study on treatment k. The settings for the aggregate data studies are the same as in Appendix 6, except for the mortality discrepancies noted in Chapter 3, Network meta-analysis using combined individual patient-level data and aggregate data.
Suppose βXY is the regression coefficient for the interaction for the comparison of treatment Y relative to treatment X. The regression coefficients corresponding to the basic parameters (e.g. βAB, βAC) are estimated by the model and are used to estimate the remaining regression coefficient (e.g. βBC = βAC – βAB).
The likelihood function for IPD studies is:
where βAA = 0; j = 1,. . . , NSIPD; xik is a patient-level covariate for the ith patient in the jth study on treatment k; and β0jb is a study-specific regression parameter that represents the difference in the log-odds of an event in treatment group b per unit increase in the covariate.
The likelihood function for aggregate data studies is:
where βAA = 0 and j = (NSIPD + 1),. . ., (NSIPD + NSAD).
The study-specific treatment effect δjbk is assumed from the following distribution.
where dAA = 0, dbk represents the mean log-OR of k versus b when the covariate value is zero (i.e. xijk = zj = 0) and τ2 represents the between-trial variance in treatment effects (heterogeneity).
The regression coefficient βAk is assumed to be exchangeable:
The model is completed by giving the trial-specific baselines, the basic parameters and the between-trial standard deviations prior distributions such that:
Appendix 5 Clinical effectiveness review: table of excluded studies with rationale
Author, year | Reason for exclusion |
---|---|
Aguilar et al., 201159 (abstract) | Non-randomised observational study (full paper reported in Aguilar et al. 2013)60 |
Aguilar et al., 201360 | Non-randomised observational study |
Fort PA, 201256 | Ongoing study (VeNIS BPCO trial) – due for completion in December 2014 |
Austin et al., 201395 | Original abstract included (multiple report) |
Baker, 198396 | Review/comment/editorial |
Baker, 200597 | Review/comment/editorial |
Berteloot et al., 2011 (abstract)61 | Non-randomised observational study |
Bledsoe et al., 201262 | Non-randomised observational study |
Bohanske et al., 201098 | Observational study with no relevant outcome data |
Bott et al., 199399 | In-hospital NIV (RCT) |
Bruge et al., 200863 | Non-randomised observational study |
Bultman et al., 200564 (abstract) | Non-randomised observational study |
Cheskes et al., 2012100 | Observational study with no relevant outcome data |
Confalonieri et al., 1999101 | In-hospital NIV (RCT) |
Crawford et al., 2008102 | In-hospital NIV (RCT) |
Cuny et al., 2013 (abstract)65 | Non-randomised observational study |
Derr et al., 200666 | Non-randomised observational study |
Dib et al., 201267 | Non-randomised observational study |
Dieperink et al., 200968 | Non-randomised observational study |
Ducros et al., 2008103 (abstract) | Full peer-reviewed paper included |
Foti et al., 200969 | Non-randomised observational study |
Freitas et al., 201070 (abstract) | Non-randomised observational study |
Fyntanidou et al., 200971 (abstract) | Non-randomised observational study |
Gardtman et al., 2000104 | Observational study with no relevant intervention |
Garuti et al., 201072 | Non-randomised observational study |
Gonzva et al., 2013105 | Observational study with no relevant outcome data |
Goss, 2008106 | Review/comment/editorial |
Goss and Zygowiec, 2006107 | Review/comment/editorial |
Grosomanidis et al., 200073 (abstract) | Non-randomised observational study |
Hastings et al., 1998108 | Review/comment/editorial |
Soo Hoo et al., 1994109 | In-hospital NIV (observational study) |
Hubble et al., 200674 | Non-randomised observational study |
Kallio et al., 200375 | Non-randomised observational study |
Kelly et al., 2002110 | In-hospital NIV (RCT) |
Klemen et al., 2009111 | Observational study with no relevant intervention |
Kosowsky and Zane, 2001112 | Review/comment/editorial |
Kosowsky et al., 200176 | Non-randomised observational study |
Lightner et al., 2010113 | Review/comment/editorial |
Lobato et al., 2012114 | Review/comment/editorial |
Maraffi et al., 2009115 | In-hospital NIV (RCT) |
Mattera, 1998116 | Review/comment/editorial |
Moritz et al., 2003117 | In-hospital NIV (RCT) |
Navalesi and Pollini, 2000118 | Review/comment/editorial |
Oliver and Narayanan, 2013119 | Review/comment/editorial |
Roggla et al., 2013120 | Review/comment/editorial |
Soma et al., 2008121 | In-hospital NIV (RCT) |
Spijker et al., 201377 | Non-randomised observational study |
Taylor et al., 2008122 | Observational study with no relevant intervention |
Templier et al., 2002123 | Observational study with no relevant outcome data |
Templier et al., 200378 | Non-randomised observational study |
Templier et al., 2011124 | Review/comment/editorial |
Templier et al., 2012125 | Observational study with no relevant outcome data |
Thys et al., 2002126 | In-hospital NIV (RCT) |
Valipour et al., 2004127 | In-hospital NIV (observational study) |
Warner, 201079 | Non-randomised observational study |
Appendix 6 Summary of trials included in the base-case network meta-analysis of pre-hospital non-invasive ventilation for acute respiratory failure
Author, year | Number of intubations | Mortality (within 30 days) | ||||||
---|---|---|---|---|---|---|---|---|
Intervention | Control | Intervention | Control | |||||
Events | Total | Events | Total | Events | Total | Events | Total | |
Studies evaluating CPAP | ||||||||
Austin and Wills, 201246 (abstract) | NR | NR | NR | NR | 1 | 24 | 9 | 26 |
aDucros et al., 201147 | 3 | 107 | 6 | 100 | 8 | 107 | 9 | 100 |
aFrontin et al., 201148 | 2 | 60 | 3 | 62 | 6 | 60 | 7 | 62 |
aPlaisance et al., 200750 | 6 | 63 | 16 | 61 | 2 | 63 | 8 | 61 |
Schmidbauer et al., 201152 | 3 | 18 | 7 | 18 | 0 | 18 | 0 | 18 |
aThompson et al., 200853 | 7 | 35 | 17 | 34 | 5 | 35 | 12 | 34 |
Studies evaluating BiPAP | ||||||||
aMas et al., 200249 (abstract) | 3 | 28 | 1 | 28 | 6 | 28 | 2 | 28 |
aRoessler et al., 201251 | 1 | 24 | 6 | 25 | 1 | 24 | 2 | 25 |
bCraven et al., 200054 | 4 | 37 | 7 | 25 | 6 | 37 | 2 | 24 |
a,bWeitz et al., 200755 | NR | NR | NR | NR | 1 | 10 | 1 | 13 |
Appendix 7 Literature search strategies for the review of cost-effectiveness: a MEDLINE example
Ovid MEDLINE(R) In-Process & Other Non-Indexed Citations and Ovid MEDLINE(R)
Provider: OvidSP.
Date range searched: from 1948 to August 2013.
Date searched: August 2013.
-
non-invasive ventilation.ti,ab.
-
non invasive ventilation.ti,ab.
-
noninvasive ventilation.ti,ab.
-
NIV.ti,ab.
-
non-invasive positive pressure ventilation.ti,ab.
-
non invasive positive pressure ventilation.ti,ab.
-
noninvasive positive pressure ventilation.ti,ab.
-
NIPPV.ti,ab.
-
exp Positive-Pressure Respiration/
-
exp Continuous Positive Airway Pressure/
-
continuous positive airway pressure.ti,ab.
-
CPAP.ti,ab.
-
bi-level positive airway pressure.ti,ab.
-
bi level positive airway pressure.ti,ab.
-
bilevel positive airway pressure.ti,ab.
-
BIPAP.ti,ab.
-
non-invasive ventilatory support.ti,ab.
-
non invasive ventilatory support.ti,ab.
-
noninvasive ventilatory support.ti,ab.
-
bag-valve-mask ventilat$.ti,ab.
-
bag valve mask ventilat$.ti,ab.
-
bvm.ti,ab.
-
or/1-22
-
pre-hospital.ti,ab.
-
pre hospital.ti,ab.
-
prehospital.ti,ab.
-
exp Ambulances/
-
ambulance$.ti,ab.
-
community.ti,ab.
-
out of hospital.ti,ab.
-
before hospital.ti,ab.
-
(prior adj5 hospital).ti,ab.
-
paramedic$.ti,ab.
-
exp Emergency Medical Services/
-
emergency medical service$.ti,ab.
-
emergency care.ti,ab.
-
emergency health service$.ti,ab.
-
ems.ti,ab.
-
exp Ambulatory Care/
-
ambulatory care.ab,ti.
-
mobile intensive care unit$.ti,ab.
-
micu.ti,ab.
-
mobile intensive care ambulance$.ti,ab.
-
mica$.ti,ab.
-
Emergency Medical Technicians/
-
paramedic$.ab,ti. (5008)
-
(emergency adj3 technician$).ab,ti.
-
emt.ab,ti.
-
or/24-48
-
23 and 49
-
Economics/
-
“costs and cost analysis”/
-
Cost allocation/
-
Cost-benefit analysis/
-
Cost control/
-
cost savings/
-
Cost of illness/
-
Cost sharing/
-
“deductibles and coinsurance”/
-
Health care costs/
-
Direct service costs/
-
Drug costs/
-
Employer health costs/
-
Hospital costs/
-
Health expenditures/
-
Capital expenditures/
-
Value of life/
-
exp economics, hospital/
-
exp economics, medical/
-
Economics, nursing/
-
Economics, pharmaceutical/
-
exp "fees and charges"/
-
exp budgets/
-
(low adj cost).mp.
-
(high adj cost).mp.
-
(health?care adj cost$).mp.
-
(fiscal or funding or financial or finance).tw.
-
(cost adj estimate$).mp.
-
(cost adj variable).mp.
-
(unit adj cost$).mp.
-
(economic$ or pharmacoeconomic$ or price$ or pricing).tw.
-
or/51-81
-
50 and 82
Appendix 8 Effect of distance on mortality
The effect of distance on mortality of emergency admissions for respiratory illness can be observed in the large cohort data set of 668 patients who presented with ‘respiratory disease’ across four ambulance services over a 4-year period from 1997 to 2001. This relationship can be observed in the raw data, which shows an increase in mortality with an increase in the distance to hospital. The relationship between ambulance journey time and mortality of emergency admissions for respiratory disease was also modelled but no clear pattern was observed. This could be because journey times depend on the accuracy and consistency with which times of leaving the scene and arrival at hospital are recorded, and they can also be affected by ‘reverse causation’, which occurs when the patient’s condition is a cause of the journey time rather than vice versa, such as when ambulances drive as fast as possible to hospital for critically ill patients but slowly and with less risk for patients not critically ill.
Table 27 below shows mortality variation with distances, categorised as short (< 10 km), medium (10–20 km) and long (> 20 km), with longer distances associated with higher mortality. The results match the original analysis for patients with respiratory disease. 6
Distance (km) | Total number of patients | Deaths | Mortality rate | Distribution |
---|---|---|---|---|
1–10 | 536 | 58 | 0.108 | Beta(58,468) |
11–20 | 93 | 13 | 0.139 | Beta(13,80) |
21–30 | 39 | 8 | 0.205 | Beta(8,31) |
The split of patients by distance in the different scenarios are shown in Table 28. For each scenario, the overall mortality rate is estimated by multiplying by the probability of death in each distance category by the proportion of patients in that distance category.
Distance (km) | General population scenario | Rural population scenario | Urban population scenario |
---|---|---|---|
1–10 | 0.802 | 0.598 | 0.971 |
11–20 | 0.139 | 0.291 | 0.015 |
> 20 | 0.058 | 0.110 | 0.013 |
Glossary
- Bilevel positive airway pressure
- A system of respiratory support used during non-invasive ventilation in which preset levels of inspiratory and expiratory positive airway pressure are applied. The pressure is higher when a person breathes in and is lower when a person breathes out.
- Continuous positive airway pressure
- A system of respiratory support used during non-invasive ventilation in which a preset constant pressure is applied during inspiration and expiration.
- Cost-effectiveness acceptability curve
- A way of illustrating cost-effectiveness results by plotting the probability that the intervention is cost-effective (y-axis) against the maximum that society is willing to pay for an improvement in health (x-axis).
- Cost-effectiveness plane
- A way of illustrating cost-effectiveness results by plotting the mean incremental cost and effectiveness on a four-quadrant graph. Interventions that are more costly and more effective fall in the north-east quadrant.
- Incremental cost-effectiveness ratio
- The difference in costs between one intervention and an alternative, divided by the difference in outcomes.
- Meta-analysis
- A statistical technique for combining (pooling) the results of a number of studies that address the same question and report on the same outcomes to produce a summary result. The aim is to derive more precise and clear information from a large data pool. It is generally more reliably likely to confirm or refute a hypothesis than the individual trials.
- Non-invasive ventilation
- A method of delivering ventilatory support through a tight-fitting mask, which is usually applied around a person’s mouth and nose. It may take the form of continuous positive airway pressure or bilevel inspiratory positive airway pressure.
- Quality-adjusted life-year
- A measure of the benefit of health care that combines the impact of the expected length of life and quality of life.
- Respiratory failure
- A condition in which the heart and lungs fail to maintain one or both of its gas exchange functions: oxygenation and carbon dioxide elimination. Respiratory failure can be acute (develops within minutes or hours in patients with no, or minor, evidence of pre-existing respiratory disease), acute on chronic (an acute deterioration in an individual with pre-existing respiratory failure) or chronic (develops over several days, or longer, in patients with existing respiratory disease).
List of abbreviations
- 3CPO
- Three Interventions in Cardiogenic Pulmonary Oedema
- ACPO
- acute cardiogenic pulmonary oedema
- BIOSIS
- Bioscience Information Service
- BiPAP
- bilevel inspiratory positive airway pressure
- CEAC
- cost-effectiveness acceptability curve
- CI
- confidence interval
- COPD
- chronic obstructive pulmonary disease
- CPAP
- continuous positive airway pressure
- CrI
- credible interval
- DIC
- deviance information criterion
- EPAP
- expiratory positive airway pressure
- EVPI
- expected value of perfect information
- EVPPI
- expected value of partial perfect information
- EVSI
- expected value of sample information
- FiO2
- fraction of inspired oxygen
- HN
- half-normal distribution
- ICER
- incremental cost-effectiveness ratio
- IPAP
- inspiratory positive airway pressure
- IPD
- individual patient-level data
- JRCALC
- Joint Royal Colleges Ambulance Liaison Committee
- NICE
- National Institute for Health and Care Excellence
- NIHR
- National Institute for Health Research
- NIV
- non-invasive ventilation
- NMA
- network meta-analysis
- OR
- odds ratio
- PaCO2
- arterial carbon dioxide tension (partial pressure of carbon dioxide)
- PaO2
- arterial oxygen tension (partial pressure of oxygen)
- PEEP
- positive end-expiratory pressure
- QALY
- quality-adjusted life-year
- RCT
- randomised controlled trial
- SpO2
- oxygen saturation (as determined by pulse oximetry)