Notes
Article history
The research reported in this issue of the journal was funded by the HTA programme as project number 12/47/01. The contractual start date was in April 2013. The draft report began editorial review in April 2014 and was accepted for publication in February 2015. The authors have been wholly responsible for all data collection, analysis and interpretation, and for writing up their work. The HTA editors and publisher have tried to ensure the accuracy of the authors’ report and would like to thank the reviewers for their constructive comments on the draft document. However, they do not accept liability for damages or losses arising from material published in this report.
Declared competing interests of authors
none
Permissions
Copyright statement
© Queen’s Printer and Controller of HMSO 2015. This work was produced by Beale et al. under the terms of a commissioning contract issued by the Secretary of State for Health. This issue may be freely reproduced for the purposes of private research and study and extracts (or indeed, the full report) may be included in professional journals provided that suitable acknowledgement is made and the reproduction is not associated with any form of advertising. Applications for commercial reproduction should be addressed to: NIHR Journals Library, National Institute for Health Research, Evaluation, Trials and Studies Coordinating Centre, Alpha House, University of Southampton Science Park, Southampton SO16 7NS, UK.
Chapter 1 Introduction
Genetic testing allows the accurate diagnosis of genetic disorders along with disease prediction, carrier testing and screening. The term ‘genetic test’ is used to describe a particular genetic variant (or set of variants) associated with a particular disease; the test therefore relies on prior knowledge of the causal mutation. 1 The 2012 NHS Directory of Genetic Testing2 reports that UK genetic testing network laboratories offer tests for 519 diseases and that new tests for 48 diseases are currently being set up within the NHS. The number of tests available is rising year on year as new gene–disease associations are identified.
Array comparative genomic hybridisation (aCGH) is recognised as a technology that is capable of replacing standard diagnostic methodologies and enhancing NHS diagnostic services. 3 To facilitate the implementation of aCGH technology for genetic testing of learning disabilities (LDs) into the NHS, the UK Genetic Testing Network (UKGTN) invited specialised services commissioners and directors of NHS cytogenetic laboratories to an expert workshop in November 2009. There was universal agreement amongst attendees that the evidence base for the use of aCGH as a first-line test (i.e. replacing traditional karyotyping methodologies) in LDs was sound, robust and conclusive. 3 However, this approach can miss certain chromosomal abnormalities. Also, its ability to detect new variations of unknown significance can generate a large quantity of false-positive results. 4 Nevertheless, Regier et al. 5 have found it to be cost-effective for diagnosing intellectual disabilities (IDs) when used instead of conventional karyotyping.
Next-generation sequencing (NGS) allows the simultaneous testing of all genes (from a standard venous blood sample). It can be used in three different ways:
-
targeted gene sequencing – sequencing of pre-identified genes (e.g. the set of genes known to be associated with LDs)
-
whole-exome sequencing (WES) – sequencing of all genes
-
whole-genome sequencing (WGS) – sequencing of all genes and also the deoxyribonucleic acid (DNA) between genes.
Next-generation sequencing technologies have the potential to facilitate a rapid diagnosis. However, in terms of diagnosing LDs, NGS is currently being used only in research and the best way to use it in practice has not yet been identified.
The National Institute for Health Research (NIHR) has commissioned the Liverpool Reviews and Implementation Group (LRiG), part of the University of Liverpool, to carry out this scoping study to consider the cost-effectiveness of NGS compared with traditional genetic testing for the diagnosis of LDs in children. This study is part of the NIHR’s Health Technology Assessment (HTA) programme (project number 12/47/01).
Background
Learning disability is a serious and lifelong condition characterised by the impairment of cognitive and adaptive skills. It may be caused by genetic, environmental, infectious or perinatal factors. However, it is not clear how many of the cases with unidentified causes are linked to genetic rather than other factors.
Definition of patient group
Valuing People,6 the 2001 White Paper on the health and social care of people with LDs, includes the following definition (p. 14):
Learning disability includes the presence of:
-
a significantly reduced ability to understand new or complex information, to learn new skills (impaired intelligence), with;
-
a reduced ability to cope independently (impaired social functioning);
-
which started before adulthood, with a lasting effect on development.
This definition is broadly consistent with that used in the current version of the World Health Organization’s International Classification of Diseases, 10th Revision (ICD-10), although the ICD-10 uses the term ‘mental retardation’ (MR). 7,8 International Classification of Diseases, 11th Revision is in development (due by 2017) and it has been suggested that this publication is likely to use the term ‘ID’ to replace both MR and LD.
Mir et al. 9 explain that the term ‘learning difficulties’ is the preferred term amongst user organisations and disability writers, whereas ‘learning disabilities’ is generally used by service organisations. In this scoping study the abbreviation LD is used throughout to denote ‘learning disability’.
However, to add further ambiguity, a number of other terms included in published papers and other documents are used synonymously with, or include the definition of, LD. These include ‘mental handicap’, ‘ID’ and ‘developmental delay’ (DD). The term ‘DD’ is sometimes applied to younger children for whom a diagnosis of LD or MR cannot be established because the child is not yet old enough for a reliable cognitive assessment to be performed. However, the term ‘DD’ can be criticised for implying that ‘developmental catch-up’ might, or will, occur; for the vast majority of children who are tested this will not happen as they have a permanent LD. Some people prefer to use the term ‘DD’ for ‘developmental disability’ whereas others favour ‘disordered development’ (i.e. following a different developmental pathway) or ‘early development impairment’ (for children under the age of 4 years for whom accurate assessment of the degree of LD is very difficult). Sometimes the term ‘learning and developmental disability’ (LDD) is used to encompass both DD in younger children and LD in older individuals (e.g. by Newman et al. 10). However, in UK education circles, LDD specifically means ‘learning difficulties and disabilities’ rather than ‘learning and developmental disability’. Other terms used in the published literature include ‘global developmental delay’ (GDD), ‘cognitive impairment’ and ‘idiopathic learning disability’ (ILD), with ILDs being LDs with no known cause. An author from the USA11 describes ‘development disabilities’ as including ‘congenital malformations, cognitive impairment, and behavioural abnormalities’ (p. 121).
Different terminology is used for similar conditions in different countries, at different times and/or in different situations. For example, ‘mental handicap’ was widely used in Britain but is now considered to have inappropriate and negative connotations. MR is considered offensive in Britain but not in the USA, although it has now been replaced by ID.
Sometimes the terms ‘autism spectrum disorder’ (ASD) and ‘multiple congenital anomalies’ (MCA) are used when researching and discussing LDs. The inclusion of children with ASD in a study of a particular technology for diagnosing LDs may have a significant effect on its identified diagnostic yield because about 50% of people with autism also have an ID. Interest in, and research on, ASD has increased in recent years. Temporal comparisons of diagnostic yields for LDs from different technologies may be compromised if the cohort of children undergoing testing also includes a significant proportion of children with ASD (although the boundaries between LD and ASD are not clear-cut).
When discussing the findings of published papers, this report uses the terminology chosen by the authors of the paper (even if it may sometimes be considered offensive by some readers of this report). Elsewhere in this report, the terms LD and DD are used to encompass learning disabilities (as defined in Valuing People6) and to capture DDs developmental impairment in younger children respectively.
Prevalence
Various attempts have been made to determine the number of children with disabilities, including LDs; however, these have had limited success. 12,13 The report of the independent inquiry into access to health care for people with learning disabilities, Healthcare for All,14 suggests that around 2.5% of the UK population has a LD. Emerson and Hatton15 used two different approaches to estimate prevalence in England. The first involved extrapolating from a subset of locally held LD ‘registers’ (the estimated administrative prevalence) whereas the second made adjustments to the ‘register’ figures, which took into account the fact that these figures are likely to include only those with severe LDs (estimated true prevalence). Prevalence figures by age group are presented in Table 1 and estimated numbers of children with LDs, calculated using Office for National Statistics subnational population projections for 2012,16 are displayed in Table 2.
Diagnosis of learning disability
The first step in the diagnostic pathway occurs after someone (perhaps a professional, relative or friend) believes that a child may have a disabling condition or additional health needs. This concern may arise as part of a formal screening or surveillance programme. For example, it could occur pre birth if, for example, amniocentesis has shown possible Down syndrome; at birth for a condition with physical abnormalities; later during a consultation with a clinician for an unrelated purpose; or, in the case of less profound DD, when the child starts school.
A paediatrician generally carries out the assessment of LDs. The paediatrician will take a medical history as well as record physical measurements and assess the child’s developmental progress. Other health professionals may also be involved in the assessment, for example a child may be referred for a vision or hearing assessment or to a neurologist. 17
If the paediatrician suspects that the child’s condition may be linked to a genetic abnormality the child will be referred to a regionally based NHS clinical genetic service. The genetic service can use a number of diagnostic tools, including:18
-
family history taking
-
information gathering
-
medical history and examination
-
clinical investigation
-
genetic testing.
Results from a genetic test can generally be obtained within 4 months. Some NHS centres offer a turnaround time of 6 weeks for specific tests. Certain specialised tests can only be carried out abroad.
The clinical genetic service provides genetic counselling. The extent of counselling depends on the result of the genetic test. Sometimes it is appropriate to counsel parents only, but in other cases the wider family may need counselling and, perhaps, also testing. For example, if both parents are found to be carriers (i.e. each parent has one ‘normal’ and one defective gene) then siblings could also be silent carriers of the condition. Even if the siblings do not have the condition themselves, they could potentially pass the mutated gene to their children.
The value of a diagnosis
In 1995 the American College of Medical Genetics [which changed its name to the American College of Medical Genetics and Genomics (ACMG) in 2011] sponsored a conference in Minnesota, USA. The purpose of the conference was to use available literature and expert opinion to produce consensus recommendations on the evaluation of MR. The benefits of a genetic evaluation, as identified at the conference, are displayed in Table 3. 19
For the individual patient | For parents |
---|---|
|
|
In the UK, as part of a larger study looking at the implications of WGS for health, Gogarty20 used feedback from interviews (38 participants), a forum (46 registered members) and discussion groups (36 people) to develop recommendations for service change with regard to the clinical investigation of children with DD. Participants included parents, patients, carers and representatives from voluntary organisations. As part of the consultation exercise, parents were asked to describe some of the advantages and disadvantages of genetic testing for LD. Some of the most common responses are displayed in Table 4.
Advantages | Disadvantages |
---|---|
|
|
Ongoing research
There are currently three large-scale genetic research studies under way in England that are of particular relevance to children with LDs. All three are based in Cambridge.
-
the Genetics of Learning Disability (GOLD) study21 was established in 2001 and aims to identify the genes on the X chromosome that contribute to significant ID and to lead the way to a greater understanding of the mechanisms by which ID occurs
-
the Deciphering Developmental Disorders (DDD) study22 aims to advance clinical genetic practice for children with developmental disorders by the systematic application of the latest microarray and sequencing methods, to see whether these technologies can help doctors understand why patients get developmental disorders, and also to address the new ethical challenges raised
-
the Specialist Pathology Evaluating Exomes in Diagnosis (SPEED) project23 is a NIHR BioResource – Rare Diseases initiative. One of its immediate aims is to develop more affordable DNA-based testing of rare diseases for which the gene is known.
The aim and objectives of this scoping study
The aim of this project was to find out whether a diagnostic pathway based on NGS might be more cost-effective than current approaches to genetic testing for children with LDs.
The objectives of the project were to:
-
describe current pathways that involve the use of genetic testing
-
collect stakeholder views on the changes in service provision that would need to be put in place before NGS could be used in clinical practice
-
describe the new systems and safeguards that would need to be put in place before NGS could be used in clinical practice
-
explore the cost-effectiveness of using NGS compared with conventional genetic testing.
The structure of the report
Information collected from the literature and stakeholder interviews was used to address the study objectives. These objectives are presented in the following three chapters of this report, with supporting appendices when relevant. Table 5 shows the locations in the report where the study objectives are addressed and the methods that were used to address them.
Chapter number | Objective addressed | Method | Appendix | |
---|---|---|---|---|
Number | Content | |||
2 | Objective 1: describe current pathways that involve the use of genetic testing; objective 3: describe the new systems and safeguards that would need to be put in place before NGS could be used in clinical practice | Literature review | 2 | Literature search strategy |
3 | Effectiveness of traditional genetic testing methods | |||
3 | Objective 2: collect stakeholder views on the changes in service provision that would need to be put in place before NGS could be used in clinical practice | Stakeholder interviews | 4 | List of interviewees and the interview protocol |
5 | Additional interview quotations – changes in service provision | |||
6 | Value of a diagnosis | |||
3 | Objective 3: describe the new systems and safeguards that would need to be put in place before NGS could be used in clinical practice | Stakeholder interviews and literature review | 7 | Additional interview quotations – new systems and safeguards |
4 | Objective 4: explore the cost-effectiveness of using NGS compared with conventional genetic testing | Stakeholder interviews and literature review |
Research advisory group
A research advisory group (RAG) was established at the outset of the study to help shape the direction of the research (see Appendix 1 for a list of members). Members were asked to identify relevant studies and ongoing research projects and were invited to comment on the drafts for the interview specifications. They were also invited to attend a workshop, which was held approximately 2 weeks before the draft report was finalised. The purpose of the workshop was to:
-
share findings from the draft report, giving attendees the opportunity to provide comments and suggestions before the report was finalised
-
discuss implications for future practice
-
discuss priorities and opportunities for future research.
In addition, RAG members were asked to make themselves available from time to time to respond to ad hoc queries by e-mail.
Chapter 2 Literature review
Introduction
This chapter addresses the first objective of this scoping study (i.e. to describe current pathways that involve the use of genetic testing) using information from the literature. It also includes information on the opportunities and challenges associated with introducing NGS technologies into routine clinical practice, that is, information that partially addresses the third objective (to describe new systems and safeguards that would need to be put in place before NGS technologies could be used in clinical practice).
Methods
Scoping searches were conducted at the beginning of the study. These identified only a few useful studies. However, a number of relevant studies and reports were highlighted during the stakeholder interviews and further helpful studies were identified through hand-searching and searching of bibliographies of informative studies. In addition, the researchers received daily alerts and updates from GenomeWeb Daily News and the Daily Scan [see www.genomeweb.com (accessed 22 April 2015)], along with regular Genomics and Policy News updates from the Public Health Genetics (PHG) Foundation [see www.phgfoundation.org (accessed 22 April 2015)].
To ensure that no important publications had been overlooked, a comprehensive search strategy was developed during the final months of the study. This included additional search terms that had been identified as important during the course of the study. The following electronic databases were searched from January 2005 to February 2014: MEDLINE, EMBASE and The Cochrane Library. The search was restricted to papers published in the English language and included a combination of index terms and free-text words. All references were exported to the EndNote reference database (version X6; Thomson Reuters, Carlsbad, CA, USA). Details of the final search strategies used and the number of references retrieved for each search are provided in Appendix 2. Before acquiring papers, titles and abstracts were scanned by two reviewers and studies that were clearly irrelevant were removed. The remaining abstracts were scrutinised in relation to the required data and those papers that were not relevant were excluded. Once this sifting process had been completed, paper copies of the selected studies or reviews were acquired.
Results I: current pathways that involve the use of genetic testing
Traditional diagnostic methods
Introduction
Many children with DD or LD do not have physical features or a medical history that are sufficiently specific to facilitate a clear clinical diagnosis. The clinical assessment of children suspected of having DD or LD typically involves examination by a paediatrician followed by appropriate biochemical and haematological tests as well as chromosomal tests and other molecular genetic tests. Among such patients, the information gained from undertaking laboratory testing can be extremely helpful and is an integral component of the diagnostic evaluation. Until recently, tests such as chromosome analysis, metabolic screens and brain imaging were the mainstay of the work-up of the child with DD. If these tests were negative, the clinician was faced with testing for numerous different single gene disorders, such as Rett syndrome. These tests generally have a very poor diagnostic yield (i.e. < 1%, according to Lynch24). Investigations might be undertaken for many years, during which time the costs would continue to rise.
Chromosome analysis (karyotyping)
Karyotyping was the ‘gold standard’ test for detecting chromosomal abnormalities for many years, particularly in patients ascertained with DD (motor or growth), ASD and moderate to severe learning disabilities/difficulties, with or without dysmorphic features or congenital abnormalities. Karyotyping is a test used to examine the chromosomes in a sample of cells and can help identify genetic problems as the cause of a disorder or a disease. It pairs and orders all of the chromosomes in an organism, thus providing a genome-wide snapshot of an individual’s chromosomes. The test can be performed on almost any tissue, including blood, bone marrow and amniotic fluid. Karyotyping can detect large genomic imbalances (losses or gains of DNA) in LD conditions such as Down syndrome (caused by the presence of all or part of a third copy of chromosome 21 and also known as trisomy 21), Turner syndrome (a completely or partially missing X sex chromosome in females) and Edwards syndrome (also known as trisomy 18). However, the resolution is insufficient to routinely detect rearrangements smaller than 5 million base pairs and even anomalies of 15 million base pairs may be missed when the banding pattern is indistinct. In other words, karyotyping can detect only relatively large chromosomal abnormalities. Because small genomic imbalances can be clinically important, there was a demand for higher-resolution assays to detect them. This was particularly true for idiopathic LD cases, which represent about 15% of referrals to clinical genetics and paediatrics clinics. 25
Sometimes a karyotype test is combined with other genetic tests such as fluorescence in situ hybridisation (FISH) or polymerase chain reaction (PCR), such as multiplex ligation-dependent probe amplification (MLPA), to provide more specific information about genetic problems. FISH is a cytogenetic technique developed in the early 1980s to detect and localise the presence or absence of specific DNA sequences on chromosomes. Examples of diseases experienced by children with a DD that are diagnosed using FISH and cytogenetic techniques include Prader–Willi syndrome, Angelman syndrome, 22q13 deletion syndrome, cri-du-chat syndrome and Down syndrome. 11 PCR is a biochemical technology developed in the mid-1980s that is used to amplify a single or a few copies of a piece of DNA across several orders of magnitude. MLPA is a variation of multiplex PCR that permits multiple targets to be amplified with multiple primer pairs under a single set of reaction conditions.
Comparative genomic hybridisation and microarrays
Comparative genomic hybridisation (CGH) is a technique that allows the detection of losses and gains in DNA copy number across the entire genetic genome without prior knowledge of specific chromosomal abnormalities. Through the use of DNA microarrays in conjunction with CGH techniques, the specific technique of aCGH was developed. It greatly improves the resolution of chromosome analysis and can identify submicroscopic chromosomal abnormalities. However, aCGH is able to detect only unbalanced chromosomal abnormalities (as balanced abnormalities do not affect copy number). Therefore, balanced chromosomal rearrangements, such as inversions or translocations, cannot be detected by microarrays. It should also be noted that benign copy number variations (CNVs) are extremely common in the human genome. In addition, low-level mosaicism may be missed by this approach. The approach does, however, allow the exploration of all 46 human chromosomes in a single test (i.e. genome-wide copy number profiling). Furthermore, it has been found to be particularly useful for the diagnosis of dysmorphism, congenital abnormalities, LDs and DD.
Microarrays offer a much higher diagnostic yield than the currently used form of karyotyping for genetic testing of individuals with unexplained DD, mainly because of their higher sensitivity to submicroscopic deletions and duplications. It has taken some time for aCGH to be adopted in clinical settings but it is now the routine first-line investigation in most Western countries and is becoming widely available in the NHS in England for children with DD.
Diagnostic yields from karyotyping and microarray analysis
Identifying an unambiguous diagnostic yield for a particular technology is far from straightforward, if not impossible. The diagnostic yield of a specific intervention will depend on the previous cytogenetic studies performed and patient selection. The effectiveness of microarray analysis will also depend on the type and resolution of the arrays. In addition, the yield may be different depending on the setting, severity and gender. 26 Use of clinical pre-selection criteria (e.g. a positive family history) is also important. Typically, the detection rate for abnormalities has been increasing as arrays have provided higher and higher resolution. However, this trend is counterbalanced as more children with milder phenotypes are investigated. Although some of these children will have causative abnormalities, the frequency will be much less than for the children with more severe phenotypes.
Many articles have been published reporting on the yield of the various diagnostic investigations available for individuals with LD and MR (e.g. see reference van Karnebeek et al. 26). Comparisons between studies are hampered because of the different technologies being used and the differences within the patient groups.
The advent of next-generation sequencing technologies
The development of deoxyribonucleic acid sequencing
Although aCGH is useful if a chromosomal deletion or duplication is suspected, gene sequencing analysis is often required. DNA sequencing is the process of determining the precise order of nucleotides within a DNA molecule. Sanger sequencing, a type of DNA sequencing, was developed in the 1970s and has been in routine use in genetics laboratories since the 1990s. It is very effective for identifying mutations in single genes whose function is well understood. This is carried out to confirm a suspected clinical diagnosis, after which other members of a family can be tested to see whether they are at risk or whether they have a risk of transmitting the mutation to future generations. For about 25 years it has been the mainstay and ‘gold standard’ method for mutation detection because of its sensitivity, accuracy, robustness and reliability. Each reaction looks at a single fragment of DNA from a single individual and it can be relatively expensive to undertake. It was used to produce the first complete human genome sequence but this required $3 billion and took more than 10 years to complete. 27
The demand for low-cost sequencing has driven the development of high-throughput sequencing technologies that parallelise the sequencing process, producing thousands or millions of sequences concurrently. These post-Sanger technologies, which are collectively described as NGS, rely on preparing millions of much shorter fragments of DNA, which are then sequenced in parallel. This reduces the cost of sequencing but is generally accompanied by lower accuracy. The length of DNA that can be sequenced in an uninterrupted read on these platforms is much shorter than that using traditional Sanger sequencing. Since about 2005, three main massively parallel NGS platforms have been commercialised, based on three different sequencing technologies. These are reversible termination (HiSeq™, Illumina/Solexa, San Diego, CA, USA), pyrosequencing (Genome Analyser, Roche/454 Life Sciences, Branford, CT, USA) and ligation (SOLiD,® Life Technologies, Paisley, UK).
It is widely recognised that the terminology surrounding sequencing technologies can be diverse and confusing, with reference commonly being made to three generations of sequencing (see, for example, work by the PHG Foundation, such as Wright et al. 28). Sanger sequencing (used from the 1970s) was the first-generation technology; second-generation or ‘next-generation’ sequencing is the current technology; and the third-generation or ‘next-next-generation’ sequencing technology (which will mainly focus on sequencing single molecules of DNA) is being developed and will become available at some point in the future.
Massively parallel sequencing was developed during the 1990s. It was suggested that WGS can be completed in weeks at a cost of ≤ $50,000, with this cost decreasing rapidly. 27 The swiftness of developments in the technology is reflected by Flinter,29 who states that a whole human genome can now be sequenced in several days, at a current cost of several thousand pounds. However, she highlights the substantial costs and challenges associated with analysing, interpreting and storing such data. 29
Despite rapidly falling costs, sequencing an entire genome using massively parallel sequencing remains an expensive and time-consuming task, both for humans and computers. A less demanding approach, WES, has been developed. This approach sequences only the protein-coding parts of the genome (the exome refers to the exons or coding units, that is, the part of the genome that contains the coding DNA). These consist of approximately 30 million base pairs or 1% of the entire genome. WES is accomplished by selectively capturing the exons with the use of one of several array-based or solution-based methods that are now commercially available. The captured DNA is then sequenced by massively parallel sequencing and single nucleotide polymorphisms (SNPs) are identified by comparison with the reference genome.
The WES approach is attractive for several technical reasons. WGS involves screening the whole genome, most of which is not functionally important (although some sections are likely to have a functional significance that is not yet clearly understood). The majority of disease-causing sequence mutations that have been identified occur in exons. It is therefore likely that sequence analysis of the exome will continue to be successful at identifying novel disease genes. In addition, the human and computer requirements for sequencing and analysing a person’s exome are much less demanding than those for sequencing and analysing the entire genome. Mefford et al. 27 suggested that WES can be undertaken in the clinic at a cost of approximately $1000 (or approximately $10,000 for data generation and interpretation of the results).
As NGS technologies have begun to move from research laboratories into clinical diagnostic laboratories, targeted gene panels associated with particular diseases or pharmacogenetic effects are starting to be developed. In these, a set of known disease genes (rather than the whole exome) is captured and subjected to massively parallel sequencing. Panels may include, for example, 50 or 100 genes and their composition is evolving over time. There are a number of approaches to targeting including several alternative chemical methods of enriching specific genes prior to sequencing. The chemical enrichment methods can be divided into three broad groups: PCR based, circularisation methods and hybrid capture. Each of these methods is suitable for a different target size, type and sample number. In addition, each has its advantages and disadvantages in relation to performance, ease of use and costs and hence different approaches may be undertaken for different projects or applications. 30 Some universities and laboratories have launched disease-specific targeted sequencing tests, in part because of a belief that it is easier to interpret a panel of genes rather than an entire exome or whole genome. Some researchers, however, argue that in certain situations it is more straightforward and appropriate to interpret an entire genome. 31
Several other developments in the UK are promoting the use of genome sequencing in this country. In 2009, the House of Lords32 published a report on the development of genetic medicine that highlighted the need for a strategic vision for implementing genomic technologies within the NHS to maximise both their health and cost benefits. The PHG Foundation’s report on the implications of WGS for health in the UK28 includes recommendations to facilitate the adoption of WGS into the NHS to improve patient care. However, when considering the health economics aspects of WGS, the authors recognise the current paucity of economic and patient outcome data associated with the use of NGS technologies. They recommend that research be conducted to examine the micro- and macroeconomic implications of WGS to enable comparison of the costs and benefits of WGS and alternative clinical pathways and to allow for more sophisticated economic assessments to be made.
In December 2012, the UK prime minister announced ambitious plans to sequence the whole genome of 100,000 NHS patients over the next 3–5 years. To progress this, the Department of Health set up Genomics England in July 2013 to help deliver the 100K Genome Project into mainstream health care in the NHS, with the initial focus being on patients having genetic testing for the diagnosis of rare disorders, cancers and infectious diseases. 33
Information on the effectiveness of traditional genetic testing techniques can be found in Appendix 3; cost-effectiveness evidence is presented in Chapter 4.
Effectiveness of whole-exome sequencing and whole-genome sequencing
As the diagnostic yields of WGS and WES are anticipated to improve over time as the technologies become more established, there are as yet no (rigorous) published studies comparing the effectiveness of these technologies with the effectiveness of more traditional diagnostic techniques. There is, however, some evidence that WES or targeted gene sequencing are, at least at present, seen as more appropriate than WGS in the clinical setting. A NGS survey undertaken in 2013 by Oxford Gene Technology34 analysed responses from 596 researchers working with NGS in a variety of countries in relation to their current use and future demands from the market. The majority of the respondents were from the UK or the USA (38.5% and 21.7% respectively) and almost three-quarters of respondents (73%) were carrying out academic research. Targeted resequencing (i.e. targeted gene panel sequencing and exome sequencing) was in high demand, with almost half (46%) of the respondents planning to use this method in the next 12 months. In contrast, only 13% of the respondents were interested in using data analysis-intensive WGS. Cancer (27%) was the most popular research area for NGS, followed by rare diseases (17%) and developmental disorders (15%). About three-fifths (61%) of respondents were planning to use targeted panel sequencing, with almost one-third (30%) favouring WES and less than one-tenth (9%) intending to use WGS for such assays. Over half of the researchers (56%) cited bioinformatics, including data analysis and interpretation, as the biggest challenge. Although this survey targeted respondents working with NGS technologies in a wide variety of countries and settings, it provides an insight into the two aspects of NGS that are currently attracting the greatest interest in this community: targeted gene sequencing and bioinformatics. Some of the strengths and weaknesses of the different approaches are considered below.
With regard to improving diagnostic yield, Vissers et al. 35 reviewed the advantages of using high-resolution array platforms targeting the entire human genome rather than conventional karyotyping to detect CNVs when exploring the causes of MR. The findings indicate that obtaining detailed copy number information increases the diagnostic yield for patients with MR. The authors estimate that copy number-dependent gene dosage variations will eventually explain up to one-quarter of cases of MR, although they stress that not all of these variants will be fully penetrant. They conclude that high-throughput (re-)sequencing may reveal disease in another 10–30% of cases, although they stress that interpretation of variants will require international and multidisciplinary collaborative team working.
Building on from this, Hayes et al. 36 considered different approaches to the genetic diagnosis of copy number by comparing the performance of a NGS platform (using Illumina technology) with that of an aCGH platform in 39 patients with DD and/or LDs. They found that all 11 imbalances detected by aCGH and confirmed by FISH or quantitative PCR (Q-PCR) were also detected by CNV-seq (a type of high-throughput sequencing). In addition, CNV-seq detected one purported pathogenic CNV that was not detected by aCGH. Non-pathogenic, unconfirmed CNVs were detected by both platforms, although few were concordant between them. They concluded that NGS technologies, particularly CNV-seq, may prove superior to array technologies for detecting chromosomal and genetic abnormalities, particularly as the cost of NGS technologies falls. CNV-seq also has less stringent sample requirements.
Some studies do, however, focus on the effectiveness of WGS. Jacob et al. 37 report on their experience in Wisconsin, USA, of converting a sequencing laboratory designed for research into a fully functional clinical programme, with the goal of using WGS to elucidate the aetiology of undiagnosed diseases in patients who had exhausted all standard care options. In their sample of 26 patients with a variety of clinical indications but without diagnoses, they were able to obtain a definitive diagnosis in 27% of cases (7/26). They also made a potential diagnosis in 34% of cases but failed in the other 39%. The authors believe that the percentage of definitive and/or potential diagnoses may increase over time with experience.
Heger31 reports on work being undertaken in Holland by a group led by Joris Veltman, some of which compares using WES and targeted gene sequencing for a range of disorders, including ID. Their early findings suggested that NGS technologies should be used in clinical settings, especially when the diagnosis is uncertain. The researchers favoured exome sequencing over a targeted approach as the former test is more comprehensive and adaptable and thus easier to update. Furthermore, it can sometimes be more straightforward to interpret an entire exome, as it is not always clear when sequencing a handful of genes whether a variant is disease causing or not. They suggest that looking at the whole exome can make it easier to rule out variants of unknown significance if there is a more obvious candidate.
Following on from this, de Ligt et al. 38 used exome sequencing in a diagnostic setting to identify genetic causes of severe [intelligence quotient (IQ) < 50], non-syndromic ID in 100 patients for whom extensive conventional genetic testing and array-based tests had failed to detect any obvious genetic causes for their condition. For each patient, DNA from both parents was also available for analysis. By determining which variants in the patient’s exome were absent in the exomes of both parents, the investigators were able to identify de novo sequence variants in the patient. The study identified the genetic cause of ID in 16% of patients, with the majority of cases caused by a mutation in a gene that was already known to cause ID. Another 19 genes carried a de novo variant that might be pathogenic, but a conclusion about the clinical significance of the genetic variant could not be made. The patients in the replication set [another set of patients (n = 765) who underwent the same study at a later date] had yet to be screened for mutations of these 19 genes, suggesting that the diagnostic yield may increase once these genes have been analysed in this series of patients. The authors concluded that exome sequencing can be utilised as a diagnostic procedure in the patient group studied, with diagnostic yield potentially increasing as methods improve and additional genes associated with ID are identified.
With regard to the development of sequencing technologies, Mefford39 concludes that exome sequencing is revolutionising the field of genetics as it allows for a diagnosis to be made in a substantial proportion of cases. She takes the view that genomic technologies are developing at a rapid pace and that WGS will shortly be used routinely such that eventually there will be no need to utilise chromosomal microarray (CMA) as a separate test. She advises clinicians and researchers to ‘proceed with both optimism and careful thought’ (p. 1953) as NGS technologies become incorporated into routine clinical practice.
Which technology when?
Each potential approach to sequencing has its strengths and weaknesses. WGS covers a much greater number of DNA fragments than WES but at a more superficial level. Therefore, some important gene mutations that can be detected using WES or Sanger sequencing will be missed when using WGS. Indeed, any mutation found by WES or WGS currently needs to be investigated further to make sure that it is not a false positive before it can be reported with confidence. It is therefore usually necessary to check that a mutation is detectable (and therefore genuine) by Sanger sequencing before it can be used for any subsequent familial testing. Furthermore, some types of mutation, including microdeletions/duplications, cannot be detected by WES or WGS and so aCGH remains the technique of choice for their detection.
It has been suggested (Professor Frances Flinter, Guy’s and St Thomas’ NHS Foundation Trust, 2013, personal communication) that, currently, the best approach to maximise patient benefit involves the use of aCGH if a chromosomal deletion or duplication is suspected; PCR and Southern blotting if a triplet repeat disorder (e.g. Huntington’s disease) is being considered; targeted Sanger sequencing and MLPA if the relevant gene is clearly implicated; and WES if the pathway is known but not the specific gene. She considers WGS to be very interesting in a research context but does not feel that it provides sufficiently detailed coverage to be useful in a clinical setting. These views are relevant for diagnosing a wide variety of conditions such as cancers and various long-term conditions as well as the causes of LDs.
When considering the most appropriate technology to use, it is also important to be aware of the infrastructure that is needed to support the analysis of the data, interpret the results and explain the details to clinicians, patients and patients’ families. Hurd and Nelson40 point out that, compared with NGS technologies, microarrays are established tools, with mature bioinformatics pipelines for array data analysis. Microarray technology is also being refined and its costs reduced. Although the pipelines for NGS technologies are developing and maturing, they are still not as established as those for the more traditional technologies.
Results II: opportunities and challenges associated with introducing next-generation sequencing into routine clinical practice
The material presented in Chapter 3 on the new systems and safeguards that will be needed before NGS technologies can be introduced into NHS clinical practice reflects the views of the interviewed stakeholders. In addition, various relevant reports, papers and other documents have been published recently and this section provides a narrative overview of their key points.
Data quality
A recent Briefing Note published in 2014 by the PHG Foundation41 outlines fundamental challenges to using whole-genome analysis in a clinical diagnostic setting, including ensuring that the raw genome data are of sufficient quality and completeness and that the validity and accuracy of clinical interpretation is not undermined by an unreliable evidence base. The report stresses that using whole-genome data in clinical diagnostic services within the NHS without first addressing the fundamental quality-related issues poses potentially unacceptable risks to patient safety and quality of care. These risks include:
-
incorrect diagnosis (false positives or false negatives) leading to inappropriate patient care and decision-making and threatening patient safety
-
failure to provide a conclusive diagnosis for the patient and a continuation of their diagnostic odyssey
-
inappropriate use of NHS resources.
As such, the authors recommend four steps to overcome these challenges: determining minimum standards for sequence generation; establishing best practices in bioinformatics; improving the quality of the evidence base; and building infrastructure. However, it is noted that implementation of these steps will be time and resource intensive and will require a methodical approach. 41
Further publications also highlight the need for a clear, evidence-based policy on how to conduct genome testing. For example, a paper on the policy challenges of clinical genome sequencing33 argued that a clear, evidence-based policy on how to conduct genome testing is both essential and urgent. It focused specifically on problems of data overload, incidental findings, widening public understanding and focusing on diagnosis. 33 Although this paper states that genomic sequencing is now sufficiently cost-effective to be offered clinically, interpretation of individual genomic variation remains challenging and the importance of incidental findings is unclear. Thus, it may be premature to offer opportunistic genome screening without knowledge of population penetrance. It is recommended that clinical genome sequencing efforts should initially focus on delivering diagnoses for patients, with efforts also focusing on developing a shared database of sequencing results linked to phenotypes to facilitate research. The authors also state that, in patients whose clinical presentation is indicative of a primarily genetic aetiology, a combination of genome-wide sequencing and clinically targeted analysis can maximise interpretable pertinent findings and minimise non-pertinent incidental findings. Furthermore, they recommend that guidance and educational material be developed for those involved in NHS genomic sequencing, particularly focusing on the purposes of and limitations associated with testing, the difficulties of interpretation and the need to share the data acquired through such testing.
Giving informed consent
Another issue associated with introducing NGS technologies into clinical practice relates to the ethical challenges and practical dilemmas associated with informed consent, particularly how incidental (or unsolicited) findings will be handled. Because NGS technologies are highly likely to generate incidental findings, it is suggested that guidance is needed on:
-
the information that should be discussed and consented to preceding the test
-
which results to disclose to the patient (which may have consequences for relatives)
-
the extent to which patients should decide on what should be reported back to them. 42
Rigter et al. 42 also propose a set of questions to help gain insight into the degree of feedback that patients (or, in the case of children, their parents) should and could be able to decide on when the results from using NGS technologies in diagnostic settings are reported back to them. These are presented in Box 1.
-
Who is giving consent?
-
What is the initial clinical enquiry?
-
Which unsolicited findings can be expected?
-
How can the different possible unsolicited findings be categorised?
-
What pre-test information and results should be communicated to the patient?
-
What does this mean for the consent procedure?
Source: Rigter et al. (p. 1327). 42
In particular, the need for an optimal informed consent procedure for exome sequencing in diagnostics has been highlighted. 43 The 2013 policy paper of the European Society of Human Genetics on WGS concluded that, to develop best practice, information sharing structures must be set up and guidance must be put in place regarding clinical utility, procedures for obtaining informed consent and how best to handle unsolicited findings/variants. 44 This paper recommended that protocols be adjusted as knowledge and patient and public responses evolve, with a view to any current recommendations being re-evaluated in a few years’ time.
In the USA, the ACMG has published guidance regarding informed consent for genome/exome sequencing. 45 Given that incidental findings are managed differently in the USA from in Europe, the ACMG document is not directly appropriate for the UK context. However, it – along with similar documents, statements and reports published in Europe – reflects that much discussion and debate about patient rights and professional duties has taken place. Despite all of this effort and expertise, no firm conclusions have been reached, and such activities will continue at local, national and international levels. It is important that such debate and discussion is undertaken in the UK on an ongoing basis to ensure that equitable, consistent and evidence-based approaches are adopted for the provision of informed consent, thus safeguarding all stakeholders in this process.
Reporting test results and managing incidental findings
Research literature differentiates between pertinent findings (those that have been purposively generated or sought) and incidental findings (those that emerge during the course of an investigation and that may have potential clinical and/or health implications but which were not specifically sought out as part of a test). 46 When considering the disclosure of findings generated by WGS undertaken in a research context, five graded possible approaches have been identified:46
-
non-disclosure of potential findings that might have an impact on the health of an individual
-
disclosing only clinically significant findings that are severely and moderately life threatening and clinically actionable
-
disclosing all clinically significant findings regardless of their severity and actionability
-
disclosing all variants (regardless of severity, clinical significance and actionability)
-
research participant chooses a disclosure policy.
It is considered part of a physician’s duty of care to his or her patients to assess the potential benefits and harms associated with the disclosure of additional findings, informed by knowledge of the patient and the clinical context. A PHG Foundation discussion paper46 identifies several aspects that the physician might consider when deciding what to disclose:
-
the seriousness of the presenting problem and the nature of the other findings
-
whether the finding represents a known clinical entity or risk factor or requires further investigation (e.g. variants of uncertain clinical significance)
-
whether the finding has been validated to an acceptable standard
-
the availability of any treatment/prophylaxis and its likely success
-
whether the finding is a risk factor for disease or represents a disease process
-
the age of the patient and co-existing morbidities and conditions
-
prior knowledge of the patient’s wishes
-
custom, practice and precedent: how other professionals have handled the same finding in similar circumstances and the extent to which there is consensus about this approach.
In a clinical context, it is suggested that it is the ‘professional obligations of beneficence and non-maleficence’ and the responsibilities of the physician that will drive the approach to the disclosure of additional findings, rather than the individual patient’s autonomous wishes. 46 Adopting this approach ideally requires physicians to have easy access to information about custom, practice and precedent. This, in turn, requires a mechanism or framework for collecting and presenting this information.
The UK10K WGS project, which took place between 2010 and 2013,47 identified the following four criteria that had to be met before a pertinent finding or an incidental finding could be passed on to the participant:
-
Respect the research participant’s right to know or, alternatively, their right not to know about clinically significant findings. Explicit consent must have always been given by the participant for incidental findings and/or pertinent findings to be returned.
-
Clinically significant findings should be returned only when they are of significant clinical importance.
-
Clinically significant findings should be clinically and analytically validated by a genetically accredited laboratory before being returned to participants by their treating clinician.
-
Feedback to individual participants should be conducted by a trained professional able to provide genetic counselling.
It may be appropriate to develop and adopt a similar ethical framework for managing clinically significant findings arising from using NGS technologies in clinical settings, although this would require strong leadership at a national level. If this is not forthcoming, there may be scope for developments at a regional level.
The 2013 paper48 on WGS in health care by the European Society of Human Genetics focused on the clinical diagnostics setting. Two of its recommendations are of particular relevance to handling incidental findings:
-
when, in the clinical setting, either targeted sequencing or analysis of genome data is possible, it is preferable to use a targeted approach first to avoid unsolicited findings or findings that cannot be interpreted
-
in case of testing minors, guidelines need to be established as to what unsolicited information should be disclosed to balance the autonomy and interests of the child and the parental rights and needs (not) to receive information that may be in the interest of their (future) family.
Finally, to show that there are no universally agreed approaches to addressing these ethical dilemmas, the work of the ACMG should be noted. The ACMG published its recommendations for reporting incidental findings in clinical exome and genome sequencing in 2013. 49 These recommendations require laboratories, regardless of the indication for which the sequencing was ordered, to explicitly seek and report on a minimum list of variants; patients are not allowed to decide whether they wish to receive such information. Instead, the ACMG recommends that patients who decline to receive information about these incidental variants should not be allowed to have their genome or exomes sequenced. Total responsibility is placed on the referring clinician by the laboratory geneticist. These recommendations are considered by some to be contrary to international ethical standards50 for a number of reasons, for example the issue of coercion has been raised with respect to not giving patients a choice of other options and denying them a test if they decline to receive information about secondary variants. It has been suggested that medico-legal considerations have played some part in their development. 50
Chapter 3 Stakeholder interviews
Introduction
This chapter uses information from the stakeholder interviews to address the second objective (i.e. to collect stakeholder views on the changes in service provision that would need to be put in place before NGS could be used in clinical practice). It also uses information collected from stakeholders to partially address the third objective (i.e. to describe new systems and safeguards that would need to be put in place before NGS could be used in clinical practice). Further information to inform the third objective has been extracted from the literature and can be found in Chapter 2 (see Results II: opportunities and challenges associated with introducing next-generation sequencing into routine clinical practice).
Methods
Sampling and participants
A sample of stakeholders (n = 33) was recruited. In a scoping exercise such as this, identification of appropriate individuals to interview is necessarily pragmatic. In effect, a ‘snowballing’ approach was employed. Three key categories of stakeholders were identified initially:
-
doctors working with children with LDs in a generic capacity in primary and secondary care settings [e.g. paediatricians and general practitioners (GPs)]
-
genetics specialists working in laboratories and/or NHS clinical settings (e.g. clinical scientists, bioinformaticians, clinical geneticists, genetic counsellors)
-
representatives from charities (or similar organisations) working with families of children with LDs.
A number of key individuals were identified by members of the RAG, through their recent publications or from web-based information about their work. Interviewees were drawn mainly from NHS settings, although several also had academic links with a university. Some also had a wider ‘national’ perspective from their activities with organisations such as the British Academy of Childhood Disability (BACD) or from their work on clinical trials. These individuals were also asked to suggest colleagues with relevant expertise or experience who might also be willing to be interviewed. This approach resulted in contact with a number of people from organisations with a national focus, such as the Department of Health, the Nuffield Council on Bioethics, Genetic Alliance UK and Nowgen (an organisation specialising in public engagement, education and professional training in biomedicine). An internationally renowned professor from Holland was able to provide a European perspective. A list of interviewees is presented in Appendix 4.
Interviews and data analysis
Semistructured interviews were conducted with all 33 participants. All but one of the interviews took place on a one-to-one basis by telephone; the remaining interview (involving two interviewees and two researchers) was undertaken face-to-face at a professional conference.
Each interviewee was sent a list of possible discussion topics before interview (see Appendix 4). Each interview commenced with the participant being asked about his or her understanding, knowledge and experience of NGS. The remainder of the interview was then tailored to reflect the knowledge and specialist area of each interviewee. Each interview concluded with a brief discussion of the participant’s views of the main strengths and weaknesses of traditional approaches and NGS, including their implications for patients. Interviews lasted between 30 and 60 minutes.
Interviews were audio recorded and transcribed verbatim. All interviewees were offered a copy of the transcription so that they could, if they wished, add any additional points that may have occurred to them after the interview had ended.
Interpreting the results
The views of the stakeholders are summarised in the following section; various quotes from the interviews are reported in Appendices 5–7 and provide an evidence base for the material in this section and the subsequent discussion in Chapter 5. Stakeholder views should be considered in conjunction with the material from published reports, papers and websites discussed in Chapter 2; the material was selected and presented to provide contextual and factual information about specific aspects and developments mentioned by interviewees. When interpreting the material from the stakeholder interviews it is important to note the influence of the varied backgrounds and experiences of the interviewees on their responses and perspectives. Clinicians, such as paediatricians and GPs with first-hand experience at a local level of caring for children with LDs and their families, generally tended to take a pragmatic perspective and consider what they believed would be needed to assist their clinical practice at a local and/or regional level. However, those with an overarching focus, such as individuals representing government bodies and charities, generally had a wider view of what would be needed/desirable from a national standpoint. Those working primarily in NHS genetics laboratories provided another dimension. Furthermore, some interviewees focused entirely on the potential use of NGS technologies for diagnosing children with LDs, whereas others considered the broader role of NGS technologies for diagnosing other conditions. Some had direct experience of using NGS technologies in research settings. A few, especially those working directly with patients in NHS settings, had little knowledge of NGS technologies but were familiar with some of the recent challenges associated with introducing aCGH into the NHS for diagnosing children with LDs.
Results I: changes in service provision
Supporting information, in the form of additional interview quotations, can be found in Appendix 5.
Data-related issues
Data capture: the laboratories
This section considers the structure of, and inter-relationships between, the NHS regional genetics laboratories. Given that this study addresses the potential use of NGS technologies in NHS clinical practice, a relatively pragmatic perspective, based on the current regional configuration of NHS laboratories, has been taken. Interviewees highlighted that substantial laboratory-related infrastructure changes would be required before NGS technologies could be introduced into routine clinical practice and also that some possible service configurations for testing using NGS technologies are being considered at a high level by the government.
Before discussing participants’ views it is important to identify the different data-related steps in the sequencing process. In essence, technicians run the tests in laboratories using a variety of sequencing machines to generate data, bioinformaticians interpret data to provide information and clinical scientists make the information clinically relevant to the person who will use it (e.g. a paediatrician). Data generation therefore requires laboratories with appropriate technology and trained technicians to run the tests. There is already a range of machines using different platforms and these are constantly being developed. The three main companies in the global market are Illumina, Life Technologies and Roche Diagnostics. Laboratories can buy these machines ‘off the shelf’, although one interviewee commented that ‘they are probably too expensive for most diagnostic laboratories [to do this]’. There did not appear to be a strong consensus among participants about the most appropriate machine/platform to use to test for specific conditions, although the Illumina platform seemed to be favoured by one or two interviewees. Indeed, it was suggested that different platforms may be required for the different conditions associated with LDs or possibly for research and diagnostic use. It was recognised that there will be many changes over the next few years, including a move from second-generation sequencing platforms to third-generation platforms.
These machines currently cost about £200,000 and interviewees suggested that this price is unlikely to fall much further as the market for NGS technologies is already quite competitive. Although this may seem expensive, interviewees did not generally consider it to be out of line with the costs of other machines used in NHS laboratories. However, one interviewee suggested that these machines will probably need updating every 2–3 years – ‘until the next big thing comes out’. It was therefore deemed unlikely that they will be purchased by all of the 23 current NHS regional genetics laboratories.
Although it was generally considered that the data capture process was relatively straightforward, interviewees stressed the importance of it being carried out properly and in a cost-efficient way. Data need to be analysed and stored and it was suggested that this may best be achieved with a few core, well-funded institutions that can ‘modernise as new technology emerges’. To this end, potential configurations for laboratories were discussed. Interviewees raised two dimensions in relation to this debate: first, whether NHS (or other) laboratory provision should be more centralised and, second, whether the sequencing should be undertaken by NHS and/or private sector laboratories.
With regard to the former, several possible configurations were suggested, including developing more formalised ‘hub and spoke’ arrangements for NHS laboratories, developing a small number of ‘super’ laboratories (possibly involving private sector providers) and developing a few ‘sequencing farms’ or ‘sequencing factories’ as centralised high-throughput facilities. Interviewees generally considered that strong and decisive actions would be required by the government to move provision away from its current structure. As previously mentioned, there are currently 23 NHS regional genetic laboratory services, but interviewees generally considered that far fewer (e.g. eight to 10) were needed to undertake sequencing for NHS diagnostic purposes. Having fewer specialist facilities could help to keep the focus on bioinformatics (as bioinformaticians are expected to remain a scarce resource) and also help to address data storage and sharing issues in a consistent manner.
One person with a national perspective stated that NHS England is currently ‘kick starting’ a process to focus on a combination of ‘hubs’ and ‘spokes’. Under such a configuration, a small number of specialist ‘super’ laboratories would be developed. These would focus on diagnostic discovery and would undertake the sequencing. They would develop expertise in, and strive to understand, the associated complexities. Other laboratories would focus on routine panels, arrays and other similar types of work. One interviewee did not think that such a reconfiguration would require a larger workforce because of the efficiencies and economies of scale that would result and because of the reduction in time that would be spent by laboratories on other ‘ineffective and pointless testing’. Another interviewee considered that a hub and spoke model for service delivery should not detract from ‘the valuable regional genetics services’ under which the principles of centres of excellence can still apply.
One genetic specialist with an academic background considered that centralisation of the laboratories was likely to be cost-efficient although implementing this would require commitment and money from the government, which they thought would be unlikely to be forthcoming. This individual considered that, if the NHS provided the laboratories, it would be important that they were adequately funded and that, if the private sector was involved, its reputation for ‘taking the lower-hanging fruit’ would need to be addressed. Furthermore, it would be important that any contracts with private sector providers were ‘very carefully written’ with regard to responsibility and liability, to ensure that the sequencing activities were connected with responsibility for subsequent clinical decisions. Some interviewees considered that ‘the NHS has a notoriously poor history of private sector commissioning’ and favoured using NHS laboratory provision if possible. However, one interviewee took the view that ‘private labs are certainly on the horizon’, with ‘a number of private concerns . . . manoeuvring into position to look at this’. One interviewee with a national perspective referred to the possibility of developing ‘sequencing farms’ or ‘sequencing factories’ as centralised, high-throughput facilities to ensure that resources are used efficiently. In theory, much of the diagnostic work could be undertaken by laboratories in other countries (e.g. China), but there was no support for this type of service model amongst the interviewees. They considered that it is vital that laboratories and clinicians can work closely together.
Data interpretation
One interviewee (with an academic research background) suggested that the diagnostic yield for all technologies is skewed by the severity of the LD. They considered that, in time, technology and interpretation skills will develop to such an extent that it will be possible to make a diagnosis for most people with severe LD (i.e. an IQ < 50). However, they considered that data interpretation will be much more difficult for people with mild to moderate LD (i.e. an IQ in the range 60–70).
Bioinformaticians are highly specialised scientists who apply computing skills (such as programming, statistics and data analysis) to biological data, such as NGS data, produced by high-throughput biological techniques. Their work is complemented by that of others, such as clinical scientists and molecular biologists. Bioinformaticians and clinical scientists work in a variety of settings and need access to appropriate computing facilities (hardware and software). Testing and interpretation do not have to take place at the same location although this is usually the case in NHS genetics services. The need for increased numbers of bioinformaticians is discussed in NHS staffing and training issues.
One interviewee considered that it is currently impossible to implement NGS technologies into the NHS because the necessary infrastructure is not in place to ‘overcome the logistics’ associated with using the technology. Problems arise because of the vast amount of data that are being generated. Taken from the perspective of a clinical scientist, NGS technologies produce data on far too many variants to have time to look through all of them to identify the actual mutation. ‘So it’s really the sheer scale of numbers that we are hitting on both the IT and the clinical interpretation side, that’s the logistic aspect we have to overcome.’ This requires having sufficient staff with the appropriate knowledge and enough computing capacity to handle the analysis of very large data sets. An interviewee working in a NHS regional genetics service considered that, although the generation of the test data is relatively straightforward, data storage and interpretation are complex. Most people currently working in laboratories have been trained as ‘wet lab scientists’, but biometricians need completely different training.
Another interviewee stressed the importance of knowing about the clinical presentation (or the phenotype) of the patient before analysing the data. It was explained that molecular biologists and clinicians need to work with bioinformaticians to interpret the data.
Data storage
The need for changes in service provision with regard to data storage was also highlighted by several interviewees. One interviewee with a national perspective stressed the importance of ensuring that ‘there is patient and public confidence around what is done with the data’. This interviewee pointed out that the storage issue really depends on how many terabytes are needed, which in turn depends on what data are actually kept – is it ‘the whole DNA sequence or just the whole exome?’ Furthermore, ‘as we move towards cheaper sequencing, will it be cheaper to resequence rather than store the original data?’
During one interview it was explained that not only is electronic storage needed for the data generated by using NGS technologies, but also this service will have to be maintained for a period of 30 years under current legislation. This is much longer than is usual for most NHS data. It may be sensible (and indeed necessary) for these legislative requirements to be reviewed (e.g. at a national level) before NGS technologies are used more widely in NHS clinical work.
One interviewee discussed the importance of adequate computing capacity for both data analysis and storage, especially as testing evolves from panel-based work to whole exomes and whole genomes. Data storage was considered to be the bigger of these two problems, especially as NHS trusts act as individual entities. Although secure cloud-based data-sharing solutions may be available in the future, currently everyone is looking to develop local ways to store the huge amounts of data that will be generated by NGS.
One genetic specialist interviewee suggested that, although cloud development may be appropriate in the future, it will take ‘a few years for people to trust this technology’. However, another interviewee with a similar background thought that raw data could be stored as they are not necessarily interpretable in this state, even if the firewalls around them are breached. This interviewee also suggested that resequencing might be the most appropriate way to answer any further questions in the future.
Data sharing
The need for better data sharing was also stressed by several interviewees. One interviewee with an academic and research perspective was ‘less concerned about whether the labs are NHS or private sector’ than about ‘access to the data, ownership of the data and analysis of the data across all health service-recruited samples’. In their view, it is vital that ‘the NHS data should be held in a central place that is freely available to other health service users’. Fragmentation of data analysis ‘would be catastrophic’.
Another interviewee from a NHS laboratory also raised the importance of having a critical mass of data and of data sharing, stating that ‘a lot of the data that we are looking at only starts to make sense when you look at large amounts of patients’. This interviewee took the view that ‘the main obstacles are technological – we don’t have the databasing facilities to do this’. When asked if this obstacle could be overcome, this interviewee said that the laboratories were currently working on it ‘but there’s not a solution at the moment’.
Finally, one interviewee referred to there being a concordat in the UK with the genetic companies about using genetic data, unlike in some other countries (e.g. the USA). This should provide some reassurance about how pooled data from different sources would be used if private sector laboratories become directly involved in the use of NGS technologies for diagnostic work in the NHS.
NHS staffing and training issues
Almost all of the interviewees highlighted the need for additional staff, especially in the short term. Although some of these personnel, especially those who will undertake diagnostic work in laboratory settings, may be recruited from staff already working on research projects, the need for increased numbers of trained staff working in clinical settings was frequently stressed, alongside the need for additional government-funded training courses, especially for bioinformaticians and clinical scientists. Several interviewees also referred to the need for wider training for clinical staff working with children with LDs (i.e. the ‘patient-facing’ professional, including nurses and GPs), to improve their knowledge and awareness of genetic issues.
Staff training: testing and interpretation
The need for more clinical bioinformaticians was often raised during interviews. One interviewee suggested that ‘there’s no point sequencing if you don’t understand the outputs’. An interviewee with a national perspective stated that ‘previously there have been microbiologists and genetic scientists, but there have never actually been clinical bioinformaticians within the NHS’.
One interviewee explained that, to help alleviate this gap, a 3-year Master’s-level training course for bioinformaticians, the Scientist Training Programme (STP) in Clinical Bioinformatics (Genomics), leading to a MSc in Clinical Science (Genomics), started in Manchester in September 2013. It is part of the NHS Modernising Scientific Careers (MSC) programme and is the first course specifically designed to train clinical bioinformaticians to work within the NHS. It is an integrated work-based course with participants employed in fixed-term, salaried training posts. Genetics aspects are rolled out within the participants’ clinical genetics centres/laboratories, but students also partake in distance learning and off-site learning with the university. University-based elements include generic sessions on clinical practice, which cover aspects such as professional practice, public health, quality, ethics, research methods and public–patient involvement. NHS trusts with appropriate laboratories can put forward candidates for STP courses and apply to their Local Education and Training Board for funding for these places. During the interview it was reported that 14 people had started the programme in autumn 2013 and it was estimated that a further eight would start in 2014/15, which would enable a body of trained clinical bioinformaticians to build up steadily over time. This training course for bioinformaticians is seen as an exciting development in the use of NGS technologies across the NHS, not just for use with children with LDs.
In addition, it was highlighted during interviews that staff currently working in laboratories as bioinformaticians (and other staff with relevant genomics expertise) need to be able to provide a support framework to their (often newly trained) colleagues, including those working in other laboratories, to ensure that data generated and received by laboratories are appropriately managed. Although directly mentioned by only two respondents, issues of governance, supervision, validation and audit were clearly relevant. It was explained that, to ensure consistent and accurate working and continuing professional development (CPD), these will need to be addressed for all those employed in this relatively new field. Profession-wide guidelines may therefore be needed, possibly developed by the Department of Health or some other appropriate body.
Many of the interviewees also considered that more widespread use of NGS technologies for clinical diagnosis in the NHS will require more clinical scientists (or health-care scientists) with genetics knowledge. The duties of a clinical scientist will depend on their specific role but may involve:
-
interpreting test results and suggesting methods of treatment to doctors
-
researching, developing and testing new methods of diagnosis and treatment.
It was explained that there is a range of MSC courses for people wanting to become qualified to work as a clinical scientist in the field of genetics. In addition, bioinformaticians and clinical scientists will also be expected to undertake CPD and it is therefore important that there is an infrastructure (at national and local levels) of ongoing support, training and education for these staff.
Staff training: clinical staff
Many interviewees referred to the need for widespread and ongoing training in genomics and genetics amongst NHS and other staff caring for children undergoing diagnostic testing for LDs and their families. This would require considerable investment in education and CPD and was seen as ‘a real challenge for Health Education England’. Some interviewees stressed instead the importance of good team or partnership working and/or the need to have knowledge only of specific aspects. Under such arrangements paediatricians would ‘deal with the day-to-day management of the child’s secondary disabilities and troubleshoot for any additional medical complications’ whereas the clinical geneticists would concentrate on gene coding aspects.
One respondent also thought that it would be important to employ neurodisability-trained paediatricians in every locality ‘so that all the management of the secondary disabilities and all of the surveillance that is required can actually get done’. A medical geneticist interviewee considered that the doctor must trust the clinical scientist and that they need to have a strong working relationship. However, when asked about the importance to clinicians of genetics-based knowledge, a general paediatrician considered that, although it might be interesting to know about the genetics in detail, in reality a front-line clinician needs to know only the significance (or otherwise) of the test results.
One interviewee thought that teaching about genomics should be included in medical education and thereafter provided through CPD. In addition, this interviewee thought that a significant proportion of the current workforce needs training (or at least their awareness raised) in this area. It was suggested that there is considerable scope for appropriate organisations to develop a range of different training media for this, including web-based opportunities. However, resources (e.g. time and money) need to be found within the NHS to enable staff to take advantage of any such training opportunities, which are likely to be in addition to their regular CPD commitments. Another interviewee discussed the effect of NGS technologies on the process of consenting patients and pointed out that those undertaking the consenting would need further genetic knowledge.
An interviewee with an international perspective considered that it was important to have more rare disease specialists, as increasing numbers of patients with new and/or rare diseases would be diagnosed using NGS technologies.
Genetic counsellors
Mixed views were expressed about the need for more genetic counsellors, although most of the interviewees considered that many more of them would be needed with the introduction of NGS technologies. Furthermore, as the use of clinical NGS technologies becomes more widespread, current counsellors would need to undergo the relevant genetics training to enable them to work effectively with families experiencing these new technologies. Finding the time for this and subsequent CPD could be an issue.
A genetics counsellor explained that the delivery of genetic counselling may change considerably, with the focus of attention shifting from the early stages of testing to the point at which families have received a diagnosis. Genetic counsellors would help families to understand this information and adjust to it. However, such an approach introduces a situation in which a family without a diagnosis would not receive any support from a genetic counsellor. This interviewee explained that increasing the numbers of genetic counsellors will be a complex and lengthy process. Genetic counsellors have to be registered with the Genetic Counsellor Registration Board (GCRB), which can require a long period of training. People generally come into this type of work through one of two routes. One is a nursing/midwifery/health visiting route, which requires completing training in counselling skills of at least 90 guided learning hours, with at least 30 of these hours being delivered through an academically accredited course with an element of formal assessment/examination. This route also requires the completion of an academically accredited course in the science of human genetics of no less than 30 guided learning hours (also with a formal assessment/examination). The other route is through a genetics degree (or similar) and a MSc in Genetic Counselling. There are only two universities in the UK (Cardiff and Manchester) that are accredited to provide this MSc course. Although well subscribed, these courses train an average of only 15 people per year.
It was also explained that, after meeting these initial requirements, applicants for GCRB registration must also complete at least 2 years of clinically focused work in an approved genetic counselling post (with appropriate supervision and mentoring) and submit a portfolio of their work. However, there has recently been much concern amongst genetic counsellors as external funding for these training posts had been time limited and has now ceased, which will make it much harder for people to become registered genetic counsellors.
In addition, the consequences for the service of the current age profile of genetic counsellors were also raised. The profession has lost many experienced staff in recent years as people have reached retirement age. Others are approaching this age. At the other end of the age spectrum, many of the younger counsellors are of child-bearing age and may therefore be requesting a period of maternity leave. These trends are expected to continue to place NHS genetic counselling services under considerable pressure.
An alternative view was provided by a paediatrician interviewee who works closely with local clinical geneticists. This interviewee considered that there was possibly a greater need for more nurse specialists with particular niche expertise (e.g. neurofibromatosis, fragile X syndrome) than for more counsellors, especially in places where clinical networks are less well developed.
Another interviewee (a consultant community paediatrician) described how their service often uses specialist health visitors to support families, although they would liaise with the genetic counsellors about unfamiliar conditions.
Much of the above discussion was summarised by a professorial interviewee, who considered that there would be a need for many more genetic counsellors who are highly trained in molecular genetics as well as having clinical skills, who can interpret data as well as explain its implications to patients. Local leaders will be needed to tackle the difficulties of developing genetics services in an area, with regional science centre hubs working closely with local clinicians.
Other aspects
Three other broad areas for changes in service provision emerged from the stakeholder interviews, namely the need for public education, the importance of family support and changes to service commissioning.
The need for public education
A few interviewees, especially those with a broad perspective of the future use of NGS technologies in the NHS, thought that there needed to be greater public education and debate about some of the implications of developments in genetics. It was highlighted that this need was also discussed in the Human Genomics Strategy Group report,51 which cited evidence that public awareness around genetics is generally low and needs to be developed.
Some of the interviewees expressed the view that there is a need for greater public awareness of the strengths and weaknesses of genetic testing and that this public awareness would become especially important as sequencing becomes more widely available at affordable prices through the private sector. A GP respondent considered that it was only a matter of time before some of his patients came to him with their genetic profile asking for information or advice about it. An interviewee with a national perspective described a recent impromptu meeting with someone selling mobile phone applications (apps) for people to have their exomes and genomes analysed. Although possibly ‘an extreme example’, this app seller could not see any downsides to this, or any role for the specialty of clinical genetics. This interviewee considered that the specialty of clinical genetics needs to ensure that it is not seen by others as being overcautious and ‘worrying about nothing’ but as a highly experienced source of expertise on important issues such as incidental findings, consent, confidentiality and potential familial implications of the genetic testing of children.
Another interviewee, however, thought that, even with clever marketing, such private sector involvement was unlikely to take off, partly because of the problems of producing any meaningful data for the client (especially in the absence of knowledge of the person’s phenotype). However, this interviewee considered that, if ‘recreational genetics’ does become popular among the ‘worried well’, the resultant data will need to be interpreted before the results are handed to a GP.
These observations suggest that the public response to these new technologies may be diverse and that there is considerable scope for public education. One interviewee explained that, although at one time the Wellcome Trust would have been a good source of funding for such public engagement activities, they have become less inclined to do so, meaning that new sources of funding – possibly from pharmaceutical or biotech companies – will have to be found.
Importance of family support
Several respondents, especially those working directly with children undergoing diagnostic testing for LDs and their families, stressed the importance of organisations offering them support and information. One such organisation is Unique, a rare chromosome disorder support group that aims to ‘inform, support and alleviate the isolation of anyone affected by a rare chromosome disorder and to raise public awareness’ [see www.rarechromo.co.uk/html/MissionStatement.asp (accessed 9 April 2015)].
Besides developing information leaflets on specific rare chromosome disorders and practical guides on aspects of daily living, Unique fulfils many other valuable functions. These include linking families whose children have similar chromosome disorders and clinical and/or practical problems and supporting and promoting relevant research projects. It holds regional social gatherings for families in the UK and holds regular conferences and study days where families and relevant specialists can meet and be informed of the latest medical, technical and practical developments. It has developed and maintains a comprehensive computerised database detailing the lifetime effects of specific chromosome disorders on affected members whilst at all times observing the need for total confidentiality. However, an interviewee with a national perspective, who is also a representative of Unique, considered that, as the use of NGS technologies becomes more widespread, the organisation will need to grow, but ‘in order to grow and have more staff, we need more funding’. Although the charity considers that ‘for a small charity we are pretty good at fundraising’, it also recognises that its need for funding ‘is only going to get more urgent as we get more families’. In addition, one interviewee explained that Unique gets some information about diagnoses from the families and asks them to obtain a copy of their genetics report from their doctor or geneticist if they have not been given one. However, staff are unsure of their ability to interpret the results from NGS technologies.
Participants also stated that, even though widespread adoption of NGS technologies will increase the numbers and proportions of children with LD with a diagnosis, over half of the children tested are likely to remain undiagnosed. As such, another charity, SWAN [Syndromes Without A Name; see http://undiagnosed.org.uk/ (accessed 9 April 15)], may face increased demand for its services as the use of NGS technologies becomes more widespread. The aims of SWAN are to:
-
develop a community of families with undiagnosed genetic conditions for mutual support and information sharing
-
develop a network of health and social care professionals with expertise in undiagnosed conditions
-
increase awareness and understanding of undiagnosed genetic conditions.
This charity was highlighted as a potentially valuable support network for (the many) families for whom NGS technologies fail to provide a diagnosis.
In addition, although not explicitly included in the initial brief for this scoping study, several of the interviewees expressed their views on the importance of a diagnosis to families, clinicians and other professionals. These views are summarised in Appendix 6.
Changes in service commissioning
Participants also highlighted that service commissioners under the new specialist commissioning arrangements will also need a greater awareness of the potential (and limitations) of NGS technologies. Previously, with regional commissioning, there were considerable differences in accessing specialist diagnostic testing. One interviewee hoped that the development of directly commissioned services at a national level will greatly improve this situation, reducing regional variations in access, which would become based on need rather than geography.
Another interviewee considered that the Royal Colleges, NHS England and the clinical commissioning groups have important and distinct roles and responsibilities when developing awareness in the NHS about the potential of NGS technologies.
Results II: new systems and safeguards
Supporting information, in the form of additional quotations, can be found in Appendix 7.
Giving informed consent
Participants highlighted the ethical challenges and practical dilemmas associated with informed consent, particularly with respect to the handling of incidental findings. Many of these issues were also identified and discussed in the literature review (see Chapter 2). In particular, several interviewees highlighted the problems of getting patients to consent to something unknown that may not even relate to their presenting condition.
Reporting test results: managing incidental findings
Participants identified that one of the most controversial aspects of WGS is the ethical issues that may arise from its use (or indeed from the use of other NGS technologies) in clinical and research settings. The research literature base pertaining to reporting test results is discussed in Chapter 2. Almost all of the interviewees mentioned this as an area where further work is needed. The preference was for the work to be undertaken at a national level but also to draw on the experiences of other countries.
It was explained that issues associated with the amount of information from testing to give patients are not new but are exacerbated by the extremely large amounts of data generated by NGS technologies. Different countries are taking different approaches to managing these issues, for example because of medicolegal considerations the approach in the USA is completely different from that being adopted in most European countries. The Americans state that incidental findings must be disclosed whereas the European approach is to undertake only targeted testing. It was suggested that these two views ‘represent opposite ends of the spectrum with Britain expected to be “somewhere in the middle” ’. Differences may also arise between diagnostic testing for research and diagnostic testing for clinical care. In reality, there are logical reasons and justifications for the different approaches being suggested in different countries.
Ethical and other frameworks
A variety of issues pertaining to ethical and other frameworks were raised during the stakeholder interviews. These are summarised in Box 2.
-
The NHS will need to be vigilant as health services develop to ensure equity of access (i.e. that people get the same service regardless of where they live).
-
This may be difficult to manage given the relative autonomy of NHS foundation trusts but could be facilitated by a strong national and/or regional structure of genetics laboratories to deliver consistent approaches when using NGS technologies in clinical settings in the NHS.
-
It could also be promoted by forging strong links and close working relationships between hospital- and community-based paediatrics services and their regional clinical genetics service (e.g. holding regular joint clinics).
-
Equity of access does not just refer to geographical distinctions between urban and rural populations; it also concerns ethnic groups and minority populations (including those for whom English is not their first language).
-
Centrally developed pathways and ‘good practice’ guidelines could also be helpful.
-
With regard to data protection, it was suggested that people are likely to be particularly concerned about access by commercial and pharmaceutical companies, especially if private sector genetics laboratories start to be used on a routine basis by the NHS.
-
However, the importance of sharing data across laboratories (in Britain, Europe and North America) was widely recognised as being crucial for improving diagnostic yields, and formal frameworks need to be developed and supported to facilitate this in ways that are acceptable to all stakeholders.
-
Various concerns were raised about aspects such as audit, quality control, accreditation, validation, supervision and mentoring (especially of new types of staff), clinical governance, professional registration, accredited training and CPD. The importance of these issues and the resource implications of addressing them must not be underestimated.
-
It was suggested that common frameworks for reporting laboratory results could be developed to promote consistent reporting and enable quality to be monitored.
-
More research will also need to be undertaken to identify the most appropriate sequencing machines and the sensitivity and specificity of the available tests will also need refining.
One interviewee raised the need for further research on false-negative rates and stated that ‘the implications of false negatives and false positives are very different’. This interviewee stressed the importance of identifying the false-positive rate over the next few years.
In addition, the importance of a regulatory framework was raised by some of the interviewees, as was the importance of public education and public debate. However, it was also pointed out by one interviewee that ‘you could never eliminate any possibilities of misuse unless you eliminate any possibilities of use’.
Pace, and extent, of change
It was considered that Holland (in particular) and Britain are seen as world leaders in the adoption of genetic testing for clinical work. Although the technologies are available in the USA and are being used for research purposes, it was suggested by some interviewees that their relatively slow adoption there for clinical work is because of the reluctance of insurers to fund them.
Interviewees had diverse views about timescales and pace of change for introducing NGS technologies into the NHS for diagnostic work. They recognised that the technologies already exist but estimated different timescales and end points. Most considered that the focus would be on targeted gene panels for the next 3–5 years but would shift to exome sequencing thereafter. There were fewer consensuses about any subsequent wholesale move from WES to WGS. Some interviewees based their views on their personal experience of working with children with LDs; others had a wider perspective on the clinical use of NGS technologies in the NHS. The variations in their views are reflected in the quotes included in Appendix 7.
It was generally considered that there would not be a national strategy for the widespread introduction of NGS technologies into NHS clinical work, resulting in developments occurring in an ad hoc, unco-ordinated way. With regard to introducing changes specifically for the clinical diagnosis of children with LDs, it should be noted that the technology for microarrays was available for several years before the NHS adopted it routinely as the first-line test instead of karyotyping. The same may also apply to the move to NGS technologies, especially without a national strategy. One interviewee neatly summarised one of the challenges of working in genetics thus:
Genetics . . . you think you understand it, you think you have got there, you think you have got your head round everything and you totally understand whether something is clear or not, and then things move on and you realise that actually things are even more complicated than you thought . . . . And even things that you thought were certain maybe are not quite as certain as you believed them to be.
Genetic specialist, NHS
Chapter 4 Economics
Introduction
This chapter considers the published cost-effectiveness evidence relating to the use of genetic testing technologies to diagnose LD in children and then presents relevant cost and resource use data. The lack of available information meant that it was not possible to undertake a comprehensive assessment of cost-effectiveness. This chapter therefore concludes with some key points that decision-makers may find helpful when considering commissioning an economic evaluation to assess whether using NGS technologies to diagnose LDs in children can offer value for money.
Methods
The methodology used to undertake the review of the literature is presented in Chapter 2. In addition to material from the literature, information has also been obtained directly through personal communication with experts.
Results I: review of the cost-effectiveness literature
Cost-effectiveness of comparator genetic testing technologies
The material in Appendix 3 shows that various studies have considered the effectiveness of microarray analysis (compared with other forms of testing such as karyotyping). However, only a few have considered the cost-effectiveness of such approaches. Information presented in this section highlights the fact that the studies that do exist were generally undertaken several years ago and not all took place in Britain.
A paper by Wordsworth et al. 25 published in 2007 considered the cost-effectiveness of microarray technology in the NHS for diagnosing ILD. The authors considered that, at that time, one obstacle to the adoption of aCGH in the NHS was concern over the proportion of confirmed genome imbalances for which the significance of the positive result was unknown (e.g. very small de novo imbalances and some inherited imbalances). However, they thought that the most significant obstacle was the perception that the technology was prohibitively expensive for use in most NHS cytogenetics laboratories. They therefore investigated the cost-effectiveness of aCGH compared with standard cytogenetic analysis for detecting genomic imbalances that diagnose ILD in the NHS.
They found that the average cost of aCGH was £442 per (single) patient sample and the average cost of karyotyping was £117 per sample (with the majority of the cost difference being accounted for by the array cost). Therefore, from a single-test perspective, aCGH was indeed more expensive than karyotyping.
However, the authors took the view that, in reality, the situation was more complex because information regarding subsequent tests for genomic imbalance must also be considered before estimates of true cost-effectiveness can emerge. They calculated that the overall cost per diagnosis of the karyotyping route, including a single multi-telomere FISH assay (£4957), was more expensive than the overall cost per diagnosis of the aCGH route (£3118), which yielded 10% more diagnoses. However, if the less conservative yield of 15% more diagnoses is correct, then the aCGH cost reduces to £2440 per diagnosis. This figure is more comparable to the cost of karyotyping plus the alternative multi-telomere assay, MLPA (£2129 per diagnosis). However, the authors state that 92% of cases tested by karyotyping and a multi-telomere assay will require further tests for a diagnosis. By contrast, they suggest that the aCGH route is unlikely to require further genome-wide tests for genome imbalance.
Wordsworth et al. 25 considered that their study had shown that it was viable for the NHS to use genome-wide aCGH for the diagnosis of ILD and that, in some cases, it might be appropriate to replace karyotyping with aCGH as the first-line test for genomic imbalance in ILD. They suggested that samples shown to be normal following aCGH could be karyotyped to identify truly balanced rearrangements or further characterise genome imbalances. Alternatively, aCGH could be useful for clarifying previous equivocal karyotyping results. They concluded that testing for genomic imbalances in ILD using microarray technology is likely to be cost-effective as an earlier diagnosis saves the cost of additional tests and a negative result minimises the choice of follow-up tests.
In the same year, in the UK, Newman et al. 10 published an exploratory cost–consequences analysis of using aCGH for the diagnosis of DD. They defined some of the resource cost implications of introducing aCGH into clinical practice in terms of avoiding or reducing the use of other investigations required to obtain a diagnosis. The medical notes for 46 consecutive patients selected for aCGH analysis by six experienced dysmorphologists at the Regional Genetics Service in Manchester were abstracted for all clinical investigations relating to the determination of the cause of LDD.
Newman et al. 10 found that the number of investigations undertaken on each child varied. With aCGH estimated to cost £590 per case, they found that the additional cost would be £2399 per positive case if aCGH had been undertaken after negative standard initial tests for LDD investigation. If the cost of aCGH was reduced to £256 per case, aCGH would become cost neutral. They also found that all chromosomal anomalies detected by aCGH had a de Vries score of ≥ 5. If aCGH had been used only for individuals with a score of ≥ 5, the cost would be £1087 per positive case identified and the sensitivity would increase considerably.
Newman et al. 10 also stated that the economic implications of introducing aCGH should be considered in the context of other diagnostic tests for LDD. For example, only about 0.6% of males undergoing fragile X syndrome mutation will be positive for a full mutation. In their laboratory, > 700 samples were screened for fragile X syndrome each year. The use of this simple, inexpensive genetic test (£63 per case) equated to a cost of > £11,000 per positive case identified. Despite these cost implications, fragile X screening for LLD was considered standard clinical practice. This suggested to the study authors that performing aCGH on selected patients with LLD would be ‘an appropriate intervention in both clinical and economic terms’.
With regard to the cost-effectiveness of diagnostic testing, Miller et al. 52 considered that, except in certain cases, for example those involving a family history of multiple miscarriages, a karyotype was not cost-effective in a child with DD/ID, ASD or MCA and a negative array study. They also considered that, although CMA testing was not inexpensive, the cost was less than the cost of a G-banded karyotype plus a customised FISH test such as subtelomeric FISH and the yield was greater.
Trakadis and Shevell53 used Canadian data to consider the cost-effectiveness of microarray as a first genetic test in GDD. They evaluated the cost-effectiveness of aCGH compared with karyotyping by retrospectively analysing the cost of work-up in a cohort of 114 children representing a consecutive series of children diagnosed with GDD. Their costing approach also included considering the costs of using different types of laboratories. In a similar manner to Wordsworth et al. ,25 they focused on the cost per diagnosis detected rather than the cost per life-year or quality-adjusted life-year gained, which are standard measures often used in economic evaluation. This was because the diagnosis of GDD is highly unlikely to save lives and evaluating quality-adjusted life-years is intrinsically problematic in children, especially those with GDD. They concluded that ‘aCGH would be cost-effective as a first genetic test in the clinical evaluation of individuals with GDD’53 (p. 994).
Lynch24 stated that, although the cost of array testing was initially prohibitively expensive, the cost has reduced over time. She considered that the test was now affordable and also saved costs as in many cases it results in an earlier diagnosis, thus removing the need for second-line diagnostic investigations, including invasive investigations such as brain imaging and muscle biopsy. She also suggested that, as they become established over time, ‘microarrays should result in fewer hospital appointments and fewer additional (now unnecessary) diagnostic investigations’24 (p. 971). However, she also stated (p. 971) that these ‘hidden’ benefits are difficult to cost for several reasons:
-
the ordering of first and second-line investigations by doctors differs not only between countries but also between hospitals (arrays will bring less financial benefit the more first-line investigations are ordered)
-
some children with an array anomaly may require additional investigations and it is difficult to estimate how many investigations can be avoided
-
it is not unusual for paediatricians to routinely order Fragile X testing at initial assessment; however, it is hoped that, in time, these requests will only be activated should the array be normal
-
many CNVs will be detected and it will require parental analysis to confirm whether each identified CNV is benign, thus adding to overall costs
-
it is difficult to estimate health-care savings as, even with a diagnosis, these children will still require long-term support from health-care providers
-
the majority of children will test negative on array and will need further investigation.
Lynch24 also mentioned the benefits associated with a diagnosis. These are explored in more detail in Appendix 6 but of particular note is the long-lasting emotional relief for parents and the fact that having a diagnosis generally facilitates the receipt of social and educational support.
Regier et al. 5 explored whether using array genomic hybridisation (AGH) for diagnostic testing of the genetic causes of ID provides value for money. The term AGH is used in the generic sense to encompass the class of array technologies that detect submicroscopic CNVs. The authors used decision analysis modelling to evaluate the trade-off between costs, clinical effectiveness and benefits of an AGH testing strategy compared with a conventional cytogenetic testing strategy. Using Canadian cost data for 2007/8, they found that the baseline AGH testing strategy led to an average cost increase of $217 [95% confidence interval (CI) $172 to $261] per patient and an additional 8.2 diagnoses in every 100 patients tested (0.082; 95% CI 0.044 to 0.119). The mean incremental cost per additional diagnosis was $2646 (95% CI $1619 to $5296). Probabilistic sensitivity analysis demonstrated that there was a 95% probability that AGH would be cost-effective if decision-makers were willing to pay $4550 for an additional diagnosis. The authors concluded from their analysis that using AGH instead of conventional karyotyping followed by FISH for most ID patients provides good value for money. Their model also showed that obtaining a karyotype for all children with ID and then testing with AGH if the cytogenetic analysis did not provide a diagnosis was ‘not cost beneficial when compared to using AGH as first-line diagnostic testing’5 (p. 771).
Cost-effectiveness of next-generation sequencing technologies
The costs of undertaking diagnostic testing using WGS and WES are steadily falling and the diagnostic yields are anticipated to improve over time as the technologies become more established. There are therefore no (rigorous) published studies comparing the costs and effectiveness of these technologies with those of more traditional diagnostic techniques.
Some work on the cost-effectiveness of NGS technologies has been undertaken in Holland, often focusing on WES and/or targeted gene sequencing. Heger31 reports in Clinical Sequencing News on the early experience of a group (led by Joris Veltman at the Radboud University Nijmegen Medical Centre) investigating the cost-effectiveness of exome sequencing.
Towne et al. 54 used American data to measure the cost-effectiveness and efficiency of WES/WGS compared with targeted gene analysis in a paediatric population with an undiagnosed potentially genetic disease. This work is in its preliminary stages. Genetic diagnoses had been identified in 22% of 45 families when initial findings were presented at the 2013 meeting of the American Society of Human Genetics. The authors concluded that their preliminary data suggest that, compared with targeted sequencing, WES/WGS is an efficient and probably cost-effective way of diagnosing the genetic basis of disease. However, they highlighted that their findings are reflective of research testing rather than testing in a clinical laboratory. Furthermore, as the study is in its early stages and is being undertaken in an American context, it is not currently clear whether its findings will be transferable to other countries.
Results II: cost data
This section includes information on the costs of tests that may currently be used in the diagnostic process and also the cost of genetic counselling.
Diagnostic test costs
In some cases it will be possible to make a diagnosis (with or without subsequent confirmatory gene testing) simply by taking a full medical history and examining the child. In other cases the medical history and examination will inform which tests should be carried out. For children who present without a suggested observation-based diagnosis, the paediatrician is likely to order a number of first-line tests. If these do not result in a diagnosis, a number of more specialised second-line tests may be requested. If the second-line tests still do not yield a diagnosis then third-line testing may be carried out on a proportion of children. A ‘representative’ list of potential tests and their NHS costs (Dr Kay Metcalfe, Central Manchester University Hospitals NHS Foundation Trust, 2014, personal communication) is included in Table 6; available information on the cost of genetic tests is provided in Table 7.
Test | Cost (£) | Source | Date |
---|---|---|---|
First line | |||
Full blood count | 5.59 | Dr Kay Metcalfe, personal communication | 2014 |
U&Es | 6.60 | Dr Kay Metcalfe, personal communication | 2014 |
Thyroid function | 5.84 | Dr Kay Metcalfe, personal communication | 2014 |
Ferritin | 11.00 | Dr Kay Metcalfe, personal communication | 2014 |
Vitamin D | 9.98 | Dr Kay Metcalfe, personal communication | 2014 |
Karyotype | 125.00 | Addenbrooke’s Hospital Genetics Laboratories55 | 2014 |
Fragile X | 145.00 | Addenbrooke’s Hospital Genetics Laboratories55 | 2014 |
Urine amino acids | 52.02 | Dr Kay Metcalfe, personal communication | 2014 |
Urine organic acids | 79.07 | Dr Kay Metcalfe, personal communication | 2014 |
Total | 440.10 | ||
Second line | |||
Lactate | 18.00 | Sheffield Children’s NHS Foundation Trust56 | 2013–14 |
Urine MPS screen | 79.07 | Dr Kay Metcalfe, personal communication | 2014 |
White cell enzymes | 226.80 | Dr Kay Metcalfe, personal communication | 2014 |
Very-long-chain fatty acids | 83.00 | Sheffield Children’s NHS Foundation Trust56 | 2013–14 |
MRI brain | 180.65 | Department of Health57 | 2012–13 |
Total | 587.52 | ||
Third line | |||
Transferrin isoelectric focusing | 64.51 | Dr Kay Metcalfe, personal communication | 2014 |
7-Dehydrocholesterol | 86.35 | Dr Kay Metcalfe, personal communication | 2014 |
Acylcarnitine | 82.19 | Dr Kay Metcalfe, personal communication | 2014 |
Biotinidase | 54.10 | Dr Kay Metcalfe, personal communication | 2014 |
Cerebrospinal fluid, lactate and glucose | 13.02 | Dr Kay Metcalfe, personal communication | 2014 |
Electroencephalogram, if relevant | 386.00 | Department of Health57 | 2012–13 |
Total | 686.17 |
Test | Cost (£) | Source | Comment |
---|---|---|---|
Karyotyping | 125 | Addenbrooke’s Hospital Genetics Laboratories55 | Peripheral blood: routine G-branded karyotype |
aCGH | 180 | Addenbrooke’s Hospital Genetics Laboratories55 | Peripheral blood: microarray: 60Ka |
Targeted gene panels | 530 | UKGTN58 | Genetic test panel for cerebral malformation disorders |
WES | 500 | Wright et al.33 | |
WGS | 4000 | Wright et al.33 |
Genetic counselling cost
Information on the cost of nurse counsellors undertaking genetic counselling is included in a study that explores ways of improving the referral process for familial breast cancer genetic counselling. 59 This study, which took place in two locations (one in Grampian, Scotland, and one in Wales), assumes that breast cancer genetic counselling consumes a similar level of resource to other forms of genetic counselling. Costs are divided into four categories, namely staff, consumables, rooms and equipment. The study reports that staff estimated that they allocated approximately 1 hour per patient during the clinic appointments and that an additional hour was required for preparation. Furthermore, there were various indirect costs. These were largely for meetings, such as weekly clinic meetings, during which all genetic patients were discussed. In addition, in both study centres, nurse counsellors were required to meet a consultant geneticist to discuss patient cases and determine the need for any further counselling and/or management recommendations (30 minutes in Grampian and 90 minutes in Wales). In the control arm in Grampian, three specialist registrars each met with a consultant geneticist for 20 minutes per month to discuss patient cases; in the control arm in Wales, a clinical assistant met with the consultant geneticist for approximately 1–2 hours each month. The staff, consumables, rooms and equipment costs are displayed in Table 8 at 2001 prices, with costs converted to 2013 prices using the Hospital and Community Health Services Index. 60
Resource | Grampian | Wales | 2013 estimatesa | ||
---|---|---|---|---|---|
Intervention (£) | Control (£) | Intervention (£) | Control (£) | ||
Staff | 63.26 | 78.92 | 90.55 | 79.66 | 114.90 |
Consumables | 2.11 | 2.11 | 2.11 | 2.11 | 3.10 |
Rooms | 26.59 | 26.59 | 26.59 | 26.59 | 39.12 |
Equipment | 2.12 | 2.12 | 2.12 | 2.12 | 3.12 |
Total | 94.08 | 109.74 | 121.37 | 110.48 | 160.24 |
Key points for decision-makers planning to commission an economic evaluation
There are challenges associated with carrying out all economic evaluations. Particular issues associated with undertaking economic evaluations to assess the cost-effectiveness of using NGS technologies to diagnose LDs in children are considered under the following headings:
-
establishing the value of using NGS technologies to diagnose LDs
-
establishing the effectiveness of NGS technologies
-
impact of a diagnosis on the NHS
-
impact of a diagnosis on the family
-
wider economic implications of using NGS technologies to diagnose LDs
-
estimating production and non-production costs associated with NGS technologies
-
other considerations.
Establishing the value of using next-generation sequencing technologies to diagnose learning disabilities
Next-generation sequencing technologies are used not only for the diagnosis of LDs but also for the diagnosis of a plethora of other conditions, including cancers, cardiac problems and neurological conditions. It is therefore difficult to estimate how much of the value of NGS technologies should be attributable to their role in diagnosing LDs and how much should be attributable to other roles. Furthermore, as LD panels are still in their infancy and WES and WGS are currently being used only in research settings, it is difficult to determine the value of NGS technologies to the NHS.
Establishing the effectiveness of next-generation sequencing technologies
Finding good evidence regarding the performance of diagnostic tests and interpreting its value for practice is more challenging and less straightforward than for interventions. 61 Most diagnostic studies focus on a test’s ability to discriminate between people with and people without a particular condition. In the case of genetic testing for LDs the case is complicated because the test has the potential to identify not just one condition but a number of different conditions. Furthermore, having a highly accurate test does not necessarily improve a patient’s outcome, although it may avoid (or limit) the necessity of carrying out further investigative procedures.
For children who present without a diagnosis the paediatrician is likely to order a number of investigative tests. There is currently no information on the number of children who receive each available diagnostic test or the diagnostic yield of these tests. The issue is complicated by the fact that diagnostic yield is influenced by the position of the test in a patient’s diagnostic pathway, which in turn may be correlated with the time span over which a diagnosis has been sought.
Identifying an unambiguous diagnostic yield for a particular technology is far from straightforward, if not impossible. A key factor is that comparing findings from different studies is difficult because of the differences in technology used as well as the differences in the characteristics of the patient groups involved in the studies. The current situation is that, although there are numerous studies reporting diagnostic yield for karyotyping and aCGH for specific populations, it is difficult to generalise results to the population with LD with any degree of certainty. There is even less certainty with regard to the diagnostic yields from NGS technologies.
Three particular limitations of NGS technologies have been highlighted (Professor Frances Flinter, 2013, personal communication):
-
NGS technologies generate poorer quality of reads than Sanger sequencing, producing an error rate of 1–1.5%.
-
Errors in machine or panel manufacture lead to false-negative results. The false-negative rate for NGS technologies is unknown but it is thought to be high and to be the greatest problem for NGS technologies at the present time.
-
The technology has yet to accurately identify CNVs (insertions/deletions/duplications) because of the short reads. Methods for detecting CNVs do not meet the standards required for a clinical diagnostic test, for example reads that fall in repetitive regions of the genome cannot be mapped unambiguously to the reference sequence.
Furthermore, although using NGS technologies to generate data is relatively straightforward, the analysis of that output to produce clinically useful information is still an evolving area of expertise. Until diagnostic yield, or another efficacy measure, is established for NGS technologies and their comparators, it will not be possible to establish effectiveness with any degree of certainty.
Drummond et al. 62 have published a paper that includes details of particular challenges faced when attempting to establish the cost-effectiveness of devices. The authors of this paper note that those undertaking economic evaluations are often quick to ‘genericise’ their recommendations unless there is specific evidence to differentiate products. Whether or not this is an issue in relation to using NGS technologies to diagnose LDs is unclear. WES and WGS appear to be a ‘step change’ from more established forms of genetic testing. However, sequencing machines made by different manufacturers do not generate identical output data and therefore, if a study comes to conclusions based on the output from one manufacturer’s machine, these findings may not hold with respect to practice using a machine made by a different manufacturer. In addition, as technology in this field is developing very rapidly, it may not be possible to generalise study findings from an earlier version of a machine to those from a later, updated version.
Impact of a diagnosis on the NHS
From a NHS perspective, a diagnosis can be cost-effective only if it leads to improved treatment, which, in turn, leads to improved outcomes for patients. The extent to which care and treatment may be modified as a result of a diagnosis is very variable and is likely to depend on the specific diagnosis. In some cases there will be no change in treatment, with clinicians simply continuing to treat the symptoms. In other cases the diagnosis may result in ongoing monitoring for, say, heart disease and/or changes in the availability of social care provision and educational support. If there is no change in treatment as a result of a diagnosis then there may be no cost savings to the NHS other than those associated with ongoing attempts to obtain a diagnosis.
In addition, it is important to consider that a diagnosis sometimes leads to further tests and that some of these tests need to be repeated throughout life. So, for example, a diagnosis of Down syndrome would be accompanied by an appointment for an echocardiogram, if the child had not already had one, and would lead to regular thyroid function tests (minimum of 2 yearly throughout life), regular hearing and vision checks and a low threshold for investigating haematological malignancy or coeliac disease. However, the extent to which an individual might have some, or all, of these tests despite not having a diagnosis is unclear.
The extent to which further confirmatory genetic testing may be carried out following a diagnosis depends on the condition that has been diagnosed. Similarly, the extent to which the child’s diagnosis leads to genetic testing (and counselling) of family members is condition specific. In the case of family members the issue is complicated by the fact that family members’ wishes will also influence the extent of any testing (and counselling).
All of these tests (and any counselling) have supporting activities (e.g. outpatient appointments) and the cost of these needs to be taken into account in any economic evaluation. In addition, if an economic evaluation takes a perspective wider than that of the NHS, then the average cost to families of attending such appointments (as well as any costs associated with not having a diagnosis) needs to be quantified.
Impact of a diagnosis on the family
Although the value of a diagnosis was not explicitly included in the initial brief for this scoping study, several of the interviewees expressed their views on the importance of a diagnosis to the families, clinicians and other professionals. These views, which are not necessarily comprehensive, are provided in Appendix 6. Some views from the published literature are included in Tables 3 and 4. There is currently no published quantitative information on the benefit of a diagnosis to the family of a child with LD. In addition, there is a lack of information on the cost consequences of a diagnosis (or lack of one) for subsequent family planning decisions.
Wider economic implications of using next-generation sequencing technologies to diagnose learning disabilities
The introduction of NGS technologies has wide economic implications in terms of both technological infrastructure and training and education.
With regard to technological infrastructure, feedback from the interviewees suggests that the biggest issue relates to storing the huge amount of data that the use of NGS technologies can generate. This has implications in terms of needs in relation to both computer hardware and computer software. There are also associated considerations around data confidentiality and the length of time that data should be stored. It has been suggested that the costs associated with processing, transfer and storage issues may be more than the costs associated with producing the data (Professor Frances Flinter, 2013, personal communication). These are all costs to the NHS, which are difficult to quantify. Moreover, these costs are likely to change substantially over time. Although these costs are very uncertain, ignoring them underestimates the cost of introducing NGS technologies into the LD diagnostic pathway.
Some storage costs could be avoided if a policy of not storing data (but rather resequencing on occasions when it was deemed appropriate to revisit a child’s genetic code) was introduced. However, there is still currently a need to store considerable amounts of data for research purposes, that is, so that it can be used to help researchers distinguish between genetic abnormalities and normal variation.
In terms of training and education, information from the interviewees, as discussed in Chapter 3, suggests that there would be a need for more bioinformaticians and more genetic counsellors. In addition, interviewees considered that a wide range of health-care professionals would need to know more about genetic testing.
Estimating production and non-production costs associated with next-generation sequencing technologies
Production costs
The production costs of NGS technologies appear to be changing on an almost daily basis. For many years the National Human Genome Research Institute (NHGRI) in the USA has tracked the costs associated with DNA sequencing performed at the sequencing centres that they fund. They present data since 2001 for two metrics: the cost per megabase (a million bases) of DNA sequence and the cost per genome. These data are shown in Figures 1 and 2 respectively. 63 Both graphs use a logarithmic scale on the y-axis. It is noteworthy that the sudden outpacing of Moore’s law (the normal expectation of the rate of change in the cost of technology over time) beginning in January 2008 corresponds to the time when the sequencing centres transitioned from Sanger-based to next-generation DNA sequencing technologies.
FIGURE 1.
Cost per raw megabase of DNA sequence. Note: Moore’s law is the observation that, over the history of computing hardware, the number of transistors or integrated circuits doubles approximately every 2 years. Technology improvements that follow the same trajectory as Moore’s law are widely regarded to be doing exceedingly well. Source: Wetterstrand. 63 Reproduced with permission.
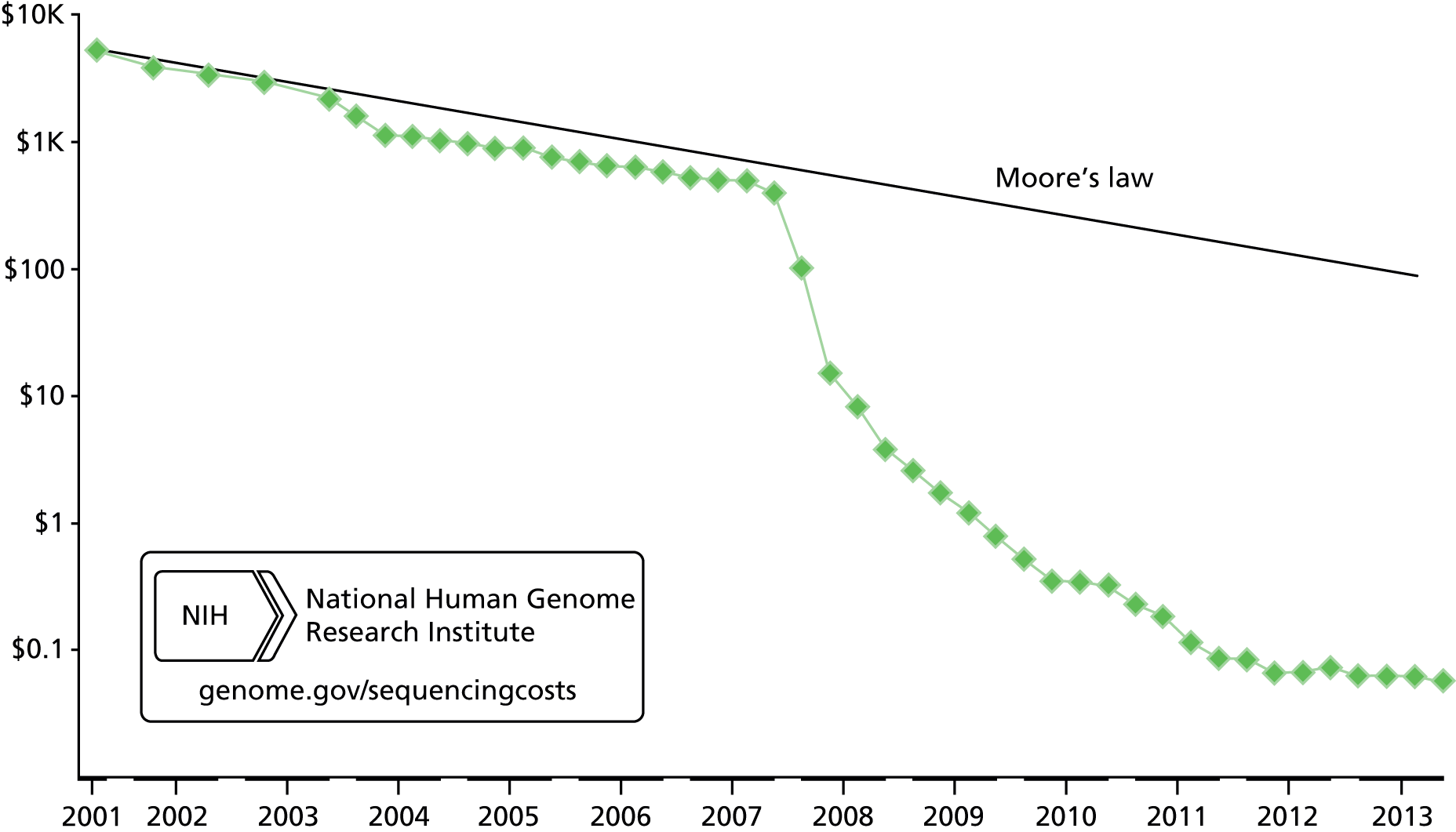
FIGURE 2.
Cost per genome of DNA sequence. Note: Moore’s Law is the observation that, over the history of computing hardware, the number of transistors or integrated circuits doubles approximately every two years. Technology improvements that follow the same trajectory as Moore’s Law are widely regarded to be doing exceedingly well. Source: Wetterstrand. 63 Reproduced with permission.

Furthermore, the PHG Foundation report Next Steps in the Sequence28 points out that:
-
new technologies have an associated ‘learning curve’ whereby the efficacy improves over time
-
laboratory protocols and the knowledge created from the use of the technology are constantly evolving
-
there are new advances in knowledge capital being made that have an inherent and tangible value which needs to be taken into consideration in any economic assessment, especially if this knowledge is not publicly shared and must be paid for through normal market-based exchange.
The authors of this report conclude that, over the next few years, as the technology continues to evolve, it is unlikely that there will be a period when the technology is sufficiently stable to allow meaningful comparisons over a given time frame. 28 An added issue is that the stabilisation of current NGS technology prices may (at least in part) be because they are being replaced by ‘next NGS’.
Non-production costs
Figures 1 and 2 include only the costs associated with ‘production activities’, that is, those activities that are essential to the routine generation of large amounts of quality DNA sequence data that are made available in public databases. Non-production costs have not been included. Table 9 displays the production costs that are included in Figures 1 and 2, along with the non-production costs that have been omitted.
Production costs (accounted for in Figures 1 and 2) | Non-production costs (not accounted for in Figures 1 and 2) |
---|---|
|
|
The price that is charged when NGS technologies are regularly used in NHS practice will need to take these non-production costs into account. The magnitude of a number of these costs (e.g. management of individual sequencing projects and quality assurance) would differ if the tests were carried out for commercial rather than for research purposes. Furthermore, costs will depend on the number of laboratories involved and the possibility of realising economies of scale.
The total costs to the NHS of carrying out a test depend not only on generating test data but also on the time taken, and resources used, to analyse and interpret the resultant data. As an example, turnaround times for Oxford Medical Genetics Laboratories are displayed in Table 10 (Kate Thomson FRCPath, Oxford Medical Genetics Laboratories, 2014, personal communication).
Genetic test | Turnaround time (working days) |
---|---|
Karyotype | 28 |
aCGH | 28 |
Targeted gene panel | 60–80, with a target of 40 for smaller panels |
WES | Not yet in service but suspect the aim will be max. 80 |
WGS | Not yet in service but suspect the aim will be max. 80 |
Other considerations
As well as NHS and personal social services, a person with LD may need help from other services, including education, housing, respite care and the benefits system. Furthermore, informal carers may carry significant burdens not only in terms of time input but also in terms of private expenditure. In addition, there may be further costs associated with loss of productivity as a result of unemployment or absence from work by carers. Moreover, knowledge of genetic risk carries potential harm and benefit to family members. 64 All of these costs are very hard to measure, primarily because they are difficult to generalise.
When designing an economic evaluation careful consideration needs to be given to the perspective of the evaluation. There is a trade-off between including all relevant costs and benefits (i.e. a societal perspective) and having to make large simplifications and/or very broad assumptions because of uncertainty and taking a very narrow view that focuses only on known costs and benefits.
A similar trade-off needs to be made when considering the time frame for the economic evaluation. One extreme would be to focus on the short period over which costs and benefits can be measured. The other extreme would be to adopt a lifetime horizon (in line with the National Institute for health and Care Excellence reference case65) and include bold assumptions and generalisations. A compromise between these two positions may be the best option.
Chapter 5 Discussion
This scoping study set out to explore the cost-effectiveness of NGS technologies compared with traditional genetic testing for the diagnosis of LD in children. This proved to be a considerable challenge.
Limitations of the method
The study employed two main research methods: a review of published articles and reports and interviews with leading stakeholders.
Formal searching for published studies and reports was complicated by the plethora of conditions that are categorised as a ‘LD’, as well as by the number of potentially relevant ‘genetic terms’ that could be used in a search strategy. A large number of hits were generated by the searches but much of this material proved to be irrelevant. However, supplementation of the results from the electronic searches with articles and reports recommended by the interviewees, hand searching and searching of the bibliographies of informative studies allows confidence that all (or at least the majority) of relevant important studies have been identified. Confidence is enhanced by the fact that there was considerable overlap between what was said during the stakeholder interviews and the findings reported in the literature, although, in some cases, this overlap may be because the interviewees were the authors of some of the key publications.
In a scoping exercise such as this, identification of appropriate individuals to interview is necessarily pragmatic. In effect, a ‘snowballing’ approach was employed. Initially, a number of key individuals were identified, through their recent publications or from information about their work posted on the internet. Interviews were arranged with these individuals and they were asked to suggest colleagues with relevant expertise or experience who might also be willing to be interviewed. This approach, although necessarily biased towards those with an interest in this area, prevented unnecessary time being expended trying to set up interviews with individuals who had little to contribute. The list of interviewees (see Appendix 4) shows the breadth and depth of their expertise. They included not only people with a local focus and specialist expertise but also a number of individuals with a national focus, as well as an internationally renowned professor from Holland who was able to provide a European perspective. Although patients and the public were not directly involved in this study, their views were relayed by clinicians and also by those representing the key voluntary organisations operating in this field.
Although a rigorous approach to the searching of databases for literature was employed, a systematic literature review was not undertaken. During protocol development the view was taken that the use of systematic review methods in a scoping study such as this could lead to important information being excluded. However, it is noted that, at the same time, the narrative approach employed here could lead to bias.
Were the objectives addressed?
This scoping study set out to address four objectives:
-
describe current pathways that involve the use of genetic testing
-
collect stakeholder views on the changes in service provision that would need to be put in place before NGS could be used in clinical practice
-
describe the new systems and safeguards that would need to be put in place before NGS could be used in clinical practice
-
explore the cost-effectiveness of using NGS compared with conventional genetic testing.
Objectives 2 and 3 were relatively straightforward to explore. However, objective 1 (describe the current pathways that involve the use of genetic testing) and objective 4 (exploring cost-effectiveness) were more difficult to address. Describing the current pathways was problematic because of the plethora of different conditions that are categorised as a ‘LD’ and the accompanying variation in presenting symptoms, meaning that diagnostic pathways are largely a matter of clinical judgement. In contrast, addressing objective 4 was problematic because of the paucity of cost-effectiveness evidence (for both NGS technologies and their potential comparators) and the very limited cost data. Undertaking an assessment of cost-effectiveness or value for money was therefore not possible. So, instead, information was presented that will help inform the design of research to determine whether using NGS technologies to diagnose LDs in children offers value for money.
Advantages and disadvantages of different approaches to genetic testing
This scoping study has found that, for children for whom a genetic test is considered appropriate, five broad genetic testing options can be identified, namely karyotyping, aCGH, targeted gene panels, WES and WGS (with these last three being categorised as NGS technologies). Karyotyping has been used in the NHS for many years as the first-line test in clinical practice for diagnosing LDs in children. However, this approach is being replaced by aCGH (unless karyotyping is specifically indicated). This move is supported by evidence from a considerable body of published studies (from Britain, other European countries and North America) showing the superior diagnostic yield of aCGH in most situations. Although specific studies of the cost-effectiveness of these two approaches are limited, the broad consensus in the published literature is that, as well as increasing the number of diagnoses, aCGH will reduce the overall number of tests required, thus reducing the length of the ‘diagnostic odyssey’ for many children and the family stresses associated with this. However, even with more widespread use of aCGH, many children (probably about 75–80%) will remain without a clinical diagnosis. Although for many children having a diagnosis may not have much effect on the medical management of their condition, it can have important wider influences. These include facilitating access to specialised education and social support, helping families cope emotionally and informing future decisions about family planning.
For many years Sanger sequencing has been the backbone of DNA technologies, but it has some limitations (e.g. it cannot be used to sequence highly repetitive regions of the genome) and it is recognised as being time-consuming and costly. The move to using NGS technologies promises many benefits (e.g. with regard to speed and efficiency), but these technologies are also not without their shortcomings. A useful overview of NGS methodology and application is provided by Grada and Weinbrecht. 66
Some of the shortcomings associated with NGS technologies relate to the sheer number of data that are generated, especially by WES and WGS. These data need processing, analysing and storing, thus placing considerable pressures on laboratory requirements for skilled staff and computing capacity. Other shortcomings encompass technical issues, such as unknown (but too high) false-positive rates and poorer-quality reads compared with those from Sanger sequencing, resulting in misread nucleotides and a higher error rate.
These (and other) shortcomings are being revealed through the use of NGS technologies for research purposes (including studies of conditions such as cancers and heart disease). They are being addressed on an ongoing basis by the machine and panel manufacturers, working closely with researchers, clinicians and laboratories. Because of these constant developments, it is not possible to accurately identify the improved diagnostic yields offered by the NGS technologies for children with LDs or predict what these might be if the technologies were adopted by the NHS for use in clinical settings. However, preliminary indications are that NGS technologies could achieve diagnoses for an additional 10–15% of children. Furthermore, accurate costs for such testing cannot be identified as costs have decreased rapidly in recent years and are likely to continue to do so.
The broad consensus from this study is that targeted gene panels or WES will be adopted into NHS settings in the next 3–5 years (or the next 5–10 years according to more cautious interviewees). Most (although not all) of the interviewees thought that, at least within the foreseeable future, WGS would be used only for research purposes. As well as being less accurate than targeted gene panels and WES, it was considered that WGS generates too much irrelevant data for clinical use. Storing data generated by NGS technologies raises several interesting dilemmas. Should such data be stored (at considerable expense) so that it can be re-examined when the body of genetic knowledge has grown sufficiently to make revisiting it appropriate? If so, for how long should it be stored? Are there other potential storage options, such as cloud technology, and would these be acceptable to the general public? Would it be simpler, and more cost-effective, to simply resequence a patient’s DNA when necessary/appropriate rather than store the data? This latter approach may become increasingly attractive as the cost of sequencing continues to fall.
Implications for practice
It seems likely that there will be a period of overlap between using aCGH and using NGS technologies if/when targeted panels and/or WES are introduced into NHS clinical settings (see, for example, Hughes et al. 67). Such an overlap would enable further refinements to be made to the NGS technologies and allow NHS personnel to build up their knowledge and confidence in them, but the long-term cost and resource requirements of using both platforms routinely are unlikely to be sustainable.
National and international databases would be needed to facilitate interpretation of the vast amounts of data (often from very rare conditions) that would be generated by the NGS technologies. The interpretation and storage of NGS data would need to be concentrated in (and, if cloud technology is used for storage, managed by) a selection of the NHS regional genetics centres.
As well as additional education and training courses to develop clinical bioinformaticians, there would also be a need for more genetic counsellors. Furthermore, a considerable amount of time would need to be invested in informing patient-facing staff of the implications for their work of developments in genetic testing. Public awareness raising of the potential impact of greater access to genetic information would also need to be promoted. As more children undergo testing, organisations supporting families of children both with and without a diagnosis would be expected to face additional demands for information and treatment and would require more funding.
Finally, it would be important to ensure equitable access to the new technologies for diagnosing LDs in children. The NHS regional genetics centres currently have good links with their local hospitals at a regional level, but there would be a need to ensure that some regions do not lag too far behind others when introducing these new approaches.
Chapter 6 Conclusions and recommendations
Next-generation sequencing technologies are at an early stage of development and it is too soon to say whether or not they can offer value for money to the NHS as part of the LD diagnosis process. However, if NGS technologies were introduced into the NHS for this purpose there would need to be a substantial number of organisational changes. In addition, a number of new systems and safeguards would be required to ensure that the new technologies operated in a safe and equitable manner.
Recommendations for future research
For different subgroups of the population suspected of having a LD, and for different NGS technologies in different places in the diagnostic pathway, research is needed to establish:
-
analytical validity (i.e. how well a genetic assessment measures the property or characteristic it is intended to measure)
-
clinical validity (i.e. the accuracy with which a test identifies a patient’s clinical status)
-
clinical utility (risks and benefits resulting from test use)
-
resource use and costs
-
the quantitative value of a diagnosis to the individual being tested and his or her family.
This field of research is moving very quickly. Therefore, almost every study that is undertaken will be out of date by the time it is published. This means that to maintain the value of research, regular updates need to be carried out.
Acknowledgements
The research team would like to thank everyone involved in this scoping study for their time and considered contributions. This includes members of the research advisory group and all of the stakeholders who participated in the telephone interviews. Particular thanks go to Professor Jill Clayton-Smith (Consultant Clinical Geneticist and Honorary Professor in Medical Genetics) for her help in formulating the protocol and recruiting the research advisory group members, Dr Karen Horridge [Consultant Paediatrician (Neurodisability)] for her comments on the draft report and Dr Sarah Wynn (Information Officer, Unique) for her comments on the draft report and her contribution to the project workshop. In addition, we are very grateful to Dr Kate Thompson (Principal Clinical Scientist) for providing information about the turnaround times for genetic tests. We are also extremely grateful to Dr Kay Metcalfe (Consultant Clinical Geneticist) and Dr Maria Hall (Specialist Registrar in Paediatrics) who made themselves available on an ongoing basis over the course of this project to provide expert advice and information. The authors would also like to thank Dr M Gemma Cherry for the time and effort she has put into editing this report.
Contribution of authors
Sophie Beale (project lead) developed the protocol, undertook the economic assessment and co-wrote the report.
Diana Sanderson undertook all of the interviews, carried out the assessment of the literature and co-wrote the report.
Anna Sanniti provided general project support and commented on a draft version of the final report.
Yenal Dundar conducted the literature searches.
Angela Boland commented on draft versions of the final report.
Disclaimers
This report presents independent research funded by the National Institute for Health Research (NIHR). The views and opinions expressed by authors in this publication are those of the authors and do not necessarily reflect those of the NHS, the NIHR, NETSCC, the HTA programme or the Department of Health. If there are verbatim quotations included in this publication the views and opinions expressed by the interviewees are those of the interviewees and do not necessarily reflect those of the authors, those of the NHS, the NIHR, NETSCC, the HTA programme or the Department of Health.
References
- Kroese M, Zimmern RL, Sanderson S. Genetic tests and their evaluation: can we answer the key questions?. Genet Med 2004;6:475-80. http://dx.doi.org/10.1097/01.GIM.0000144060.84960.36.
- UK Genetic Testing Network . NHS Directory of Genetic Testing 2012 n.d. http://ukgtn.nhs.uk/fileadmin/_migrated/tt_news/news_files/NHSDirectoryforGeneticTestingV9.pdf (accessed 2 November 2012).
- UK Genetic Testing Network . Supporting Genetic Testing in the NHS: Second Report of the UKGTN 2010 n.d. www.ukgtn.nhs.uk/gtn/digitalAssets/0/929_SECONDREPORT.pdf (accessed 2 November 2012).
- Burton H. Evaluation of the Use of Array Comparative Genomic Hybridisation in the Diagnosis of Learning Disability. Cambridge: Public Health Genetics; 2006.
- Regier D, Friedman J, Marra C. Value for money? Array genomic hybridization for diagnostic testing for genetic causes of intellectual disability. Am J Med Genet 2010;86:765-72. http://dx.doi.org/10.1016/j.ajhg.2010.03.009.
- Department of Health . Valuing People: A New Strategy for Learning Disability for the 21st Century. A White Paper 2001. www.dh.gov.uk/en/Publicationsandstatistics/Publications/PublicationsPolicyAndGuidance/DH_4009153 (accessed 24 October 2012).
- The ICD-10 Classification of Mental and Behavioural Disorders: Clincial Descriptions and Diagnostic Guidelines. Geneva: WHO; 1992.
- ICD-10 Guide for Mental Retardation. Geneva: WHO; 1996.
- Mir G, Nocon A, Amad W, Jones L. Learning Difficulties and Ethnicity. London: Department of Health; 2001.
- Newman WG, Hamilton S, Ayres J, Sanghera N, Smith A, Gaunt L, et al. Array comparative genomic hybridization for diagnosis of developmental delay: an exploratory cost–consequences analysis. Clin Genet 2007;71:254-9. http://dx.doi.org/10.1111/j.1399-0004.2007.00756.x.
- Beaudet A. The utility of chromosomal microarray analysis in developmental and behavioral pediatrics. Child Dev 2013;84:121-32. http://dx.doi.org/10.1111/cdev.12050.
- Mooney A, Owen C, Statham J. Disabled Children: Numbers, Characteristics and Local Service Provision 2008. www.education.gov.uk/publications/eOrderingDownload/DCSF-RR042.pdf (accessed 9 October 2012).
- Read J, Spencer N, Blackburn C. Can We Count Them? Disabled Children and their Households. Swindon: Economic and Social Research Council; 2007.
- Michael J. Healthcare for All: Report of the Independent Inquiry into Access to Healthcare for People With Learning Disabilities 2008. webarchive.nationalarchives.gov.uk/20130107105354/http:/www.dh.gov.uk/prod_consum_dh/groups/dh_digitalassets/@dh/@en/documents/digitalasset/dh_106126.pdf (accessed 2 April 2014).
- Emerson E, Hatton C. Estimating the Current Need Demand for Supports for People With Learning Disabilities in England 2004. www.improvinghealthandlives.org.uk/uploads/doc/vid_7008_Estimating_Current_Need_Emerson_and_Hatton_2004.pdf (accessed 24 October 2012).
- Office for National Statistics . Subnational Population Projections, Interim 2011-Based 2012. www.ons.gov.uk/ons/publications/re-reference-tables.html?edition = tcm%3A77–274527 (accessed 25 October 2012).
- Parents’ Guide to the Investigation of Children with Developmental Delay. Cambridge: Cambridge University Hospitals NHS Foundation Trust; 2014.
- Temple I, Westwood G. Do Once and Share. 2006.
- Curry C, Stevenson R, Aughton D, Byrne J, Carey J, Cassidy S, et al. Evaluation of mental retardation: recommendations of a consensus conference. Am J Med Genet 1997;72:468-77. http://dx.doi.org/10.1002/(SICI)1096-8628(19971112)72:4<468::AID-AJMG18>3.0.CO;2-P.
- Gogarty B. Parents as Partners. A Report and Guidelines on the Investigation of Children with Developmental Delay; By Parents, for Professionals. Cambridge: Cambridge Genetics Knowledge Park; 2006.
- University of Cambridge, Cambridge Institute for Medical Research . Welcome to the GOLD Study – Finding the Genetic Basis of Learning Disability 2014. http://goldstudy.cimr.cam.ac.uk (accessed 27 March 2014).
- Wellcome Trust Sanger Institute . Deciphering Developmental Disorders 2014. www.sanger.ac.uk/research/areas/humangenetics/ddd/ (accessed 27 March 2014).
- National Institute for Health Research . Specialist Pathology Evaluating Exomes in Diagnostics (SPEED) 2014. http://bioresource.nihr.ac.uk/rare-diseases/study-specialist-pathology-evaluating-exomes-in-diagnostics/ (accessed 27 March 2014).
- Lynch SA. What price a diagnosis?. Dev Med Child Neurol 2011;53. http://dx.doi.org/10.1111/j.1469-8749.2011.04085.x.
- Wordsworth S, Buchanan J, Regan R, Davison V, Smith K, Dyer S, et al. Diagnosing idiopathic learning disability: a cost-effectiveness analysis of microarray technology in the National Health Service of the United Kingdom. Genomic Med 2007;1:35-4. http://dx.doi.org/10.1007/s11568-007-9005-6.
- van Karnebeek C, Jansweijer M, Leenders A, Offringa M, Hennekam C. Diagnostic investigations in individuals with mental retardation: a systematic literature review of their usefulness. Eur J Hum Genet 2005;13:6-25. http://dx.doi.org/10.1038/sj.ejhg.5201279.
- Mefford H, Batshaw M, Hoffman E. Genomics, intellectual disability and autism. N Engl J Med 2012;366:733-43. http://dx.doi.org/10.1056/NEJMra1114194.
- Wright C, Burton H, Hall A, Moorthie S, Pokorska-Bocci A, Sagoo G, et al. Next Steps in the Sequence: The Implications of Whole Genome Sequencing for Health in the UK. Cambridge: PHG Foundation; 2011.
- Flinter F. Should we sequence everyone’s genome? No. BMJ 2013;346. http://dx.doi.org/10.1136/bmj.f3132.
- Mamanova L, Coffey A, Scott C, Kozarewa I, Turner E, Kumar A, et al. Target-enrichment strategies for next-generation sequencing. Nat Methods 2010;7:111-19. http://dx.doi.org/10.1038/nmeth.1419.
- Heger M. Dutch Study Aims to Demonstrate Cost-Effectiveness of Reimbursing for Exome Sequencing Dx 2011. www.genomeweb.com/print/981951 (accessed 3 April 2014).
- Genomic Medicine. Volume 1: Report. London: The Stationery Office; 2009.
- Wright C, Middleton A, Burton H, Cunningham F, Humphries S, Hurst J, et al. Policy challenges of clinical genome sequencing. BMJ 2013;347. http://dx.doi.org/10.1136/bmj.f6845.
- NGS Survey 2013. Begbroke: Oxford Gene Technology; 2013.
- Vissers L, de Vries B, Veltman J. Genomic microarrays in mental retardation: from copy number variation to gene, from research to diagnosis. J Med Genet 2010;47:289-97. http://dx.doi.org/10.1136/jmg.2009.072942.
- Hayes JL, Tzika A, Thygesen H, Berri S, Wood HM, Hewitt S, et al. Diagnosis of copy number variation by Illumina next generation sequencing is comparable in performance to oligonucleotide array comparative genomic hybridisation. Genomics 2013;102:174-81. http://dx.doi.org/10.1016/j.ygeno.2013.04.006.
- Jacob H, Abrams K, Bick D, Brodie K, Dimmock D, Farrell M, et al. Genomics in clinical practice: lessons from the front lines. Sci Transl Med 2013;5. http://dx.doi.org/10.1126/scitranslmed.3006468.
- de Ligt J, Willemsen M, van Bon B, Kleefstra T, Yntema H, Kroes T, et al. Diagnostic exome sequencing in persons with severe intellectual disability. N Engl J Med 2012;367:1921-9. http://dx.doi.org/10.1056/NEJMoa1206524.
- Mefford H. Diagnostic exome sequencing – are we there yet?. N Engl J Med 2012;367:1951-3. http://dx.doi.org/10.1056/NEJMe1211659.
- Hurd P, Nelson C. Advantages of next-generation sequencing versus the microarray in epigenetic research. Brief Funct Genomic Proteomic 2009;8:174-83. http://dx.doi.org/10.1093/bfgp/elp013.
- Luheshi L, Raza S. Clinical Whole Gene Analysis: Delivering the Right Diagnosis. Cambridge: PHG Foundation; 2014.
- Rigter T, Henneman L, Kristoffersson U, Hall A, Yntema H, Borry P, et al. Reflecting on earlier experiences with unsolicited findings: points to consider for next-generation sequencing and informed consent in diagnostics. Hum Mutat 2013;34:1322-8. http://dx.doi.org/10.1002/humu.22370.
- Rigter T, van Aart C, Elting M, Waisfisz Q, Cornel M, Henneman L. Informed consent for exome sequencing in diagnostics: exploring first experiences and views of professionals and patients. Clin Genet 2014;85:417-22. http://dx.doi.org/10.1111/cge.12299.
- Hastings R, de Wert G, Fowler B, Krawczak M, Vermeulen E, Bakker E, et al. The changing landscape of genetic testing and its impact on clinical and laboratory services and research in Europe. Eur J Hum Genet 2012;20:911-16. http://dx.doi.org/10.1038/ejhg.2012.56.
- American College of Medical Genetics and Genomics . Points to consider for informed consent for genome/exome sequencing. Genet Med 2013;15:748-9. http://dx.doi.org/10.1038/gim.2013.94.
- Hall A, Hallowell N, Zinnern R. Managing Incidental and Pertinent Findings from WGS in the 100,000 Genomes Project: A Discussion Paper from the PHG Foundation. Cambridge: PHG Foundation; 2013.
- Kaye J, Hurles M, Griffin H, Grewal J, Bobrow M, Timpson N, et al. Managing clinically significant findings in research: the UK10K example. Eur J Hum Genet 2014;22:1100-4. http://dx.doi.org/10.1038/ejhg.2013.290.
- van El CG, Cornel MC, Borry P, Hastings RJ, Fellmann F, Hodgson SV, et al. Whole-genome sequencing in health care: recommendations of the European Society of Human Genetics. Eur J Hum Genet 2013;21:580-4. http://dx.doi.org/10.1038/ejhg.2013.46.
- American College of Medical Genetics and Genomics . ACMG recommendations for reporting of incidental findings in clinical exome and genome sequencing. Genet Med 2013;15:565-74. http://dx.doi.org/10.1038/gim.2013.73.
- Burton H, Zimmern R. ACMG Recommendations on Incidental Findings: A Commentary. Cambridge: PHG Foundation; 2013.
- Human Genomics Strategy Group . Building on Our Inheritance: Genomic Technology in Healthcare 2012. www.gov.uk/government/uploads/system/uploads/attachment_data/file/213705/dh_132382.pdf (accessed 28 March 2014).
- Miller DT, Adam MP, Aradhya S, Biesecker LG, Brothman AR, Carter NP, et al. Consensus statement: chromosomal microarray is a first-tier clinical diagnostic test for individuals with developmental disabilities or congenital anomalies. Am J Hum Genet 2010;86:749-64. http://dx.doi.org/10.1016/j.ajhg.2010.04.006.
- Trakadis Y, Shevell M. Microarray as a first genetic test in global developmental delay: a cost-effectiveness analysis. Dev Med Child Neurol 2011;53:994-9. http://dx.doi.org/10.1111/j.1469-8749.2011.04080.x.
- Towne C, Beggs A, Agrawal P. Efficiency of Whole Exome Genome Sequencing for Achieving a Diagnosis in Rare Presentations 2013. www.ashg.org/2013meeting/abstracts/fulltext/f130122905.htm.
- Addenbrooke’s Hospital Genetics Laboratories . Services and Tests 2014. www.cuh.org.uk/addenbrookes/services/clinical/genetics/genetics_labs/services_test/services_test_index.html (accessed 19 February 2014).
- Sheffield Children’s NHS Foundation Trust . Clinical Chemistry and Sheffield Diagnostic Genetics Service. User’s Handbook for Metabolic Investigations 2013. www.sheffieldchildrens.nhs.uk/downloads/metabolicbiochemistry/MetabolicHandbook.pdf (accessed 20 March 2014).
- NHS Reference Costs 2012 to 2013. London: Department of Health; 2013.
- UK Genetic Testing Network . Genetic Testing for Neurological Conditions 2013. http://ukgtn.nhs.uk/fileadmin/uploads/ukgtn/Documents/Resources/Library/Reports_Guidelines/UKGTN%20Genetic%20Testing%20for%20Neurological%20Conditions%202013.pdf (accessed 2 April 2014).
- Wilson B, Torrance N, Mollison J, Wordsworth S, Gray J, Haiter N, et al. Improving the referral process for familial breast cancer genetic counselling: findings of three randomised controlled trials of two interventions. Health Technol Assess 2005;9. http://dx.doi.org/10.3310/hta9030.
- Curtis L. Unit Costs of Health and Social Care 2013. Canterbury: PSSRU, University of Kent; 2013.
- Leeflang M, Deeks J, Takwoingi Y, Macaskill P. Cochrane diagnostic test accuracy reviews. Syst Rev 2013;2. http://dx.doi.org/10.1186/2046-4053-2-82.
- Drummond M, Griffin A, Tarricone R. Economic evaluation for devices and drugs – same or different. Value Health 2009;12:402-4. http://dx.doi.org/10.1111/j.1524-4733.2008.00476_1.x.
- Wetterstrand K. DNA Sequencing Costs: Data from the NHGRI Genome Sequencing Program (GSP). National Human Genome Research Institute; 2014.
- McAllister M, Payne K, Nicholls S, MacLeod R, Donnai D, Davies L. Improving service evaluation in clinical genetics: identifying effects of genetic diseases on individuals and families. J Genet Couns 2007;16:71-83. http://dx.doi.org/10.1007/s10897-006-9046-3.
- Guide to the Methods of Technology Appraisals 2013. London: NICE; 2013.
- Grada A, Weinbrecht K. Next-generation sequencing: methodology and application. J Invest Dermatol 2013;133. http://dx.doi.org/10.1038/jid.2013.248 (accessed 3 April 2014).
- Hughes S, Capper R, Lam S, Sparkes N. Sequencing and Microarrays for Genome Analysis: Complementary Rather Than Competing?. Begbroke: Oxford Gene Technology; 2011.
- Knight S, Regan R. Idiopathic learning disability and genome imbalance. Cytogenet Genome Res 2006;115:215-24. http://dx.doi.org/10.1159/000095917.
- Sagoo GS, Butterworth AS, Sanderson S, Shaw-Smith C, Higgins JP, Burton H. Array CGH in patients with learning disability (mental retardation) and congenital anomalies: updated systematic review and meta-analysis of 19 studies and 13,926 subjects. Genet Med 2009;11:139-46. http://dx.doi.org/10.1097/GIM.0b013e318194ee8f.
- Subramonia-Iyer S, Sanderson S, Sagoo G, Higgins J, Burton H, Zimmern R, et al. Array-based comparative genomic hybridization for investigating chromosomal abnormalities in patients with learning disability: systematic review meta-analysis of diagnostic and false-positive yields. Genet Med 2007;9:74-9. http://dx.doi.org/10.1097/GIM.0b013e31803141f2.
- Miller D, Shen Y, Harris D, Wu B-L, Sobeih M. Genetic testing for developmental delay: keep searching for an answer. Clin Chem 2009;55:827-32. http://dx.doi.org/10.1373/clinchem.2008.119438.
- Ahn JW, Bint S, Bergbaum A, Mann K, Hall RP, Ogilvie CM. Array CGH as a first line diagnostic test in place of karyotyping for postnatal referrals – results from four years’ clinical application for over 8,700 patients. Mol Cytogenet 2013;6. http://dx.doi.org/10.1186/1755-8166-6-16.
- Costain G, Chow E, Ray P, Bassett A. Caregiver and adult patient perspectives on the importance of a diagnosis of 22q11.2 deletion syndrome. J Intellect Disabil Res 2012;56:641-51. http://dx.doi.org/10.1111/j.1365-2788.2011.01510.x.
- Rosenthal E, Biesecker L, Biesecker B. Parental attitudes toward a diagnosis in children with unidentified multiple congenital anomaly syndromes. Am J Med Genet 2001;103:106-14. http://dx.doi.org/10.1002/ajmg.1527.
Appendix 1 Research advisory group members
Organisation/expertise | Details |
---|---|
Clinical geneticist | Professor Jill Clayton-Smith is a Clinical Geneticist at Central Manchester University Hospitals NHS Foundation Trust with an extensive background in research relating to genetic disorders in children. Her current research interests involve examining the use of newer genetic technologies, particularly NGS for the diagnosis of developmental disorders and ID |
Genetic counsellor | Dr Laura Boyes is the Principal Genetic Counsellor at Birmingham Women’s NHS Foundation Trust. Laura has a PhD in molecular genetics alongside molecular and psychosocial research experience in genetics. She is also on the committee of the Association of Genetic Nurses and Counsellors |
Bioinformation specialist | Mr Sanjeev Bhaskar is the Lead Bioinformatician at Central Manchester University Hospitals NHS Foundation Trust. He leads the development of NGS analysis pipelines for diagnostics |
Representatives from a testing centre | Dr Simon Ramsden is a Consultant Clinical Scientist at Central Manchester University Hospitals NHS Foundation Trust who has been delivering traditional genetics services for LDs for a number of years |
Mr James O’Sullivan is a Clinical Molecular Geneticist who leads the Central Manchester University Hospitals NHS Foundation Trust NGS team | |
Paediatricians | Dr Megan Thomas is a Consultant Community Paediatrician at Blenheim House Child Development and Family Support Centre. She has a background in genetics and a PhD in a LD topic |
Dr Imelda Hughes is a Consultant Paediatric Neurologist at Central Manchester University Hospitals NHS Foundation Trust | |
GP | Dr Matt Hoghton is the Royal College of General Practitioners Clinical Champion for LDs and a GP in Clevedon, Somerset |
SWAN | Dr Alastair Kent is the Director of Genetic Alliance UK. SWAN is a project run by Genetic Alliance UK offering support and information to families of children with undiagnosed genetic conditions. Alastair is a member of a number of local, national and European genetics-related committees |
BACD | Dr Karen Horridge is a Disability Paediatrician in Sunderland. She is the chairperson of the North of England Collaborative Cerebral Palsy Survey and, from 25 March 2013, will be chairperson of the BACD. She has established regular joint clinics with colleagues from clinical genetics in a number of districts as she recognises the importance of timely genetic diagnosis to inform health-care planning. As well as representing the BACD, Karen will be reporting back to Karen Turner at the Department of Health |
Unique | Dr Sarah Wynn is an Information Officer at Unique. Unique is a source of information and support to families and individuals affected by any rare chromosome disorder and to the professionals who work with them. Sarah is currently preparing a family-friendly guide on NGS. She has hands-on sequencing experience through many years of research work at the University of Hong Kong and for the MRC. She also has experience of talking to families about their children’s diagnostic pathways |
Individual with expertise in ethics and genetics | Professor Anneke Lucassen is a Clinical Geneticist and academic who leads an interdisciplinary programme of research into the social, ethical and legal aspects of developments in genetics. She is cofounder of the UK Genethics Group, which is a national forum for the exploration of ethicolegal issues arising in genetic practice. She sits on the Nuffield Council on Bioethics and is currently on its working party exploring ethical issues in genomics and data linkage |
Appendix 2 Literature search strategies
Ovid MEDLINE(R) including MEDLINE In-Process & Other Non-Indexed Citations (1946 to February Week 1 2014)
-
exp Learning Disorders/ (18,568)
-
exp Intellectual Disability/ (79,515)
-
exp Mentally Disabled Persons/ (2172)
-
(intellect$ adj5 disabl$).tw. (326)
-
(mental$ adj5 retard$).tw. (28,906)
-
(mental$ adj5 handicap$).tw. (3107)
-
(mental$ adj5 disabl$).tw. (874)
-
(learning adj5 disabl$).tw. (1319)
-
(intellect$ adj5 impair$).tw. (1631)
-
(learn$ adj5 disorder$).tw. (2136)
-
(learning adj5 difficult$).tw. (2721)
-
(mental$ adj5 deficien$).tw. (2097)
-
exp Developmental Disabilities/ (14,294)
-
(developmental delay$ or (genom$ adj5 imbalance$) or (pathologic$ adj5 imbalance$)).tw. (8038)
-
exp Sequence Analysis, DNA/ (150,827)
-
sequenc$.tw. (754,127)
-
exp Genetic Testing/ (25,376)
-
((clinical adj5 genome) or (genome adj5 sequenc$)).tw. (33,726)
-
(target$ adj5 panel$).tw. (590)
-
exp Microarray Analysis/ (71,214)
-
microarray$.tw. (62,584)
-
exp Comparative Genomic Hybridization/ (3462)
-
(arrayCGH or array CGH or (genomic adj5 hybridisation$)).tw. (2350)
-
(genetic$ adj5 diagnos$).tw. (11,967)
-
(genetic$ adj5 test$).tw. (18,615)
-
exp Genetic Testing/ (25,376)
-
exp Diagnostic Tests, Routine/ (6704)
-
(diagnostic$ adj5 test$).tw. (39,673)
-
exp “Costs and Cost Analysis”/ or exp Health Care Costs/ or exp Cost-Benefit Analysis/ (176,972)
-
exp Economics/ or exp “Value of Life”/ (485,940)
-
(burden adj5 (disease or illness)).tw. (13,400)
-
(cost$ adj2 (effective$ or utilit$ or benefit$ or minimi$ or evaluat$ or analy$ or study or studies or consequenc$ or compar$ or efficienc$ or estimate$ or variable$ or unit)).ti,ab. (100,135)
-
(cost$ or economic$ or expenditure$ or pharmacoeconomic$ or pharmaco-economic$ or price$ or pricing).tw. (452,633)
-
(resource adj5 (allocation$ or utilit$)).tw. (4370)
-
(value adj5 money).tw. (976)
-
(budget$ or fiscal or funding or financial or finance$).tw. (89,797)
-
exp quality of life/ (112,779)
-
(quality$ adj5 (life or survival)).tw. (134,729)
-
(or/1-14) and (or/15-28) and (or/29-38) (348)
-
limit 39 to (english language and humans and yr=”2005 -Current”) (180)
EMBASE (1996 to 2014 Week 7)
-
exp learning disorder/ (18,356)
-
exp intellectual impairment/ (251,357)
-
(intellect$ adj5 disabl$).tw. (445)
-
(mental$ adj5 retard$).tw. (21,213)
-
(mental$ adj5 handicap$).tw. (845)
-
(mental$ adj5 disabl$).tw. (735)
-
(learning adj5 disabl$).tw. (362)
-
(intellect$ adj5 impair$).tw. (1468)
-
(learn$ adj5 disorder$).tw. (2579)
-
(learning adj5 difficult$).tw. (3359)
-
(mental$ adj5 deficien$).tw. (810)
-
exp developmental disorder/ (22,862)
-
(developmental delay$ or (genom$ adj5 imbalance$) or (pathologic$ adj5 imbalance$)).tw. (10,359)
-
exp sequence analysis/ (99,948)
-
sequenc$.tw. (697,616)
-
exp genetic screening/ (42,442)
-
((clinical adj5 genome) or (genome adj5 sequenc$)).tw. (40,426)
-
(target$ adj5 panel$).tw. (826)
-
exp genetic analysis/ or exp microarray analysis/ or exp DNA microarray/ or exp expressed sequence tag/ (297,968)
-
microarray$.tw. (97,703)
-
exp comparative genomic hybridization/ (12,455)
-
(arrayCGH or array CGH or (genomic adj5 hybridisation$)).tw. (4332)
-
(genetic$ adj5 diagnos$).tw. (15,665)
-
(genetic$ adj5 test$).tw. (25,910)
-
*genetic procedures/ (9476)
-
exp health economics/ (473,488)
-
exp “health care cost”/ or exp “cost-effectiveness analysis”/ or exp “cost of reproduction”/ or exp “drug cost”/ or exp “cost control”/ or exp “cost utility analysis”/ or exp “cost minimization analysis”/ or exp “cost benefit analysis”/ or exp “cost”/ (310,190)
-
(burden adj5 (disease or illness)).tw. (21,366)
-
(cost$ adj2 (effective$ or utilit$ or benefit$ or minimi$ or evaluat$ or analy$ or study or studies or consequenc$ or compar$ or efficienc$ or estimate$ or variable$ or unit)).ti,ab. (128,047)
-
(cost$ or economic$ or expenditure$ or pharmacoeconomic$ or pharmaco-economic$ or price$ or pricing).tw. (518,405)
-
(resource adj5 (allocation$ or utilit$)).tw. (5044)
-
(value adj5 money).tw. (1318)
-
(budget$ or fiscal or funding or financial or finance$).tw. (102,019)
-
*”quality of life”/ (50,147)
-
(quality$ adj5 (life or survival)).tw. (207,899)
-
(or/1-13) and (or/14-25) and (or/26-35) (1177)
-
limit 36 to (human and english language and yr=”2005 -Current”) (732)
The Cochrane Library (17 February 2014)
#1 MeSH descriptor: [Learning Disorders] explode all trees (436)
#2 MeSH descriptor: [Intellectual Disability] explode all trees (964)
#3 learning disabil* (Word variations have been searched) (1281)
#4 mental retard* (Word variations have been searched) (1196)
#5 mental handicap* (Word variations have been searched) (337)
#6 mental disabl* (Word variations have been searched) (740)
#7 learning disabl* (Word variations have been searched) (468)
#8 intellect* impair* (Word variations have been searched) (543)
#9 learning disorder* (Word variations have been searched) (3070)
#10 learning difficult* (Word variations have been searched) (1901)
#11 mental deficien* (Word variations have been searched) (1095)
#12 #1 or #2 or #3 or #4 or #5 or #6 or #7 or #8 or #9 or #10 or #11 (7352)
#13 MeSH descriptor: [Sequence Analysis] explode all trees (597)
#14 sequenc*:ti,ab,kw (Word variations have been searched) (8702)
#15 MeSH descriptor: [Genetic Testing] explode all trees (464)
#16 clinical genome*:ti,ab,kw (Word variations have been searched) (210)
#17 genome sequenc*:ti,ab,kw (Word variations have been searched) (119)
#18 target panel*:ti,ab,kw (Word variations have been searched) (149)
#19 MeSH descriptor: [Microarray Analysis] explode all trees (237)
#20 microarray*:ti,ab,kw (Word variations have been searched) (347)
#21 MeSH descriptor: [Comparative Genomic Hybridization] explode all trees (8)
#22 (genomic hybridisation*) or (genomic hybridization*):ti,ab,kw (Word variations have been searched) (38)
#23 genetic diagnos*:ti,ab,kw (Word variations have been searched) (727)
#24 genetic test*:ti,ab,kw (Word variations have been searched) (2020)
#25 #13 or #14 or #15 or #16 or #17 or #18 or #19 or #20 or #21 or #22 or #23 or #24 (11,502)
#26 #12 and #25 (236)
Total no. of references: 1148
Total no. of references (duplicates removed electronically): 767
Appendix 3 Effectiveness of traditional genetic testing methods
As well as information on the effectiveness of NGS technologies, the literature searches also identified material on the effectiveness of traditional genetic testing methods. This material is summarised in this appendix in the order in which it was published.
The first study of note, that by Van Karnebeek et al. ,26 was a systematic literature review of the usefulness of six major approaches to diagnosing LDs. The authors found the following:
-
cytogenetic studies – mean yield of chromosome aberrations 9.5% (variation: 5.4% in school populations to 13.3% in institute populations; 4.1% in borderline to mild LD to 13.3% in moderate to profound LD; more frequent structural anomalies in females)
-
subtelomeric studies (FISH) – median yield 4.4% (also showing a female predominance)
-
-
fragile X screening – cytogenetic studies 5.4% and molecular studies 2.0%, with a higher yield in moderate to profound LD; using phenotype checklists sometimes increased the yield
-
dysmorphological examination – very high yield (variation 39–81%) but very limited number of relevant studies.
Early research reviewed by Knight and Regan68 suggested that advances in genetic technology (such as multi-telomere FISH and aCGH) provided a 15–20% improvement in diagnostic capability (i.e. provided 15–20% more clinical diagnoses). Sagoo et al. 69 published a systematic review and meta-analysis of the use of aCGH for patients with LD and congenital anomalies. This was an update of a previous meta-analysis by Subramonia-Iyer et al. 70 evaluating the diagnostic and false-positive yields of this technology. 70 This previous meta-analysis had concluded that there was insufficient evidence to recommend introducing aCGH as a routine addition to current cytogenetic analysis, although it could be helpful in certain circumstances. In the updated 2009 study,69 the overall diagnostic yield of causal abnormalities was found to be 10% (95% CI 8% to 12%), the overall number needed to test to identify an extra causal anomaly was 10 (95% CI 8 to 13) and the overall false-positive yield of non-causal abnormalities was 7% (95% CI 5% to 10%). The authors concluded that their study provides new evidence to support the use of aCGH in investigating patients with LD and congenital anomalies in whom conventional cytogenetic tests have been negative (i.e. as well as, not instead of, karyotyping). However, given that the technology was also found to identify genetic anomalies deemed to be non-causal or of unknown relevance (i.e. false positives) at a similar frequency to that for causal variants, caution regarding use in clinical practice is advised. The authors suggested that future studies should concentrate on trying to minimise the number of false positives being identified. They also stated that further evaluation through large prospective studies was needed before aCGH could be recommended as a first-line investigation for all patients with LD, in particular when the LD may be less severe and there is less likelihood of a genetic cause.
Miller et al. 71 stated that using microarray-based CGH to detect changes in genomic copy number, more than any other single test, had dramatically increased the rate of diagnosis among individuals with unexplained DD and MR. G-banded karyotypes have typically identified abnormalities in 3–4% of individuals with idiopathic MR. Subtelomere FISH (ST-FISH) for submicroscopic deletions and duplications added to the diagnostic yield. In the largest study of ST-FISH, presumed pathogenic changes were found in 2.6% of 11,688 unselected cases with a previously normal karyotype. The authors considered that multiple studies now supported the conclusion that aCGH has a higher diagnostic yield than G-banded karyotyping and ST-FISH in the evaluation of patients with DD and MR, detecting abnormalities in up to 8% of patients with a previously normal karyotype using arrays targeted to clinically relevant areas of the genome. They considered that this detection rate would increase as more laboratories implemented arrays with whole-genome coverage and that no other clinical laboratory test at that time had a comparable clinical sensitivity for patients with a diagnosis of DD and MR.
Microarray-based genomic copy number analysis is offered to this patient population under various names, such as CMA and molecular karyotyping. Miller et al. 71 use the term ‘CMA’ to encompass all types of array-based genomic copy number analysis, including aCGH and SNP arrays. Miller et al. 52 provided an international perspective on diagnostic yield and produced a consensus statement explaining that CMA is a first-tier clinical diagnostic test for individuals with DD or congenital anomalies. Their review of 33 studies (including 21,698 patients) compared CMA with G-banded karyotyping with respect to technical advantages and limitations, diagnostic yield for various types of chromosomal aberrations and issues that affect test interpretation. CMA was found to offer a much higher diagnostic yield (15–20%) for genetic testing of individuals with unexplained DD/ID, ASD or MCA than G-banded karyotyping (about 3%, excluding Down syndrome and other recognisable chromosomal syndromes). This was primarily because of CMA’s higher sensitivity in relation to detecting submicroscopic deletions and duplications. Although truly balanced rearrangements and low-level mosaicism are generally not detected by arrays, these are relatively infrequent causes of abnormal phenotypes in this population (< 1%). The authors concluded that available evidence strongly supports the use of CMA rather than G-banded karyotyping as a first-line diagnostic test for patients with DD/ID, ASD or MCA. Furthermore, they stated that G-banded karyotype analysis should be utilised only for patients with obvious chromosomal syndromes, a family history of chromosomal rearrangement or a history of multiple miscarriage. 52
More recently, Beaudet11 considered the utility of CMA in developmental and behavioural paediatrics. Summarising existing papers he stated that reviews have found that karyotype analysis alone identifies underlying abnormalities in 3–5% of children with moderate to severe ID excluding Down syndrome. Combining karyotype with selected FISH increases the level of detection to approximately 8–10%. Arrays with the highest resolution for coverage are thought to detect abnormalities in 15–20% of children with more severe disabilities. However, Beaudet11 recognised that, although most studies report a 20–25% detection rate of causative CNVs in children with moderate or severe ID, there will also be a significant number of children with novel CNVs of uncertain significance and thus it is not always possible to determine whether a CNV is disease causing or not. In addition, disease-causing CNVs are found in 5–10% of cases of autism, being more frequent in severe phenotypes. Beaudet11 stated, however, that it is not possible to determine what percentage of children with particular phenotypes or developmental disabilities will have causative genetic abnormalities detected by array analysis. He explains that this variability is partly dependent on the phenotypes of the individuals in any sample, as these phenotypes play a major role in determining the rate of detection of causative abnormalities. He states that the more severe the disabilities, and the more that these are accompanied by birth defects, epilepsy and dysmorphology, the higher the likelihood of finding a causative abnormality (as stated by Miller et al. 52). However, Beaudet11 recognised that, although most studies report a 20–25% detection rate of causative CNVs in children with moderate or severe ID, there will also be a significant number of children with novel CNVs of uncertain significance and thus it is not always possible to determine whether a CNV is disease causing or not. In addition, Beaudet11 considers that parental studies are often helpful for interpreting the potential causative significance of a rare CNV (as well as being valuable for genetic counselling). As outlined by Miller et al. ,52 Beaudet stated that G-banded karyotype analysis is generally not indicated as an additional test for a child with an ID phenotype if array analysis is normal, unless there is clinical evidence of an obvious chromosomal disorder such as Down syndrome or a family history of chromosomal rearrangement. Beaudet11 concluded that CMA has replaced G-banded karyotype analysis as the first-tier test for genetic evaluation of children with development and behavioural disabilities.
Taking a practical focus, Ahn et al. 72 reported on a study at Guy’s and St. Thomas’ NHS Foundation Trust in London using oligonucleotide aCGH as a first-line diagnostic test in place of karyotyping for postnatal referrals (ranging from neonatal congenital anomalies to adult neurodisabilities). Two-thirds of the patients tested with aCGH (8794/13,412) had this as their first-line test. The authors found that 25% of these patients had CNVs either in known pathogenic regions or in other regions where imbalances have not been reported in the normal population. Of these CNVs, 46% were deletions or nullisomy, 53% were duplications or triplications and the remainder were mosaic imbalances. In total, 87% were < 5 million base pairs and would likely not be detected by G-banded chromosome analysis. For cases with completed inheritance studies (about 50% of the patients with imbalances), one-fifth of the imbalances were de novo.
With regard to the potential limitations of aCGH, Ahn et al. 72 point out that, despite the increase in resolution and the higher diagnostic yield associated with aCGH testing, there may be concern that, without visualisation of chromosomes by traditional cytogenetic techniques, balanced rearrangement will not be detected. These rearrangements may disrupt gene function without causing any loss of coding material and hence may be important diagnostically. However, they believe that patient benefit associated with the increased diagnostic yield of aCGH outweighs the very small number of cases in which a balanced rearrangement may lead to a disruption in an important gene. The authors also considered that, given cost restraints, the additional benefit of undertaking traditional banded chromosome analysis as well as aCGH testing is negligible. They conclude that aCGH is a robust and cost-effective alternative to traditional cytogenetic methodology and that experience in reporting and interpreting aCGH results will inform the implementation of NGS technologies into clinical diagnostic services.
Appendix 4 List of interviewees and the interview protocol
List of interviewees
Name of interviewee | Role(s) |
---|---|
Professor Gillian Baird OBE | Consultant Paediatrician, Guy’s and St Thomas’ NHS Foundation Trust and King’s Health Partners; past chairperson of the BACD |
Dr Mark Bale | Deputy Director, Health Science & Bioethics Division, Department of Health |
Mr Sanjeev Bhaskar | Group Lead Clinical Bioinformatician, Central Manchester University Hospitals NHS Foundation Trust |
Dr Laura Boyes | Lead Consultant Genetic Counsellor, Birmingham Women’s NHS Foundation Trust; chairperson of Association of Genetic Nurses and Counsellors |
Professor Han Brunner | Professor of Medical Genetics, Radboud University Nijmegen Medical Center, the Netherlands |
Professor Jill Clayton-Smith | Consultant Clinical Geneticist, Central Manchester University Hospitals NHS Foundation Trust, and Honorary Professor in Medical Genetics |
Ms Kate Dack | Public Programmes Manager, Nowgen, Manchester |
Dr Angela Davies | Professional Training Manager, Nowgen, Manchester, and Co-Director MSc Clinical Bioinformatics, University of Manchester; work with the National School of Healthcare Science and Department of Health to develop the Clinical Bioinformatics STP curriculum |
Dr Helen Firth | Consultant Clinical Geneticist, Cambridge University Hospitals NHS Foundation Trust; Clinical Lead on the DDD study |
Professor Frances Flinter | Consultant in Clinical Genetics and Caldicott Guardian, Guy’s and St Thomas’ NHS Foundation Trust; Professor of Clinical Genetics, King’s College, London; chairperson of the Medical Genetics Clinical Reference Group, NHS England |
Dr Maria Hall | Specialist Registrar Community Paediatrics/Neurodisability, Blackpool, Fylde and Wyre Hospitals NHS Foundation Trust |
Dr Tammy Hedderley | Consultant Children’s Neurologist, Guy’s and St Thomas’ NHS Foundation Trust |
Dr Matthew Hoghton | General Practitioner, Clevedon Riverside Group, Somerset; Visiting Fellow in Social and Community Medicine, Bristol University; Clinical Expert in Learning Disabilities, Royal College of General Practitioners |
Dr Karen Horridge | Disability Paediatrician, City Hospitals Sunderland NHS Foundation Trust; chairperson of the BACD |
Dr Imelda Hughes | Consultant Paediatric Neurologist, Central Manchester University Hospitals NHS Foundation Trust |
Dr Alastair Kent OBE | Director, Genetic Alliance UK (incorporating SWAN); chairperson of Rare Disease UK and of the UK Rare Disease Forum (a Ministerial Advisory Committee set up to monitor the implementation of the UK Strategy for Rare Diseases) |
Dr Bronwyn Kerr | Consultant Clinical Geneticist and Honorary Senior Lecturer, Central Manchester University Hospitals NHS Foundation Trust; chairperson, Joint Committee on Genomics in Medicine |
Dr Nigel Laycock | Consultant Paediatrician, Blackpool, Fylde and Wyre Hospitals NHS Foundation Trust |
Professor Anneke Lucassen | Professor and Honorary Consultant in Clinical Genetics, University of Southampton and Wessex Clinical Genetics Service; Council Member of Nuffield Council on Bioethics; Co-founder/Organiser of UK Genethics Club; Ethics Advisory Group Member, Genomics England |
Dr Kay Metcalfe | Consultant Clinical Geneticist, Central Manchester University Hospitals NHS Foundation Trust |
Dr Helen Middleton-Price | Director, Nowgen, Manchester; Consultant Clinical Scientist and Visiting Fellow, University of Manchester |
Dr Peter Mills | Assistant Director, Nuffield Council on Bioethics |
Professor William Newman | Professor of Translational Genomic Medicine, University of Manchester |
Dr Keri Oliver | Genetic Counsellor, Nottingham University Hospitals NHS Trust |
Mr James O’Sullivan | Ophthalmic Geneticist and Clinical Molecular Geneticist, University of Manchester and Central Manchester University Hospitals NHS Foundation Trust |
Mr Colin Pavelin | Head of Genomics and Rare Diseases, Department of Health (and currently on secondment to Health Education England as Head of the Genomics and Rare Diseases Programme) |
Dr Simon Ramsden | Consultant Clinical Geneticist, Central Manchester University Hospitals NHS Foundation Trust; Member of the UKGTN Laboratory Membership and Audit Working Group |
Dr Lucy Raymond | Leader in Neurogenetics, University of Cambridge, and Consultant Clinical Geneticist; Lead on the GOLD study |
Dr Richard Scott | Consultant in Clinical Genetics, Great Ormond Street Hospital for Children NHS Foundation Trust; Honorary Senior Lecturer, Genetics and Genomic Medicine, University College London Institute of Child Health; Clinical Advisor to UK NEQAS (UK National External Quality Assessment Service) Molecular Genetics Specialist Advisory Group |
Professor Karen Temple | Professor of Medical Genetics, University of Southampton, and Honorary Consultant in Clinical Genetics; UK GTN Steering Committee |
Dr Megan Thomas | Consultant Community Paediatrician, Blackpool, Fylde and Wyre Hospitals NHS Foundation Trust, and Director of Research and Development; North West Regional Representative for the BACD |
Dr Caroline Wright | Senior Scientific Manager, Wellcome Trust Sanger Institute, Cambridge; Project Manager for the DDD study |
Dr Sarah Wynn | Information Officer, Unique; Lay Member of the Clinical Reference Group for Medical Genetics (and sits on several other Advisory Boards) |
Interview protocol
The cost-effectiveness of next-generation sequencing compared with traditional genetic testing for the diagnosis of learning disabilities in children
Information sheet and topic guide for stakeholder interviewees
Who are we?
We are a team of researchers from the Liverpool Reviews and Implementation Group (LRiG) at the University of Liverpool. We have been commissioned by the NIHR to undertake a scoping study to explore the cost-effectiveness of NGS compared with traditional sequential genetic testing for the diagnosis of learning disabilities in children. Although we intend to focus primarily on children of preschool age, we would also welcome any views you might have relating to diagnosing children and young people up to the age of 18 years. The study is due for completion in the winter of 2013.
Background to the study
Conventional genetic testing for learning disabilities has focused on sequential testing, which is often based on individual clinical characteristics. These include karyotyping, aCGH and single gene tests. Such testing can be a time-consuming process and in many cases does not provide a diagnosis. The development of NGS technologies enables sequencing to be extended to large panels of genes, providing a method for simultaneous analysis that reduces time to diagnosis and may increase diagnostic yield. These panels are made up of genes that are known to be related to specific clinical presentations. This is known as targeted exome sequencing. Analysis using NGS can also be undertaken of the whole exome/genome if desired.
Next-generation sequencing technology is developing rapidly and its use may offer advantages when diagnosing learning disabilities in children. However, although panels of genes targeted at specific clinical presentations are currently being developed for this patient group, with access to some available in the NHS, their use is not widespread. NGS technologies for whole genome and exome sequencing are currently only available for research purposes. This study explores the desirability and feasibility of employing NGS routinely (in particular in relation to looking at panels of genes) in clinical practice by the NHS, for young patients suspected of having learning disabilities. The study will also look at associated requirements for additional resources and the accompanying cost implications.
Study objectives
-
Describe current pathways for this patient group that involve the use of genetic testing.
-
Collect stakeholder views on the changes in service provision that would need to be put in place before NGS could be used in clinical practice.
-
Describe the new systems and safeguards that would need to be put in place before NGS could be used in clinical practice.
-
Explore the cost-effectiveness of NGS compared with conventional genetic testing.
Study format
The study is drawing on qualitative and quantitative material from several sources, including interviews with stakeholders from a variety of relevant backgrounds. Some of the invited interviewees have agreed to be members of our RAG; others have been suggested by the RAG members or by other people with whom we’ve already spoken. You have been recommended because of your knowledge and experience as (select as relevant):
-
a paediatrician/community paediatrician
-
a clinical geneticist
-
a genetic counsellor
-
a bioinformatician
-
a staff member from a NHS genetic testing centre
-
a representative of a voluntary or similar organisation.
Your contribution
We recognise that genetic testing is a rapidly changing and developing area. We also appreciate that many potential costs and benefits associated with various aspects of diagnosing learning disabilities in children are very difficult to quantify. Nevertheless, we need to identify and document as many of the potential tangible and intangible costs and benefits as possible. To assist us with this, we would like to hold a semistructured telephone interview with you to discuss your thoughts and views. We anticipate that the interview will last for about 20–30 minutes.
We would like to start the interview by (briefly) exploring your understanding, knowledge and experience of NGS as we recognise that this will vary considerably across the interviewees. During the remainder of the interview we can discuss anything that you think is relevant to our research. However, we are particularly interested in discussing the potential resource implications (e.g. workforce requirements; training requirements) for your organisation and/or profession of a move towards greater use of NGS when diagnosing learning disabilities in children. To this end, we would like to include the following broad areas during the interview (include relevant text).
Paediatrician/community paediatrician/paediatric neurologist
-
(If relevant) How does the work of a paediatric neurologist differ from that of a paediatrician/community paediatrician (especially with reference to the patient group)?
-
Who or what influences the decisions to use traditional methods or other approaches when seeking diagnoses in children who may have learning disabilities?
-
What are their strengths and weaknesses?
-
What are their resource implications?
-
Who bears the associated costs?
-
How closely do you work with clinical geneticists after referring a child for genetic testing?
-
Have you personally used NGS for targeted exome sequencing in this patient group?
-
If so, what did you like/dislike about it? How was it funded?
-
If not, would you like to be able to use NGS?
-
-
Do you think that NGS will be acceptable to this patient group?
-
How do you see the use of NGS by the NHS changing over the next year and over the next 5 years?
-
How do you think this will impact on your work, your department and your clients (e.g. with regard to extra resources and training)?
Clinical geneticist
-
Who or what influences the decisions to use traditional methods or other approaches when seeking diagnoses in children who may have learning disabilities?
-
What are their strengths and weaknesses?
-
What are their resource implications?
-
Who bears the associated costs?
-
How closely do you work with the referring paediatricians (from your own and other hospitals)?
-
Have you personally used NGS for targeted exome sequencing in this patient group?
-
If so, what did you like/dislike about it? How was it funded?
-
If not, would you like to be able to use NGS?
-
-
Do you think that NGS will be acceptable to this patient group?
-
How do you see the use of NGS by the NHS changing over the next year and over the next 5 years?
-
How do you think this will impact on your work, your department and your clients (e.g. with regard to extra resources and training)?
Genetic counsellor
-
During the interview we wish to explore:
-
the role and day-to-day work of a genetic counsellor
-
the types and places of employment of genetic counsellors
-
their required qualifications and training (and the time required for these)
-
an estimate of a ‘typical’ caseload for a genetic counsellor (specialist and/or generic)
-
your views (and/or those of your colleagues) on the acceptability of NGS to patients
-
the potential implications for genetic counsellors of an increase in the use of NGS for targeted exome sequencing when seeking a diagnosis for children who may have learning disabilities
-
how this might impact on the required numbers of genetic counsellors – in the next year and in the next 5 years
-
are there other resource-related implications (e.g. for the NHS)?
-
what other (broad) changes and developments will need to occur to enable NGS to be used more widely for the diagnosis of learning disabilities in children?
-
Bioinformatician
-
During the interview we wish to explore:
-
the role and day-to-day work of bioinformaticians
-
the types and places of employment of bioinformaticians
-
their required qualifications and training (and the time required for these)
-
typical arrangements for supervision and audit of their work
-
the potential implications for bioinformaticians of an increase in the use of NGS when seeking a diagnosis for children who may have learning disabilities
-
how this might impact on the required numbers of bioinformaticians – in the next year and in the next 5 years
-
are there other resource-related implications (e.g. for the NHS)?
-
what other (broad) changes and developments will need to occur (in the NHS and/or in the private sector) to enable NGS to be used more widely for the diagnosis of learning disabilities in children?
-
Staff member from a NHS genetic testing centre
-
During the interview we wish to explore:
-
the current and potential roles of NHS genetic testing centres in the diagnosis of learning disabilities in children
-
the likely involvement of NHS genetic testing centres in the use of NGS in this patient group over the next year and the next 5 years
-
what developments would be needed at NHS genetic testing centres to implement any changes?
-
what would be their likely resource implications?
-
Representative of a voluntary organisation or other body
-
During the interview we wish to explore:
-
the importance for families of having a diagnosis for a child with learning disabilities
-
how might having (or not having) a diagnosis for such a child affect decisions about their health care, social care and education?
-
how might other family members be affected by having (or not having) a diagnosis for such a child?
-
do you think that NGS will be acceptable to this patient group?
-
how might potential developments in genetic testing (over the next year and over the next 5 years) affect the lives of the children and/or their families (positively and/or negatively)?
-
what are the potential resource implications of such developments (for your organisation and for other voluntary organisations and support groups)?
-
If we have not already covered these, we would like to conclude your interview with a brief discussion of what you think are the main strengths and weaknesses of traditional approaches and NGS, including their implications for the patient group. Finally, if you know of any colleagues with expertise or experience that will inform this project then please provide us with their contact details.
Potential benefits of the study
We anticipate that all the professional groups contributing to the scoping study will benefit from a greater knowledge and understanding of the strengths and weaknesses of NGS compared with traditional approaches for the diagnosis of learning disabilities in children. The study findings will be published as a report by the NIHR and will be available from their website. In addition, everyone participating in the face-to-face and telephone interviews will be invited to a dissemination event. This is likely to be held in Liverpool (at the University) once the project report has been finalised (i.e. early 2014).
For further information please contact: xxx (xxx@liverpool.ac.uk or 0151 xxx xxxx).
Appendix 5 Additional interview quotations: changes in service provision
This appendix provides an evidence base for material in Chapter 3 that relates to changes in service provision. It reports verbatim some of the views expressed by the interviewees about various topics. Because of their different experiences and areas of expertise, not all of the interviewees had views on all of the topics. However, the quotes show where views were broadly similar irrespective of profession and where opinions differed across the professions.
Data issues
Data capture by the laboratories
There did not appear to be a strong consensus among participants about the most appropriate machine/platform to use to test for specific conditions. Indeed, different platforms may be required for the different conditions associated with LD or possibly for research and diagnostic use:
The technology is out there, but if I’m honest, it’s still a bit embryonic. There are a lot of different technologies, so we haven’t yet arrived at a single platform, so there are a lot of other technology validation and technology assessments going on to work out whether there is a single platform that is best suited to our needs. It will change a lot over the next few years; there is no doubt about it. We are currently in what we call ‘second generation sequencing’ platforms. I don’t think it will end in second generation platforms; we will have third generation platforms.
Genetic specialist, NHS
Although it was generally considered that the data capture process was relatively straightforward, interviewees stressed the importance of it being carried out properly:
There is an ever-increasing discrepancy between the research domain and the NHS. . . . There are a very large number of genes that have been identified that cause LD and we should be [testing for them] now. What we need is money; we need an infrastructure of machines to do it. We need information technology to store data and we need human beings to analyse the data. But we also need to do it in a cost-efficient way, and be mindful of the long-term sustainability; the technology is moving along at such a pace that it would not be efficient to have multiple places doing it. One would imagine that we would need a few core, well-funded institutions that would have the infrastructure to replace and modernise as the new technology emerges. It’s perfectly possible; it just needs money and commitment to do it.
Genetic specialist, NHS and university, with a national perspective
With regard to laboratory provision, views differed about the extent to which this would be achieved by the NHS and/or the private sector. One interviewee expressed strong concerns that ‘the NHS has a notoriously poor history of private sector commissioning’ and favoured using NHS laboratory provision if possible. Another interviewee considered that:
Private labs are certainly on the horizon. Currently there are a number of private concerns that are manoeuvring into position to look at this. So at the moment private labs aren’t doing NGS for UK patients, but I can see it coming on the agenda very quickly.
Clinical geneticist, NHS, with a national perspective
Data interpretation
A biometrician’s work was described thus:
The day-to-day profile is about pipelining, and building robust pipelines to put the data through the systems in the diagnostic labs, as well as for the clinical research work that is being done. The targeted panel approach (which I believe LD is more connected with) is where you select certain genes that are known to be associated with LD. You [i.e. a bioinformatician] would use NGS technologies to try and ascertain all the variants across these known genes, and the clinical scientist would then try to come up with some sort of an interpretation based on any of these variants that have been known previously to be associated with a mutation causing LD in this particular instance.
Genetic specialist, NHS
Another interviewee working in a NHS genetics laboratory said:
You need the infrastructure and the machines to do the sequencing. You also need computing infrastructure and bioinformatics support, that’s very important. That’s one of the most important unticked boxes – getting people to do the bioinformatics and having the computer equipment to do it.
Genetic specialist, university and NHS
An interviewee working in a NHS regional genetics service considered that:
In theory, the generation of the test data is going to be relatively straightforward. The complex thing is the data storage and interpretation. . . . And most of the people who are in laboratories, be it biochemical, genetic or whatever, they have not been trained as bioinformaticians, they have been trained as wet lab scientists. It’s completely different training that you need.
Genetic specialist, university and NHS
Another interviewee stressed the importance for data analysis of knowing about the clinical presentation (or the phenotype) of the patient:
It’s the clinical input that people overlook, but yet without that clinical input the bioinformaticians are not going to be able to produce any data that is much use to anybody. . . . Data need to be analysed really carefully, and you can’t analyse it properly without knowing about the clinical presentation of the patient. . . . So the interpretation of all those data requires not only bioinformaticians, but molecular biologists and clinicians who know what those particular diseases look like and can help make a call as to whether there are variants in a particular gene that are likely to be significant or not and can help steer people to look at the right genes in the first place.
Genetic specialist, university and NHS, with a national perspective
Data storage
One interviewee discussed the importance of adequate computing capacity for both data analysis and storage:
Data storage and sharing of information is a huge problem for the NHS, and most genetics labs will not have the infrastructure, computing wise, to deal with this particular problem. And even if they have got it now, it’s certainly going to be a problem in the near future, as we move from panel-based work, limited to dozens or maybe even hundreds of genes, up to whole exomes and whole genomes.
Genetic specialist, NHS
Data storage was considered to be the bigger of these two problems:
Because each trust acts as an individual entity, we have to be responsible for our own data, we have to store our own data. There may come, eventually, solutions around secure cloud-based data sharing, but clearly that is for the future, it’s not here yet. So at the moment most of us are looking for local solutions, storing huge amounts of data on local systems and having to build our own.
Genetic specialist, NHS, with a national perspective
Data sharing
The need for better data storage facilities was also stressed. One interviewee with an academic and research perspective was ‘less concerned about whether the labs are NHS or private sector’ than about ‘access to the data, ownership of the data, and analysis of the data across all health service-recruited samples’. In their view:
What would be catastrophic is if the process of analysis for LD was fragmented into different people doing it and there was no central access to the data. . . . So what is most important over anything else is that the NHS data should be held in a central place that is freely available to other health service users.
Genetic specialist, university and NHS, with a national perspective
Several others also pointed out that it is important that those interpreting the data can access appropriate comparative data to support their work:
Testing and interpretation can be done in the same place or in different places – but research has made it clear that data interpretation predicates on access to large data sets, so it isn’t sensible if everyone is doing this on their small, discrete, little families. Powerful data interpretation requires having access to large data sets. A certain amount of data analysis can be automated, suggesting a centralised system as the most efficient.
Genetic specialist, university and NHS, with a national perspective
NHS staffing and training issues
Several interviewees referred to the need for wider training for clinical staff working with children with LDs to improve their knowledge and awareness of genetic issues:
It’s not just that we need people like bioinformaticians, which do exist to some extent within the NHS, and they definitely exist within the research field, it’s how we develop them within the diagnostic pathway to better interpret genomic data. But also, it’s how we increase awareness of the role of genetic testing as one of the solid pillars in the diagnostic pathway with more patient-facing professionals – GPs, nurses and others.
Interviewee with a national perspective
Staff training: testing and interpretation
The Manchester-based course for bioinformaticians was seen as an exciting development in the use of NGS technologies across the NHS, not just for children with LDs:
Without doubt, NGS is going to be used across the entire pathology discipline, and it’s already being used in different ways in microbiology, virology and ‘across the piste’. I think that clinical bioinformatics will have to be part of all pathology disciplines, so I think we are going to really need people who can give us the information in a format that can be interpreted. I’m not quite sure where the interface between the data and interpretation is going to lie – i.e. between the bioinformatician, clinical scientist and clinician. But obviously we are going to need loads more bioinformaticians.
Interviewee with a national perspective
Staff training: clinical staff
Many interviewees referred to the need for widespread ongoing training in genetics amongst NHS staff caring for children undergoing diagnostic testing for LDs and their families:
We need to look at the capacity of the health service to use the information from NGS appropriately. That means investing in infrastructure that would make speedy and accurate diagnoses scientifically and technically. But it also means investing in education and continuing professional development so that doctors and nurses, nutritionists, etc. who will have sequence information coming into their lives have the skills and support to be able to use the information appropriately.
Interviewee with a national perspective
We need a better-educated workforce – about genomics in general (including its benefits and limitations) – across all health and social care services.
Genetic specialist, NHS, with a national perspective
The wider workforce – secondary specialists, the whole primary care sector, pharmacy, nursing, dieticians, etc. – need to start to understand what genomics is – and how it differs from genetics. This is a real challenge for Health Education England – but programmes are in place and/or being developed.
Interviewee with a national perspective
Some interviewees stressed instead the importance of good team or partnership working and/or of needing to have knowledge only of specific aspects. One participant considered that:
At the moment, the way I work in partnership with my clinical genetics colleagues is that they would deal with the interpretation and the explanation of the ‘spelling mistakes’ in the gene coding. I would very much deal with the day-to-day management of the child’s secondary disabilities and troubleshooting for any additional medical complications that might be predicted because we know what the diagnosis is. So I think we are quite well off here in having that arrangement, but don’t think all localities have it.
Paediatrician/GP with a national perspective
This interviewee also thought that it was important that there are neurodisability-trained paediatricians in every locality ‘so that all the management of the secondary disabilities and all of the surveillance that is required can actually get done’.
A medical geneticist interviewee said that:
A laboratory clinician will do the interpretation, but before I tell someone I need to feel that I agree with the results. It depends how much you trust your clinical scientist, therefore there has to be a strong relationship between the doctor and the clinical scientist.
Genetic specialist, university and NHS, with a national perspective
When asked about the importance of education about genetics for clinicians, a general paediatrician considered that:
[Although] it would be great, because you could have someone giving you hundreds of talks on it, and explaining the nitty-gritty and it would be interesting to know, but in terms of practicality as a front-line clinician, you just need to know that it’s a genetic test, and that there is someone looking at it and interpreting it and saying ‘this could be significant’ or ‘no, it isn’t’.
Paediatrician/GP
One interviewee thought that teaching about genomics should be included in medical education and thereafter provided through CPD. In addition, this interviewee thought that a significant proportion of the current workforce need training (or at least considerable awareness raising) in this area. There would appear to be considerable scope for appropriate organisations to develop a range of different training media for this, including web-based opportunities. As one interviewee stated:
Classroom things are always good; and I think online is fine. It is very effective when the problem arises to have access to information. I mean, you can educate people around the area, but until they come across the need for it, I suspect they won’t really engage fully.
Paediatrician/GP
Another interviewee mentioned the effect of NGS on the process of consenting patients and handling findings of uncertain significance, both of which have a significant educative element:
[With NGS] a lot of education will be needed. You will generate a lot of findings of uncertain significance. So we will need a bigger work force, and education for people doing the consenting of these patients. It won’t be just the domain of the clinical geneticists; the paediatricians will be doing a lot of consenting. They will need education, and they will need more time.
Genetic specialist, university and NHS
An interviewee with an international perspective considered that it was important to have more rare disease specialists, as increasing numbers of new rare diseases would be diagnosed with NGS technologies:
For these rare diseases, we need clinical geneticists. At the very least, we need rare disease specialists. Whichever way the technology goes, that’s where the investment should be. Actually, more than in the bioinformatics. In the long term, I think the bioinformatics will sort itself out.
Genetic specialist with an international perspective
Genetic counsellors
Mixed views were expressed about the need for more genetic counsellors, although most of the interviewees considered that many more of them would be needed with the introduction of NGS technologies:
There are two reasons why you need more counsellors; the first is because this test is much more widely applicable than previous tests. And that means that you will be doing more testing, and so more patients get tested. So you need more counsellors because more patients get tested. But you also need more counsellors because the process of counselling for these complex tests is more involved, so it actually takes longer to counsel for these more involved tests than it would for a very simple test. . . . So I do think that more counsellors, or more counselling time, are required.
And will the counsellors need training in a wider number of areas?
Absolutely, I think more so than the scientists. The counsellors will need training on how to inform patients of the implications of this testing. That certainly has been our experience of rolling out the current testing we are using, that counsellors find it quite hard to understand the implications, the full implications. This is partly because you don’t know what the implications are – you sometimes can’t predict them going in to the test.
Furthermore, as the use of clinical NGS becomes more widespread, current counsellors would need to undergo the relevant genetics training to enable them to work effectively with families experiencing these new technologies:
Finding the time and the CPD to get existing counsellors trained when they are already very busy is going to be an issue and a problem.
Genetic specialist, NHS
A genetics counsellor explained that the delivery of genetic counselling may change considerably:
If we can test for lots of different conditions once [i.e. with NGS], then hopefully families won’t have a diagnostic odyssey. And that may have implications for how genetic counselling works. . . . Rather than putting the focus and attention in the very early stage, genetic counsellors are more likely to pick up families after they have got their diagnosis, to help with the adjustment, to help with the understanding. Because probably the results you are generating are going to be more complex if we are testing for lots of different things at the same time.
Genetic specialist, NHS, with a national perspective
This in turn raises additional problems:
One of the concerns for patient groups, and perhaps for [genetic counsellors] as well, is that those types of models where we are providing more post-diagnostic support will be great for the families that get a diagnosis, but where it might fall down is that it won’t give the same level of support to families that don’t end up with a diagnosis from any of these tests. And we won’t know if that’s because they don’t have a genetic diagnosis or the technology just hasn’t got there yet. So if all the NGS tests that we are able to throw at families all come back normal, it does leave this group of individuals without a diagnosis and without support.
Genetic specialist, NHS, with a national perspective
An alternative view was provided by a paediatrician interviewee, who works closely with the local clinical geneticists. This interviewee considered that there was possibly a greater need for more nurse specialists than for more counsellors, especially in places where clinical networks are less well developed. When asked if more genetic counsellors were needed, the interviewee replied:
I don’t think so. Certainly in our patch we cope across the consultant clinical geneticists and myself and my team. . . . I think they bring their nurse specialists to certain conditions and it may be that more nurse specialist posts will be developed around particular families of conditions as more knowledge becomes available. And then it would become more highly specialised and then would have resource implications. So, at the moment, I know in our area that there is a clinical nurse specialist for neurofibromatosis and [one] for fragile X syndrome. . . . But you can see in the future that there may well need to be other nurse specialists with particular niche expertise that would need to give extended support, perhaps where networks are less well developed.
Paediatrician/GP with a national perspective
Another interviewee (a consultant community paediatrician) described how their service often uses specialist health visitors to support families:
Within my service, I have specialist health visitors who offer support to families, so that would be our normal system. But if it was a condition that we were very unfamiliar with, we would liaise with the genetic counsellors around that.
Paediatrician/GP
Much of the above discussion was summarised thus by a professorial interviewee:
Medics that can interpret clinical history and molecular results are going to be gold dust, because everyone is going to want these genome results and no-one is going to know quite how to interpret them. We either need an absolutely huge education campaign with lots more clinical geneticists, or we need many more clinical geneticists working with doctors. I would say that the new breed of counsellors is going to be able to take on quite a large amount of that work. It needs genetic counsellors who are highly trained in molecular genetics as well as the clinical skills. And it is a big range of needs, going from data interpretation to explaining to patients. . . . You also need local leaders who are going to push things forward, who are going to tackle the issues and difficulties you have with developing genetics services. In addition, there has got to be a close relationship between the scientist doing the high throughput sequencing and the people interpreting it. We are going to have to have science centre hubs close to the local clinicians who are going to interpret the result. I think the regional system is pretty good for these hubs.
Genetic specialist, university and NHS, with a national perspective
Other aspects
Need for public education
Concern was expressed by one interviewee that the private sector may become involved in promoting WGS to the public. Another interviewee, however, thought that, even with clever marketing, such private sector involvement was unlikely to take off, partly because of the problems of producing any meaningful data for the client (especially in the absence of knowledge of the person’s phenotype):
But without some clear purpose for WGS, i.e. for somebody without any clear clinical question, it is likely to be almost impossible to find useful information. I doubt that it’s going to be a problem – the fear of the ‘genetic iceberg’, as it used to be called, is probably a bit misplaced. Having said that, if WGS does become popular amongst the ‘worried well’ – ‘recreational’ genetics was how one colleague in the DH [Department of Health] described it – it is clear that any interpretation will have to be done before results are handed to a GP, who could not reasonably be expected to interpret it.
Interviewee with a national perspective
Importance of family support
Several respondents, especially those working directly with children undergoing diagnostic testing for LDs and their families, stressed the importance of organisations offering them support and information. One respondent, when discussing problems associated with finding out more about some conditions, said:
I think Unique’s rare syndrome support has done a wonderful job in pulling together the literature on these new, rare microdeletions and duplications [i.e. the ones with alphanumeric names rather than being named syndromes] and developing leaflets. They’re fantastic, and that does give people some information.
Genetic specialist, NHS, with a national perspective
A representative from Unique stated that:
The increasing diagnostic capabilities [of microarrays] are brilliant for us, but there is also an implication that at some point we are a bit nervous that we won’t be able to cope with the increased numbers. It’s difficult to get a really good handle on how many more families there will be and what the real impact is. And we are just sort of waiting to see what happens with DNA sequencing.
Interviewee with a national perspective
Furthermore, Unique gets information about diagnoses only from the families and asks them to get a copy of their genetics report from their doctor or geneticist if they have not been given one:
I think that what we are looking at in the near future is combinations of [traditional testing and NGS], but probably eventually it will just be NGS. What we feel a bit nervous about with NGS is the ability to interpret the results. And about our ability to interpret the results, because we don’t really know what families are going to be given, so we don’t know what we are going to be dealing with. So it’s a bit of unknown.
Interviewee with a national perspective
Changes in service commissioning
One interviewee hoped that the development of directly commissioned services at a national level would be a great improvement on recent arrangements:
I think you have to look at the structure the NHS is creating for directly commissioned services, those that are not appropriately dealt with through the local commissioning services. It’s all about developing a level playing field for those who are in need of specialist services. Historically, under regional specialist commissioning groups in previous arrangements, there was significant regional variation in terms of access to specialised services. NHS England is claiming that it will put systems in place that will ensure that patients who need specialist services are able to access them on the basis of need rather than geography.
Interviewee with a national perspective
Another interviewee, considering the bodies with overall responsibility for developing awareness in the NHS about the potential of NGS technologies, summarised several themes thus:
The Royal Colleges have a big role to play in education and making sure the understanding is there and making sure the professionals know what to expect and how to deal with that. NHS England has a big role to play in ensuring that the NHS is ready for that, and that includes service reconfiguration and looking at workforce redevelopment. And also, as we move into the new world of nationally commissioned specialised services, that CCGs [Clinical Commissioning Groups] and others have an understanding of the importance of NGS in the diagnostic pathway, and how that can be accessed and what it can deliver.
Interviewee with a national perspective
Appendix 6 Value of a diagnosis
Introduction
Published articles on diagnosing LDs in children sometimes include a discussion of the value of a diagnosis. Although this aspect was not explicitly included in the initial brief for this scoping study, several of the interviewees expressed their views on the importance of a diagnosis to the families and to clinicians and other professionals. These are summarised here as they add useful contextual information to the scoping study.
With regard to published articles, see Table 3 for a brief summary of the benefits of a genetic evaluation and Table 4 for parents’ views on the advantages and disadvantages of genetic testing for LD. Costain et al. 73 published an interesting study on the caregiver and adult patient perspective on the importance of a diagnosis for 22q11.2 deletion syndrome. They include a useful summary of the perceived benefits within the framework of Maslow’s hierarchy of needs and their study results both validate and expand on the theoretical benefits of a diagnosis proposed by clinicians and researchers. Although their study focuses on the value of a diagnosis of 22q11.2 deletion syndrome in adult patients, its findings are also relevant for the genetic diagnosis of ID during childhood.
Rosenthal et al. 74 explored parental attitudes towards a diagnosis in children with unidentified MCA syndromes. The parents in this study had been aware of their child’s anomalies for 2–23 years and all had sought multiple evaluations to find a diagnosis. They identified six areas in which parents claimed a diagnosis would have an impact: labels, causes, prognosis, treatment, acceptance and social support. Significant issues included obtaining special education services; anticipating the child’s future and potential medical complications; life expectancy; recurrence risks; finding sources of social support; and ensuring that the child was receiving appropriate treatment. The authors concluded that ‘The significance of diagnostic information is complex and varies for different parents. Providers should explore the underlying issues associated with a parental quest for a diagnosis in order to identify and address specific concerns’ (p. 106).
Effect of no diagnosis
An interviewee with a national perspective pointed out that, although having a diagnosis does not necessarily alter the prognosis, it is important to look at life for families without the reality of a diagnosis and at the opportunities that are created by having a diagnosis.
If you haven’t got a diagnosis, you don’t know what to expect. The future progression of the condition is something you discover as it happens. That means you can’t anticipate, and you can’t prepare. If you’ve got a degenerative condition, for example, that results in physical and mental impairment, you can’t have things set up in advance to take account of that. It makes it difficult to organise services and support, and if there is an intervention available – a therapy that can improve things or manage the symptoms better – then you probably don’t get access to it. So although having a diagnosis in itself doesn’t alter the trajectory of the condition, it can make a huge difference to how you understand the situation in which you find yourself.
Interviewee with a national perspective
An interviewee with a national perspective considered that:
I think we forget about how many people with genetic conditions do not receive a diagnosis. I think we need to reflect that there is a considerable percentage who do not, and live in the situation where their condition really just gets palliative care as it goes on its merry way, because they have no real information of what they have and what can be done about it.
Interviewee with a national perspective
If the condition is an inheritable one, a diagnosis means that the family can be forewarned and, if they wish, take steps to avoid having another child with the same disorder. A paediatrician shared this experience:
There are many families that I know that have gone for years before they have achieved a diagnosis. There was a child recently, who was at least 9 before they achieved a diagnosis that she did have a chromosome deletion. And this was a family, although they were brilliant parents, and absolutely adored their child, said that they could not risk having another child with these difficulties, due to the amount of care and everything she needed. And subsequently they have gone ahead and happily had an unaffected child, which they never would have had the confidence to do without that additional information. So I do think [a diagnosis] is enormously important.
Paediatrician/GP
Removing reproductive uncertainty is therefore an important benefit of having a diagnosis.
It was suggested by an interviewee with a national perspective that, even with more sophisticated testing techniques, there would still be many children without a diagnosis:
And the whole syndromes without a name issue needs to be assessed, or referred to as ‘pre-diagnosis care’. When somebody is obviously not well, but we don’t know what it is.
Interviewee with a national perspective
Benefits of a named syndrome diagnosis
A NHS genetic specialist pointed out that:
In my experience, parents are desperate for a diagnosis. One of the difficulties they have with the new technologies like arrays is that they don’t end up with a named syndrome diagnosis, they end up instead with something like ‘40q21 microdeletion’.
Genetic specialist, NHS
Nevertheless, this interviewee considered that it is still better to have a diagnosis, even if it does not have a syndrome name, as it can at least be explained (for example) that the child has a microdeletion that takes out some of the genes affecting brain function.
Effect on the emotional well-being of the family
The emotional impact of having a diagnosis is potentially hugely beneficial to families. It was opined that having a diagnostic title makes a massive difference to families, even though any treatment or therapy does not necessarily change:
The more detailed view we can get of the genetic information, the more we can give parents answers, and the more it helps them to move forward and cope with the difficulties of the child.
Paediatrician/GP
This interviewee pointed out that:
Research shows that the earlier the family received the diagnosis, the earlier they can move on – I think there’s an emotional reflex mechanism that helps with the grieving process of knowing you’ve got a child with special needs.
Paediatrician/GP
This interviewee also pointed out that having a diagnosis also reduces the opportunity for blame:
Families with a child with complex neurodevelopmental needs often split up – there’s an increased incidence here – probably due to the stresses and strains. If you help a family understand why, usually they can move through the grieving process quicker and there is less anger and fallout. Potentially that may make it easier for families to come to terms and cope. So there are lots of subtle potential knock-on effects.
Paediatrician/GP
A NHS genetic specialist had found that:
There are a very small number of parents who don’t want [testing] because they don’t want their child to be labelled, and because they have a fear of finding out something genetic. But that’s a very small number of patients. And most of those people, in my experience, go away and think about it and decide to have testing in any case. And when I say small, I mean two, and I see 400–500 families a year.
Genetic specialist, NHS
Practical help for family
For children with LDs:
Having a diagnosis opens doors, particularly as the child moves towards school age, to education and social care. They can access a specialist social services disabilities team, rather than trying to go through a general social services team, which has experiences of supporting different problems. So I think that definitely it makes a difference.
Paediatrician/GP
With regard to attending school:
It can be easier to access a disability school (even if this is obviously appropriate for the child) if you have a title for their particular syndrome – having a name would avoid the need for some meetings with educational psychologists, etc.
Paediatrician/GP
Another interviewee with a national perspective pointed out that ‘providing a diagnosis can also help with statementing and education statementing and other social educational needs’. A diagnosis can also help parents to access respite care – ‘there are lots of wins all over the place for a family’.
It was also pointed out by a paediatrician/GP with a national perspective that:
A diagnosis gives the opportunity for families to be involved in choice making, about levels of care, about levels of intervention and, if the child’s death is inevitable, about where they want the child to die, how they want their care to be.
Paediatrician/GP with a national perspective
Timing of the diagnosis
One interviewee made an interesting observation about the right time for giving families a diagnosis. Although recognising that families usually want an answer as quickly as possible, this may not always be appropriate:
But it can also be a problem if you give a really devastating diagnosis when a child is very, very young, because the family haven’t had a chance to bond with that child before they know how difficult things might be. So there are always pros and cons of when you get a diagnosis, but I guess families usually say they want it as early as possible. As a professional, you sometimes think that would be too early to tell them. Getting an answer really early isn’t always easy for families to deal with. Sometimes the doctors go and see newborns, and we then review them in a few months’ time to see how they’ve been getting on. Families often want an answer when the child is 2 days old, and we rarely can get an answer. And often when you see them a few months further down the line, they have really bonded with that child, and that child is a person that they know rather than someone that arrived yesterday. So I think it’s a benefit to have things speeded up to some degree, but not necessarily immediate answers, if that makes sense.
Genetic specialist, NHS
Other benefits for families
A paediatrician/GP with a national perspective cited the following benefits of a diagnosis, based on their personal experience:
I can list endless examples of where having the right diagnosis has informed clinical care, has stopped the parents blaming themselves about their child’s predicament, and has often led to other family members having new insights into their own predicaments in terms of their learning of their health.
Paediatrician/GP with a national perspective
Another interviewee with a national perspective pointed out that, with a clear diagnosis:
You can offer risk assessments to other members of the family as to whether they are likely to have children with the condition.
Interviewee with a national perspective
A NHS genetic specialist considered that:
Not having a diagnosis (as a way of getting access to resources) is particularly problematic for people with ‘mild’ developmental disability, as in many ways they are the most incapacitated. If you’ve got really profound difficulties, someone is going to look after you (even if the quality of this care may be very variable) – there is an acknowledgement by society that you will be looked after at some level. However, people with mild problems find it impossible to get help and are actually completely unable to function – they are so vulnerable. Getting help for people with mild disabilities is just getting more and more difficult. It’s not so bad when you are of preschool age, but the older you get, then the harder it becomes.
Genetic specialist, NHS
However, one interviewee also pointed out that there is some risk that knowing the diagnosis may lead to inappropriate use of this knowledge. This interviewee considered that it may be used as a tool for rationing scarce resources or making prejudicial and discriminatory decisions about people’s quality of life and the care that they should receive, rather than being used to inform rational and evidence-based decision-making processes.
Benefits for other professionals
A diagnosis can be hugely helpful to professionals, not only clinicians but also those in social care and education, as it may help in terms of managing the ways that care and support are provided, ‘enabling the child to fulfil their potential in a more efficient way, whatever that might be’. One interviewee with a national perspective pointed out that:
Some conditions require specific learning strategies to help the child develop skills and independence to whatever extent is possible. So I think it is a very important issue that sometimes is neglected by clinicians, because sometimes they say that [a diagnosis] doesn’t make any difference to what they do, so why go chasing after it?
Interviewee with a national perspective
This interviewee believes that NGS has the potential to make ‘a huge difference’, as making a diagnosis will enable the health service and other statutory agencies to plan more systematically for the provision of health and support. This will also establish the need for interventions in a way that makes best use of scarce knowledge and resources.
Effect on other families
Families without a diagnosis can also benefit when another family receives a diagnosis:
There is a kind of Facebook members’ community for the SWAN project. A number of families have already received a diagnosis as a result of being part of DDD, and if Facebook had audio you would hear the cheer that goes round the community.
Interviewee with a national perspective
A diagnosis received by one family is seen as encouraging for others as well. It helps to show the situation is not immutable. The progress that comes in advances in sequencing and the interpretation of sequence data has provided scope for optimism. They are realistic; they know that this is not the imminent arrival of miracle cures, that there is still a long road to walk down, and you may never get to the end of it in terms of your life or your children’s life, but it begins to show that this is not just an immutable and unsolvable problem. And that is good.
Interviewee with a national perspective
Another indirect benefit for other families is that making diagnoses helps to create the critical mass of knowledge that is essential for research:
So what was once incurable or untreatable or life limiting can move back up the down escalator towards something that becomes treatable and manageable and, hopefully, eventually curable or preventable.
Interviewee with a national perspective
Conclusion
The following quote from a highly experienced genetic specialist summarises many of the points made above:
I think these patients get a very poor deal, they’ve always got a poor deal and I think there is still a vast amount that should be done. What is very clear is that the families hugely appreciate the point at which we identify the cause of the disease. And I think it’s therapeutic in itself. And I think one has to be mindful that in the next 5–10 years we are not going to make significant inroads into curing these diseases. But I think it’s still terribly important. And I think knowing your child has a problem that is not related to your parenting is incredibly important. I think making an early diagnosis is very important. It can affect how people bring up their children, what facilities they tap into, and also how much longer do we have to be waiting for a diagnosis. I’ve got some patients that have been waiting 30 years for a diagnosis. And they still appreciate it when we’ve found the answer.
Genetic specialist, NHS and university, with a national perspective
Appendix 7 Additional interview quotations: new systems and safeguards
This appendix provides an evidence base for material in Chapter 3 that relates to new systems and safeguards. It reports verbatim some of the views expressed by the interviewees about various topics. Because of their different experiences and areas of expertise, not all of the interviewees had views on all of the topics. However, the quotes show where views were broadly similar irrespective of profession and where opinions differed across the professions.
Overview
Although the issues discussed here are very important, they are not necessarily new nor specific to using NGS technologies, nor unique to the diagnosis of LD in children. As one interviewee stated:
Genetics has probably spent more effort on the ethics, ethical questions and the ethical, legal and social implications than any other area. And I’m always sort of sceptical that NGS and WGS raise any new, spectacularly different situations, any new ethical dilemmas, really.
Interviewee with a national perspective
Giving informed consent
Participants highlighted the ethical challenges and practical dilemmas associated with informed consent, especially getting patients to consent to something unknown that may not even relate to their presenting condition:
The consent and confidentiality issues that come around are still going to need a degree of resolution. How do you consent patients for a whole raft of things that may or may not relate to the condition that they are presenting with? And that they may or may not be able to treat, or manage or do anything about, and all of the implications? So we are talking about obtaining really blanket-level consent for really anything that could be discovered, and that’s quite an issue. And I’m not sure quite how we are going to resolve it.
Genetic specialist, NHS
There is a conversation to be had with people at the time they are being tested to find out what they are expecting of the tests and what sort of information they want to be fed back. And is it very difficult to get consent for something when you don’t know what it is that you are consenting to. How do you consent to whether or not you want to know something, when you don’t yet know what that fact is? And those sorts of conversations with patients are the things that I think it’s easy to underestimate how long they will take.
Genetic specialist, NHS
Furthermore, the resource implications must not be underestimated:
The test can be taken anywhere. But right from the start someone has to see the patient, take a careful family history, and examine them carefully so you know what you are thinking about. They then have to consent the family appropriately, and that means discussing with them exactly what is going to be done, what sort of results will be generated, and establishing what they want to know and have fed back and when and so on. The sequencing bit is easy, but the analysis and interpretation are then difficult.
Genetic specialist, NHS
Reporting test results: managing incidental findings
Participants also highlighted that one of the most controversial aspects of WGS is the ethical issues that may arise from its use (or indeed from the use of other NGS technologies) in clinical and research settings. Almost all of the interviewees mentioned this as being an area where further work is needed. The preference was for the work to be undertaken at a national level but also to draw on the experiences of other countries. However, as one interviewee said:
We need to keep this proportionate, because there are also ethical issues about deciding not to do something, and these very often get swept under the carpet.
Interviewee with a national perspective
With regard to the disclosure of incidental findings in Britain, one interviewee considered that:
I think there has been a lot of furore recently about this, because the Americans released some guidelines about incidental findings and said that they must be disclosed. And the Europeans said we shouldn’t be looking for them in the first place – we should only be doing targeted testing. They represent two opposite ends of the spectrum, and I think the reality will be somewhere in the middle.
Interviewee with a national perspective
Ethical and other frameworks
One interviewee raised the need for further research on false-negative rates:
I’d like to see NGS, I hope we will see it, readily available for diagnostic purposes in laboratories, but properly validated so that we can rely on the results that we get and with as low as possible false-negative rate, and false-positive rate. The implications of false negatives and false positives are very different. I think that one of the Achilles heels of NGS is always thought to be false negatives, so at the moment we miss some mutations. So if you’ve screened a particular gene and not found a mutation, how sure can you be that that patient hasn’t got that condition? At the moment, not very sure at all. Somehow, false positives seem to be less of a problem, just from the way things are working out scientifically at the moment. And then, generally, you can look at the clinical phenotype of the patient, and if you find a variant and the patient has no signs of that condition whatsoever, you can say, well clearly the patient hasn’t got that condition, clearly it’s not significant. We don’t know at the moment what the false-negative rate is, and that is a concern, so I’d very much like to see that addressed over the next few years.
Genetic specialist, university and NHS, with a national perspective
In addition, the importance of a regulatory framework was raised by some of the interviewees, as was the importance of public education and public debate, as illustrated by the following quote:
We all need to be vigilant about developments in the relationship between the private sector and the public sector and to ensure these are underpinned by an appropriate regulatory framework. . . . This requires vigilance from all of us, it’s not just the responsibility of the government, but it’s also part of our role as citizens to be more articulate about the things we value. . . . As the science advances and the whole interface between bioinformatics and genomics creates new possibilities, it is something we need to engage with. There needs to be public education and public debate in order to ensure we create a regulatory environment. . . . We need a regulatory framework that is proportionate, that is flexible, that is likely to permit what society would largely regard as ‘a good thing’, as well as disincentivise those things society would probably regard as being undesirable.
Interviewee with a national perspective
Pace and extent of change
Some interviewees based their views on their personal experience of working with children with LDs; others had a wider perspective on the clinical use of NGS technologies in the NHS:
I think within the next year we will get some gradual changes, but I don’t think there will be a big revolution within the next year. I think within the next 5 years, things will look very different to what they look like now, but whether we have mainstreamed them to be available to people routinely or if there would still be access through rather ad hoc means, I’m not sure.
Paediatrician/GP
Five years from now, I think there still will be clinical geneticists, the paediatrician will be sending off the test, and we would have moved on from array to some sequence-based test, which would cover more of the genome than we currently cover, and look at sequence as well as copy number. Uncertain findings will still be the case in 5 years; maybe in 20 years we’ll know what we are doing.
Genetic specialist, NHS
Within the next 5 years for LD, you could have the resolution to be screening the whole exomes and the whole genomes, but I would throw caution to the idea of whole genomes because currently the scientific community do not understand the whole genome, the complexities or the relevance to any particular disease. So diagnostically it would not be feasible.
Genetic specialist, NHS
I think at the moment we don’t know whether we will be doing whole exome or gene packages, I think we just don’t know the answer to that. And I think the question of exome versus whole genomes is another step beyond that, if you like . . . . And I think we will have an answer to the gene packages question within 3 years. The exome versus whole genome might take 5 years.
Genetic specialist, NHS
I think the quality of sequencing is going to improve, and I think in 5–10 years we will be doing WGS up front; it will be cheap enough to offer that front line. What they are doing on the DDD study, that will become standard clinical practice. So I think there will be ongoing refinement of the sensitivity and specificity of the testing that is going to be available. And I see that only as good, as it saves children from other expensive, painful and more intrusive tests.
Paediatrician/GP
I think within the next 3–5 years we’ll have an increasing number of panels around very heterogeneous conditions, including for retinal diseases, cardiac diseases, epilepsies, these sorts of things. And there will be the gradual introduction of exome sequencing, which I think in time will supersede all the panels. I think exome sequencing will get very good in terms of its coverage, its ease. It’s a single pipeline, it’s a single test. If you can apply that to every individual with a genetic condition, it’s going to be so much easier than interpreting this panel or that panel. We will have our exome sequencing up and available within the year, but it won’t be widely taken up. I think it will be more widely taken up over the next 3–5 years. And I think after 5 years it will replace panels. But there is a window of requiring panels.
Genetic specialist, NHS
The NHS is very slow, if I’m honest with you. But for good reason, it’s not just tardy! There are good reasons why the NHS brings on new technologies in a slow and measured way. I’m conformable with that. Panel-based testing is still not common in the UK, there still aren’t that many tests available even though there is a lot of talk about it. There are only a handful of tests that are actually available, and they are not very big panels, most of them. I think they will develop over the next 2–3 years, and there will be more available.
Genetic specialist, NHS
I would have thought that a timescale of 5–10 years was reasonable to anticipate. Hopefully I will be proved wrong and it will happen more quickly. And if it’s not 5–10 years, but 10–20 years, don’t forget that this is the first time in human history that we have had the opportunity to understand the basic biology. If it takes 20 years instead of 5, that’s still a blink of an evolutionary eye, although for patients and families who live with the disease today, it’s still far too long. They want it yesterday. But I think it’s important to recognise, and I think it’s one of the things that we have lost, is that things take time.
Interviewee with a national perspective
Finally, one interviewee neatly summarised one of the challenges of working in genetics thus:
Genetics . . . you think you understand it, you think you’ve got there, you think you’ve got your head round everything and you totally understand whether something is clear or not, and then things move on and you realise that actually things are even more complicated than you thought. . . . And even things that you thought were certain maybe aren’t quite as certain as you believed them to be.
Genetic specialist, NHS
Glossary
- Array comparative genomic hybridisation
- This is a technique that allows the detection of losses and gains in DNA copy number across the entire genetic genome without prior knowledge of specific chromosomal abnormalities. It produces a map of DNA sequence copy number at multiple chromosomal locations throughout the genome and allows the detection of genetic deletions, duplications and amplifications.
- Bioinformatics
- A discipline that allows for the extraction of useful results from a large amount of raw data and which therefore aids in the interpretation of sequencing data and annotating genomes.
- Chromosomal microarray analysis
- Same meaning as Array comparative genomic hybridisation.
- CNV-seq
- A method to detect copy number variation using high-throughput sequencing.
- Comparative genomic hybridisation
- Same meaning as Array comparative genomic hybridisation.
- Copy number variation
- A variation in the number of copies of sections of genetic code. Can be normal variation or pathogenic.
- Cytogenetics
- A branch of genetics that is concerned with the study of the structure and function of the cell, especially the chromosomes.
- Deoxyribonucleic acid
- Deoxyribonucleic acid is a nucleic acid, the sequence of which forms the genetic instructions that in turn determine the proteins that make up living organisms. Deoxyribonucleic acid is a double-stranded molecule held together by weak bonds between base pairs of nucleotides.
- Deoxyribonucleic acid sequence
- The relative order of base pairs in a fragment of deoxyribonucleic acid, a gene, a chromosome or the entire genome.
- de Vries score
- A score that takes into account the presence of malformations, dysmorphism, growth anomalies and familial history to predict the likelihood of a chromosomal aberration.
- Diagnostic yield
- The likelihood that a test or procedure will provide the information needed to establish a diagnosis.
- Exome
- The sections of an individual’s genes that are copied (transcribed into messages) are called exons. Exons are thought to make up approximately 1% of a person’s genome. Together, all of the exons in a genome are known as the exome.
- Fluorescence in situ hybridisation
- A cytogenetic technique that is used to detect and localise the presence, or absence, of specific deoxyribonucleic acid sequences on chromosomes. It is more sensitive than karyotyping and less sensitive than array comparative genomic hybridisation but can be used only to target areas of interest on a chromosome, not to screen all of the deoxyribonucleic acid.
- Gene
- The basic biological unit of heredity – a segment of deoxyribonucleic acid that contributes to phenotype/function.
- Genome
- Sum total of the genetic material included in almost every cell of the human body.
- Genotype
- The genetic make-up of an organism or group of organisms with reference to a single trait, a set of traits or an entire complex of traits. Also, the sum total of genes transmitted from parent to offspring.
- Incidental findings (unsolicited findings)
- Findings discovered unintentionally during a clinical or research investigation that are unrelated to the original investigation.
- Karyotyping
- A test used to count the number of chromosomes in a cell as well as to look for structural changes in chromosomes.
- Massively parallel sequencing
- A technique used in next-generation sequencing.
- Mosaicism
- Mosaicism is the term used when a genetic or chromosomal abnormality occurs in only a proportion of the body’s cells rather than in all of them. Examples of mosaicism include mosaic Down syndrome, mosaic Klinefelter syndrome and mosaic Turner syndrome.
- Next-generation sequencing
- Next-generation sequencing is an approach to genetic testing that allows the simultaneous testing of large numbers of genes from a standard venous blood sample. Its novelty is its speed and reduced cost.
- Nucleic acids
- Large biological molecules essential for all known forms of life. They include deoxyribonucleic acid and ribonucleic acid.
- Nucleotide
- Organic molecules that serve as the subunits of nucleic acids such as deoxyribonucleic acid and ribonucleic acid. Nucleotides are composed of a nitrogenous base, a five-carbon sugar (ribose for ribonucleic acid or deoxyribose for deoxyribonucleic acid) and at least one phosphate group. They are abbreviated to G, A, T, C and U.
- Pathogenic
- Causing, or capable of causing, disease.
- Pertinent findings
- Findings that have been generated or sought with the purpose of answering a particular clinical or research question.
- Phenotype
- An organism’s observed properties, such as development or behaviour.
- Ploidy
- The number of sets of chromosomes in the nucleus of a biological cell.
- Ribonucleic acid
- A large single-stranded biological molecule that is formed of a string of individual nucleotides. Different types of ribonucleic acid perform multiple vital roles in the coding, decoding, regulation and expression of genes.
- Sanger sequencing
- A method of determining the sequence of nucleotides in a short segment of deoxyribonucleic acid. Used as the standard method of sequencing before the introduction of next-generation sequencing techniques.
- Sensitivity
- The number of truly affected people who are identified by a test, also called the true positive rate. For example, how often is the test positive when a mutation is present?
- Specificity
- The number of truly unaffected people who are identified by a test, also called the true negative rate. For example, how often is the test negative when a mutation is not present?
- Targeted gene sequencing
- An approach that targets the section(s) of the genetic code to be sequenced, rather than sequencing the whole exome or genome.
List of abbreviations
- aCGH
- array comparative genomic hybridisation
- ACMG
- American College of Medical Genetics and Genomics
- AGH
- array genomic hybridisation
- ASD
- autism spectrum disorder
- BACD
- British Academy of Childhood Disability
- CI
- confidence interval
- CMA
- chromosomal microarray
- CNV
- copy number variation
- CPD
- continuing professional development
- DD
- developmental delay
- DDD
- Deciphering Developmental Disorders
- DNA
- deoxyribonucleic acid
- FISH
- fluorescence in situ hybridisation
- GCRB
- Genetic Counsellor Registration Board
- GDD
- global developmental delay
- GOLD
- Genetics of Learning Disability
- GP
- general practitioner
- ICD-10
- International Classification of Diseases, 10th Revision
- ID
- intellectual disability
- ILD
- idiopathic learning disability
- IQ
- intelligence quotient
- LD
- learning disability
- LDD
- learning and developmental disability
- MCA
- multiple congenital anomalies
- MLPA
- multiplex ligation-dependent probe amplification
- MR
- mental retardation
- MSC
- Modernising Scientific Careers
- NGS
- next-generation sequencing
- NIHR
- National Institute for Health Research
- PCR
- polymerase chain reaction
- PHG
- Public Health Genetics
- RAG
- research advisory group
- SNP
- single nucleotide polymorphism
- ST-FISH
- subtelomere fluorescence in situ hybridisation
- STP
- Scientist Training Programme
- SWAN
- Syndromes Without A Name
- UKGTN
- UK Genetic Testing Network
- WES
- whole-exome sequencing
- WGS
- whole-genome sequencing