Notes
Article history
The research reported in this issue of the journal was funded by the HTA programme as project number 08/13/47. The contractual start date was in March 2010. The draft report began editorial review in May 2015 and was accepted for publication in October 2015. The authors have been wholly responsible for all data collection, analysis and interpretation, and for writing up their work. The HTA editors and publisher have tried to ensure the accuracy of the authors’ report and would like to thank the reviewers for their constructive comments on the draft document. However, they do not accept liability for damages or losses arising from material published in this report.
Declared competing interests of authors
Michael Millar was a member of the National Institute for Health Research Health Technology Assessment Diagnostic Technologies and Screening Panel for the duration of the CATCH study.
Permissions
Copyright statement
© Queen’s Printer and Controller of HMSO 2016. This work was produced by Harron et al. under the terms of a commissioning contract issued by the Secretary of State for Health. This issue may be freely reproduced for the purposes of private research and study and extracts (or indeed, the full report) may be included in professional journals provided that suitable acknowledgement is made and the reproduction is not associated with any form of advertising. Applications for commercial reproduction should be addressed to: NIHR Journals Library, National Institute for Health Research, Evaluation, Trials and Studies Coordinating Centre, Alpha House, University of Southampton Science Park, Southampton SO16 7NS, UK.
Chapter 1 Introduction
Use in practice
Central venous catheters (CVCs) are widely used for patients of all ages who need intensive or high-dependency care to provide venous access for resuscitation, drug delivery, intravenous feeding, monitoring and blood sampling. CVCs are associated with an increased risk of bloodstream infection (BSI), which is hypothesised to be caused by organisms tracking along the CVC from the skin or from the external parts of the CVC to colonise the CVC tubing and tip. 1–5
Risk factors for BSI include catheter dwell time, the frequency of ‘breaching’ the line for medication or sampling, multiple compared with single-lumen CVCs and infusion of lipid solution as part of parenteral nutrition. 6–10 The risk of BSI is reduced by strict adherence to aseptic procedures during CVC insertion and whenever the CVC is breached. 11–13 To help ensure staff follow aseptic procedures, audited checklists (called CVC bundles) have been introduced in several countries. 14–19
In this report we focus on children who need a CVC as part of their intensive care treatment. Paediatric intensive care units (PICUs) have one of the highest reported rates of hospital-acquired BSI of any clinical specialty20–23 and BSI is an important cause of adverse clinical outcome and health-care costs in critically ill children. 21,24–26 We estimate that approximately 60% of the 16,000 children admitted to 23 PICUs each year in England require insertion of a CVC as part of their acute care. 27 We do not include CVCs used for very preterm babies in neonatal intensive care or long-term CVCs, which are widely used to administer medication or parenteral nutrition for children with conditions such as cancer, cystic fibrosis, renal failure or short gut syndrome.
Rationale
Central venous catheter impregnation with anti-infective substances has been used for over 25 years. 28 Recent systematic review evidence from 48 randomised controlled trials (RCTs) and cost-effectiveness analyses including 11,586 patients demonstrated substantial benefits of impregnated compared with standard CVCs for catheter-related BSI (CR-BSI). 2,5,28–30 One of the most recent systematic reviews included a meta-analysis of direct and indirect comparisons of different types of impregnated and standard CVCs. 28 Heparin-bonded or antibiotic-impregnated CVCs were found to be the most effective options, being associated with similar reductions (70–80%) in the risk of CR-BSI. Heparin bonding acts by reducing thrombus formation and bacterial adherence to thrombi, but the bonding agent, benzalkonium chloride, also has anti-infective properties. Antibiotic-impregnated CVCs act by preventing biofilm formation and thereby prevent bacterial colonisation.
Despite the large number of RCTs and the substantial reductions seen in the risk of BSI in adults, impregnated CVCs have not been recommended for children in US or UK guidelines and their use in UK practice has been limited. 3,15,31,32 A recent survey showed that impregnated CVCs had been adopted for some or all children by less than half of British PICUs surveyed. 32 Lack of implementation in PICUs relates to (1) gaps in the evidence relating to children; (2) concerns about the quality of previous trials; and (3) uncertainty about the generalisability of RCT findings to settings where improved infection control strategies have been associated with steep declines in BSI rates. 33,34
In children, there is a lack of evidence on the most effective type of CVC and on the expected effect size. According to the network meta-analysis by Wang et al. ,28 heparin-bonded and antibiotic-impregnated CVCs are the most effective options, having similar effects compared with standard CVCs. However, there is a lack of evidence on which type of CVCs would be most effective as there have been no adequately powered, direct ‘head-to-head’ comparisons of these options. 28 In the UK, the additional costs of heparin-bonded or antibiotic-impregnated CVCs are similar and so the decision on which type to adopt depends on their relative benefits and adverse effects. Only one of the eight RCTs comparing antibiotic-impregnated CVCs with standard CVCs (n = 2073 patients) included children and this study was terminated early because of a lower than expected event rate. 35–42 As CVCs for children are much narrower than adult CVCs and the risk of thrombus formation, bacterial adhesion and infection is much higher, it is hypothesised that the relative effect of antibiotic-impregnated compared with standard CVCs may differ in children and adults. Evidence is stronger for the benefits of heparin-bonded CVCs, as two of the three RCTs comparing heparin-bonded CVCs with standard CVCs (n = 472) included children. 43–45
Several systematic reviews have raised concerns that the poor quality of previous studies means that the benefits of impregnated CVCs may have been overestimated. 5,29,46,47 First, few trials have reported good concealment of treatment allocation or blinding of clinicians to the intervention and many have failed to account for losses or withdrawals, all factors that could lead to overestimation of the effect. 5,29 Second, all previous trials relied on CR-BSI as the primary outcome measure, which requires positive cultures from the blood and catheter tip. This measure is highly susceptible to bias, as the tip can be easily contaminated during removal and residual antibiotic in the catheter tip may inhibit culture in the laboratory. Aside from the potential biases in measuring CR-BSI, impregnated CVCs may impact on all BSIs after CVC insertion, not just on CR-BSIs, and on the risks of mortality, complications and increased length of stay associated with BSI.
Few trials have determined the effect of impregnated CVCs on all BSIs in PICU in the context of ongoing reductions in BSI rates associated with the introduction of CVC care bundles. 11,13,14,33,48 Neither of the two trials of heparin-bonded CVCs in children and few of the trials of antibiotic-impregnated CVCs in adults have been conducted in the context of these strenuous efforts to reduce BSI. It is not known whether or not the relatively large reductions in relative risk (RR) and absolute risk seen in trials predating CVC care bundles would be sustained in PICUs where rates of infection have already been reduced by improved CVC care. 34 Even though a UK cost-effectiveness analysis estimated that impregnated CVCs would be cost-effective given baseline rates of CR-BSI as low as 0.2%,29 there remains the question of whether or not the relative effect of impregnation would be less given improved catheter care.
Risks and benefits
Prevention of BSI is undoubtedly a clinically important outcome. Although evidence on attributable mortality varies, BSI is clearly associated with a longer stay in hospital and more intensive support. 20,21,24–26,49 For children in intensive care, CR-BSI has been associated with an additional 9–21 days’ stay in hospital (6.5–15 days in PICU). 24–26 In adults, the additional acute health-care costs attributable to a BSI are an estimated £9148 per patient and could range between £2500 and £71,000. 29 The few studies of the costs of BSIs in PICU patients have found a difference of US$33,039–39,219 in PICU direct costs between infected and uninfected patients. 21,25 However, quantifying the effects of BSI are complicated by the time-dependent exposure: BSI increases hospital stay; increased length of stay is a risk factor for BSI. 50 Estimates of the attributable length of stay are subject to this time-dependent bias, leading to potentially overestimated BSI costs in previous studies. 51,52 On the other hand, no study has taken into account the long-term costs associated with BSI in children.
Potential adverse effects of CVCs are rare. Heparin bonding could theoretically trigger an allergic response, leading to heparin-induced thrombocytopenia, although no case has been reported to the manufacturers. Antibiotic impregnation could potentially lead to antibiotic resistance, although a systematic review showed no increased risk of resistant organisms isolated from blood cultures. 1
Overview of aims and research questions
From a policy perspective, there could potentially be significant gains in terms of children’s health and health-care costs across the NHS if impregnated CVCs could be confirmed to substantially reduce rates of BSI. We compared both types of impregnated CVC previously shown to be most effective (antibiotic and heparin) with standard CVCs to determine the effectiveness of CVC impregnation in children. Secondary analyses were conducted to evaluate the effectiveness of each type of CVC.
We aimed to inform NHS policy regarding impregnated CVCs for intensive care of children by undertaking a large pragmatic RCT to determine (1) clinical effectiveness; (2) cost-effectiveness of impregnated compared with standard CVCs; and (3) the generalisability and cost impact of adopting impregnated CVCs for all children who need them.
The main objectives and data sources for the three parts of the study were:
-
Clinical effectiveness:
-
to determine the effectiveness of impregnated compared with standard CVCs for reducing BSI in children admitted to intensive care
-
to determine which type of CVC is most effective, based on three-way comparisons of measures of BSI, mortality and adverse events
-
data source: clinical outcomes captured on case report forms (CRFs) in the RCT.
-
-
Cost-effectiveness:
-
to determine the cost-effectiveness of impregnated compared with standard CVCs for reducing BSIs, based on incremental acute health-care costs per BSI avoided
-
data sources: clinical outcomes captured on CRFs in the RCT and records of health-care use captured by linkage of RCT data with hospital administrative data.
-
-
Generalisability and cost impact:
-
to estimate the net cost impact to NHS PICUs given a policy to adopt impregnated CVCs for all children who need them
-
data sources: national data on PICU admissions (Paediatric Intensive Care Audit Network; PICANet) linked with infection surveillance data collated by Public Health England (PHE) and costs from the economic evaluation.
-
The specific objectives, methods and results for each of the three phases of the study are reported in Chapters 2–5. We discuss the implications of our findings for policy and recommendations for future research in Chapter 6.
Chapter 2 Clinical effectiveness: methods
Trial design
We conducted a parallel, three-arm RCT. 53 Children admitted to 14 PICUs in England between December 2010 and November 2012 were randomised to CVCs impregnated with antibiotics or heparin or to standard CVCs in a ratio of 1 : 1 : 1.
Setting and participants
Children aged < 16 years were eligible if they were admitted to a participating PICU or were being prepared for PICU admission by an emergency retrieval team and were expected to require a CVC for ≥ 3 days. Children who had already participated in the trial were ineligible.
Interventions
We used polyurethane CVCs manufactured by Cook Medical Incorporated (Bloomington, IN, USA). Sizes used were French gauge 4 (double lumen), 5 or 7 (triple lumen). Both types of impregnation involve internal and external surfaces. Cook Medical Inc. reports a concentration of 503 μg/cm of minocycline and 480 µg/cm of rifampicin for their antibiotic-impregnated CVC, which reduces biofilm formation. 54 Heparin bonding reduces thrombus and thereby biofilm formation and uses benzalkonium chloride as an anti-infective bonding agent. 5,55
Randomisation and consent
For children admitted to the PICU following elective surgery, we sought prospective parental consent during preoperative assessment. Randomisation took place in theatre or in the anaesthetic room prior to entry into theatre. For children who required a CVC as an emergency, we sought parental consent after randomisation and stabilisation (deferred consent) to avoid delaying treatment, which was usually within 48 hours of randomisation. Children who required a CVC as part of their emergency care or resuscitation were randomised at the bedside in the PICU or at another hospital, where they were randomised by the PICU retrieval team prior to transfer to the PICU. Further details are given in the protocol [see www.nets.nihr.ac.uk/projects/hta/081347 (accessed 20 November 2015)].
At randomisation, the clinician or research nurse opened a pressure-sealed, sequentially numbered opaque envelope containing the CVC allocation. Randomisation sequences were computer generated by an independent statistician in random blocks of three and six, stratified by method of consent (deferred or prospective), site and envelope storage location within the site to facilitate easy access to envelopes (e.g. for insertion in theatre and in the PICU).
Parents consented to the use of their child’s data for the trial, to follow-up using routinely recorded clinical data and to 0.5 ml of blood being collected whenever a blood culture was clinically required. 56 Samples were sent for polymerase chain reaction (PCR) testing for 16S ribosomal ribonucleic acid (rRNA) of bacterial ribosome protein to detect bacterial infection.
We also sought consent to link data from PICANet57 to the child’s study data to categorise the primary reason for admission and the Paediatric Index of Mortality (PIM2)58 score on admission and to link to administrative hospital data for the economic analyses and death registration data from the Office for National Statistics (ONS) [see www.ons.gov.uk (accessed 4 January 2016)] to determine mortality after discharge from the PICU.
Blinding
Central venous catheter allocation was not blinded to the clinician responsible for inserting the CVC (because of the different colour strips for antibiotic and heparin CVCs) but, as the CVCs looked identical whilst in situ, allocation was concealed from patients, their parents and PICU personnel responsible for their care. Labels identifying the type of CVC were held securely in a locked drawer in case unblinding was required. Participant inclusion in analyses and occurrence of outcome events were established prior to release of the randomisation sequence for analysis.
Comparisons and outcomes
The primary analysis for the trial compared antibiotic or heparin CVCs with standard CVCs. Secondary analyses compared antibiotic with standard CVCs, heparin with standard CVCs and antibiotic with heparin CVCs.
The primary outcome was time to the first BSI based on blood cultures taken between 48 hours after randomisation and 48 hours after CVC removal (or prior to death). We used time to event analyses as the risk of BSI increases the longer a CVC is in place. This time interval was intended to capture BSIs related to the type of CVC. All blood culture samples were clinically indicated, defined by removal of the CVC because of suspected infection or other recorded evidence of infection (one or more of temperature instability, change in inotrope requirements, haemodynamic instability or poor perfusion). Any positive blood culture was accepted for a non-skin organism, but for skin organisms two or more positive cultures of the same organism were required within 48 hours of each other. A clinical committee reviewed all primary outcomes involving positive cultures.
We conducted a sensitivity analysis for potentially missing microbiology data by assuming that children with a record of clinical indication but no sample taken in the primary outcome time window did actually experience the primary outcome.
The main secondary outcomes were:
-
CR-BSI: based on the same organisms cultured from blood and the CVC tip between 48 hours after randomisation and 48 hours after CVC removal; or differential positivity of cultures from multiple CVC lumens on two or more occasions; or BSI and exit site infection or BSI and CVC removed for suspected infection
-
rate of BSI per 1000 CVC-days, based on one or more BSI between randomisation and CVC removal
-
time to a composite measure of BSI consisting of the primary outcome or a negative blood culture combined with (1) a positive 16S PCR result for bacterial rRNA; (2) removal of the CVC because of suspected infection; or (3) start of antibiotics or change in type of antibiotics on the same or next day.
Other secondary outcomes were:
-
time to CVC thrombosis (defined by two episodes within 5 days of each other of difficulty flushing the CVC or drawing back blood from the CVC, one episode of swollen limb, CVC removal because of thrombosis or a positive ultrasound indicating thrombosis)
-
time to CVC removal
-
mortality by 30 days
-
length of PICU admission
-
length of hospital stay (up to 6 months post randomisation)
-
type of bacteria or fungi isolated from the BSI included in the primary outcome.
CVC-related outcomes evaluated in the safety analyses were:
-
CVC-related adverse events (unexplained thrombocytopenia after insertion of the CVC, exit site infection, hypersensitivity, trauma from line insertion, line displacement, line breakage/mechanical problem/manufacture complication)
-
mortality recorded up until hospital discharge
-
antibiotic resistance to minocycline (> 0.5 µg/ml) or rifampicin (> 1.0 µg/ml).
Antibiotic resistance outcomes were based on Etest® strips [see www.biomerieux-diagnostics.com/etest (accessed 20 November 2015)] applied to organisms isolated from the BSI included in the primary outcome. Incomplete laboratory testing and reporting prevented analysis of resistance in cultures from the CVC tip (as specified in the protocol).
Sample size
We based the sample size calculation for the primary analysis on a RR. We assumed that detection of a RR of 0.5 in patients with a baseline risk of 10% would change policy. We assumed that the RR would remain relatively constant across baseline risks whereas the absolute risk difference would be more variable. A total of 1200 children were required in a 2 : 1 ratio (impregnated : standard) to achieve 80% power to detect a RR of 0.5 at a 5% level of significance, based on an estimated BSI rate of 10% and allowing for 5% loss to follow-up. A lower than expected BSI rate of 5% would have 62% power to detect a RR of 0.5 or 80% power for a RR of 0.32.
The Independent Data and Safety Monitoring Committee (IDSMC) recommended continuation of the study after (1) reviewing the first 209 children; (2) an interim analysis of 650 children using the Peto–Haybittle stopping rule for the primary outcome; and (3) recruitment had reached the original target of 1200 pre schedule in June 2012, before exhausting available funding (see Acknowledgements, Trial Oversight Committees and Table 23).
Statistical methods
Outcome data were analysed according to the intention-to-treat principle, meaning that children who were consented and randomised were analysed according to the type of CVC randomised, regardless of whether or not CVC insertion was attempted or the type of CVC received. Safety analyses included the subset of children for whom CVC insertion was attempted, grouped by CVC actually received.
The statistical analysis plan was developed prior to analysis and is available in Appendix 1. A 5% level of statistical significance and 95% confidence intervals (CIs) were used throughout. Absolute risk differences were calculated for proportions. Time-to-event outcomes were analysed using Kaplan–Meier curves and the log-rank test. Cox regression was used to adjust the primary analysis of time to BSI for the use of prospective or deferred consent and suspected infection at baseline. Poisson regression was used to analyse the secondary outcome of rate of BSIs (defined as the total number of BSIs per 1000 CVC-days occurring between randomisation and CVC removal). All analyses were conducted using SAS software version 9.3 (SAS Institute Inc., Cary, NC, USA).
Post hoc analyses evaluated competing risks from death or time to first BSI, using cumulative incidence curves. We applied Gray’s test to detect whether or not there was a difference between impregnated and standard CVCs for the primary outcome. 59 This analysis was conducted using R statistical software (The R Foundation for Statistical Computing, Vienna, Austria).
Study oversight and role of funders
The Research Ethics Committee for South West England approved the study protocol. The manufacturer Cook supplied CVCs to participating units at a 20% discounted price. Neither the manufacturer nor the funder (the National Institute for Health Research) had any role in the design of the study, the collection or interpretation of the data or the reporting of the results. The CATCH trial is registered with ClinicalTrials.gov (NCT01029717). The protocol is available at www.nets.nihr.ac.uk/projects/hta/081347 (accessed 20 November 2015) and the statistical analysis plan is provided in Appendix 1.
Chapter 3 Clinical effectiveness: results
Study population
In total, 1859 children were randomised, of whom 501 children were randomised prospectively and 1358 were randomised as an emergency. Of those randomised as an emergency, 984 subsequently provided deferred consent for inclusion in the analyses (Figure 1; see Appendix 2, Figures 11 and 12 for participant flow by emergency/elective randomisation). Reasons for non-consent in the deferred consent group included not approached [n = 180 (48%), mainly because of transfer to a non-participating unit or early discharge from the PICU], no response [n = 17 (4.5%)] or consent refused [n = 177 (47%)]. Detailed reasons for non-consent are reported elsewhere. 60 Numbers enrolled by site and by month are provided in Appendix 2 (see Table 24 and Figure 13).
FIGURE 1.
Consolidated Standards of Reporting Trials (CONSORT) flow diagram for all trial participants. a, Based on clinically indicated blood culture sample taken ≥ 48 hours after randomisation and < 48 hours after CVC removal. ITT, intention to treat.

Comparison of interventions
The intention-to-treat sample included 1485 children, of whom 1345 children received the allocated CVC. Threats to validity because of protocol deviations are provided in Appendix 2 (see Table 26). Very few children had a clinical indication but no blood culture taken in the primary outcome time window (see Figure 1). Timings of samples for positive BSIs included in the primary and secondary outcomes are provided in Table 1.
Randomisation | 48 hours after randomisation to CVC removal | 48 hours after CVC removal | |
---|---|---|---|
Primary outcome of BSI | |||
n = 40 | n = 2 | ||
Rate of BSI per 1000 CVC-days | |||
n = 10 | n = 40 | ||
CR-BSI | |||
n = 24 | n = 1 |
Baseline characteristics
Table 2 shows that baseline characteristics were similar between the randomised groups. Over half (58%) of children were aged < 12 months at admission, with one-third aged < 3 months. One-third of children had surgery prior to admission to the PICU and half of all children randomised had cardiovascular problems as their primary diagnosis at admission.
Variable | Standard | Antibiotic | Heparin | |||
---|---|---|---|---|---|---|
n a | % | n a | % | n a | % | |
Patient characteristics | 502 | 100 | 486 | 100 | 497 | 100 |
Emergency (deferred consent) | 333 | 66.3 | 320 | 65.8 | 331 | 66.6 |
Elective (prospective consent) | 169 | 33.7 | 166 | 34.2 | 166 | 33.4 |
Male | 285 | 56.8 | 291 | 59.9 | 277 | 55.7 |
Age | ||||||
< 3 months | 159 | 31.7 | 159 | 32.7 | 175 | 35.2 |
3–12 months | 129 | 25.7 | 123 | 25.3 | 116 | 23.3 |
1–10 years | 174 | 34.7 | 154 | 31.7 | 174 | 35.0 |
11+ years | 40 | 8.0 | 50 | 10.3 | 32 | 6.4 |
Weight at admission | ||||||
< 3 kg | 41 | 8.2 | 38 | 7.8 | 56 | 11.3 |
3–10 kg | 278 | 55.4 | 280 | 57.6 | 273 | 54.9 |
> 10 kg | 183 | 36.5 | 166 | 34.2 | 168 | 33.8 |
Missing | 0 | 0.0 | 2 | 0.4 | 0 | 0.0 |
Admitted for surgery | 174 | 34.7 | 171 | 35.2 | 181 | 36.4 |
PICU assessment (from linked PICANet data) | 479 | 95.4 | 456 | 93.8 | 473 | 95.2 |
Primary reason for admission | ||||||
Cardiovascular | 235 | 49.1 | 233 | 51.1 | 250 | 52.9 |
Endocrine/metabolic | 30 | 6.3 | 34 | 7.5 | 30 | 6.3 |
Infection | 39 | 8.1 | 30 | 6.6 | 31 | 6.6 |
Cancer | 9 | 1.9 | 6 | 1.3 | 8 | 1.7 |
Respiratory | 102 | 21.3 | 86 | 18.9 | 84 | 17.8 |
Neurological | 22 | 4.6 | 31 | 6.8 | 29 | 6.1 |
Trauma | 18 | 3.8 | 10 | 2.2 | 18 | 3.8 |
Other | 24 | 5.0 | 26 | 5.7 | 22 | 4.7 |
Unknown | 0 | 0.0 | 0 | 0.0 | 1 | 0.2 |
PIM2 | ||||||
< 1% | 54 | 11.3 | 48 | 10.5 | 48 | 10.1 |
1 to < 5% | 264 | 55.1 | 236 | 51.8 | 247 | 52.2 |
5 to < 15% | 116 | 24.2 | 123 | 27.0 | 119 | 25.2 |
15 to < 30% | 34 | 7.1 | 31 | 6.8 | 39 | 8.2 |
30%+ | 11 | 2.3 | 18 | 3.9 | 20 | 4.2 |
Clinical condition at randomisation | 502 | 100.0 | 486 | 100.0 | 497 | 100.0 |
< 72 hours before randomisation | ||||||
Other CVC in situ | 95 | 18.9 | 91 | 18.7 | 83 | 16.7 |
Anticoagulants received | 50 | 10.0 | 59 | 12.1 | 61 | 12.3 |
Antibiotics received | 286 | 57.0 | 276 | 56.8 | 284 | 57.1 |
Positive blood culture | 40 | 8.0 | 25 | 5.1 | 36 | 7.2 |
At randomisation | ||||||
Infection suspected | 214 | 42.6 | 181 | 37.2 | 199 | 40.0 |
Immunocompromised | 44 | 8.8 | 31 | 6.4 | 29 | 5.8 |
During follow-up
Table 3 provides details of the CVC insertion and characteristics at 48 hours post randomisation. CVC insertion took place in the operating room for 437 out of 493 (89%) in the prospective consent (elective) group, but in only 34 out of 917 (4%) of the deferred consent (emergency) group.
Variable | Standard | Antibiotic | Heparin | |||
---|---|---|---|---|---|---|
n a | % | n a | % | n a | % | |
CVC details (inserted CVCs) | 481 | 95.8 | 465 | 95.7 | 464 | 93.4 |
Deferred consent, CVC inserted | 314 | 65.3 | 301 | 64.7 | 302 | 65.1 |
Inserted at same hospital | ||||||
ICU | 276 | 57.4 | 264 | 56.8 | 259 | 55.8 |
Theatre | 5 | 1.0 | 4 | 0.9 | 7 | 1.5 |
Other | 2 | 0.4 | 3 | 0.6 | 1 | 0.2 |
Inserted at other hospitalb | ||||||
ICU | 5 | 1.0 | 6 | 1.3 | 3 | 0.6 |
Theatre | 3 | 0.6 | 8 | 1.7 | 7 | 1.5 |
Other | 23 | 4.8 | 16 | 3.4 | 23 | 5.0 |
Missing | 0 | 0.0 | 0 | 0.0 | 2 | 0.4 |
Prospective consent, CVC inserted | 167 | 34.7 | 164 | 35.3 | 162 | 34.9 |
Inserted at same hospital | ||||||
ICU | 15 | 3.1 | 23 | 4.9 | 16 | 3.4 |
Theatre | 152 | 31.6 | 141 | 30.3 | 144 | 31.0 |
Other | 0 | 0.0 | 0 | 0.0 | 1 | 0.2 |
Missing | 0 | 0.0 | 0 | 0.0 | 1 | 0.2 |
Size of line | ||||||
4 | 28 | 5.8 | 45 | 9.7 | 39 | 8.4 |
5 | 421 | 87.5 | 384 | 82.6 | 391 | 84.3 |
7 | 21 | 4.4 | 23 | 4.9 | 18 | 3.9 |
Missing | 11 | 2.3 | 13 | 2.8 | 16 | 3.4 |
Triple-lumen CVC | 450 | 93.6 | 421 | 90.5 | 422 | 90.9 |
CVC inserted into femoral vein | 253 | 52.6 | 217 | 46.7 | 235 | 50.6 |
48 hours post randomisation | 502 | 100.0 | 486 | 100.0 | 497 | 100.0 |
Number of devices in situ | ||||||
< 4 | 160 | 31.9 | 169 | 34.8 | 185 | 37.2 |
≥ 4 | 340 | 67.7 | 311 | 64.0 | 311 | 62.6 |
Missing | 2 | 0.4 | 6 | 1.2 | 1 | 0.2 |
Presence of an intrabody cavity devicec | ||||||
Yes | 404 | 80.5 | 381 | 78.4 | 380 | 76.5 |
No | 96 | 19.1 | 100 | 20.6 | 116 | 23.3 |
Missing | 2 | 0.4 | 5 | 1.0 | 1 | 0.2 |
Table 4 shows the number of arterial, peripheral and CVC samples taken by trial arm. Overall, 3583 blood samples were taken and 1216 out of 1485 (81.9%) of children had a sample taken. Sampling was similar by trial arm and site (see Appendix 2, Table 25).
Sample | Standard (n = 502) | Antibiotic (n = 486) | Heparin (n = 497) | |||
---|---|---|---|---|---|---|
n randomiseda/n samplesb | % | n randomiseda/n samplesb | % | n randomiseda/n samplesb | % | |
Samples clinically indicated and in the primary outcome time window | 213/328 | 42.4 | 190/269 | 39.1 | 190/326 | 38.2 |
Type of sample | ||||||
Arterial | 49/55 | 9.8 | 39/44 | 8.0 | 41/55 | 8.2 |
Peripheral | 19/22 | 3.8 | 32/33 | 6.6 | 35/39 | 7.0 |
CVC | 161/226 | 32.1 | 129/167 | 26.5 | 136/208 | 27.4 |
Primary outcome
The number of blood samples contributing to the primary outcome is shown in Appendix 2 (see Figure 14). Blood cultures were taken between 48 hours after randomisation and CVC removal for 40% of those randomised (593/1485; see Figure 1). BSI was recorded for 42 children [standard 18/502 (3.6%); antibiotic 7/486 (1.4%); heparin 17/497 (3.4%)]. Gram-positive organisms accounted for the majority of BSIs (Table 5).
Outcome | Standard (n = 502) | Antibiotic (n = 486) | Heparin (n = 497) | |||
---|---|---|---|---|---|---|
n a | % | n a | % | n a | % | |
BSI | 18 | 3.6 | 7 | 1.4 | 17 | 3.4 |
Median time to first BSI in days (IQR) | 7.5 | (4.5–11.2) | 6.9 | (6.0–8.0) | 4.2 | (3.1–8.4) |
Organism type | ||||||
Non-skin | 15b | 2.99 | 6 | 1.23 | 16 | 3.22 |
Skin | 3 | 0.60 | 1 | 0.21 | 1 | 0.20 |
Organism groupc | ||||||
Gram positived | 10 | 1.99 | 3 | 0.62 | 10 | 2.01 |
Gram negative | 6 | 1.20 | 4 | 0.82 | 5 | 1.01 |
Candida | 2 | 0.40 | 0 | 0.00 | 3 | 0.60 |
Figure 2 shows the Kaplan–Meier curve for the primary outcome of time to first BSI. There was no significant difference in time to first BSI when comparing any impregnated CVC (antibiotic or heparin) with standard CVCs (Table 6). However, the risk of BSI was significantly lower for antibiotic compared with standard CVCs [hazard ratio (HR) 0.43, 95% CI 0.20 to 0.96] and for antibiotic compared with heparin CVCs (HR 0.42, 95% CI 0.19 to 0.93). The direction of these results was robust to the sensitivity analysis (see Appendix 2, Table 27). Regression analysis showed no significant effect of prespecified variables (type of consent and suspected infection at randomisation) and the effect of type of CVC was similar after adjusting for these variables (Table 7).
Analysis | Comparison | Risk difference (95% CI) | HR (95% CI) | p-value |
---|---|---|---|---|
Primary | Any impregnated (n = 983) vs. standard (n = 502) CVC | –1.14 (–3.04 to 0.75) | 0.71 (0.37 to 1.34) | 0.29 |
Secondary | Antibiotic (n = 486) vs. standard (n = 502) CVC | –2.15 (–4.09 to –0.20) | 0.43 (0.20 to 0.96) | 0.04 |
Heparin (n = 497) vs. standard (n = 502) CVC | –0.17 (–2.45 to 2.12) | 1.04 (0.53 to 2.03) | 0.90 | |
Antibiotic (n = 486) vs. heparin (n = 497) CVC | –1.98 (–3.90 to –0.06) | 0.42 (0.19 to 0.93) | 0.03 |
Analysis | Variable (n with outcome) | Comparator (n with outcome) | HRa (95% CI) | p-value |
---|---|---|---|---|
Primary | Antibiotic or heparin CVC (24) | Standard CVC (18) | 0.71 (0.38 to 1.33) | 0.29 |
Deferred consentb (30) | Prospective consentb (12) | 0.87 (0.40 to 1.90) | 0.73 | |
Suspected infection(18) | No suspected infection (24) | 0.69 (0.33 to 1.42) | 0.31 | |
Secondary | Antibiotic CVC (7) | Standard CVC (18) | 0.40 (0.17 to 0.96) | 0.04 |
Heparin CVC (17) | Standard CVC (18) | 1.05 (0.54 to 2.05) | 0.89 | |
Deferred consentb (30) | Prospective consentb (12) | 0.87 (0.40 to 1.90) | 0.35 | |
Suspected infection (18) | No suspected infection (24) | 0.68 (0.33 to 1.40) | 0.30 | |
Secondary | Antibiotic CVC (7) | Heparin CVC (17) | 0.39 (0.16 to 0.95) | 0.04 |
Deferred consentb (30) | Prospective consentb (12) | 0.85 (0.30 to 2.45) | 0.76 | |
Suspected infection (18) | No suspected infection (24) | 0.99 (0.40 to 2.43) | 0.98 |
Competing risk analysis using Gray’s test indicated no difference between the treatments for either competing risk (p-values of p = 0.29 for BSI and p = 0.89 for death; Table 8).
Outcome | HR (95% CI) | Gray’s test p-value |
---|---|---|
Time to first BSI (hours) | 0.71 (0.39 to 1.31) | 0.29 |
Time to death (hours) | 1.08 (0.63 to 1.85) | 0.89 |
Secondary outcomes
No children had more than one BSI whilst the trial CVC was in situ. The relationship between BSI outcomes and time since randomisation is shown in Table 1.
Overall, 25 (1.7%) children experienced a CR-BSI (Table 9). There was no significant difference between any impregnated CVC and standard CVCs (p = 0.13), but the risk of CR-BSI was significantly lower for antibiotic than for standard CVCs (p = 0.03). There was no significant difference between antibiotic and heparin CVCs (p = 0.09) or between heparin and standard CVCs (p = 0.68).
Outcome | Standard (n = 502) | Antibiotic (n = 486) | Heparin (n = 497) | |||
---|---|---|---|---|---|---|
n a | % | n a | % | n a | % | |
CR-BSI | 12 | 2.4 | 3 | 0.6 | 10 | 2.0 |
Rate of BSI per 1000 CVC-days [number of BSIs/(number of days at risk/1000 days)] (95% CI) | 8.2 [21/2.547] | (4.7 to 11.8) | 3.3 [8/2.418] | (1.0 to 5.6) | 8.8 [21/2.391] | (5.0 to 12.6) |
Composite measure of BSI | 112 | 22.3 | 103 | 21.2 | 102 | 20.5 |
CVC thrombosis | 125 | 24.9 | 126 | 25.9 | 105 | 21.1 |
Median time to CVC removal in days (IQR) | 4.28 | (2.3–7.0) | 4.3 | (2.1–7.0) | 4.20 | (2.2–7.0) |
Mortality by 30 days | 42 | 8.4 | 39 | 8.0 | 28 | 5.6 |
Median time to PICU discharge in days (IQR) | 5.1 | (2.8–10.0) | 4.4 | (2.2–9.3) | 4.9 | (2.3–8.9) |
Median time to hospital discharge in days (IQR) | 12.0 | (6.4–25.6) | 12.0 | (6.7–22.7) | 12.1 | (6.4–22.5) |
The rate of BSI per 1000 CVC-days did not differ in the primary comparison between any impregnated and standard CVCs (see Table 9). However, the rate of BSI was significantly lower for antibiotic compared with standard (p = 0.04) and heparin (p = 0.03; Table 10) CVCs. There was no significant difference in the rate of BSI between heparin and standard CVCs (p = 0.85).
Outcome | Any impregnated vs. standard CVCs (primary analysis) | Antibiotic vs. standard CVCs (secondary analysis) | Heparin vs. standard CVCs (secondary analysis) | Antibiotic vs. heparin CVCs (secondary analysis) | ||||||||
---|---|---|---|---|---|---|---|---|---|---|---|---|
Risk difference (95% CI) | HRa (95% CI) | p-value | Risk difference (95% CI) | HRa (95% CI) | p-value | Risk difference (95% CI) | HRa (95% CI) | p-value | Risk difference (95% CI) | HRa (95% CI) | p-value | |
CR-BSI | –1.07 (–2.58 to 0.45) | 0.55b (0.25 to 1.21) | 0.13 | –1.77 (–3.28 to -0.27) | 0.25b (0.07 to 0.90) | 0.03 | –0.38 (–2.20 to 1.44) | 0.84b (0.36 to 1.96) | 0.68 | –1.39 (–2.81 to 0.02) | 0.30b (0.08 to 1.11) | 0.09 |
Rate of BSI per 1000 CVC-days | –2.21 (–6.36 to 1.94) | 0.73b (0.40 to 1.34) | 0.31 | –4.94 (–9.14 to -0.73) | 0.40b (0.17 to 0.97) | 0.04 | 0.55 (–4.60 to 5.70) | 1.07b (0.55 to 2.06) | 0.85 | –5.49 (–9.89 to –1.08) | 0.38b (0.16 to 0.89) | 0.03 |
Composite measure of BSI | –1.46 (–5.90 to 2.98) | 0.95 (0.75 to 1.20) | 0.65 | –1.12 (–6.26 to 4.03) | 0.94 (0.72 to 1.23) | 0.67 | –1.79 (–6.87 to 3.30) | 0.95 (0.73 to 1.25) | 0.73 | 0.67 (–4.41 to 5.75) | 0.99 (0.75 to 1.30) | 0.93 |
CVC thrombosis | –1.40 (–6.02 to 3.22) | 0.98 (0.79 to 1.22) | 0.88 | 1.03 (–4.40 to 6.46) | 1.09 (0.85 to 1.40) | 0.49 | –3.77 (–8.99 to 1.44) | 0.88 (0.68 to 1.14) | 0.34 | 4.80 (–0.50 to 10.10) | 1.24 (0.96 to 1.60) | 0.11 |
CVC removal | 1.04 (0.93 to 1.16) | 0.53 | 1.02 (0.90 to 1.17) | 0.67 | 1.05 (0.92 to 1.19) | 0.51 | 0.99 (0.87 to 1.13) | 0.87 | ||||
Mortality by 30 days | 0.80 (0.54 to 1.20) | 0.96 (0.61 to 1.51) | 0.65 (0.40 to 1.07) | 1.46 (0.88 to 2.42) | 0.14 | |||||||
Time to PICU discharge | 1.08 (0.97 to 1.20) | 0.17 | 1.07 (0.95 to 1.22) | 0.27 | 1.08 (0.96 to 1.23) | 0.21 | 0.98 (0.86 to 1.11) | 0.73 | ||||
Time to hospital discharge | 1.04 (0.93 to 1.16) | 0.47 | 1.03 (0.91 to 1.16) | 0.68 | 1.05 (0.93 to 1.19) | 0.42 | 0.98 (0.87 to 1.11) | 0.77 |
A change in antibiotics on the same day as a negative blood culture or the next day made the largest contribution to the composite measure of BSI (see Appendix 2, Table 28). Overall, 317 (21%) children experienced the composite measure of BSI and this outcome did not differ by CVC type (see Table 10).
There was no difference in any other secondary outcome by CVC allocation (see Table 10). The types of bacteria and fungi isolated from positive blood cultures are provided in Appendix 2 (see Table 29).
Safety analyses
More children in the cohort for the safety analyses were in the standard group (n = 533) than in the antibiotic (n = 451) or heparin (n = 479) groups. As standard CVCs were the default option in the majority of PICUs, more children received the allocated CVC in the standard arm (93%) than in the antibiotic (90%) or heparin (89%) arms.
No serious adverse events (e.g. intervention causing death or prolonging hospitalisation) were reported. CVC-related adverse events (i.e. unable to perform routine activity) were reported for 31 children (n = 21 mild, n = 8 moderate and n = 2 severe) (Table 11). No children had more than one adverse event and no events were attributed to the type of CVC.
Adverse event | Standard (n = 533) | Antibiotic (n = 451) | Heparin (n = 479) | Total (n = 1463) | ||||
---|---|---|---|---|---|---|---|---|
n a | % | n a | % | n a | % | n a | % | |
Unexplained thrombocytopenia | 0 | 0.0 | 1 | 0.2 | 1 | 0.2 | 2 | 0.1 |
Exit site infection | 1 | 0.2 | 0 | 0.0 | 0 | 0.0 | 1 | 0.1 |
Hypersensitivity | 0 | 0.0 | 0 | 0.0 | 0 | 0.0 | 0 | 0.0 |
Trauma from line insertion | 2 | 0.4 | 2 | 0.4 | 3 | 0.6 | 7 | 0.5 |
Line displacement | 4 | 0.8 | 6 | 1.3 | 3 | 0.6 | 13b | 0.9 |
Line breakage/mechanical problem/manufacture complication | 2 | 0.4 | 3 | 0.7 | 2 | 0.4 | 7b | 0.5 |
Unclassifiable | 0 | 0.0 | 1 | 0.2 | 0 | 0.0 | 1 | 0.1 |
Total | 9 | 1.7 | 13 | 2.9 | 9 | 1.9 | 31 | 2.1 |
Mortality | ||||||||
Deathsc | 66 | 12.4 | 44 | 9.8 | 38 | 7.9 | 148 | 10.1 |
Median time to death in days (IQR) | 15.3 | (6.0–39.0) | 9.0 | (2.6–25.6) | 14.8 | (5.3–32.6) |
Of the 1463 children whose CVC insertion was attempted, 148 (10%) died before discharge from the PICU (see Table 11). The majority of deaths were the result of related comorbidities at admission (see Appendix 2, Table 30).
Testing for antibiotic resistance varied by centre. Only 12 of the 42 children with the primary outcome had minocycline and rifampicin resistance reported using Etest strips; 8 out of 12 were resistant, in each case to both antibiotics (3/5 standard group; 2/2 antibiotic group; 3/5 heparin group). Resistant organisms by trial arm are provided in Appendix 2 (see Table 31).
Post hoc analyses
A total of 1573 valid PCR samples were taken from 715 (48%) of the children. Of these children, 11 (1.5%) had a positive PCR result based on any detectable deoxyribonucleic acid (DNA) (12 samples) (Table 12). Positive PCR results were observed for two (8.3%) children with the primary outcome compared with nine (1.3%) children without the primary outcome. Values of the positive PCR results are provided in Appendix 2 (see Table 32).
Group | Sample taken from child with primary outcome | n randomised | n (%) with PCR sample | n (%) with positive PCR result | |
---|---|---|---|---|---|
Standard | No | 484 | 239 (49.4) | 4 (1.7) | |
Yes | 18 | 12 (66.7) | 1 (8.3) | ||
Antibiotic | No | 479 | 221 (46.1) | 3 (1.4) | |
Yes | 7 | 5 (71.4) | 0 (0.0) | ||
Heparin | No | 480 | 231 (48.1) | 2 (0.9) | |
Yes | 17 | 7 (41.2) | 1 (14.3) | ||
Total | No | 1443 | 691 (47.9) | 9 (1.3) | |
Yes | 42 | 24 (57.1) | 2 (8.3) | ||
1485 | 715 (48.1) | 11 (1.5) |
Chapter 4 Cost-effectiveness analysis
Introduction
Central venous catheter infections are a substantial and preventable cause of iatrogenic morbidity, mortality, excess length of stay and health-care costs. In the setting of the PICU, BSIs related to CVCs have been reported to occur in 3–8% of all CVC insertions. As approximately two-thirds of the 16,000 admissions to English PICUs each year57 require CVCs, the overall impact represents a major burden to patients and the NHS. 20,21
Impregnated CVCs are nearly twice as expensive as standard CVCs, requiring decisions on their use to be informed by evidence of their cost-effectiveness. However, current economic evaluations are limited in their transferability to the PICU setting in the UK as they all relate to adult populations and, with one exception,29 apply to different health-care systems (Australia,61 Germany62 and the USA63–65). Although care pathways and costs may differ in the UK setting, these studies consistently demonstrated antibiotic-impregnated CVCs to be cost saving while yielding improved outcomes.
Hockenhull et al. 29 modelled the cost-effectiveness of impregnated CVCs compared with standard CVCs in adult patients. The cost of managing CR-BSIs, estimated as £9148, was taken from a systematic review of economic studies. Based on a systematic review of RCTs, impregnated CVCs were estimated to reduce the incidence of CR-BSIs from 3% to 1.4%. The incremental cost-effectiveness ratio (ICER) of £8530 saved for each CR-BSI averted was calculated as the additional cost of the impregnated CVC less the expected cost per patient of managing excess CR-BSIs divided by the absolute risk reduction. Although intuitively simple, the model did not consider mortality effects or discriminate between different types of impregnated CVCs and the authors recommended that decision-makers interpret the results with caution.
Halton et al. 61 used a Markov decision model to compare the cost-effectiveness of a range of antimicrobial-coated CVCs, including minocycline- and rifampicin-coated catheters, relative to uncoated catheters in adult intensive care unit patients. Simulations suggested that antibiotic CVCs prevented 15 CR-BSIs per 1000 CVCs placed, with a corresponding gain of 1.6 quality-adjusted life-years (QALYs). The model predicted that 32 intensive care unit (ICU) bed-days and 95 general ward bed-days would be released, with a cost saving of AU$130,289 per 1000 CVCs.
Frank et al. 62 performed a case–control analysis of resource use and costs among 30 adults who developed a CR-BSI and 108 control subjects, each in an ICU setting. The marginal cost per infectious episode was estimated as €231, but the calculation and meaning of the ICER presented for silver-impregnated CVCs were unclear.
Marciante et al. 63 developed a series of decision models with patient-level clinical trial data to determine whether or not minocycline- and rifampin-impregnated CVCs are cost-effective in adults. Cost-effectiveness was indeterminable for CVCs inserted for ≤ 1 week as no infections had occurred during this time. Antibiotic CVCs were modelled to be cost-effective for longer periods of insertion, with expected savings of US$67 and gains of 0.009 QALYs per patient.
Shorr et al. 64 presented another decision-analytic model based on a hypothetical cohort of 1000 adult patients requiring a CVC. The incidence of CR-BSIs, excess lengths of ICU and ward stays and associated costs were selected from published studies. Compared with standard CVCs, minocycline- and rifampin-impregnated CVCs were estimated to reduce the incidence of CR-BSIs from 3.3% to 1.4%, resulting in a saving of US$9605 for each CR-BSI averted.
Veenstra et al. 65 used data from RCTs, meta-analyses, and case–control studies within a decision-analytic modelling framework to estimate the incremental cost-effectiveness of antiseptic-impregnated CVCs in a hypothetical cohort of hospitalised patients at high risk for CR-BSIs. Modelling the use of chlorhexidine/silver sulfadiazine-impregnated compared with standard CVCs resulted in a 2.2% decrease in the incidence of CR-BSIs, a 0.33% decrease in the incidence of death and a saving of US$196 per CVC used.
An important limitation of these studies was that each analysis modelled the costs and consequences of BSIs using data from disparate sources and as such relied heavily on assumptions relating to attribution of hospital lengths of stay (the main cost driver) and mortality to BSIs. The only UK-based economic evaluation considered an adult population and assumed that a patient with a CR-BSI spends 6 additional days in the ICU and 5 additional days in a general medical ward. 29 A recent study of 1339 cases of CR-BSI sampled from a US paediatric population and matched to control subjects by propensity score revealed a higher mean attributable length of stay of 19 days. 66 Although this is comparable with the 21-day excess length of stay estimated for paediatric haematology/oncology patients,67 these estimates are reliant on retrospective observational data and are susceptible to bias.
Aim
We aimed to assess the cost-effectiveness of antibiotic, heparin and standard CVCs in an English PICU setting using data from the CATCH RCT. Although the primary comparison showed no evidence that impregnated CVCs (antibiotic or heparin) were more effective than standard CVCs, important differences in secondary comparisons among the three CVCs suggested that an economic evaluation was warranted to inform decisions on resource allocation. This would be especially relevant if one type of CVC were to reduce total costs, be associated with shorter periods in the PICU or reduce the length of ward stays.
Methods
Although cost–utility analyses, based on QALYs, are more appropriate for informing decisions concerning allocative efficiency, there are practical and methodological challenges in estimating utility values in children, especially very young children in the PICU setting. These include difficulties in responding to or understanding questions on health-related quality of life, whether for reasons of age, illness or consciousness; the limitations of using proxy utilities; the low event rate for the primary end point; and the inclusion of a wide range of clinical conditions. A cost-effectiveness analysis was therefore performed, which allowed for an assessment of technical efficiency (i.e. determination of the most efficient CVC for reducing the incidence of BSIs). The study methods were consistent with those used in other economic evaluations of CVCs. 62
Resource use
The perspective of the analysis was that of the NHS in England, with the expectation that the main cost driver of inpatient hospital care would represent the greatest proportion of direct medical costs. The principal cost components were PICU, high-dependency unit (HDU) and ward stays (including readmissions), outpatient clinic visits, accident and emergency (A&E) admissions and the CVCs. The time horizon of the base-case analysis was selected to include the costs associated with managing BSIs and any sequelae within the 6-month period from randomisation. Shorter time horizons were examined in sensitivity analyses.
The measurement of resource use required complementary approaches using data collected as part of the trial and as part of routine care. Patients’ use of hospital services was obtained from the following sources (Figure 3):
-
The trial CRFs. Research nurses completed the relevant sections of the CRF to record the dates during which patients were in neonatal intensive care units (NICUs) or PICUs, HDUs and paediatric wards within the hospitals participating in the CATCH trial. Data recorded on CRFs were used for the dates of hospital discharge, transfer to another hospital and CVC removal.
-
Hospital Episode Statistics (HES) data from the Health and Social Care Information Centre. 68 HES data contain details of all admissions to NHS hospitals in England and provide Healthcare Resource Groups (HRGs) for the type of care patients receive at a ward level, outpatient visits and A&E admissions, but do not provide details on ICU and HDU stays. HES data were used for estimating HRGs for ward stays, outpatient and A&E attendances.
-
The PICANet data set. 57 This data set includes all ICU length of stays for paediatric patients in the UK and allows for the tracking over time of patients who have been transferred between ICUs in different hospitals. PICANet data were used for the national schedule of reference costs HRGs for HDU and ICU stays69 and for checking hospital admission, transfer and discharge dates.
-
Hospital Patient Administration Systems (PASs) of CATCH-participating hospitals. These were accessed for patient lengths of stay on ICUs and wards and for relevant HRGs. These were used to supplement data that were missing from other sources.
FIGURE 3.
Flow diagram of the methods employed for the economic evaluation.

Unit costs
Healthcare Resource Groups were chosen as the main currency of the economic analysis as these most closely reflect payments relating to patient stays. Cost codes based on the 2012–13 national tariff were applied to ward, outpatient and A&E codes. 70 These are bundled care packages, that is, they are reimbursed at a national level according to the NHS Payment by Results scheme71 (see Appendix 3, Table 33). The 2012–13 national schedule of reference costs69 was applied to PICU, NICU and HDU codes. These are unbundled care packages as they are locally reimbursed services (Table 13). Obsolete national tariff and schedule codes and hospital bed-day rates used between 2010 and 2012 were inflated using the Consumer Price Index (4.3% for 2010–11 and 2.7% for 2011–12). The preferred Hospital Price Index was available only for 2010–11, but was similar to the Consumer Price Index at 4.1%. The list prices of CVC devices were obtained from the supplier (Cook Medical Inc.).
HRG code | HRG name | Primary description | Secondary description | Cost per day (£) |
---|---|---|---|---|
XB01Z | Paediatric Critical Care, Intensive Care, ECMO/ECLS | Highly specialised intensive care treatment, e.g. by ECMO | ECMO, ventricular assist devices and other highly complex procedures | 4391 |
XB02Z | Paediatric Critical Care, Intensive Care, Advanced Enhanced | Unstable multisystem failure with other complications | 2409 | |
XB03Z | Paediatric Critical Care, Intensive Care, Advanced | Intensive nursing supervision at all times, undergoing complex monitoring and/or therapeutic procedures and including advanced respiratory support | Invasive ventilation with multisystem failure | 2017 |
XB04Z | Paediatric Critical Care, Intensive Care, Basic Enhanced | Intensive ventilation with more than one system failure | 2110 | |
XB05Z | Paediatric Critical Care, Intensive Care, Basic | Continuous nursing supervision | Invasive ventilation with single system failure or non-invasive ventilation with more than one system failure | 1743 |
XB06Z | Paediatric Critical Care, High Dependency, Advanced | Requiring closer observation and monitoring than is usually available on an ordinary children’s ward, with higher than usual staffing levels | Non-invasive ventilation (e.g. CPAP and BIPAP by mask with intravenous drugs) | 1335 |
XB07Z | Paediatric Critical Care, High Dependency | Close monitoring, oxygen by mask, no invasive ventilation | 886 | |
XB08Z | Paediatric Critical Care, Transportation | As paediatric critical care facilities are centralised in a small number of hospitals providing expert specialist care, specialist transport teams are required to deliver clinical management during transfer of patients | 2799 | |
XA01Z | Neonatal Critical Care, Intensive Care | Care provided for babies who are the most unwell or unstable and have the greatest needs in relation to staff skills and staff to patient ratios | Baby receives any form of mechanical respiratory support via a tracheal tube and/or parenteral nutrition | 1118 |
Cost analysis
Bundled national tariff costs were based on the hospital spell and incorporated excess ward-days, a market forces factor and whether the case was elective or emergency. Tariff codes were obtained primarily from HES data (see Appendix 3) or, when unavailable, PAS data. If bundled HRGs were missing from both of these sources, ward costs were assigned from the ward bed-day rates supplied by hospital finance departments (Table 14). Similarly, bed-day rates were applied to stays with unassignable national tariff HRG codes (such as UZ01C and WA14Z) appearing in the HES and PAS data. These bed-day rates were needed for < 1% of admissions.
Hospital | HES hospital ID | Market forces factora | Ward rate (£)b |
---|---|---|---|
Birmingham Children’s Hospital | RQ3 | 1.05 | 290 |
Bristol Royal Hospital for Children | RA7 | 1.08 | 366 |
Evelina London Children’s Hospital (Guy’s and St Thomas’) | RJ1 | 1.28 | 595c |
Freeman Hospital | RTD | 1.04 | 595c |
Alder Hey Children’s Hospital | RBS | 1.04 | 364d |
Glenfield Hospital | RWE | 1.04 | 751 |
Great Ormond Street Hospital | RP4 | 1.29 | 2157 |
Leeds General Infirmary | RR8 | 1.05 | 542 |
Leicester Royal Infirmary | RWE | 1.04 | 751 |
Queen’s Medical Centre | RX1 | 1.04 | 374 |
Royal Brompton Hospital | RT3 | 1.25 | 370 |
Royal Victoria Infirmary | RTD | 1.25 | 342 |
Southampton General Hospital | RHM | 1.09 | 212 |
St Mary’s Hospital, London | RYJ | 1.24 | 394 |
Unbundled, locally reimbursed costs were calculated from the national schedule ‘per day’ codes taken from PICANet (see Table 13) or were assigned as XA01C in the cases in which neonatal critical care was indicated in CRF data. In the 10% of cases in which unbundled codes were missing, CRF data were consulted to determine whether the patient stay was in a PICU or a HDU. In addition, PICANet database entries (such as patient note summaries) were examined for any evidence of advanced and/or enhanced care. In the absence of any higher cost code indicators, a basic HDU code (XB07Z) or a basic ICU code (XB05Z) was applied from the national schedule of reference costs. 69
Baseline costs, relating to the 6 months preceding randomisation, were calculated from HES and PICANet data on ward, PICU and HDU costs.
For the 6 months subsequent to randomisation, an adjustment was necessary to apportion costs given that ward, PICU and HDU costs related to episodes of care could start prior to randomisation. Patients admitted to hospital n days before randomisation and spending N days in hospital after randomisation had their total costs calculated as:
Patients’ use of health-care resources and total costs were calculated for the intention-to-treat population, with summary statistics generated by intervention group.
Outcomes
The clinical outcome for the cost-effectiveness analysis was the presence of a first BSI defined by a positive blood culture from a sample that was clinically indicated and taken more than 48 hours after CVC insertion and up to 48 hours after CVC removal. The likelihood of a BSI was estimated using a logistic regression analysis with intervention group as the explanatory variable.
Incremental analysis
The cost-effectiveness of each CVC was evaluated by (1) ranking CVCs according to decreasing effectiveness and (2) eliminating dominated interventions (those that were less effective or ineffective) or any extendedly dominated interventions. The ICER for the remaining CVCs was consequently calculated according to the following equation:
where Δcosts is the difference in mean total costs between interventions and ΔBSIs is the difference in the risk of BSIs between interventions.
Uncertainty analysis
Non-parametric bootstrapping (10,000 replicates) was used to calculate bias-adjusted 95% central ranges for differences in costs and BSIs and their joint distributions. Uncertainty was represented using a cost-effectiveness acceptability curve (CEAC), which presented the probability of CVCs being cost-effective for given ceiling thresholds of costs per BSI averted. 72
Uncertainty in total costs was further explored by adjusting for the contribution of independent baseline factors to overall variability. 73
The following predefined explanatory variables were tested for independent associations with total costs: age group, body weight, 6-month pre-randomisation costs (all log-transformed), gender, pre-existing CVC 72 hours prior to randomisation, health status before PICU admission, reason for admission (cardiovascular, endocrine or metabolic, infection, neurological, oncology, respiratory, trauma, other), suspected infection at randomisation, immunocompromised, positive blood culture within 72 hours prior to randomisation, numbers of devices in situ, intervention group and admission type (elective or emergency). Assumptions were necessary to account for missing data with respect to some variables: patients were assumed to be healthy (n = 1), not immunocompromised (n = 19) and to have no positive blood cultures (n = 5). Missing data for weight (n = 2) were imputed with the mean participant weight (11.95 kg). Missing reasons for admission (n = 20) were cross-checked against PICANet, PAS and available HES data. All were correctly assigned as cardiovascular patients.
Independent variables were tested in univariate analyses for their association with total costs, with risk factors that were significant at the 5% level selected for the multivariable regression using a stepwise approach. Given the non-normality of the cost data, generalised linear models were specified using a range of families and links. Assessment of goodness of fit using the Akaike information criterion (AIC) and the modified Park test was inconclusive, but the best-fitting link function, determined from the Pearson correlation, Pregibon link and modified Hosmer and Lemeshow tests, was the identity link. Although the underlying true distributions of costs are not normal, the analysis depends only on sample means and variances. Based on the comparatively large sample size, the central limit theorem was assumed to guarantee near normality of sample means and an ordinary least squares regression was considered appropriate. 73
Bias-corrected CIs for costs and BSIs were estimated from bootstrapped data generated using the recycled predictions method. 74
Sensitivity analysis
The prespecified time horizon of 6 months in the base-case analysis was selected to capture longer-term costs resulting from potential complications of BSIs but was somewhat arbitrary. The sensitivity of total costs and the ICERs to the time horizon of analysis was therefore considered by limiting costs to those incurred during the index hospitalisation (i.e. excluding any subsequent readmissions that may have occurred during the 6 months) and by analysing their relationship with time, from 1 month (when all BSIs had occurred) to 6 months.
Value of health-care resources associated with bloodstream infection
In an exploratory analysis, a variable representing the presence of a BSI was included in the cost regression to estimate the value of the health-care resources associated with managing a BSI. To avoid collinearity, the variable representing intervention group was omitted from this regression.
All analyses were performed using Stata version 10 (StataCorp LP, College Station, TX, USA) and the economic evaluation was reported according to the Consolidated Health Economic Evaluation Reporting Standards (CHEERS) statement. 75
Results
Resource use and total costs
Complete cost data were available for all patients. In the 6 months preceding randomisation, the mean costs (length of stay) of ICU/HDU admissions were £6026 (3.19 days) for the standard CVC group, £5188 (2.76 days) for the antibiotic CVC group and £6616 (3.47 days) for the heparin CVC group. The mean total hospital costs for the corresponding period were £15,588, £16,933 and £16,722, respectively. Neither ICU/HDU costs nor total hospital costs differed by intervention group.
Patients randomised to antibiotic-impregnated CVCs spent a mean of 10.8 days (95% CI 9.3 to 12.5) in the PICU in the 6 months following randomisation compared with 9.9 days (95% CI 8.6 to 11.4) for those in the heparin-bonded CVC group and 10.5 days (95% CI 9.2 to 11.9) for those in the standard CVC group (Table 15). There were no significant differences between groups in length of stay in the PICU (p = 0.70), HDU (p = 0.43) or ward (p = 0.52). The mean total hospital stay in the 6 months after randomisation was 34.8 days (95% CI 31.2 to 38.5 days) for antibiotic CVCs, 31.4 days (95% CI 28.2 to 34.7 days) for heparin-bonded CVCs and 31.7 (95% CI 28.8 to 34.8 days) for standard CVCs. The six most significant HRGs (of 349 in total) accounted for 50% of ward costs. These related to surgical correction of congenital malformations, cardiac surgery or disorders of the lower respiratory tract.
Unit | Antibiotic | Heparin | Standard | |||
---|---|---|---|---|---|---|
Mean (median) | 95% CI | Mean (median) | 95% CI | Mean (median) | 95% CI | |
Days on ICU | 10.79 (5.00) | 9.28 to 12.48 | 9.91 (5.00) | 8.57 to 11.44 | 10.50 (5.00) | 9.17 to 11.93 |
Paediatric Critical Care, Intensive Care, ECMO/ECLS (XB01Z) | 0.31 (0.00) | 0.07 to 0.72 | 0.39 (0.00) | 0.09 to 0.80 | 0.41 (0.00) | 0.17 to 0.72 |
Paediatric Critical Care, Intensive Care, Advanced Enhanced (XB02Z) | 0.16 (0.00) | 0.09 to 0.26 | 0.12 (0.00) | 0.09 to 0.15 | 0.16 (0.00) | 0.10 to 0.26 |
Paediatric Critical Care, Intensive Care, Advanced (XB03Z) | 0.77 (0.00) | 0.51 to 1.05 | 0.62 (0.00) | 0.43 to 0.83 | 0.65 (0.00) | 0.46 to 0.87 |
Paediatric Critical Care, Intensive Care, Basic Enhanced (XB04Z) | 2.30 (0.49) | 1.92 to 2.72 | 2.69 (0.78) | 2.09 to 3.44 | 2.76 (0.00) | 2.14 to 3.54 |
Paediatric Critical Care, Intensive Care, Basic (XB05Z) | 6.96 (2.00) | 5.65 to 8.45 | 5.63 (2.00) | 4.75 to 6.59 | 6.40 (2.95) | 5.42 to 7.47 |
Neonatal Critical Care, Intensive Care (XA01C) | 0.29 (0.00) | 0.10 to 0.55 | 0.46 (0.00) | 0.13 to 1.03 | 0.11 (0.00) | 0.04 to 0.20 |
Days on HDU | 2.00 (0.59) | 1.48 to 2.62 | 1.60 (0.59) | 1.28 to 1.99 | 1.73 (0.00) | 1.44 to 2.05 |
Paediatric Critical Care, High Dependency, Advanced (XB06Z) | 1.28 (0.00) | 0.94 to 1.70 | 1.09 (0.00) | 0.80 to 1.45 | 1.22 (0.00) | 0.98 to 1.49 |
Paediatric Critical Care, High Dependency (XB07Z) | 0.72 (0.00) | 0.42 to 1.16 | 0.51 (0.00) | 0.40 to 0.64 | 0.51 (0.00) | 0.40 to 0.64 |
Days on ward | 22.01 (9.13) | 19.26 to 24.80 | 19.85 (9.00) | 17.40 to 22.40 | 19.48 (8.57) | 17.12 to 21.94 |
Total days in hospital | 34.80 (20.00) | 31.21 to 38.48 | 31.36 (17.00) | 28.18 to 34.65 | 31.72 (17.97) | 28.75 to 34.81 |
Count of non-PICU/-HDU inpatient HRGs | ||||||
Complex Congenital Surgery (EA24Z) | 100 | 103 | 109 | |||
Intermediate Congenital Surgery (EA25Z) | 68 | 70 | 72 | |||
Major Complex Congenital Surgery (EA23Z) | 45 | 39 | 37 | |||
Cardiac Conditions with Complication and Comorbidity (PA23A) | 109 | 102 | 74 | |||
Lower Respiratory Tract Disorders without Acute Bronchiolitis with Length of Stay ≥ 1 day with Complication and Comorbidity (PA14C) | 95 | 78 | 105 | |||
Implantation of Prosthetic Heart or Ventricular Assist Device (EA43Z) | 2 | 2 | 4 | |||
Other inpatient HRGs | 1103 | 1055 | 964 |
Total and disaggregated costs are presented in Table 16. The mean 6-month cost was £44,503 (median £28,952, range £1786–360,983, 95% CI £40,619 to £48,666) for standard CVCs, £45,663 (median £29,793, range £2189–442,365, 95% CI £41,647 to £50,009) for antibiotic-impregnated CVCs and £42,065 (median £27,621, range £2638–382,431, 95% CI £38,322 to £46,110) for heparin CVCs (Figure 4). These costs were not statistically significantly different between intervention groups (p = 0.46) or when disaggregated according to bundled costs (p = 0.43) and unbundled costs (p = 0.73).
Unit | Antibiotic | Heparin | Standard | |||
---|---|---|---|---|---|---|
Mean (median) | 95% CI | Mean (median) | 95% CI | Mean (median) | 95% CI | |
Paediatric critical care, intensive care | ||||||
ECMO/ECLS (XB01Z) | 1358 (0) | 310 to 3159 | 1703 (0) | 386 to 3509 | 1796 (0) | 723 to 3156 |
Advanced Enhanced (XB02Z) | 388 (0) | 207 to 636 | 289 (0) | 216 to 371 | 395 (0) | 228 to 620 |
Advanced (XB03Z) | 1545 (0) | 1031 to 2124 | 1250 (0) | 872 to 1674 | 1318 (0) | 933 to 1752 |
Basic Enhanced (XB04Z) | 4861 (1023) | 4060 to 5738 | 5675 (1646) | 4418 to 7260 | 5822 (0) | 4512 to 7460 |
Basic (XB05Z) | 12,137 (3486) | 9855 to 14,730 | 9822 (3486) | 8274 to 11,489 | 11,159 (5133) | 9440 to 13,025 |
Neonatal Critical Care, Intensive Care (XA01C) | 325 (0) | 113 to 613 | 517 (0) | 142 to 1150 | 125 (0) | 42 to 225 |
Paediatric critical care, high dependency | ||||||
High Dependency, Advanced (XB06Z) | 1709 (0) | 1254 to 2271 | 1450 (0) | 1972 to 1940 | 1629 (0) | 1301 to 1992 |
High Dependency (XB07Z) | 635 (0) | 372 to 1025 | 454 (0) | 354 to 567 | 456 (0) | 356 to 566 |
Transportation (XB08Z) | 1158 (0) | 1022 to 1293 | 1258 (0) | 1109 to 1413 | 1208 (0) | 1068 to 1353 |
Subtotal (PICU/HDU/NICU)a | 24,115 (12,201) | 20,824 to 27,764 | 22,417 (11,903) | 19,429 to 25,771 | 23,907 (12,495) | 20,989 to 27,049 |
Inpatient stayb | ||||||
Complex Congenital Surgery (EA24Z) | 3011 (0) | 2445 to 3593 | 2908 (0) | 2363 to 3481 | 3144 (0) | 2565 to 3753 |
Intermediate Congenital Surgery (EA25Z) | 2166 (0) | 1670 to 2699 | 1934 (0) | 1470 to 2440 | 2044 (0) | 1583 to 2545 |
Major Complex Congenital Surgery (EA23Z) | 1865 (0) | 1315 to 2481 | 1915 (0) | 1310 to 2603 | 1466 (0) | 1013 to 1960 |
Cardiac Conditions with Complication and Comorbidity (PA23A) | 1277 (0) | 818 to 1845 | 1173 (0) | 831 to 1558 | 739 (0) | 495 to 1025 |
Lower Respiratory Tract Disorders without Acute Bronchiolitis with Length of Stay ≥ 1 day with Complication and Comorbidity (PA14C) | 858 (0) | 593 to 1157 | 668 (0) | 454 to 913 | 943 (0) | 657 to 1268 |
Implantation of Prosthetic Heart or Ventricular Assist Device (EA43Z) | 273 (0) | 0 to 684 | 298 (0) | 0 to 762 | 548 (0) | 103 to 1155 |
Other inpatient HRG costs | 10,316 (4017) | 8616 to 12,231 | 8803 (3058) | 7524 to 10,106 | 9930 (3259) | 7860 to 12,409 |
Subtotal (inpatient) | 19,766 (14122) | 17,934 to 21,755 | 17,700 (13,716) | 16,308 to 19,182 | 18,814 (13,748) | 16,649 to 21,327 |
Other | ||||||
A&E costc | 89 (0) | 76 to 104 | 85 (0) | 73 to 99 | 91 (0) | 78 to 104 |
Outpatient costc | 1615 (883) | 1412 to 1838 | 1784 (837) | 1496 to 2109 | 1648 (881) | 1453 to 1871 |
CVC costd | 78.28 | 78 to 78 | 78.25 | 78 to 78 | 42.91 | 43 to 43 |
Total cost (full 6 months) | 45,663 (29,793) | 41,647 to 50,009 | 42,065 (27,621) | 38,322 to 46,110 | 44,503 (28,952) | 40,619 to 48,666 |
FIGURE 4.
Ranking of 6-month total costs by intervention group, indicating patients who experienced a BSI. (a) Antibiotic CVCs; (b) heparin CVCs; and (c) standard CVCs.


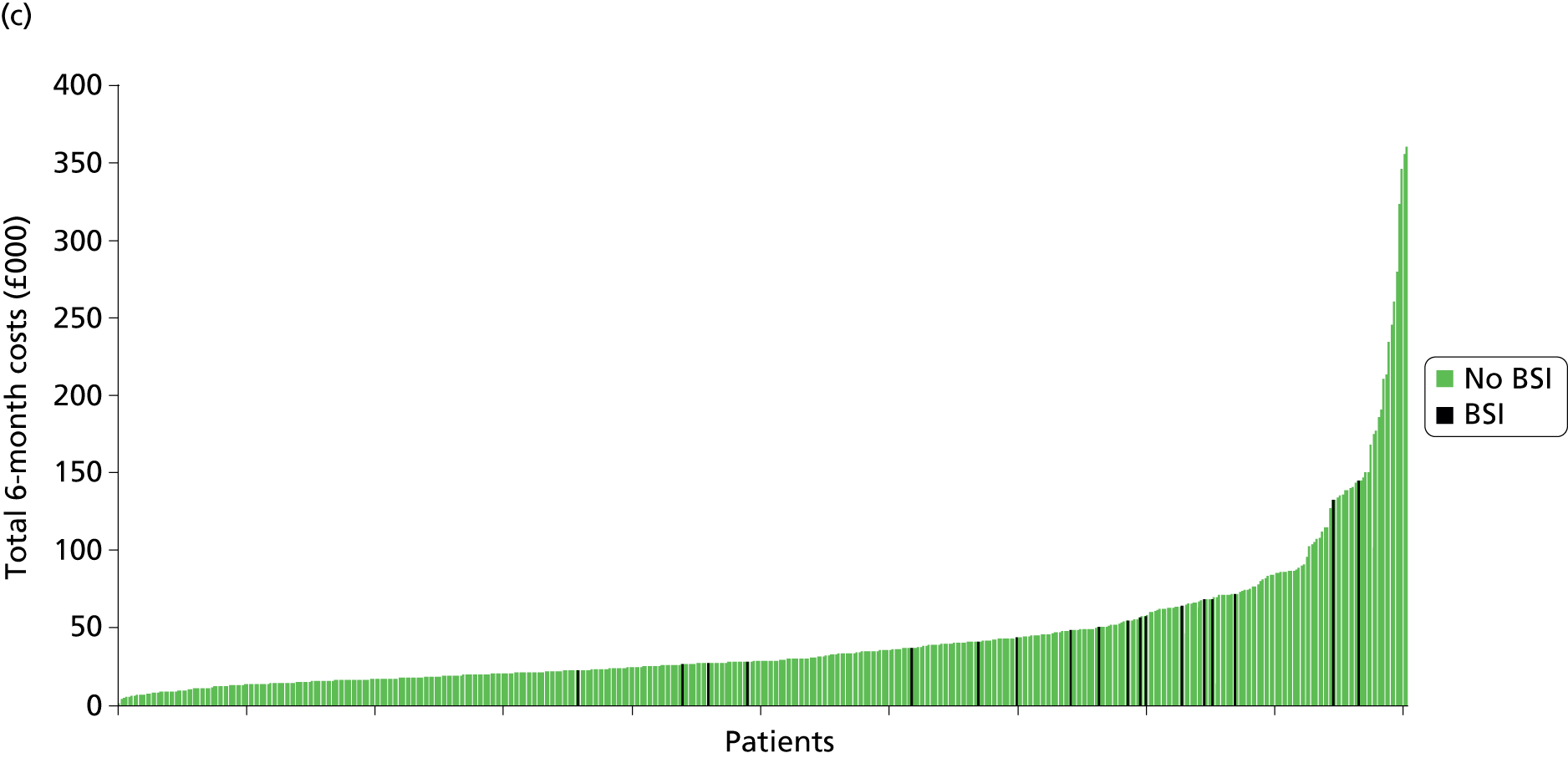
Incremental costs
Mean unadjusted costs over the 6-month time frame were not significantly different by CVC but tended to be higher (by £1160, 95% CI –£4743 to £6962) for antibiotic CVCs than for standard CVCs and lower (–£2439, 95% CI –£8164 to £3359) for heparin CVCs compared with standard CVCs.
Randomisation ensured that all variables tested for the cost regression were well balanced between intervention groups. Only a small proportion (< 10%) of the residual variability in total cost could be explained by the significant independent predictor variables: natural logarithm (ln) of age (in days), ln of 6-month pre-randomisation costs, health status before PICU admission, reason for admission, whether or not immunocompromised and admission type (elective or emergency; Table 17). The adjusted incremental costs associated with the antibiotic and heparin CVC groups in relation to the standard CVC group were £1220 (95% CI –£4332 to £6773) and –£2399 (95% CI –£7914 to £3120), respectively, resulting in small improvements in precision.
Variable | Coefficient (£) | 95% CI (£) | p-value |
---|---|---|---|
Ln of pre-randomisation cost | 1444 | 602 to 2287 | < 0.001 |
Admission type | 27,423 | 20,993 to 33,853 | < 0.001 |
Intervention group (antibiotic) | 1221 | –4332 to 6773 | 0.67 |
Intervention group (heparin) | –2399 | –7917 to 3120 | 0.39 |
Prior health status (0 = not healthy; 1 = healthy) | –9974 | –15,807 to –4140 | < 0.001 |
Reason for admission (endocrine/metabolic) | –1921 | –11,889 to 8048 | 0.71 |
Reason for admission (infection) | –22,300 | –32,609 to –11,992 | < 0.001 |
Reason for admission (neurological) | –21,854 | –32,780 to –10,927 | < 0.001 |
Reason for admission (oncology) | 2641 | –16,052 to 21,333 | 0.78 |
Reason for admission (other) | –3510 | –14,355 to 7335 | 0.53 |
Reason for admission (respiratory) | –8289 | –15,609 to –968 | 0.03 |
Reason for admission (trauma) | –12,144 | –26,764 to 2477 | 0.1 |
Compromised immunity (yes/no) | 8476 | –1246 to 18,198 | 0.09 |
Ln of age in days | –236 | –1300 to 828 | 0.66 |
Constant | 24,086 | 13,255 to 34,916 | < 0.001 |
Value of health-care resources associated with bloodstream infection
Over 6 month, patients who had experienced a BSI (n = 42) experienced 6.5 more days (95% CI 1.4 to 11.6 days) in the PICU than those with no BSI (n = 1443) and 15.1 additional total days (95% CI 4.0 to 26.2 days) of hospitalisation. The unadjusted mean 6-month cost for patients with a BSI was £60,481 (95% CI £47,873 to £73,809) and for patients without a BSI was £43,578 (95% CI £41,185 to £45,970), a difference of £17,263 (95% CI –£3076 to £31,450). The regression-derived adjusted difference in cost, representing the value of the resources used to manage BSI, was £10,975 (95% CI –£2801 to £24,751) (Table 18).
Variable | Coefficient (£) | 95% CI (£) | p-value |
---|---|---|---|
Ln of pre-randomisation cost | 1439 | 598 to 2281 | 0.001 |
Admission type | 27,341 | 20,916 to 33,767 | < 0.001 |
Prior health status (0 = not healthy; 1 = healthy) | –9593 | –15,440 to –3745 | 0.001 |
Reason for admission (endocrine/metabolic) | –2005 | –11,968 to 7959 | 0.693 |
Reason for admission (infection) | –22,585 | –32,896 to –12,274 | < 0.001 |
Reason for admission (neurological) | –21,648 | –32,559 to –10,736 | < 0.001 |
Reason for admission (oncology) | 2335 | –16,347 to 21,017 | 0.806 |
Reason for admission (other) | –2948 | –13,789 to 7894 | 0.594 |
Reason for admission (respiratory) | –8170 | –15,484 to –856 | 0.029 |
Reason for admission (trauma) | –12,412 | –27,016 to 2192 | 0.096 |
Compromised immunity (yes/no) | 7965 | –1770 to 17,700 | 0.109 |
Ln of age (in days) | –178 | –1243 to 885 | 0.742 |
BSI (0 = no; 1 = yes) | 10,975 | –2801 to 24,751 | 0.118 |
Constant | 23,064 | 12,759 to 33,369 | < 0.001 |
Outcomes
Seven of 486 children randomised to antibiotic CVCs experienced a BSI compared with 17 out of 497 in the heparin CVC group and 18 out of 502 in the standard CVC group. A statistically significant absolute risk difference was found only for antibiotic CVCs compared with standard CVCs (–2.15%, 95% CI –4.09% to –0.20%). Compared with standard CVCs, the unadjusted odds of acquiring a BSI with an antibiotic CVC was 0.39 (95% CI 0.16 to 0.95; p = 0.04) and with a heparin CVC was 0.95 (95% CI 0.49 to 1.87; p = 0.89).
Incremental and uncertainty analysis
As heparin CVCs were shown not to be clinically effective compared with standard CVCs there is no case for an incremental analysis: a clinically ineffective intervention cannot be cost-effective according to the same measure of BSI. The calculation of the ICER was therefore limited to the comparison between antibiotic and standard CVCs, which resulted in an ICER of £54,057 per BSI averted (Table 19).
Analysis | Antibiotic | Heparin | Standard |
---|---|---|---|
Base-case analysis (6-month time horizon) | |||
Total cost (£) | 45,663 (41,647 to 50,009) | 42,064 (38,322 to 46,110) | 44,503 (40,619 to 48,666) |
Incremental cost (vs. standard) (£) | 1160 (–4743 to 6692) | –2438 (–8164 to 3359) | – |
BSI (%) | 1.44 (0.4 to 2.5) | 3.42 (1.8 to 5.0) | 3.59 (2.0 to 5.2) |
Incremental BSI (vs. standard) (%) | –2.15 (–4.1 to –0.2) | –0.17 (–2.5 to 2.1) | – |
ICER (vs. standard) (£) | 54,057 per BSI averted | –a | – |
Sensitivity analysis (index hospitalisation) | |||
Total cost (£) | 33,073 (30,047 to 36,337) | 32,245 (29,013 to 35,823) | 35,165 (31,864 to 38,670) |
Incremental cost (vs. standard) (£) | –2093 (–6919 to 2583) | –2920 (–7833 to 2180) | – |
BSI (%) | 1.44 (0.4 to 2.5) | 3.42 (1.8 to 5.0) | 3.59 (2.0 to 5.2) |
Incremental BSI (vs. standard) (%) | –2.15 (–4.1 to –0.2) | –0.17 (–2.5 to 2.1) | – |
ICER (vs. standard) (£) | –97,543 per BSI avertedb | –a | – |
The CEAC yielded probabilities of 0.38, 0.49 and 0.62 of antibiotic CVCs being cost-effective at (arbitrary) thresholds of £10,000, £50,000 and £100,000 per BSI averted respectively (Figure 5). The probability of antibiotic CVCs dominating standard CVCs was estimated as 0.35.
FIGURE 5.
Cost-effectiveness acceptability curve based on a 6-month time horizon presenting the probabilities of antibiotic and standard CVCs being cost-effective for given values of ceiling ratio expressed as cost per BSI averted.

Sensitivity analysis
The mean number of days in hospital during the index hospitalisation was substantially shorter (e.g. 22.1 days for antibiotic CVCs) than the mean number of days in hospital during the 6 months from randomisation (e.g. 34.8 days for antibiotic CVCs; Table 20 and see Table 15). Considering only the index hospitalisation, total costs tended to be lower in the antibiotic CVC group (£33,073, 95% CI £30,047 to £36,337) and in the heparin CVC group (£32,245, 95% CI £29,013 to £35,823) than in the standard CVC group (£35,165, 95% CI £31,864 to £38,670). The unadjusted incremental cost saving for antibiotic compared with standard CVCs was –£2093 (95% CI –£6919 to £2583) and for heparin compared with standard CVCs was –£2920 (95% CI –£7833 to £2180).
Unit | Antibiotic | Heparin | Standard | |||
---|---|---|---|---|---|---|
Mean | 95% CI | Mean | 95% CI | Mean | 95% CI | |
Days on ICU | 9.31 | 8.09 to 10.70 | 8.93 | 7.71 to 10.32 | 9.79 | 8.60 to 11.03 |
Days on HDU | 1.70 | 1.25 to 2.25 | 1.39 | 1.09 to 1.76 | 1.51 | 1.24 to 1.80 |
Days on ward | 11.13 | 9.19 to 13.18 | 10.32 | 8.59 to 12.18 | 10.79 | 9.03 to 12.70 |
Total days in hospital | 22.14 | 19.48 to 24.89 | 20.65 | 18.27 to 23.16 | 22.09 | 19.76 to 24.51 |
Based only on the costs of the index stay, antibiotic CVCs dominated standard CVCs with a saving of £97,543 per BSI averted (see Table 19).
An analysis of the cumulative mean costs over the course of the 6 months (Figure 6) shows that costs in the heparin CVC group were lower overall, whereas costs in the antibiotic CVC group were variably cost incurring and cost saving in comparison to costs in the standard CVC group.
FIGURE 6.
Relation between total costs (cumulative) and time since randomisation according to intervention group.

The resulting ICER for antibiotic compared with standard CVCs fluctuated considerably (Figure 7), ranging from £82,204 saved per BSI averted by day 50 post randomisation to being cost neutral by day 122 and to the base-case cost of £54,057 per BSI averted by 6 months.
FIGURE 7.
Relation between the ICER for antibiotic CVCs compared with standard CVCs and time since randomisation. Positive ICERs are cost incurring and negative ICERs represent incremental savings per BSI averted.
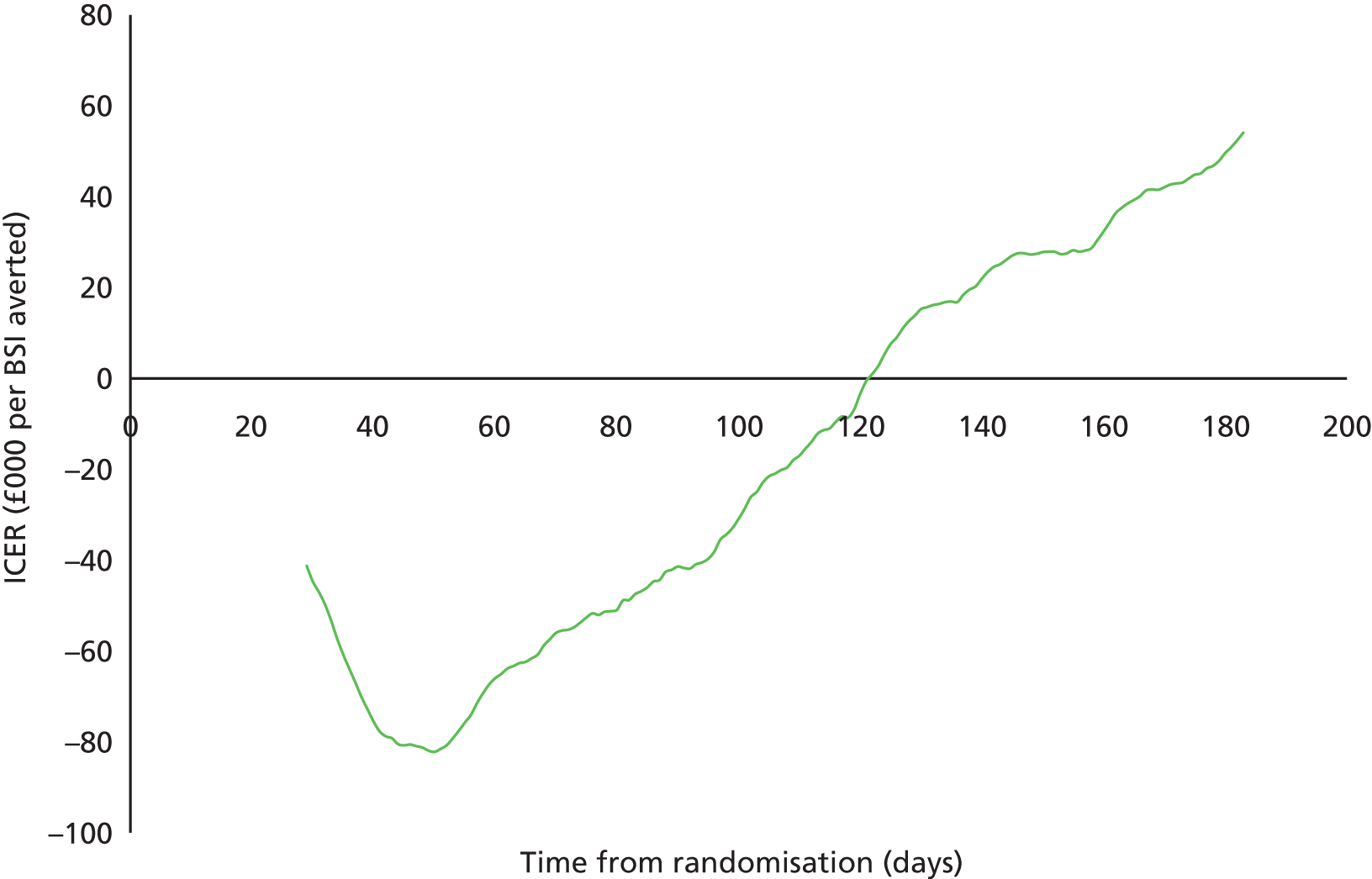
Chapter 5 Generalisability study
Introduction
The CATCH trial was the largest trial carried out in PICUs to date, recruiting 1485 children within 14 PICUs in 12 NHS trusts in England, corresponding to 5% of children admitted to all PICUs in England and Wales during the trial period (2010–12). However, if antibiotic-impregnated CVCs were to be adopted, it is likely that these CVCs would be bulk purchased and used for all children requiring CVCs in PICUs, not just children like those in the trial. Those making decisions on whether or not to purchase antibiotic-impregnated CVCs therefore need to take into account the generalisability of benefits to all children who need a CVC and the cost impact of purchasing the more expensive impregnated CVCs.
In terms of generalisability, trial populations may have different characteristics and outcomes from the characteristics and outcomes of those who receive the intervention in practice, for a variety of reasons. 76 In the CATCH trial there were two specific reasons why those recruited might differ from those likely to receive impregnated CVCs outside the trial setting. First, children recruited to the CATCH trial were expected to require a CVC for ≥ 3 days and would therefore have a higher risk of BSI than those staying for < 3 days. Second, the introduction of CVC care bundles and ongoing improvements in infection control in recent years have been associated with rapidly decreasing rates of BSI over the past decade, meaning that the background BSI rate may be lower now than it was at the start of the trial. 33,34
In terms of budget impact, impregnated CVCs are approximately twice as expensive as standard CVCs. However, the additional costs might be outweighed by the number of BSIs averted through using the more effective CVCs and the associated reduction in the use of health-care resources.
We determined the generalisability of the CATCH trial findings by estimating risk-adjusted trends in the rate of BSIs for children expected to require CVCs in PICUs, based on a data linkage study including children not participating in the CATCH trial. 77 We determined the budget and cost impacts of adopting antibiotic-impregnated CVCs for all children requiring a CVC in the PICU by synthesising the following evidence: (1) the estimated risk of BSI using standard CVCs (derived from the data linkage study); (2) the number of BSIs potentially averted by using antibiotic-impregnated CVCs (based on the relative treatment effect in the trial); (3) the additional costs associated with purchasing impregnated CVCs for all children expected to require a CVC (numbers of CVCs based on PICU survey data); and (4) the value of the health-care resources associated with each BSI (from the CATCH cost-effectiveness analysis).
Methods
Rate of bloodstream infections using standard central venous catheters
Data sources
There is no single data set from which the rate of BSIs in PICUs across the NHS can be estimated for children requiring standard CVCs. Linkage between the national laboratory surveillance system co-ordinated by PHE (LabBase2)78 and data from PICANet57 has provided an enhanced data set from which to estimate the baseline rate of BSIs.
Details of the data linkage study have been published elsewhere. 77 Briefly, a combination of deterministic linkage and a method called prior-informed imputation was used to identify PICANet admission records that had a corresponding record of a BSI in LabBase2. 79,80 A set of deterministic rules based on agreement between NHS number, hospital number, first name, surname, date of birth and postcode was used to identify unequivocal links. For the remaining records, match probabilities were calculated based on date of birth, Soundex code for surname, sex and location (laboratory and hospital). Match probabilities were used to inform imputation of values for uncertain links using prior-informed imputation. 79,80 Five imputed data sets were produced and analysed separately, with results combined using Rubin’s rules. 81
The resulting linked data set captured approximately 71% of all children aged < 16 years admitted to 20 of the 25 PICUs in England and Wales between March 2003 and December 2012 and is broadly representative of the whole PICU population. 82 As some PICUs used impregnated CVCs for some patients, we restricted the linked data set to children expected to require a standard CVC in a PICU in England. Types of CVCs used for emergency and elective admissions at each PICU were derived from responses to a PICU practice survey sent to a designated consultant at each PICU in 2009. 32 When no response was obtained or the PICU was not included in the survey, we assumed that standard CVCs were used.
Identifying children with central venous catheters
Central venous catheter use is not routinely captured for all admissions in PICANet, so we identified admissions likely to have included use of a CVC using a statistical model. We estimated the probability of CVC use for all admissions based on a subset of individual-level audit data in which CVC used was recorded. 82 Presence of a CVC was recorded for 2488 admissions as part of two audits: London’s Great Ormond Street Hospital (January 2006–December 2010) and Cambridge’s Addenbrooke’s Hospital (July 2009–December 2009). We used a multivariable logistic regression model to predict the probability of CVC use for all admissions, based on potentially predictive variables recorded in PICANet (e.g. use of vasoactive agents, length of stay and other clinical factors). The best-fitting predictive model was chosen based on the Bayesian information criterion (BIC).
The internal validity of the model was assessed using bootstrapping, accounting for any model overfitting from developing and testing the model in the same data set. 83–85 The external validity was assessed using aggregate data from a further two PICUs. We identified the subset of admissions most likely to have required a CVC using a probability cut-off based on the Youden Index. 86 Full details of the predictive model are provided in Appendix 4.
Estimated BSI rates were based on the subset of admissions identified by the predictive model as most likely to have received standard CVCs.
Case definition
We estimated CVC-days at risk of BSI by assuming that, for children expected to require a CVC, bed-days in the PICU were equivalent to CVC-days, that is, CVCs were inserted at admission and removed at discharge from the PICU. We defined an episode of BSI as any positive blood culture isolated from a blood sample taken from 2 days after admission to 2 days after discharge from the PICU. Repeated samples with positive cultures of the same organism within 14 days were treated as the same episode.
Statistical analysis
Rates of BSI per 1000 CVC-days were modelled using multilevel Poisson regression. We accounted for clustering of admissions within PICUs by including a random effect for PICU. The appropriateness of the Poisson model was verified using a goodness of fit test based on the deviance statistic. For comparisons between units and over time, rates were adjusted for risk factors identified as being significant (p < 0.05). Likelihood ratio tests were used to identify significant interactions between risk factors.
We compared BSI rates between CATCH participants using a standard CVC and non-participating admissions expected to require a standard CVC and non-participating admissions in the same PICUs but not expected to require a CVC. For non-participating PICUs, the trial period was defined as the period between December 2010 (when the first PICU began recruiting) and December 2012 (when the last PICU stopped recruiting).
Number of bloodstream infections averted using antibiotic central venous catheters
We estimated the difference in the number of BSIs if antibiotic CVCs were used in place of standard CVCs. We asked PICUs to provide the percentage of emergency and elective admissions receiving CVCs in a second PICU practice survey conducted in 2012 (not published but a repeat of the first survey32). The number of admissions requiring CVCs in all 23 PICUs in England was then estimated by applying these percentages to the number of emergency and elective admissions within each PICU. The total number of CVC-days was estimated by multiplying the number of CVCs required by the mean number of CVC-days for children expected to require a CVC in PICANet.
We estimated the BSI rate using antibiotic CVCs in place of standard CVCs by applying the relative treatment effect (rate ratio) from the trial to the BSI rate using standard CVCs.
We assumed that the relative treatment effect would be the same regardless of the baseline rate of BSIs, that is, the effect would be the same for children who would have been ineligible for the trial because they were expected to stay for < 3 days in the PICU. We reasoned that the biological mechanism through which impregnated CVCs work is the same for low- and high-risk patients (impregnated CVCs reduce the chance that bacteria track internally or externally along the CVC from the insertion site). RCTs of impregnated CVCs show similar results for long- and short-term CVCs, suggesting that the effect is not modified in groups with a different baseline risk or length of stay. 2 In reality, 72% of children recruited in the CATCH trial required a CVC for ≥ 3 days.
Budget impact: additional costs of antibiotic central venous catheters
Antibiotic CVCs are more expensive than standard CVCs: £73 compared with £42 for double-lumen CVCs and £79 compared with £43 for triple-lumen CVCs. The total additional costs of antibiotic CVCs were calculated by multiplying the number of CVCs required by the maximum additional cost per CVC, that is £36. We assumed, conservatively, that any change in PICU length of stay, nursing or other resources would not impact on hospital budgets. The budget impact was therefore based on the additional costs of antibiotic CVCs only.
Cost impact: value of resources associated with managing bloodstream infections
Assuming that any differences in costs between arms were the result of differences in the number of BSIs, the cost impact analysis utilised the estimated difference in the 6-month risk-adjusted costs between patients who had a BSI and those who did not (£10,975 per BSI, 95% CI –£2801 to £24,751) (cost-effectiveness analysis; see Table 18).
The total number of BSIs potentially averted was estimated by applying the BSI rate assuming that all children in 2012 had used either standard CVCs or antibiotic CVCs. The cost impact (total value of resources associated with managing BSIs with standard CVCs) was calculated by multiplying the costs per BSI by the estimated number of BSIs averted if antibiotic CVCs were used instead of standard CVCs.
Sensitivity analysis
We estimated the budget and cost impacts based on best- and worst-case scenarios for the total number of CVCs required and the excess number of BSIs for standard compared with antibiotic CVCs. We also performed probabilistic sensitivity analysis using Monte Carlo simulation to reflect uncertainty in both costs and number of BSIs. Values for each parameter were sampled from probability distributions based on observed data and 5000 iterations were performed to provide a 95% uncertainty interval for the cost impact. 87
Results
Rate of bloodstream infections using standard central venous catheters
Of the 2488 admissions in the CVC audit data, 1431 (58%) required a CVC. The best-fitting prediction model included length of stay, vasoactive agent, admission from ward, renal support and invasive ventilation (see Appendix 4, Table 37). With a probability cut-off of 0.57, the sensitivity of the predictive model for capturing admissions requiring a CVC was 61%, specificity was 82%, the positive predictive value was 82% and the negative predictive value was 61%. The predictive model identified 80% of the CATCH admissions as requiring a CVC.
Survey responses for the types of CVCs used prior to the CATCH trial were obtained for 18 of the 23 PICUs in England (see Appendix 4, Table 36). Only two PICUs reported not using standard CVCs for any admissions (both used heparin CVCs). BSI rates were estimated based on linked data from the remaining 16 English PICUs.
Applying the predictive model to the 16 PICUs in the linked data set identified a subset of 21,381 admissions most likely to have received a standard CVC between 2003 and 2012. The characteristics of these admissions (based on PICANet data) are provided in Appendix 4 (see Table 38). Risk-adjusted rates of BSI using standard CVCs decreased steadily between 2003 and 2012 and were greater for CATCH PICUs (5.27, 95% CI 5.06 to 5.49 per 1000 CVC-days in 2012) than for non-participating PICUs (2.09, 95% CI 1.60 to 2.58 per 1000 CVC-days in 2012) (Figure 8). Of the subset of admissions predicted to receive a CVC in 2012, 103 out of 3021 (3.4%) experienced a BSI, corresponding to an overall BSI rate using standard CVCs of 4.58 (95% CI 4.42 to 4.74) per 1000 CVC-days (Table 21). This was non-significantly lower than the rate observed during the trial (8.24, 95% CI 4.7 to 11.8 per 1000 CVC-days; see Table 9), partially because of the inclusion of all children with CVCs (not just those requiring CVCs for ≥ 3 days). Further explanations for this difference are the potentially incomplete reporting of BSIs to the national infection surveillance system, use of bed-days instead of CVC-days in the estimated rate and the increased frequency of sampling in trial PICUs during the CATCH trial.
FIGURE 8.
Risk-adjusted rates of BSIs for children expected to have a CVC based on linked PICANet–LabBase2 data for 16 PICUs in England. Symbols = observed rates; lines = smoothed adjusted rates (log scale).

Variable | Base case | Source | Sensitivity analysis |
---|---|---|---|
BSI rate using standard CVCs in 2012 per 1000 CVC-days | 4.58 (95% CI 4.42 to 4.74) | 3021 admissions in 15 PICUs – subset of admissions identified as most likely to have received a standard CVC by applying the predictive model to the linked data set. Admissions identified by survey responses as receiving non-standard (heparin or antibiotic) CVCs were excluded | Random sample taken with replacement from the linked data set for the number of admissions expected to require a CVC |
Rate ratio | 0.40 (95% CI 0.17 to 0.97) | Trial clinical effectiveness analyses (see Table 10) | Log-normal distribution with parameters (–0.913, 0.415) |
Estimated BSI rate using antibiotic CVCs in 2012 per 1000 CVC-days | 1.83; worst case 4.29, best case 0.81 | Rate ratio from the CATCH trial applied to the estimated BSI rate using standard CVCs for PICUs in England | Derived from (i) the BSI rate using standard CVCs and (ii) the rate ratio |
Number of admissions requiring CVCs in 2012 | 8831 | Average survey estimates for the percentage of emergency (60%) and elective (50%) admissions requiring CVCs, applied to all admissions in PICANet in 2012 (15,739 admissions in 23 PICUs) | Beta distributions with stated parameters: emergency: beta(60,40); elective: beta (50,50) |
Number of CVC-days in 2012 | 85,971 | Average bed-days per admission in the subset of admissions identified as most likely to have received a standard CVC by applying the predictive model to the linked data set, multiplied by the number of admissions requiring a CVC in 2012 | Random sample taken with replacement from the linked data set for admissions expected to require a CVC |
Number of BSIs averted in 2012 | 232 | BSI rate applied to CVC-days for admissions requiring a CVC in 2012 | Derived from (i) the number of admissions requiring a CVC in 2012 and (ii) the estimated BSI rate using antibiotic CVCs |
Additional cost of antibiotic CVCs | £36 | Difference in costs between standard (£43) and antibiotic (£79) CVCs (conservative case assuming triple-lumen CVCs used for all children) | Fixed at £36 |
Costs associated with managing each BSI | £10,975 (95% CI –£2801 to £24,751) | CATCH trial cost-effectiveness analysis (see Table 18) | Normal distributions with parameters (£10,975, £7,023) |
Number of bloodstream infections averted using antibiotic central venous catheters
Survey responses indicated that, on average, 60% of emergency admissions and 50% of elective admissions require a CVC (see Appendix 4, Table 36). The estimated number of children using CVCs in 2012 was 8831, corresponding to a total of 85,971 CVC-days (see Table 21). The rate ratio for BSIs for antibiotic compared with standard CVCs was estimated as 0.40 (95% CI 0.17 to 0.97; see Table 10) in the trial. The point estimate of the number of BSIs averted by switching from standard to antibiotic CVCs for all children requiring CVCs in 2012 was therefore 232, with best- and worst-case scenarios of 332 and 11 respectively (Table 22).
Scenario | BSI rate using standard CVCs | Rate ratio | BSI rate using antibiotic CVCs | BSI with standard CVCs | BSI with antibiotic CVCs | BSI averted cost–impact | ||
---|---|---|---|---|---|---|---|---|
Cost per BSI: –£2801 | Cost per BSI: £10,975 | Cost per BSI: £24,751 | ||||||
Base case | 4.58 | 0.40 | 1.83 | 385.9 | 154.4 | 231.6 | 231.6 | 231.6 |
–648,606 | 2,541,397 | 5,731,401 | ||||||
Worst case | 4.42 | 0.97 | 4.29 | 372.5 | 361.3 | 11.2 | 11.2 | 11.2 |
–31,297 | 122,631 | 276,559 | ||||||
Best case | 4.74 | 0.17 | 0.81 | 399.4 | 67.9 | 331.5 | 331.5 | 331.5 |
–928,583 | 3,638,415 | 8,205,414 | ||||||
1 | 0.40 | 0.40 | 3.4 | 1.3 | 2.0 | 2.0 | 2.0 | |
–5,645 | 22,119 | 49,884 | ||||||
2 | 0.40 | 0.80 | 6.7 | 2.7 | 4.0 | 4.0 | 4.0 | |
–11,290 | 44,238 | 99,767 | ||||||
3 | 0.40 | 1.20 | 10.1 | 4.0 | 6.0 | 6.0 | 6.0 | |
–16,936 | 66,358 | 149,651 | ||||||
4 | 0.40 | 1.60 | 13.4 | 5.4 | 8.1 | 8.1 | 8.1 | |
–22,581 | 88,477 | 199,534 | ||||||
5 | 0.40 | 2.00 | 16.8 | 6.7 | 10.1 | 10.1 | 10.1 | |
–28,226 | 110,596 | 249,418 | ||||||
6 | 0.40 | 2.40 | 20.2 | 8.1 | 12.1 | 12.1 | 12.1 | |
–33,871 | 132,715 | 299,301 | ||||||
7 | 0.40 | 2.80 | 23.5 | 9.4 | 14.1 | 14.1 | 14.1 | |
–39,516 | 154,834 | 349,185 | ||||||
8 | 0.40 | 3.20 | 26.9 | 10.7 | 16.1 | 16.1 | 16.1 | |
–45,161 | 176,954 | 399,069 |
Budget impact: additional costs of antibiotic central venous catheters
Based only on a CVC cost difference of £36, the additional cost of purchasing antibiotic CVCs for all children in 2012 was 8831 × £36 = £317,916.
Cost impact: value of additional costs associated with managing BSI
Based on each BSI being associated with a mean cost of £10,975 (95% CI –£2801 to £24,751; see Table 18), over 6 months the value of resources made available in 2012 through averting BSIs associated with standard CVCs (i.e. the total costs of managing these BSIs) would have been 232 × £10,975 = £2,541,397, with best- and worst-case scenarios of –£648,606 and £5,731,401 based on CIs for both estimates. The probabilistic sensitivity analysis provided a 95% uncertainty interval of –£66,544 to £5,557,451 for the total resources made available through averting BSIs in 2012. There was a probability of 0.90 that the value of resources made available would be more than the additional costs of purchasing antibiotic CVCs (Figure 9).
FIGURE 9.
Probability distribution for the value of resources made available by averting BSIs using antibiotic CVC in all PICUs in England during 2012. In total, 90% of the distribution represented costs greater than the additional cost of purchasing antibiotic CVCs.

The estimated cost impact for a typical PICU with 350 admissions per year is shown for a range of BSI rates in Table 22. Figure 10 shows that the cost of purchasing antibiotic CVCs for all children who require them will be less than the cost of managing BSIs with standard CVCs for PICUs with BSI rates > 1.2 per 1000 bed-days. This break-even value is substantially lower than the BSI rate observed in the standard arm of the trial (8.24, 95% CI 4.7 to11.8 per 1000 bed-days) or in the linked data set for PICUs in England (4.58, 95% CI 4.42 to 4.74 per 1000 bed-days).
FIGURE 10.
Cost impact: number of BSIs averted and value of resources made available using antibiotic in place of standard CVCs for a range of baseline BSI rates, assuming that each BSI is associated with a mean cost of £10,975.

Chapter 6 Discussion
Introduction
We aimed to inform NHS policy regarding impregnated CVCs for the intensive care of children. To address the question of whether or not impregnated CVCs should be adopted by PICUs in England and Wales, we undertook a large pragmatic RCT to determine the clinical effectiveness and cost-effectiveness of impregnated compared with standard CVCs. To determine the implications of adopting impregnated CVCs for all children who need them, we conducted a generalisability and cost impact study, using linked data from two national sources.
Clinical effectiveness
The primary analysis showed no evidence of a statistically significant difference in time to first BSI between any impregnated CVCs (antibiotic-impregnated and heparin-bonded CVCs combined) and standard CVCs. However, secondary analyses showed that antibiotic impregnation reduced the hazard of BSIs by 57% compared with standard CVCs and by 58% compared with heparin-bonded CVCs. Antibiotic-impregnated CVCs were associated with an absolute risk reduction of 2.15% compared with standard CVCs, meaning that 47 children would need to be treated with an antibiotic-impregnated CVC instead of a standard CVC to prevent one case of BSI.
Our choice of any BSI as a clinically important primary outcome and a recognised quality indicator is an important strength of our study, avoiding the biases inherent in measuring CR-BSI. 2,46,88,89 CR-BSI requires positive cultures from the blood and catheter tip and is highly susceptible to bias, as the tip can be easily contaminated during removal and residual antibiotic in the catheter tip may inhibit culture in the laboratory. 55,88
A further strength of the study is the restriction to positive blood cultures that were clinically indicated. This increased the clinical relevance of the primary outcome, but diminished the sensitivity of the study to detect bacteraemia, as only 40% of children had a blood culture taken in the relevant time window. A third strength is the representativeness of the study population in terms of children admitted to the 14 largest PICUs (of 23) across England. We were able to enrol a similar proportion of emergency patients (two-thirds) as seen in practice, enabled by the inclusion of retrieved children and the use of deferred consent. 90
A potential limitation of the study is that clinicians inserting the CVCs could not be blinded to allocation. However, we found no evidence of differential sampling by trial arm (see Figure 1). The number of children who received their allocated CVC was slightly higher for those in the standard arm, probably reflecting the fact that standard CVCs were the default CVC used in many units. 32 A second limitation is that, because of the lower than expected BSI rate in the standard arm of the trial, we had limited power to detect differences in the primary outcome comparing impregnated with standard CVCs. This choice of primary outcome was justified by the best available evidence to date – a systematic review and meta-analysis of direct and indirect comparisons of different types of impregnated and standard CVCs28 – which showed that heparin-bonded and antibiotic-impregnated CVCs resulted in similar reductions in the risk of CR-BSI (70–80%). A third limitation is that resistance testing was not standardised across sites. This reflects local laboratory administration and processing, which centralised testing of all positive cultures could have mitigated. When reported, resistance occurred in all trial arms, predominantly in Gram-negative isolates, as expected. The low rates are consistent with the previous lack of evidence for the emergence of resistance. 1
Few previous trials have reported the effectiveness of impregnated CVCs for any BSI. 45 However, the superiority of antibiotic-impregnated CVCs in children was consistent with the most recent systematic review reporting a pooled odds ratio for CR-BSI of 0.18 (95% CI 0.08 to 0.34). 28 Although our finding of a clinically important reduction in any BSI with antibiotic-impregnated CVCs (HR 0.25, 95% CI 0.07 to 0.09; p = 0.04) was based on a secondary comparison and should be viewed as exploratory, this result does add important evidence of the overall effectiveness of antibiotic-impregnated CVCs.
The finding that heparin CVCs were not effective for BSIs or CR-BSIs contradicts past evidence showing a pooled odds ratio for CR-BSI for heparin-bonded compared with standard CVCs of 0.20 (95% CI 0.06 to 0.44). 28 The difference in findings may reflect poor data quality in previous trials, highlighted by systematic reviews. Only one of the three trials comparing heparin with standard CVCs reported adequate concealment of randomisation, and this trial did not state whether or not clinicians were blinded to the intervention. 2 A further explanation for the discrepancy may be the low baseline event rate observed in the CATCH trial, which was conducted after implementation of CVC care bundles in PICUs to improve aseptic procedures during CVC insertion and maintenance. 32 It is conceivable that heparin CVCs are most effective in the context of high rates of surface colonisation, as they prevent thrombosis, which aids organism adherence to the CVC. Finally, the pairwise comparisons used to determine the most effective type of impregnation were not adequately powered to detect the anticipated small differences between antibiotic and heparin CVCs. However, our results suggest that antibiotic-impregnated CVCs can achieve further reductions in BSI rates, over and above that achieved by CVC care bundles. 33,34
Cost-effectiveness
The ICER of antibiotic-impregnated CVCs compared with standard CVCs was £54,057 per BSI averted over the 6 months after randomisation. Assuming that the health impact of a BSI is no greater (on average) than a reduction of 1 year of full health (i.e. 1 QALY), then, at the cost-effectiveness threshold of £30,000 per QALY, antibiotic CVCs may not represent a cost-effective alternative to standard CVCs in a PICU setting. However, there is considerable uncertainty surrounding this estimate, which is driven mainly by the time horizon of analysis.
The sensitivity analysis in which costs were restricted to the index hospital stay resulted in antibiotic CVCs dominating standard CVCs, with £97,543 saved for each BSI averted. Antibiotic CVCs therefore appear highly cost-effective when considering events and costs accruing over comparable periods.
A secondary analysis of the CATCH trial indicated that heparin CVCs were not clinically effective, with a risk difference for a first BSI of –0.17 (95% CI –2.45 to 2.12) compared with standard CVCs. It follows, therefore, that heparin CVCs cannot be cost-effective by the same measure. Theoretically, a cost-minimisation analysis might apply, to assess whether or not heparin CVCs are less costly overall than standard CVCs. However, heparin CVCs are more expensive than standard CVCs (in terms of unit prices) and, as the only difference among CVCs can be in BSI rates, any difference in total cost (which was not statistically significant) was caused by random variation. A cost-minimisation analysis might therefore lead to an erroneous conclusion that heparin CVCs are more cost-effective than standard CVCs.
Our economic evaluation benefits from being conducted alongside a pragmatic clinical trial, which is representative of current practice in the UK PICU setting. The evaluation utilises data from a definitive and unbiased comparison of impregnated and standard CVCs and was conducted robustly according to accepted methods of trial-based economic evaluations. 74 We used patient-level HES data to reflect the reimbursement costs for hospitals and multiple data sources to measure hospital resource use to ensure that cost data were complete.
However, there are limitations that affect the strength of our findings. First, the CATCH trial was not powered to determine cost differences between each of the three CVCs. As a consequence, the results are susceptible to random variation in costs between trial arms. Although hypothesis testing may be considered less relevant to decision making in the context of net benefits, the non-statistically significant differences in costs between groups translated to uncertainty in the joint distribution of costs and benefits such that, in the base-case analysis, antibiotic CVCs had a probability of 0.35 of dominating standard CVCs. 91 Mean total costs associated with heparin CVCs were lower than those for both antibiotic and standard CVCs, despite their ineffectiveness in avoiding BSIs compared with standard CVCs. Being a rare event, BSI costs were diluted compared with the overall costs relating to the intensive care of patients.
Second, the economic evaluation did not consider QALYs, which is the standard metric for informing decisions on resource allocation. This was because the estimation of utilities in paediatric ICU populations is empirically and conceptually challenging92,93 and because the main long-term consequence of BSIs, the impact on neurological outcomes, is poorly measured in children and was not measured in this trial. Short-term outcomes not considered in our economic analysis include mortality, antibiotic resistance and other adverse events. However, antibiotic resistance to minocycline or rifampicin did not differ by CVC allocation. There were no also no differences in 30-day mortality for antibiotic compared with standard CVCs (HR 0.96, 95% CI 0.61 to 1.51) or for heparin compared with standard CVCs (HR 0.65, 95% CI 0.40 to 1.07) and no differences in adverse events (see Table 11).
Assumptions regarding the time horizon of analysis represent a third limitation. The base-case, 6-month analysis was selected to include the costs of hospital readmissions in addition to the costs of the index hospitalisation and transfers that may have occurred subsequently. This was intended to capture the costs of managing any longer-term complications from BSIs, but, as the economic outcome was chosen to align with the primary clinical outcome, the health impacts of these complications were not included in the ICER. Consequently, as costs accrue over time with no corresponding change in the number of BSIs (these all occurred within 30 days), the ICER continued to increase over time.
Our findings are consistent with those of other studies in terms of the estimation of the costs associated with the management of BSIs. However, our ICER differs considerably and is inconclusive with regard to determining the cost-effectiveness of antibiotic CVCs. Published economic evaluations, including those that adopted a lifetime horizon of analysis, suggest dominance of antibiotic-impregnated CVCs over standard CVCs. One explanation for this discrepancy is in the methods of analysis. A decision-analytic model, based on a synthesis of data from various sources, is fundamentally different from a prospective RCT, in which differences between intervention groups are less evident, particularly in the context of rare events such as BSIs. In the evaluation by Hockenhull et al. ,29 for instance, the incremental cost saving of £138.20 per patient receiving an impregnated CVC was calculated as the additional cost of the antibiotic CVC less the expected cost per patient of managing excess BSIs. The equivalent calculation based on CATCH data for antibiotic CVCs results in a value of £200.08 saved for each antibiotic CVC used [(£78.28 – £42.91) – (£10,975 × 2.15%)]. Extending this further to calculate the ICER gives a value of £9326 saved per BSI averted (£200.08/2.15%), which differs appreciably from our base-case result [the differences between the ICER stated and the ICER calculated from the numbers in the text is due to rounding (difference in risk of BSI is 2.1453%)]. However, by analysing the data as a cohort study, separating the apparent costs of BSIs from the total costs relating to each intervention group, biases are likely to arise from assuming that the cost of managing BSIs is independent of CVC type.
In conclusion, the results of the cost-effectiveness analysis indicate that a policy of replacing standard CVCs with antibiotic-impregnated CVCs in paediatric ICUs would be more beneficial in terms of fewer patients developing BSIs. Given the low BSI rate, the variation in costs between arms and the sensitivity of analyses to the specified time horizon, there remains considerable uncertainty whether or not use of antibiotic CVCs represents a cost-effective use of NHS resources.
Generalisability and cost impact
We explored the generalisability of the CATCH trial results and the cost impact of changing practice in PICUs across England based on the trial results. In terms of generalisability, the observed rates of BSI using standard CVCs declined steadily over the past decade, including the period when children were enrolled into the CATCH trial. 34,94 In addition, children participating in the CATCH trial had a higher risk of BSI than all children receiving CVCs in practice, as they were expected to require a CVC for ≥ 3 days. This means that children currently receiving CVCs in PICUs are likely to have a lower BSI risk than those participating in the trial. This was reflected in the higher rate of BSIs observed in the standard arm of the trial (8.24 per 1000 bed-days) compared with linked administrative data from 16 PICUs in England for 2012 (4.58 per 1000 bed-days; see Figure 8).
In terms of budget impact, antibiotic CVCs are more expensive than standard CVCs. If adopted in PICUs, antibiotic CVCs would likely be bulk purchased for all children (including those with a lower risk of BSI than the risk for children participating in the trial). By estimating the number of BSIs potentially averted using antibiotic CVCs for all children (including those with a low risk of BSI), we showed that the additional cost of purchasing antibiotic CVCs is less than the value of resources associated with managing excess BSIs associated with using standard CVCs. A limitation of this study was that estimated BSI rates using standard CVCs relied on a predictive model for identifying children most likely to have required a CVC. Another limitation was the possible error in estimating CVC-days: we assumed that, for children in the linked data set likely to have required a CVC, the CVC would remain in place for the entire PICU stay. There is no clear direction of bias as we may have over- or underestimated CVC-days, but our assumptions are reasonable based on the subset of CATCH participants. Finally, we relied on survey responses to estimate the number of CVCs required in PICUs, but we addressed this and the uncertainty in other parameter estimates by performing sensitivity analyses. 95,96
The generalisability of the RCT results can be assessed by accounting for differences in subgroup treatment effects, for example by reweighting treatment effects based on population distributions. 97,98 In the CATCH trial, the event rate was low and there was limited power to assess variation in the treatment effect according to the duration of CVC insertion. However, because of the nature of the intervention, we assumed that the treatment effect would be constant across groups and would be the same in children who were not enrolled into the trial, as there was no a priori reason for an interaction.
Our results suggest that the benefits of using impregnated CVCs apply even for PICUs with BSI rates as low as 1.2 per 1000 CVC-days. These finding are consistent with systematic review evidence on the cost-effectiveness of impregnated CVCs in adults, which indicates that implementation of impregnated CVCs would be cost-effective for a range of RRs and for a baseline incidence of CR-BSIs as low as 0.2%. 29 The CATCH trial is the first trial to assess the effectiveness of antibiotic-impregnated compared with standard CVCs in children, and our results adds to the strong evidence of effectiveness in adults. Furthermore, as our cost estimates consider only use of hospital resources, the true cost of BSIs and the benefits of antibiotic CVCs may be even greater when longer-term outcomes of BSIs are taken into account.
Other conclusions
Deferred consent
There is a growing recognition of the need for better evidence in paediatric settings, as evidence in adults cannot always be safely extrapolated to children. 99,100 However, achieving informed consent in emergency paediatric settings is complicated by the stressful situation and the need to avoid any delay in treatment. 56,101 As the CATCH trial was one of the first UK studies to use deferred consent in children, there was a lack of evidence on which to make decisions about the design and conduct of this aspect of the trial. 102,103 Our experience of deferred consent in the CATCH trial could help to inform future studies.
In the CATCH trial, deferred consent was obtained from 84% of families who were approached. 60 The use of deferred consent allowed us to recruit emergency admissions, reach the target sample size within the available funding and provide results that are convincing to clinicians working in the emergency setting. Participation in the CATCH trial after the intervention had taken place represented a minimal burden to children (use of data already collected and follow-up data collection only). However, a proportion of parents chose not to consent because of a perceived burden on the child. Ongoing in-depth research as part of the CONseNt methods in paediatric Emergency and urgent Care Trials (CONNECT) study56,104 may help to explain further the experiences and choices of parents of children involved in the CATCH trial.
One of the main concerns relating to deferred consent in the CATCH trial was whether or not the decision to consent was related to the child’s outcome. The ethics committee recommended not approaching families whose child had been discharged or transferred before the original approach for consent could be made. Inclusion rates were also lower in the group of children who died. Although there were no deaths related to the type of CVC in the CATCH trial, the low rate of consent for children who died could bias the validity of comparisons between treatment arm and outcomes, including adverse events. We propose that, in future, ethics committees allow use of linked administrative records without consent, when reasonable efforts to obtain consent have been made or are not feasible or are considered to be harmful. 60
There is still uncertainty about the most appropriate ways to approach bereaved parents of children randomised in an emergency. 105 Our experience in the CATCH trial highlights that further in-depth research should be incorporated into the design of emergency trials involving populations with high mortality rates. 106,107
Co-enrolment
Another challenge to improving evidence in paediatric settings is the limited population of children who can be recruited into trials. The CATCH trial was the largest RCT conducted in paediatric intensive care to date and overlapped with the second largest RCT [the Control of Hypoglycaemia in Paediatric Intensive Care (CHiP) trial],108 which recruited 1369 children in 13 centres. Allowing co-enrolment into several trials at the same time can potentially enable efficient recruitment of children and has been successful in particular settings, for example in evaluating treatments for acquired immune deficiency syndrome. 88,90,109,110 Aside from statistical concerns, perceived burden to the child, ethics requirements and stress of recruiting into multiple trials are barriers to co-enrolment. 111–114
Of five PICUs with the opportunity to recruit simultaneously to both the CATCH trial and the CHiP trial, only two units decided to allow co-enrolment. Of the remaining three units, one delayed recruitment of elective patients to the CATCH trial until the CHiP trial had closed, resulting in a loss of 6 recruiting weeks. Reasons provided for not allowing co-enrolment related to concerns about jeopardising recruitment targets for the earlier trial, asking too much of parents because of the overwhelming amounts of information involved for two trials and the stressful situation of intensive care. 112
On the other hand, we found that parents were accepting of co-enrolment: recruitment rates at the same PICU were similar whether parents were approached for a single study (78% for CATCH, 51% for CHiP) or both studies (82% for CATCH, 51% for CHiP). Concerns of the PICUs were therefore not supported by evidence on parental decisions. 115,116
Our experience with the CATCH trial highlighted that co-enrolment can be successful and acceptable, but that barriers to co-enrolment remain. Decisions on the appropriateness of co-enrolment need to take into account the potential impact on results, interaction between therapies, safety and internal and external validity. Strategies that allow increasing research capacity whilst minimising burden on patients and parents should continue to be developed.
Administrative/electronic health-care data to support randomised controlled trials
This study provides a convincing example of how administrative and electronic health-care data can be used to support and enhance RCTs. 117 It would not have been possible to provide such comprehensive information relating to the use of impregnated CVCs without the use of administrative data, which contributed to all three aspects of the study:
-
clinical effectiveness: trial participant data were linked with (i) mortality data from the ONS to allow evaluation of deaths within 30 days of randomisation and (ii) PICANet data to ascertain the primary diagnosis at admission and PIM2 score
-
cost-effectiveness: HES and PICANet data were used to estimate hospital, ICU and HDU costs up to 6 months after randomisation
-
generalisability and cost impact: PICANet data linked with national laboratory surveillance data were used to estimate rates of BSI outside of the trial setting.
There are other areas in which administrative and electronic health-care data could be used to enhance and support RCTs. 117 First, in terms of capturing outcomes, we used administrative data up to 6 months post randomisation. Ongoing linkage with administrative data could be useful to many RCTs for capturing further long-term outcomes and safety measures. 118
Second, the sample size calculation in the CATCH trial was based on audit data from several PICUs prior to the trial. If PICANet and infection surveillance data had been linked prior to the study, even more accurate event rates, taking into account the context of decreasing BSI rates, could have been calculated. Using administrative data to identify variation in care across services and to aid site selection will lead to more well-designed trials that are likely to meet targets and provide evidence more quickly.
Third, we used administrative data collected during the trial period to assess the generalisability of trial participants and to identify the population for whom impregnated CVCs may be purchased. This could be extended post trial by monitoring the scaling up of effective interventions and for the continued study of the safety and efficacy of new medicines and devices.
Barriers to realising the full potential of integrating administrative data into RCTs include concerns about data quality, regulatory compliance and ethical issues relating to consent for data linkage. Decisions on the appropriateness of using administrative data should be made on a trial-by-trial basis. However, administrative data provide an opportunity to efficiently investigate short- and long-term effectiveness in real health-care settings, assess the broader impact of treatments across the NHS and provide evidence on interventions to help implement improved treatments quickly for those who would benefit most. The potential to improve quality and decrease the burden and cost of RCTs is particularly important in the paediatric setting. 99,119,120
Implications for practice
Although our primary outcome showed no difference between the different types of CVC, secondary analyses demonstrated strong evidence of effectiveness of antibiotic-impregnated CVCs compared with standard and heparin-bonded CVCs in children. Based on these results, we consider that use of antibiotic-impregnated CVCs for children admitted to PICUs could result in clinically important reductions in BSI rates. We expect that the benefits of antibiotic-impregnated CVCs would be likely to apply even for PICUs with low BSI rates. At the cost-effectiveness threshold of £30,000 per QALY, antibiotic-impregnated CVCs may not represent a cost-effective alternative to standard CVCs in a PICU setting. However, there is considerable uncertainty surrounding this estimate, which is driven mainly by the time horizon of analysis. Careful monitoring of implementation would help to build up further evidence.
Recommendations for future research
Our research suggests that adoption of impregnated CVCs in PICUs should be considered. Implementation could be monitored through continued linkage of electronic health-care data and information on PICU practice. Such monitoring could allow routine feedback to PICUs and could be enhanced by routine capture of CVC insertion and removal dates in hospital records.
We do not recommend any further trials of antibiotic-impregnated or heparin-bonded CVCs compared with standard CVCs for children or adults in intensive care. However, further trials could be justified to determine whether or not antibiotic CVCs would be similarly effective in preterm neonates (for whom smaller line sizes are required, with potentially different mechanisms for BSI) or in those with long-term CVCs (to determine whether or not the effect of impregnation remains for longer periods). The NHS should work with industry to evaluate different types of impregnation for specific patient groups.
Use of linked administrative data should be considered for future trials to determine the generalisability of interventions in contexts in which outcomes are likely to change substantially over the lifetime of the trial and to monitor implementation of effective interventions. 117
Acknowledgements
We thank the children and families who participated in the CATCH trial and the principal investigators and research nurses at each study site (in order of number of patients recruited): Great Ormond Street Hospital – Quen Mok, Twin Yen Lee and Samantha Riordan; Southampton General Hospital – Iain Macintosh, Jenni McCorkell, Katie Stearn and Rosie Mitchell; Evelina London Children’s Hospital – Shane Tibby, Julie Harris and Paul Wellman; Birmingham Children’s Hospital – Oliver Bagshaw, Jenna Spry, Simon Laker and Nikki Holdback; Leeds General Infirmary – John Roche, Sian Cooper and Darren Hewett; Alder Hey Children’s Hospital – Steve Kerr and Felicity Haigh; Bristol Royal Hospital for Children – Michelle White, Margrid Schindler, Clare Traub and Nina Worrin; Glenfield Hospital – Raghu Ramaiah and Rekha Patel; Royal Brompton Hospital – Duncan Macrae and Sarah Bacon; St Mary’s Hospital, London – Mehrengise Cooper, Amina Abdulla and Amy Brewer; Royal Victoria Infirmary – Rachel Agbeko and Christine Mackerness; Queen’s Medical Centre – Patrick Davies, Daniel Walsh and Lindsay Crate; Freeman Hospital – Rachel Agbeko and Clare Simmister; and, Leicester Royal Infirmary – Raghu Ramaiah and Rekha Patel. We thank Hazel Greig-Midlane for contributing a lay perspective to the design and conduct of the study.
We also thank the Local Research Networks in England whose work and support was invaluable in supporting the CATCH trial (see Trial oversight committees). We would also like to thank the British Antibiotic and Silver-Impregnated Catheters (BASICS) data managers Susan Howlin and Lynsey Finnetty.
We would like to thank all of the staff in the participating hospitals who have collected data for PICANet or responded to survey questionnaires. We are grateful to the UK Paediatric Intensive Care Society for its continued support and to the members of the PICANet Steering Group and Clinical Advisory Group, who are listed on the PICANet website [see www.picanet.org.uk/About/ (accessed 25 November 2015)].
We would like to acknowledge Nicola Panton who carried out the 16S analyses, Mark Wilks who co-ordinated the collection and storage of relevant samples and Michaela Blundell who undertook quality assurance checks.
We wish to acknowledge the contribution of Catrin Plumpton to the economic analysis. For work relating to the generalisability study, we would like to acknowledge the input of Angie Wade and Harvey Goldstein, who made substantial contributions to the data linkage study. We would also like to thank Roger Parslow, Tom Fleming, Phil McShane and Lee Norman (PICANet) for facilitation of PICANet data retrieval. We thank Dr Roger Parslow and The Healthcare Quality Improvement Partnership for contributing data from the PICANet Audit. The PICANet Audit is commissioned by the Healthcare Quality Improvement Partnership (HQIP) as part of the National Clinical Audit (NCA) Programme. HQIP is led by a consortium of the Academy of Medical Royal Colleges, the Royal College of Nursing and National Voices. Its aim is to promote quality improvement and in particular to increase the impact that clinical audit has on health-care quality in England and Wales. HQIP holds the contract to manage and develop the NCA Programme, comprising more than 30 clinical audits that cover care provided to people with a wide range of medical, surgical and mental health conditions. The PICANet Audit is funded by NHS England, NHS Wales, NHS Lothian/National Service Division NHS Scotland, the Royal Belfast Hospital for Sick Children, The National Office of Clinical Audit, Republic of Ireland and Hospital Corporation of America® Healthcare. We also thank Sue Howlin and Andrew McKay from the Medicines for Children Clinical Trials Unit for collating trial data. Thanks also go to those contributing CVC audit data: John Hartley and Paul Lock (Great Ormond Street Hospital), Jim Grey and Adrian Bigland (Birmingham Children’s Hospital), Rachel Agbeko (Newcastle upon Tyne Hospitals NHS Foundation Trust) and Sarah Morley, Tim Peto and Anke Top (Cambridge University Hospitals NHS Foundation Trust).
Trial oversight committees
We would like to thank all of the members of the trial oversight committees for their support and work throughout the study and the recommendations in Table 23: Trial Steering Committee – Robert Tasker (Chair), Stephen Playfor (Chair), Andy Vail, Derek Roebuck, Jim Gray and Hazel Greig-Midlane; IDSMC – Paul Ewings (Chair), Mike Sharland, Neena Modi; and the Trial Management Group – Ruth Gilbert (Chair and Chief Investigator), Carroll Gamble, Kerry Dwan, Tracy Moitt, Rachel Breen, Colin Ridyard, Angie Wade, Dyfrig Hughes, Quen Mok, Liz Draper, Shane Tibby, Michael Millar, Oliver Bragshaw, Padmanabhan Ramnarayan, Julia Harris and Darren Hewett.
Oversight committee | Meeting dates | Recommendations |
---|---|---|
IDSMC | 26 October 2009, 26 March 2010, 10 February 2012, 14 June 2012 | Trial to be continued |
Trial Steering Committee | 26 October 2009, 26 March 2010, 12 March 2012, 2 August 2012 | Support for continuing to recruit past the target number |
All members of the Trial Management Group, the Trial Steering Committee and the IDSMC were invited to comment on the final draft of the report.
Ethics
For PICANet, collection of personally identifiable data was approved by the National Information Governance Board for Health and Social Care (formerly the Patient Information Advisory Group) [see www.nigb.nhs.uk/s251/registerapp (accessed 25 November 2015)] and ethical approval was granted by the Trent Medical Research Ethics Committee (reference number 05/MRE04/17). PICANet also has specific permission from the National Research Ethics Service for linkage with PHE laboratory data on BSIs using personal identifiers and to share PICANet data with PHE. An exemption under Section 251 of the NHS Act 2006121 (previously Section 60 of the Health and Social Care Act 2001122) allows PHE to receive patient-identifiable data from other organisations without patient consent to monitor infectious disease. Specific permission for the PICANet–PHE linkage has been granted by the National Information Governance Board for Health and Social Care.
Funding
This work was supported by funding from the National Institute for Health Research Health Technology Assessment (NIHR HTA) programme (project number 08/13/47). The views and opinions expressed therein are those of the authors and do not necessarily reflect those of the HTA programme, NIHR, NHS or Department of Health. No funding was provided by the manufacturer (Cook Medical Inc.) of the CVCs, although participating units could purchase CVCs at a discount of 20% during recruitment to the study. Neither the funder nor the manufacturer had any involvement in the study design, interpretation of the results or writing of the report.
Contributions of authors
Katie Harron (research fellow), Dyfrig A Hughes (Professor of Pharmacoeconomics) and Ruth E Gilbert (Professor of Clinical Epidemiology) prepared drafts of the report, which were reviewed and amended by coauthors [Quen Mok (Consultant in Paediatric Intensive Care), Kerry Dwan (Statistician), Tracy Moitt (senior trials manager), Michael Millar (Consultant in Infection), Padmanabhan Ramnarayan (Consultant in Paediatric Intensive Care and Retrieval), Shane M Tibby (Consultant in Paediatric Intensive Care), Berit Muller-Pebody (Consultant in Infection Control) and Carrol Gamble (Professor in Medical Statistics)].
Statistical analyses were conducted by Kerry Dwan (research associate) and Carrol Gamble (Professor of Medical Statistics).
Colin H Ridyard (research officer in Health Economics) performed the cost-effectiveness analyses.
Berit Muller-Pebody co-ordinated the linkage of PICANet data to laboratory surveillance data.
The end-point review for the primary outcome was carried out by Quen Mok (Consultant in Paediatric Intensive Care), Michael Millar and Ruth E Gilbert (Professor of Clinical Epidemiology).
All authors were invited to comment on the final draft of the report.
Publications
Research articles
Harron K, Ramachandra G, Mok Q, Gilbert R. Consistency between guidelines and reported practice for reducing the risk of catheter-related infection in British paediatric intensive care units. Intensive Care Med 2011;37:1641–7.
Goldstein H, Harron K, Wade A. The analysis of record-linked data using multiple imputation with data value priors. Stat Med 2012;31:3481–93.
Harron K, Lee T, Ball T, Mok Q, Gamble C, Macrae D, et al. Making co-enrolment feasible for randomised controlled trials in paediatric intensive care. PLOS ONE 2012;7:e41791.
Harron K, Goldstein H, Wade A, Muller-Pebody B, Parslow R, Gilbert R. Linkage, evaluation and analysis of national electronic healthcare data: application to providing enhanced blood-stream infection surveillance in paediatric intensive care. PLOS ONE 2013;8:e85278.
Harron K, Wade A, Muller-Pebody B, Goldstein H, Parslow R, Gray J, et al. Risk-adjusted monitoring of blood-stream infection in paediatric intensive care: a data linkage study. Intensive Care Med 2013;39:1080–7.
Woolfall K, Frith L, Gamble C, Young B. How experience makes a difference: practitioners’ views on the use of deferred consent in paediatric and neonatal emergency care trials. BMC Med Ethics 2013;14:45.
Harron K, Mok Q, Parslow R, Muller-Pebody B, Gilbert R, Ramnarayan P. Risk of bloodstream infection in children admitted to paediatric intensive care units in England and Wales following emergency inter-hospital transfer. Intensive Care Med 2014;40:1916–23.
Harron K, Wade A, Gilbert R, Muller-Pebody B, Goldstein H. Evaluating bias due to data linkage error in electronic healthcare records. BMC Med Res Methodol 2014;14:36.
Harron K, Parslow R, Mok Q, Tibby S, Wade A, Muller-Pebody B, et al. Monitoring quality of care through linkage of administrative data: national trends in bloodstream infection in UK paediatric intensive care units 2003–2012. Crit Care Med 2015;43:1070–8.
Harron K, Woolfall K, Dwan K, Gamble C, Mok Q, Ramnarayan P, et al. Deferred consent for randomized controlled trials in emergency care settings. Pediatrics 2015;136:e1316–22.
Woolfall K, Frith L, Gamble C, Gilbert R, Mok Q, Young B, et al. How parents and practitioners experience research without prior consent (deferred consent) for emergency research involving children with life threatening conditions: a mixed method study. BMJ Open 2015;5:e008522.
Gilbert RE, Mok Q, Dwan K, Harron K, Moitt T, Millar M, et al. Impregnated central venous catheters for prevention of bloodstream infection in children (the CATCH trial): a randomised controlled trial [published online ahead of print March 3 2016]. Lancet 2016. http://dx.doi.org/10.1016/S0140-6736(16)00340-8
Harron K, Mok Q, Hughes D, Muller-Pebody B, Parslow R, Ramnarayan P, et al. Generalisability and cost-impact of antibiotic-impregnated central venous catheters for reducing the risk of bloodstream infection in paediatric intensive care units in England. PLOS ONE 2016; in press.
Letters and editorials
Mok Q, Gilbert R. Interventions to reduce central venous catheter-associated infections in children: which ones are beneficial? Intensive Care Med 2011;37:566–8.
Harron K, Wade A, Muller-Pebody B, Goldstein H, Gilbert R. Opening the black box of record linkage. J Epidemiol Community Health 2012;66:1198.
Gilbert R, Harron K, Mok Q, Gamble C. Impregnated central venous catheters should be readily used to reduce risk of bloodstream infection. BMJ 2013;347:f7169.
Harron K, Gilbert R. Research: increasing value, reducing waste. Lancet 2014;383:1124.
Harron K, Gamble C, Gilbert R. E-health data to support and enhance randomised controlled trials in the United Kingdom. Clin Trials 2015;12:180–2.
Data sharing statement
All available data can be obtained, subject to data sharing agreements, from the Medicines for Children Clinical Trials Unit.
Disclaimers
This report presents independent research funded by the National Institute for Health Research (NIHR). The views and opinions expressed by authors in this publication are those of the authors and do not necessarily reflect those of the NHS, the NIHR, NETSCC, the HTA programme or the Department of Health. If there are verbatim quotations included in this publication the views and opinions expressed by the interviewees are those of the interviewees and do not necessarily reflect those of the authors, those of the NHS, the NIHR, NETSCC, the HTA programme or the Department of Health.
References
- Falagas ME, Fragoulis K, Bliziotis IA, Chatzinikolaou I. Rifampicin-impregnated central venous catheters: a meta-analysis of randomized controlled trials. J Antimicrob Chemoth 2007;59:359-69. http://dx.doi.org/10.1093/jac/dkl522.
- Gilbert R, Harden M. Effectiveness of impregnated central venous catheters for catheter related blood stream infection: a systematic review. Curr Opin Infect Dis 2008;21:235-45. http://dx.doi.org/10.1097/QCO.0b013e3282ffd6e0.
- O’Grady N, Alexander M, Dellinger E, Gerberding J, Heard S, Maki D, et al. Guidelines for the prevention of intravascular catheter-related infections. Am Acad Pediatr Policy 2002;110:e51-74. http://dx.doi.org/10.1067/mic.2002.129427.
- Pellowe C, Pratt R, Loveday H, Harper P, Robinson N, Jones S. The epic project. Updating the evidence-base for national evidence-based guidelines for preventing healthcare-associated infections in NHS hospitals in England: a report with recommendations. J Hosp Infect 2005;5. http://dx.doi.org/10.1016/j.jhin.2004.11.007.
- Raad I, Hanna H, Maki D. Intravascular catheter-related infections: advances in diagnosis, prevention, and management. Lancet Infect Dis 2007;7:645-57. http://dx.doi.org/10.1016/S1473-3099(07)70235-9.
- Costello J, Graham D, Morrow D, Potter-Bynoe G, Sandora T, Laussen P. Risk factors for central line-associated bloodstream infection in a pediatric cardiac intensive care unit. Pediatr Crit Care Med 2009;10:453-9. http://dx.doi.org/10.1097/PCC.0b013e318198b19a.
- Mello M, Albuquerque M, Lacerda H, Souza W, Correia J, Britto M. Risk factors for healthcare-associated infection in pediatric intensive care units: a systematic review. Cad Saude Publica 2009;25:373-91. http://dx.doi.org/10.1590/S0102-311X2009001500004.
- Milstone AM, Sengupta A. Do prolonged peripherally inserted central venous catheter dwell times increase the risk of bloodstream infection?. Infect Control Hosp Epidemiol 2010;31:1184-7. http://dx.doi.org/10.1086/656589.
- Niedner MF, Huskins WC, Colantuoni E, Muschelli J, Harris JM II, Rice TB, et al. Epidemiology of central line-associated bloodstream infections in the pediatric intensive care unit. Infect Control Hosp Epidemiol 2011;32. http://dx.doi.org/10.1086/662621.
- Wylie MC, Graham DA, Potter-Bynoe G, Kleinman ME, Randolph AG, Costello JM, et al. Risk factors for central line-associated bloodstream infection in pediatric intensive care units. Infect Control Hosp Epidemiol 2010;31:1049-56. http://dx.doi.org/10.1086/656246.
- Bhutta A, Gilliam C, Honeycutt M, Schexnayder S, Green J, Moss M, et al. Reduction of bloodstream infections associated with catheters in paediatric intensive care unit: stepwise approach. BMJ 2007;334:362-5. http://dx.doi.org/10.1136/bmj.39064.457025.DE.
- Miller MR, Griswold M, Harris JM II, Yenokyan G, Huskins WC, Moss M, et al. Decreasing PICU catheter-associated bloodstream infections: NACHRI’s quality transformation efforts. Pediatrics 2010;125:206-13. http://dx.doi.org/10.1542/peds.2009-1382.
- Pronovost P, Goeschel C, Colantuoni E, Watson S, Lubomski L, Berenholtz S, et al. Sustaining reductions in catheter related bloodstream infections in Michigan intensive care units: observational study. BMJ 2010;340. http://dx.doi.org/10.1136/bmj.c309.
- Bion J, Richardson A, Hibbert P, Beer J, Abrusci T, McCutcheon M, et al. ‘Matching Michigan’: a 2-year stepped interventional programme to minimise central venous catheter-blood stream infections in intensive care units in England. BMJ Qual Saf 2012;22:110-23. http://dx.doi.org/10.1136/bmjqs-2012-001325.
- Saving Lives: Reducing Infection, Delivering Clean and Safe Care. London: DH; 2007.
- Jeong IS, Park SM, Lee JM, Song JY, Lee SJ. Effect of central line bundle on central line-associated bloodstream infections in intensive care units. Am J Infect Control 2013;41:710-16. http://dx.doi.org/10.1016/j.ajic.2012.10.010.
- Pronovost P, Needham D, Berenholtz S, Sinopoli D, Chu H, Cosgrove S, et al. An intervention to decrease catheter-related bloodstream infections in the ICU. New Engl J Med 2006;355:2725-32. http://dx.doi.org/10.1056/NEJMoa061115.
- Reddy KK, Samuel A, Smiley KA, Weber S, Hon H. Reducing central line-associated bloodstream infections in three ICUs at a tertiary care hospital in the United Arab Emirates. Jt Comm J Qual Patient Saf 2014;40.
- Zuschneid I, Schwab F, Geffers C, Rüden H, Gastmeier P. Reducing central venous catheter-associated primary bloodstream infections in intensive care units is possible: data from the German nosocomial infection surveillance system. Infect Control Hosp Epidemiol 2003;24:501-5. http://dx.doi.org/10.1086/502236.
- Abou Elella R, Najm H, Balkhy H, Bullard L, Kabbani M. Impact of bloodstream infection on the outcome of children undergoing cardiac surgery. Pediatr Cardiol 2010;31:483-9. http://dx.doi.org/10.1007/s00246-009-9624-x.
- Elward AM, Hollenbeak CS, Warren DK, Fraser VJ. Attributable cost of nosocomial primary bloodstream infection in pediatric intensive care unit patients. Pediatrics 2005;115:868-72. http://dx.doi.org/10.1542/peds.2004-0256.
- Lakshmi KS, Jayashree M, Singhi S, Ray P. Study of nosocomial primary bloodstream infections in a pediatric intensive care unit. J Trop Pediatr 2007;53:87-92. http://dx.doi.org/10.1093/tropej/fml073.
- Yogaraj JS, Elward AM, Fraser VJ. Rate, risk factors, and outcomes of nosocomial primary bloodstream infection in pediatric intensive care unit patients. Pediatrics 2002;110:481-5. http://dx.doi.org/10.1542/peds.110.3.481.
- Dominguez TE, Chalom R, Costarino ATJ. The impact of adverse patient occurrences on hospital costs in the pediatric intensive care unit. Crit Care Med 2001;29:169-74. http://dx.doi.org/10.1097/00003246-200101000-00033.
- Nowak JE, Brilli RJ, Lake MR, Sparling KW, Butcher J, Schulte M, et al. Reducing catheter-associated bloodstream infections in the pediatric intensive care unit: business case for quality improvement. Pediatr Crit Care Med 2010;11:579-87. http://dx.doi.org/10.1097/PCC.0b013e3181d90569.
- Slonim AD, Kurtines HC, Sprague BM, Singh N. The costs associated with nosocomial bloodstream infections in the pediatric intensive care unit. Pediatr Crit Care Med 2001;2:170-4. http://dx.doi.org/10.1097/00130478-200104000-00012.
- Health Protection Agency . English National Point Prevalence Survey on Healthcare-Associated Infections and Antimicrobial Use: 2011 n.d. www.gov.uk/government/publications/healthcare-associated-infections-hcai-point-prevalence-survey-england (accessed 6 October 2014).
- Wang H, Huang T, Jing J, Jin J, Wang P, Yang M, et al. Effectiveness of different central venous catheters for catheter-related infections: a network meta-analysis. J Hosp Infect 2010;76:1-11. http://dx.doi.org/10.1016/j.jhin.2010.04.025.
- Hockenhull J, Dwan K, Boland A, Smith G, Bagust A, Dündar Y, et al. The clinical effectiveness and cost-effectiveness of central venous catheters treated with anti-infective agents in preventing bloodstream infections: a systematic review and economic evaluation. Health Technol Asses 2008;12.
- Lai NM, Chaiyakunapruk N, Lai NA, O’Riordan E, Pau WSC, Saint S. Catheter impregnation, coating or bonding for reducing central venous catheter-related infections in adults. Cochrane Database Syst Rev 2013;6. http://dx.doi.org/10.1002/14651858.cd007878.pub2.
- Gilbert R, Harron K, Mok Q, Gamble C. Impregnated central venous catheters should be readily used to reduce risk of bloodstream infection. BMJ 2013;347. http://dx.doi.org/10.1136/bmj.f7169.
- Harron K, Ramachandra G, Mok Q, Gilbert R. Consistency between guidelines and reported practice for reducing the risk of catheter-related infection in British paediatric intensive care units. Intensive Care Med 2011;37:1641-7. http://dx.doi.org/10.1007/s00134-011-2343-9.
- Harron K, Wade A, Muller-Pebody B, Goldstein H, Parslow R, Gray J, et al. Risk-adjusted monitoring of blood-stream infection in paediatric intensive care: a data linkage study. Intensive Care Med 2013;39:1080-7. http://dx.doi.org/10.1007/s00134-013-2841-z.
- Harron K, Parslow R, Mok Q, Tibby S, Wade A, Muller-Pebody B, et al. Monitoring quality of care through linkage of administrative data: national trends in bloodstream infection in UK paediatric intensive care units 2003–2012. Crit Care Med 2015;43:1070-8. http://dx.doi.org/10.1097/CCM.0000000000000941.
- Chatzinikolaou I, Finkel K, Hanna H, Boktour M, Foringer J, Ho T, et al. Antibiotic-coated hemodialysis catheters for the prevention of vascular catheter–related infections: a prospective, randomized study. Am J Med 2003;115:352-7. http://dx.doi.org/10.1016/S0002-9343(03)00367-X.
- Cox EG, Knoderer CA, Jennings A, Brown JW, Rodefeld MD, Walker SG, et al. A randomized, controlled trial of catheter-related infectious event rates using antibiotic-impregnated catheters versus conventional catheters in pediatric cardiovascular surgery patients. J Ped Infect Dis 2013;2:67-70. http://dx.doi.org/10.1093/jpids/pis066.
- Darouiche RO, Berger DH, Khardori N, Robertson CS, Wall MJ, Metzler MH, et al. Comparison of antimicrobial impregnation with tunneling of long-term central venous catheters: a randomized controlled trial. Ann Surg 2005;242. http://dx.doi.org/10.1097/01.sla.0000171874.29934.61.
- Hanna H, Benjamin R, Chatzinikolaou I, Alakech B, Richardson D, Mansfield P, et al. Long-term silicone central venous catheters impregnated with minocycline and rifampin decrease rates of catheter-related bloodstream infection in cancer patients: a prospective randomized clinical trial. J Clin Oncol 2004;22:3163-71. http://dx.doi.org/10.1200/JCO.2004.04.124.
- León C, Ruiz-Santana S, Rello J, Maria V, Vallés J, Álvarez-Lerma F, et al. Benefits of minocycline and rifampin-impregnated central venous catheters. Intensive Care Med 2004;30:1891-9. http://dx.doi.org/10.1007/s00134-004-2378-2.
- Marik PE, Abraham G, Careau P, Varon J, Fromm RE. The ex vivo antimicrobial activity and colonization rate of two antimicrobial-bonded central venous catheters. Crit Care Med 1999;27:1128-31. http://dx.doi.org/10.1097/00003246-199906000-00034.
- Raad I, Darouiche R, Dupuis J, Abi-Said D, Gabrielli A, Hachem R, et al. Central venous catheters coated with minocycline and rifampin for the prevention of catheter-related colonization and bloodstream infections: a randomized, double-blind trial. Ann Intern Med 1997;127:267-74. http://dx.doi.org/10.7326/0003-4819-127-4-199708150-00002.
- Yücel N, Lefering R, Maegele M, Max M, Rossaint R, Koch A, et al. Reduced colonization and infection with miconazole–rifampicin modified central venous catheters: a randomized controlled clinical trial. J Antimicrob Chemoth 2004;54:1109-15. http://dx.doi.org/10.1093/jac/dkh483.
- Abdelkefi A, Achour W, Ben Othman T, Ladeb S, Torjman L, Lakhal A, et al. Use of heparin-coated central venous lines to prevent catheter-related bloodstream infection. J Support Oncol 2007;5:273-8.
- Appelgren P, Ransjo U, Bindslev L, Espersen F, Larm O. Surface heparinization of central venous catheters reduces microbial colonization in vitro and in vivo: results from a prospective, randomized trial. Crit Care Med 1996;24:1482-9. http://dx.doi.org/10.1097/00003246-199609000-00009.
- Pierce CM, Wade A, Mok Q. Heparin-bonded central venous lines reduce thrombotic and infective complications in critically ill children. Intensive Care Med 2000;26:967-72. http://dx.doi.org/10.1007/s001340051289.
- Geffers C, Zuschneid I, Eckmanns T, Rüden H, Gastmeier P. The relationship between methodological trial quality and the effects of impregnated central venous catheters. Intensive Care Med 2003;29:403-9.
- Niel-Weise B, Stijnen T, Van den Broek P. Anti-infective-treated central venous catheters for total parenteral nutrition or chemotherapy: a systematic review. J Hosp Infect 2008;69:114-23. http://dx.doi.org/10.1016/j.jhin.2008.02.020.
- Mok Q, Gilbert R. Interventions to reduce central venous catheter-associated infections in children: which ones are beneficial?. Intensive Care Med 2011;37:566-8. http://dx.doi.org/10.1007/s00134-011-2135-2.
- Mermel L. Prevention of intravascular catheter-related infections. Ann Intern Med 2000;132:391-402. http://dx.doi.org/10.7326/0003-4819-132-5-200003070-00009.
- Barnett AG, Batra R, Graves N, Edgeworth J, Robotham J, Cooper B. Using a longitudinal model to estimate the effect of methicillin-resistant Staphylococcus aureus infection on length of stay in an intensive care unit. Am J Epidemiol 2009;170:1186-94. http://dx.doi.org/10.1093/aje/kwp249.
- Graves N, Harbarth S, Beyersmann J, Barnett A, Halton K, Cooper B. Estimating the cost of health care-associated infections: mind your p’s and q’s. Clin Infect Dis 2010;50:1017-21. http://dx.doi.org/10.1086/651110.
- Halton K, Graves N. Economic evaluation and catheter-related bloodstream infections. Emerging Infectious Diseases 2007;13:815-23. http://dx.doi.org/10.3201/eid1306.070048.
- Gilbert RE, Mok Q, Dwan K, Harron K, Moitt T, Millar M, et al. Impregnated central venous catheters for prevention of bloodstream infection in children (the CATCH trial): a randomised controlled trial [published online ahead of print March 3 2016]. Lancet 2016. http://dx.doi.org/10.1016/S0140-6736(16)00340-8.
- Cook Spectrum® Central Venous Catheter Minocycline/Rifampin Antibiotic Impregnated Power Injectable. Bjaeverskov, Denmark: William Cook Europe; 2013.
- Mermel LA, Stolz SM, Maki DG. Surface antimicrobial activity of heparin-bonded and antiseptic-impregnated vascular catheters. J Infect Dis 1993;167:920-4. http://dx.doi.org/10.1093/infdis/167.4.920.
- Woolfall K, Frith L, Gamble C, Young B. How experience makes a difference: practitioners’ views on the use of deferred consent in paediatric and neonatal emergency care trials. BMC Med Ethics 2013;14. http://dx.doi.org/10.1186/1472-6939-14-45.
- Paediatric Intensive Care Audit Network: A Decade of Data. Leeds: University of Leeds; 2014.
- Slater A, Shann F, Pearson G. PIM2: a revised version of the Paediatric Index of Mortality. Intensive Care Med 2003;29:278-85.
- Gray RJ. A class of K-sample tests for comparing the cumulative incidence of a competing risk. Ann Stat 1988;16:1141-54. http://dx.doi.org/10.1214/aos/1176350951.
- Harron K, Woolfall K, Dwan K, Gamble C, Mok Q, Ramnarayan P, et al. Deferred consent for randomized controlled trials in emergency care settings. Pediatrics 2015;136:e1316-22. http://dx.doi.org/10.1542/peds.2015-0512.
- Halton KA, Cook DA, Whitby M, Paterson DL, Graves N. Cost effectiveness of antimicrobial catheters in the intensive care unit: addressing uncertainty in the decision. Crit Care 2009;13. http://dx.doi.org/10.1186/cc7744.
- Frank U, Chojnacki T, Dettenkofer M, Daschner FD. Cost-effectiveness of an antiseptic-impregnated central venous catheter in the ICU. Intensive Care Med 2003;29.
- Marciante KD, Veenstra DL, Lipsky BA, Saint S. Which antimicrobial impregnated central venous catheter should we use? Modeling the costs and outcomes of antimicrobial catheter use. Am J Infect Control 2003;31:1-8. http://dx.doi.org/10.1067/mic.2003.35.
- Shorr AF, Humphreys CW, Helman DL. New choices for central venous catheters: potential financial implications. Chest 2003;124:275-84. http://dx.doi.org/10.1016/S0012-3692(15)36021-9.
- Veenstra DL, Saint S, Sullivan SD. Cost-effectiveness of antiseptic-impregnated central venous catheters for the prevention of catheter-related bloodstream infection. JAMA 1999;282:554-60. http://dx.doi.org/10.1001/jama.282.6.554.
- Goudie A, Dynan L, Brady PW, Rettiganti M. Attributable cost and length of stay for central line-associated bloodstream infections. Pediatrics 2014;133:e1525-32. http://dx.doi.org/10.1542/peds.2013-3795.
- Wilson MZ, Rafferty C, Deeter D, Comito MA, Hollenbeak CS. Attributable costs of central line-associated bloodstream infections in a pediatric hematology/oncology population. Am J Infect Control 2014;42:1157-60. http://dx.doi.org/10.1016/j.ajic.2014.07.025.
- Health & Social Care Information Centre . Data Linkage &Amp; Extract Service n.d. www.hscic.gov.uk/dles (accessed 8 June 2014).
- Department of Health . National Schedule of Reference Costs 2012–13 n.d. www.gov.uk/government/publications/nhs-reference-costs-2012-to-2013 (accessed 8 June 2014).
- Department of Health . PbR Tariff Information Spreadsheet for 2012 to 2013 n.d. www.gov.uk/government/publications/confirmation-of-payment-by-results-pbr-arrangements-for-2012–13 (accessed 8 June 2014).
- Department of Health . A Simple Guide to Payment by Results 2012. www.gov.uk/government/uploads/system/uploads/attachment_data/file/213150/PbR-Simple-Guide-FINAL.pdf (accessed 11 December 2015).
- Fenwick E, Claxton K, Sculpher M. Representing uncertainty: the role of cost-effectiveness acceptability curves. Health Econ 2001;10:779-87. http://dx.doi.org/10.1002/hec.635.
- Mihaylova B, Briggs A, O’Hagan A, Thompson SG. Review of statistical methods for analysing healthcare resources and costs. Health Econ 2011;20:897-916. http://dx.doi.org/10.1002/hec.1653.
- Glick HA, Doshi JA, Sonnad SS, Polsky D. Economic Evaluation in Clinical Trials. Oxford: Oxford University Press; 2007.
- Husereau D, Drummond M, Petrou S, Carswell C, Moher D, Greenberg D, et al. Consolidated Health Economic Evaluation Reporting Standards (CHEERS) statement. BMJ 2013;346. http://dx.doi.org/10.1136/bmj.f1049.
- Braunholtz DA, Edwards SJ, Lilford RJ. Are randomized clinical trials good for us (in the short term)? Evidence for a ‘trial effect’. J Clin Epidemiol 2001;54:217-24. http://dx.doi.org/10.1016/S0895-4356(00)00305-X.
- Harron K, Goldstein H, Wade A, Muller-Pebody B, Parslow R, Gilbert R. Linkage, evaluation and analysis of national electronic healthcare data: application to providing enhanced blood-stream infection surveillance in paediatric intensive care. PLOS ONE 2013;8. http://dx.doi.org/10.1371/journal.pone.0085278.
- Wilson J, Elgohari S, Livermore DM, Cookson B, Johnson A, Lamagni T, et al. Trends among pathogens reported as causing bacteraemia in England, 2004–2008. Clin Microbiol Infect 2011;17:451-8. http://dx.doi.org/10.1111/j.1469-0691.2010.03262.x.
- Goldstein H, Harron K, Wade A. The analysis of record-linked data using multiple imputation with data value priors. Stat Med 2012;31:3481-93. http://dx.doi.org/10.1002/sim.5508.
- Harron K, Wade A, Gilbert R, Muller-Pebody B, Goldstein H. Evaluating bias due to data linkage error in electronic healthcare records. BMC Med Res Methodol 2014;14. http://dx.doi.org/10.1186/1471-2288-14-36.
- Rubin D. Multiple Imputation for Nonresponse in Surveys. New York: Wiley; 1987.
- Harron K. Evaluating Data Linkage Techniques for the Analysis of Bloodstream Infection in Paediatric Intensive Care 2014.
- Steyerberg EW, Harrell FE, Borsboom GJJM, Eijkemans MJC, Vergouwe Y, Habbema JDF. Internal validation of predictive models: efficiency of some procedures for logistic regression analysis. J Clin Epidemiol 2001;54:774-81. http://dx.doi.org/10.1016/S0895-4356(01)00341-9.
- Steyerberg EW, Eijkemans MJC, Harrell FE, Habbema JDF. Prognostic modeling with logistic regression analysis: in search of a sensible strategy in small data sets. Med Decis Making 2001;21:45-56. http://dx.doi.org/10.1177/0272989X0102100106.
- Harrell F, Lee KL, Mark DB. Tutorial in biostatistics multivariable prognostic models: issues in developing models, evaluating assumptions and adequacy, and measuring and reducing errors. Stat Med 1996;15:361-87. http://dx.doi.org/10.1002/(SICI)1097-0258(19960229)15:4<361::AID-SIM168>3.0.CO;2-4.
- Fluss R, Faraggi D, Reiser B. Estimation of the Youden Index and its associated cutoff point. Biom J 2005;47:458-72. http://dx.doi.org/10.1002/bimj.200410135.
- O’Hagan A, McCabe C, Akehurst R, Brennan A, Briggs A, Claxton K, et al. Incorporation of uncertainty in health economic modelling studies. Pharmacoeconomics 2005;23:529-36. http://dx.doi.org/10.2165/00019053-200523060-00001.
- Angus DC, Mira JP, Vincent JL. Improving clinical trials in the critically ill. Crit Care Med 2010;38. http://dx.doi.org/10.1097/CCM.0b013e3181c0259d.
- Schierholz J, Fleck C, Beuth J, Pulverer G. The antimicrobial efficacy of a new central venous catheter with long-term broad-spectrum activity. J Antimicrob Chemoth 2000;46:45-50. http://dx.doi.org/10.1093/jac/46.1.45.
- Dondorp AM, Fanello CI, Hendriksen ICE, Gomes E, Seni A, Chhaganlal KD, et al. Artesunate versus quinine in the treatment of severe falciparum malaria in African children (AQUAMAT): an open-label, randomised trial. Lancet 2010;376:1647-57. http://dx.doi.org/10.1016/S0140-6736(10)61924-1.
- Claxton K. The irrelevance of inference: a decision-making approach to the stochastic evaluation of health care technologies. J Health Econ 1999;18:341-64. http://dx.doi.org/10.1016/S0167-6296(98)00039-3.
- Kerren R, Pati S, Feudtner C. The generation gap; difference between children and adults pertinent to economic evaluations of health interventions. Pharmacoeconomics 2004;22:71-8.
- Tarride JE, Burke N, Bischof M, Hopkins RB, Goeree L, Campbell K, et al. A review of health utilities across conditions common in paediatric and adult populations. Health Qual Life Outcomes 2010;8. http://dx.doi.org/10.1186/1477-7525-8-12.
- Harron K, Mok Q, Parslow R, Muller-Pebody B, Gilbert R, Ramnarayan P. Risk of bloodstream infection in children admitted to paediatric intensive care units in England and Wales following emergency inter-hospital transfer. Intensive Care Med 2014;40:1916-23. http://dx.doi.org/10.1007/s00134-014-3516-0.
- Briggs A. Probabilistic analysis of cost-effectiveness models: statistical representation of parameter uncertainty. Value Health 2005;8:1-2. http://dx.doi.org/10.1111/j.1524-4733.2005.08101.x.
- Claxton K, Sculpher M, McCabe C, Briggs A, Akehurst R, Buxton M, et al. Probabilistic sensitivity analysis for NICE technology assessment: not an optional extra. Health Econ 2005;14:339-47. http://dx.doi.org/10.1002/hec.985.
- Cole SR, Stuart EA. Generalizing evidence from randomized clinical trials to target populations: the ACTG 320 Trial. Am J Epidemiol 2010;172:107-15. http://dx.doi.org/10.1093/aje/kwq084.
- Stuart EA, Cole SR, Bradshaw CP, Leaf PJ. The use of propensity scores to assess the generalizability of results from randomized trials. J R Stat Soc Ser A Stat Soc 2011;174:369-86. http://dx.doi.org/10.1111/j.1467-985X.2010.00673.x.
- Junker A, Klassen T. Improving standards for paediatric clinical trials. Paediatr Child Health 2011;16:539-40.
- Hartling L, Wittmeier KDM, Caldwell P, van der Lee H, Klassen TP, Craig JC, et al. StaR Child Health: developing evidence-based guidance for the design, conduct, and reporting of pediatric trials. Pediatrics 2012;129:S112-17. http://dx.doi.org/10.1542/peds.2012-0055C.
- Gamble C, Nadel S, Snape D, McKay A, Hickey H, Williamson P, et al. What parents of children who have received emergency care think about deferring consent in randomised trials of emergency treatments: postal survey. PLOS ONE 2012;7. http://dx.doi.org/10.1371/journal.pone.0035982.
- Molyneux S, Njue M, Boga M, Akello L, Olupot-Olupot P, Engoru C, et al. ‘The words will pass with the blowing wind’: Staff and parent views of the deferred consent process, with prior assent, used in an emergency fluids trial in two African hospitals. PLOS ONE 2013;8. http://dx.doi.org/10.1371/journal.pone.0054894.
- Brierley J, Larcher V. Emergency research in children: options for ethical recruitment. J Med Ethics 2011;37:429-32. http://dx.doi.org/10.1136/jme.2010.040667.
- Woolfall K, Young B, Frith L, Appleton R, Iyer A, Messahel S, et al. Doing challenging research studies in a patient-centred way: a qualitative study to inform a randomised controlled trial in the paediatric emergency care setting. BMJ Open 2014;4. http://dx.doi.org/10.1136/bmjopen-2014-005045.
- Jansen T, Kompanje EJ, Druml C, Menon D, Wiedermann C, Bakker J. Deferred consent in emergency intensive care research: what if the patient dies early? Use the data or not?. Intensive Care Med 2007;33:894-900. http://dx.doi.org/10.1007/s00134-007-0580-8.
- Davies H, Shakur H, Padkin A, Roberts I, Slowther A-M, Perkins GD. Guide to the design and review of emergency research when it is proposed that consent and consultation be waived. Emerg Med J 2014;31:794-5. http://dx.doi.org/10.1136/emermed-2014-203675.
- Maitland K, Molyneux S, Boga M, Kiguli S, Lang T. Use of deferred consent for severely ill children in a multi-centre Phase III trial. Trials 2011;12. http://dx.doi.org/10.1186/1745-6215-12-90.
- Macrae D, Grieve R, Allen E, Sadique Z, Morris K, Pappachan J, et al. A randomized trial of hyperglycemic control in pediatric intensive care. New Engl J Med 2014;370:107-18. http://dx.doi.org/10.1056/NEJMoa1302564.
- Ellenberg SS, Finkelstein DM, Schoenfeld DA. Statistical issues arising in AIDS clinical trials. JAMA 1992;87:562-9.
- Maitland K, Kiguli S, Opoka RO, Engoru C, Olupot-Olupot P, Akech SO, et al. Mortality after fluid bolus in African children with severe infection. New Engl J Med 2011;364:2483-95. http://dx.doi.org/10.1056/NEJMoa1101549.
- Cook DJ, Blythe D, Rischbieth A, Hebert PC, Zytaruk N, Menon K, et al. Enrollment of intensive care unit patients into clinical studies: A trinational survey of researchers’ experiences, beliefs, and practices. Crit Care Med 2008;36:2100-5. http://dx.doi.org/10.1097/CCM.0b013e31817c00b0.
- Harron K, Lee T, Ball T, Mok Q, Gamble C, Macrae D, et al. Making co-enrolment feasible for randomised controlled trials in paediatric intensive care. PLOS ONE 2012;7. http://dx.doi.org/10.1371/journal.pone.0041791.
- Myles PS, Williamson E, Oakley J, Forbes A. Ethical and scientific considerations for patient enrollment into concurrent clinical trials. Trials 2014;15. http://dx.doi.org/10.1186/1745-6215-15-470.
- Shilling V, Williamson PR, Hickey H, Sowden E, Beresford MW, Smyth RL, et al. Communication about children’s clinical trials as observed and experienced: qualitative study of parents and practitioners. PLOS ONE 2011;6. http://dx.doi.org/10.1371/journal.pone.0021604.
- Burgess E, Singhal N, Amin H, McMillan D, Devrome H, Fenton A. Consent for clinical research in the neonatal intensive care unit: a retrospective survey and a prospective study. Arch Dis Child Fetal Neonatal Ed 2003;88. http://dx.doi.org/10.1136/fn.88.4.F280.
- Morley C, Lau R, Davis P, Morse C. What do parents think about enrolling their premature babies in several research studies?. Arch Dis Child Fetal Neonatal Ed 2005;90. http://dx.doi.org/10.1136/adc.2004.061986.
- Harron K, Gamble C, Gilbert R. E-health data to support and enhance randomised controlled trials in the UK. Clinical Trials 2015;12:180-2. http://dx.doi.org/10.1177/1740774514562030.
- Ford I, Murray H, Packard CJ, Shepherd J, Macfarlane PW, Cobbe SM. Long-term follow-up of the West of Scotland Coronary Prevention Study. New Engl J Med 2007;357:1477-86. http://dx.doi.org/10.1056/NEJMoa065994.
- Rawlins M. A New Pathway for the Regulation and Governance of Health Research 2011. www.acmedsci.ac.uk/p47prid88.html (accessed 15 June 2012).
- Stephenson T. The medicines for children agenda in the UK. Br J Clin Pharmacol 2006;61:716-19. http://dx.doi.org/10.1111/j.1365-2125.2006.02676.x.
- NHS Act 2006. London: The Stationery Office; 2006.
- Health and Social Care Act 2001. London: The Stationery Office; 2001.
Appendix 1 Statistical analysis plan
Appendix 2 Clinical effectiveness study additional data
FIGURE 11.
Consolidated Standards of Reporting Trials (CONSORT) flow diagram for prospective consent. ITT, intention to treat.

FIGURE 12.
Consolidated Standards of Reporting Trials (CONSORT) flow diagram for deferred consent. ITT, intention to treat.
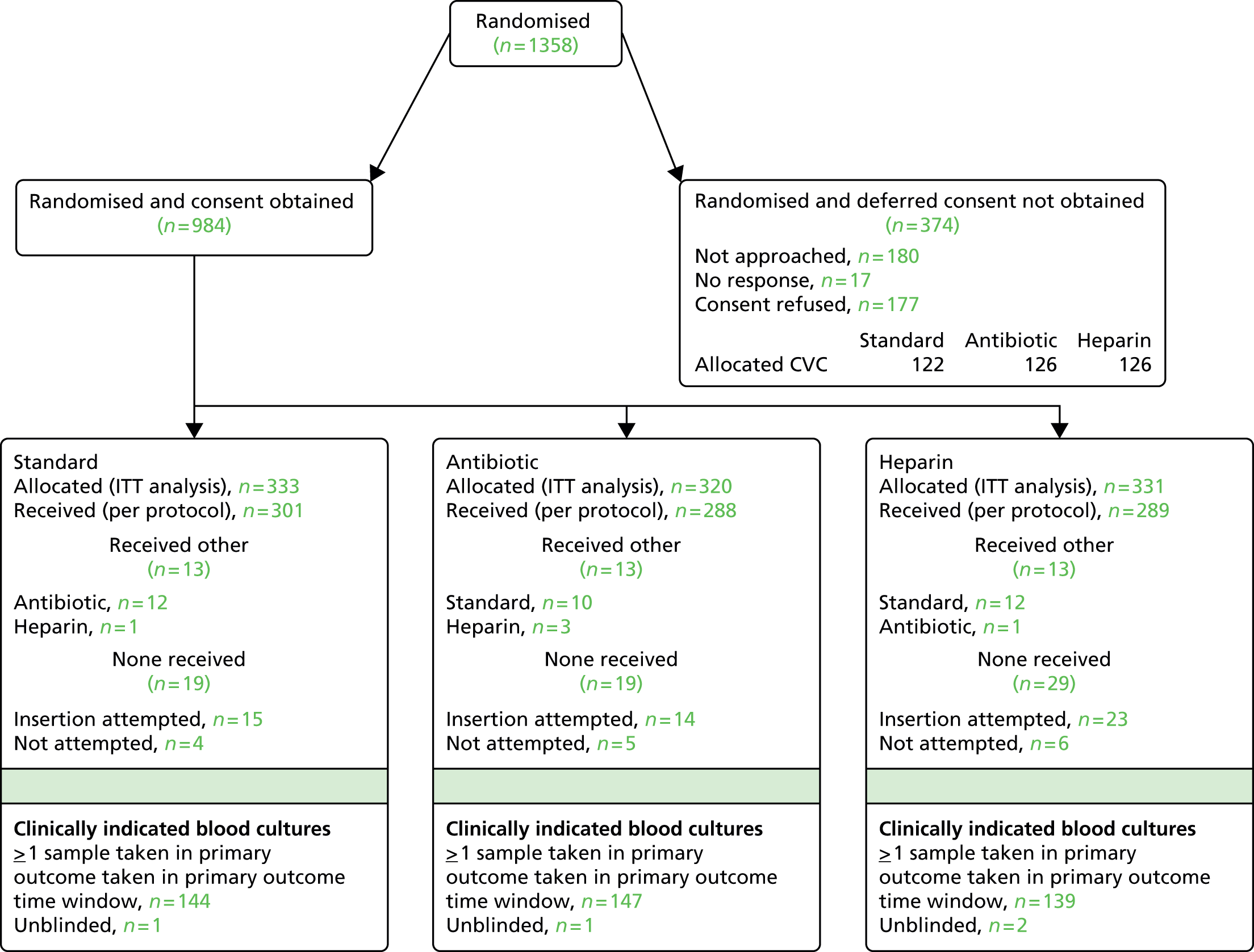
Centre | Date site initiated | Date of first randomisation | Target recruitment | Number randomised and consented | Prospective consent | Deferred consent |
---|---|---|---|---|---|---|
Great Ormond Street Hospital PICU/CICU | 10/02/2011 | 15/04/2011 | 200 | 362 | 27 | 335 |
Evelina London Children’s Hospital (Guy’s and St Thomas’) | 25/11/2010 | 06/01/2011 | 100 | 161 | 43 | 118 |
Royal Brompton Hospital | 17/06/2011 | 24/08/2011 | 100 | 49 | 29 | 20 |
St Mary’s Hospital, London | 01/02/2012 | 07/02/2012 | 100 | 26 | 0 | 26 |
Southampton General Hospital | 27/06/2011 | 11/07/2011 | 100 | 200 | 140 | 60 |
Bristol Royal Hospital for Children | 20/06/2011 | 24/06/2011 | 100 | 109 | 61 | 48 |
Alder Hey Children’s Hospital | 05/07/2011 | 11/07/2011 | 100 | 113 | 69 | 44 |
Birmingham Children’s Hospital | 22/08/2011 | 01/09/2011 | 100 | 150 | 34 | 116 |
Glenfield Hospital | 13/10/2011 | 22/10/2012 | 100 | 65 | 48 | 17 |
Leicester Royal Infirmary | 13/10/2011 | 11/01/2012 | 15 | 3 | 12 | |
Royal Victoria Infirmary | 25/01/2012 | 03/02/2012 | 50 | 41 | 0 | 41 |
Freeman Hospital | 26/01/2012 | 10/02/2012 | 18 | 13 | 5 | |
Leeds General Infirmary | 14/12/2010 | 22/12/2010 | 100 | 149 | 32 | 117 |
Queen’s Medical Centre | 11/05/2012 | 16/05/2012 | 50 | 27 | 2 | 25 |
Total | 1200 | 1485 | 501 | 984 |
FIGURE 13.
Recruitment by month.

Centre | Standard (n = 502) | Antibiotic (n = 486) | Heparin (n = 497) | Total (n = 1485) | ||||||||
---|---|---|---|---|---|---|---|---|---|---|---|---|
Total randomised and consented | Patients sampled (no. of samples) | % | Total randomised and consented | Patients sampled (no. of samples) | % | Total randomised and consented | Patients sampled (no. of samples) | % | Total randomised and consented | Patients sampled (no. of samples) | % | |
Great Ormond Street Hospital PICU/CICU | 128 | 106 (273) | 82.8 | 117 | 90 (260) | 76.9 | 117 | 96 (304) | 82.1 | 362 | 292 (837) | 80.7 |
Evelina London Children’s Hospital (Guy’s and St Thomas) | 54 | 49 (163) | 90.7 | 50 | 40 (110) | 80.0 | 57 | 50 (123) | 87.7 | 161 | 139 (396) | 86.3 |
Royal Brompton Hospital | 15 | 11 (29) | 73.3 | 17 | 11 (29) | 64.7 | 17 | 13 (46) | 76.5 | 49 | 35 (104) | 71.4 |
St Mary’s Hospital, London | 7 | 5 (10) | 71.4 | 10 | 9 (21) | 90.0 | 9 | 9 (31) | 100.0 | 26 | 23 (62) | 88.5 |
Southampton General Hospital | 65 | 53 (162) | 81.5 | 66 | 63 (143) | 95.5 | 69 | 63 (162) | 91.3 | 200 | 179 (467) | 89.5 |
Bristol Royal Hospital for Children | 35 | 29 (71) | 82.9 | 39 | 34 (91) | 87.2 | 35 | 33 (88) | 94.3 | 109 | 96 (250) | 88.1 |
Alder Hey Children’s Hospital | 41 | 33 (94) | 80.5 | 36 | 29 (62) | 80.6 | 36 | 28 (87) | 77.8 | 113 | 90 (243) | 79.6 |
Birmingham Children’s Hospital | 50 | 41 (128) | 82.0 | 48 | 32 (124) | 66.7 | 52 | 34 (123) | 65.4 | 150 | 107 (375) | 71.3 |
Glenfield Hospital | 21 | 15 (42) | 71.4 | 22 | 14 (27) | 63.6 | 22 | 13 (31) | 59.1 | 65 | 42 (100) | 64.6 |
Leicester Royal Infirmary | 6 | 4 (8) | 66.7 | 4 | 3 (5) | 75.0 | 5 | 5 (16) | 100.0 | 15 | 12 (29) | 80.0 |
Royal Victoria Infirmary | 13 | 13 (44) | 100.0 | 14 | 13 (45) | 92.9 | 14 | 13 (44) | 92.9 | 41 | 39 (133) | 95.1 |
Freeman Hospital | 7 | 5 (14) | 71.4 | 5 | 3 (9) | 60.0 | 6 | 4 (10) | 66.7 | 18 | 12 (33) | 66.7 |
Leeds General Infirmary | 50 | 44 (151) | 88.0 | 48 | 41 (156) | 85.4 | 51 | 46 (187) | 90.2 | 149 | 131 (494) | 87.9 |
Queen’s Medical Centre | 10 | 7 (26) | 70.0 | 10 | 7 (21) | 70.0 | 7 | 5 (13) | 71.4 | 27 | 19 (60) | 70.4 |
Total | 502 | 415 (1215) | 82.7 | 486 | 389 (1103) | 80.0 | 497 | 412 (1265) | 82.9 | 1485 | 1216 (3583) | 81.9 |
Threats to validity | Standard (n = 502) | Antibiotic (n = 486) | Heparin (n = 497) | Total (n = 1485) | ||||
---|---|---|---|---|---|---|---|---|
n | % | n | % | n | % | n | % | |
CVC inserted | 481 | 95.8 | 465 | 95.7 | 464 | 93.4 | 1410 | 94.9 |
Internal validity | ||||||||
Randomised multiple times | 15 | 3.0 | 12 | 2.5 | 11 | 2.2 | 38 | 2.6 |
Clinical indication 48 hours after randomisation, no sample taken in primary outcome time windowa | 183 | 38.0 | 196 | 42.2 | 196 | 42.2 | 575 | 40.8 |
External validity | ||||||||
Child aged > 16 years | 2 | 0.4 | 4 | 0.8 | 0 | 0.0 | 6 | 0.4 |
CVC inserted but removed before 48 hoursa,b | 94 | 19.5 | 96 | 20.6 | 96 | 20.7 | 286 | 20.3 |
CVC inserted > 12 hours after randomisationa | 1 | 0.2 | 1 | 0.2 | 4 | 0.9 | 6 | 0.4 |
Line not required following randomisation (post 12 hours); randomisation pack returned to the CTU | ||||||||
CVC attempted but not inserted | 15 | 3.0 | 14 | 2.9 | 24 | 4.8 | 53 | 3.6 |
CVC insertion not attempted | 6 | 1.2 | 7 | 1.4 | 9 | 1.8 | 22 | 1.5 |
Incorrect randomisation envelope used | 4 | 0.8 | 8 | 1.6 | 9 | 1.8 | 21 | 1.4 |
FIGURE 14.
Samples contributing to the primary outcome. a, The non-skin organism was from a sample taken at 47 hours and 55 minutes after randomisation. Shading indicates whether samples contributing to the primary outcome included skin organisms (dark), non-skin organisms (light) or both skin and non-skin organisms (medium). POTW, primary outcome time window.

Group | Total randomised | Primary outcome | Clinical indication but no sample taken in time window | Total included in sensitivity analysis | HR vs. standarda (95% CI) | p-value | |||
---|---|---|---|---|---|---|---|---|---|
n | % | n | % | n | % | ||||
Standard | 502 | 18 | 3.6 | 8 | 1.6 | 26 | 5.2 | ||
Any impregnated | 983 | 24 | 2.4 | 9 | 0.9 | 33 | 3.4 | 0.67 (0.39 to 1.15) | 0.15 |
Antibiotic | 486 | 7 | 1.4 | 6 | 1.2 | 13 | 2.6 | 0.54 (0.29 to 1.02) | 0.06 |
Heparin | 497 | 17 | 3.4 | 3 | 0.6 | 20 | 4.1 | 0.83 (0.47 to 1.49) | 0.54 |
Total | 1485 | 42 | 2.8 | 17 | 1.1 | 59 | 4.0 |
Indicator | Standard (n = 502) | Antibiotic (n = 486) | Heparin (n = 497) | Total (n = 1485) | ||||
---|---|---|---|---|---|---|---|---|
n | % | n | % | n | % | n | % | |
Primary outcome only | 2 | 0.4 | 0 | 0.0 | 4 | 0.8 | 6 | 0.4 |
Clinical indication, blood culture, plus | ||||||||
Bacterial DNA detected by PCR only | 2 | 0.4 | 1 | 0.2 | 1 | 0.2 | 4 | 0.3 |
Change in antibiotic on the same day or next day only | 79 | 15.7 | 71 | 14.6 | 64 | 12.9 | 214 | 14.4 |
CVC removal for infection only | 6 | 1.2 | 12 | 2.5 | 7 | 1.4 | 25 | 1.7 |
Primary outcome and CVC removal for infection | 1 | 0.2 | 0 | 0.0 | 0 | 0.0 | 1 | 0.1 |
Primary outcome and antibiotic change | 8 | 1.6 | 6 | 1.2 | 6 | 1.2 | 20 | 1.3 |
CVC removal for infection and antibiotic change | 7 | 1.4 | 11 | 2.3 | 13 | 2.6 | 31 | 2.1 |
PCR positive and antibiotic change | 1 | 0.2 | 1 | 0.2 | 0 | 0.0 | 2 | 0.1 |
Primary outcome, CVC removal for infection and antibiotic change | 6 | 1.2 | 1 | 0.2 | 6 | 1.2 | 13 | 0.9 |
CVC removal for infection, PCR positive and antibiotic change | 0 | 0.0 | 0 | 0.0 | 0 | 0.0 | 0 | 0.0 |
Primary outcome, bacterial DNA detected by PCR, CVC removal for infection and antibiotic change | 1 | 0.2 | 0 | 0.0 | 1 | 0.2 | 2 | 0.1 |
Totala | 113 | 22.5 | 103 | 21.2 | 102 | 20.5 | 318 | 21.4 |
Organism group | Organism | Standard | Antibiotic | Heparin | Total |
---|---|---|---|---|---|
Gram positive | Staphylococcus aureus | 1 | 1 | 3 | 5 |
Streptococcus spp. | 1 | 0 | 0 | 1 | |
Methicillin-resistant S. aureus | 1 | 0 | 0 | 1 | |
Enterococcus spp. | 2 | 0 | 4 | 6 | |
Streptococcus mitis | 1 | 0 | 1 | 2 | |
Streptococcus parasanguis and Streptococcus salivarius | 0 | 1 | 0 | 1 | |
Gram negative | Serratia marcescens | 1 | 1 | 0 | 2 |
Pseudomonas aeruginosa | 2 | 1 | 1 | 4 | |
Gram-negative bacillus | 1 | 0 | 1 | 2 | |
Escherichia coli | 0 | 1 | 0 | 1 | |
E. coli and coliform | 0 | 1 | 0 | 1 | |
Coliform | 1 | 0 | 0 | 1 | |
Klebsiella spp. | 0 | 0 | 1 | 1 | |
Cellulomas spp. | 0 | 0 | 1 | 1 | |
Raoultella panticola and Enterobacter spp. | 1 | 0 | 0 | 1 | |
Gram positive and Gram negative | Enterococcus spp. and Klebsiella pneumoniae | 0 | 0 | 1 | 1 |
Fungi | Candida spp. | 2 | 0 | 2 | 4 |
Candida albicans | 0 | 0 | 1 | 1 | |
Skin bacteria and Gram positive | Coagulase-negative staphylococcus and enterococcus spp. | 1 | 0 | 0 | 1 |
Skin bacteria | Coagulase-negative staphylococcus spp. | 3 | 1 | 1 | 5 |
Total | 18 | 7 | 17 | 42 |
Related/unrelated | Cause of death | Standard (n = 533) | Antibiotic (n = 451) | Heparin (n = 479) | Total (n = 1463) | ||||
---|---|---|---|---|---|---|---|---|---|
n | % | n | % | n | % | n | % | ||
Unrelated | Related to comorbidities at admission | 58 | 10.9 | 37 | 8.2 | 35 | 7.3 | 130 | 8.9 |
Cerebral haemorrhage | 0 | 0.0 | 0 | 0.0 | 1 | 0.2 | 1 | 0.1 | |
Multiorgan failure as a result of calcification of the arteries | 1 | 0.2 | 0 | 0.0 | 0 | 0.0 | 1 | 0.1 | |
Pneumonitis and multiorgan failure | 1 | 0.2 | 0 | 0.0 | 1 | 0.2 | 2 | 0.1 | |
Pseudomonas septicaemia second to peritonitis | 0 | 0.0 | 1 | 0.2 | 0 | 0.0 | 1 | 0.1 | |
Severe birth asphyxia | 1 | 0.2 | 0 | 0.0 | 0 | 0.0 | 1 | 0.1 | |
Complication of treatment | 1 | 0.2 | 0 | 0.0 | 0 | 0.0 | 1 | 0.1 | |
Cerebral bleeding by ventricular assist device | 1 | 0.2 | 0 | 0.0 | 0 | 0.0 | 1 | 0.1 | |
Group B streptococcus infection/sepsis | 1 | 0.2 | 0 | 0.0 | 0 | 0.0 | 1 | 0.1 | |
Multiorgan failure and systemic inflammatory response syndrome | 0 | 0.0 | 0 | 0.0 | 1 | 0.2 | 1 | 0.1 | |
Multiorgan failure | 0 | 0.0 | 1 | 0.2 | 0 | 0.0 | 1 | 0.1 | |
Exact cause not known | 0 | 0.0 | 1 | 0.2 | 0 | 0.0 | 1 | 0.1 | |
Pulmonary haemorrhage | 1 | 0.2 | 0 | 0.0 | 0 | 0.0 | 1 | 0.1 | |
Unlikely to be related | Related to comorbidities at admission | 0 | 0.0 | 2 | 0.4 | 0 | 0.0 | 2 | 0.1 |
Missing | 1 | 0.2 | 2 | 0.4 | 0 | 0.0 | 3 | 0.2 | |
Total | 66 | 12.4 | 44 | 9.8 | 38 | 7.9 | 148 | 10.1 |
CVC allocation | Organism | Etest result | |
---|---|---|---|
Minocycline | Rifampicin | ||
Standard | Coliform bacilli | Resistant | Resistant |
Enterococcus faecalis | Resistant | Resistant | |
Serratia marcescens | Resistant | Resistant | |
Staphylococcus aureus | Sensitive | Sensitive | |
Methicillin-resistant S. aureus | Sensitive | Sensitive | |
Antibiotic | Escherichia coli | Resistant | Resistant |
Staphylococcus spp. | Resistant | Resistant | |
Heparin | Klebsiella pneumoniae | Resistant | Resistant |
K. pneumoniae | Resistant | Resistant | |
S. aureus | Sensitive | Sensitive | |
Coagulase-negative staphylococcus spp. | Sensitive | Sensitive | |
Enterococcus hirae and coagulase-negative staphylococcus spp. | Resistant, sensitive | Sensitive, resistant |
Appendix 3 Cost-effectiveness study additional data
HRG | HRG name (inpatient) | Elective spell tariff (£) | Elective long-stay trim point (days) | Non-elective spell tariff (£) | Non-elective long-stay trim point (days) | Per-day long-stay payment (for days exceeding trim point) (£) |
---|---|---|---|---|---|---|
LB08Zb | Ureter Major Endoscopic Procedure | 1379.00 | 5 | 2468.00 | 12 | 301.00 |
PA03Zb | Febrile Convulsions | 928.00 | 1 | 661.00 | 3 | 304.00 |
PA14Ab | Lower Respiratory Tract Disorders without Acute Bronchiolitis with CC | 3215.00 | 13 | 2473.00 | 14 | 222.00 |
CZ06Nb | Minor Throat Procedures with CC | 1431.00 | 3 | 3222.00 | 24 | 281.00 |
FZ03Bc | Diagnostic and Intermediate Procedures on the Upper GI Tract ≤ 18 Years | 852.00 | 5 | 1267.00 | 5 | 223.00 |
GB04Ac | Endoscopic/Radiology Category 1 with Major CC | 1879.00 | 8 | 6347.00 | 54 | 228.00 |
AA16Z | Intracranial Procedures except Trauma with Non-Transient Stroke or Cerebrovascular Accident, Nervous System Infections or Encephalopathy – Category 1 or 2 | 4255.00 | 24 | 7371.00 | 66 | 210.00 |
EA24Z | Complex Congenital Surgery | 9631.00 | 21 | 14,934.00 | 46 | 205.00 |
EA25Z | Intermediate Congenital Surgery | 9571.00 | 19 | 13,009.00 | 58 | 205.00 |
PA14C | Lower Respiratory Tract Disorders without Acute Bronchiolitis with Length of Stay ≥ 1 Day with CC | 3602.00 | 22 | 2301.00 | 15 | 291.00 |
DZ07B | Fibreoptic Bronchoscopy ≤ 18 Years | 1146.00 | 5 | 1394.00 | 5 | 190.00 |
VA11D | Multiple Trauma Diagnoses Score ≥ 51 with Interventions Score 1–8 | 5246.00 | 94 | 5246.00 | 94 | 232.00 |
PA16A | Major Infections with CC | 1719.00 | 8 | 2856.00 | 22 | 291.00 |
QZ15B | Therapeutic Endovascular Procedures with Intermediate CC | 1523.00 | 5 | 5389.00 | 49 | 227.00 |
DZ37B | Non-Invasive Ventilation Support Assessment ≤ 18 Years | 927.00 | 5 | 927.00 | 5 | 190.00 |
PA23B | Cardiac Conditions without CC | 1420.00 | 5 | 1427.00 | 5 | 291.00 |
PA15B | Acute Bronchiolitis without CC | 1066.00 | 8 | 910.00 | 8 | 291.00 |
PA23A | Cardiac Conditions with CC | 1956.00 | 5 | 3638.00 | 16 | 291.00 |
EA23Z | Major Complex Congenital Surgery | 12,638.00 | 36 | 19,436.00 | 66 | 205.00 |
PA14E | Lower Respiratory Tract Disorders without Acute Bronchiolitis with Length of Stay 0 Days | 561.00 | 5 | 434.00 | 5 | 291.00 |
FZ11A | Large Intestine – Major Procedures with Major CC | 5441.00 | 33 | 8053.00 | 68 | 228.00 |
PA63B | Head, Neck and Ear Disorders with Length of Stay ≥ 1 Day with CC | 2514.00 | 9 | 972.00 | 6 | 291.00 |
PA48A | Blood Cell Disorders with CC | 1474.00 | 8 | 2335.00 | 10 | 291.00 |
PB02Z | Minor Neonatal Diagnoses | 1041.00 | 14 | 1041.00 | 14 | 291.00 |
FZ07A | Major Small Intestine Procedures with CC | 4569.00 | 19 | 8028.00 | 62 | 228.00 |
VA15D | Multiple Trauma Diagnoses Score ≥ 51 with Interventions Score ≥ 45 | 20,844.00 | 143 | 20,844.00 | 143 | 232.00 |
HC12Z | Intradural Spine Minor 1 | 571.00 | 5 | 739.00 | 5 | 231.00 |
PA12Z | Asthma or Wheezing | 563.00 | 5 | 622.00 | 5 | 291.00 |
FZ06A | Very Major Small Intestine Procedures with CC | 7781.00 | 40 | 8308.00 | 63 | 228.00 |
PA25B | Major Gastrointestinal or Metabolic Disorders without CC | 949.00 | 5 | 1177.00 | 8 | 291.00 |
AA21Z | Intracranial Procedures except Trauma with Other Diagnoses – Category 1 or 2 | 1096.00 | 5 | 5346.00 | 42 | 210.00 |
HB99Z | Other Procedures for Non Trauma | 331.00 | 5 | 331.00 | 5 | 231.00 |
GA05B | Hepatobiliary Procedures Category 5 without CC | 5598.00 | 17 | 5980.00 | 35 | 221.00 |
PA47Z | Sickle-Cell Anaemia with Crisis | 494.00 | 8 | 1587.00 | 9 | 291.00 |
QZ15A | Therapeutic Endovascular Procedures with Major CC | 9835.00 | 86 | 10,258.00 | 107 | 227.00 |
AA02Z | Intracranial Procedures for Trauma with Intermediate Diagnosis | 6738.00 | 40 | 6738.00 | 40 | 210.00 |
EA26Z | Standard Congenital Surgery | 5615.00 | 15 | 5615.00 | 15 | 205.00 |
QZ14A | Vascular Access except for Renal Replacement Therapy with CC | 548.00 | 5 | 1353.00 | 8 | 227.00 |
PA02A | Epilepsy Syndrome with CC | 1043.00 | 5 | 942.00 | 5 | 291.00 |
PA15A | Acute Bronchiolitis with CC | 2254.00 | 15 | 1962.00 | 14 | 291.00 |
PA25A | Major Gastrointestinal or Metabolic Disorders with CC | 1715.00 | 5 | 2583.00 | 14 | 291.00 |
QZ13A | Vascular Access for Renal Replacement Therapy with CC | 1287.00 | 5 | 1571.00 | 8 | 227.00 |
VA14D | Multiple Trauma Diagnoses Score ≥ 51 with Interventions Score 30–44 | 11,259.00 | 129 | 11,259.00 | 129 | 232.00 |
DZ06Z | Minor Thoracic Procedures | 729.00 | 5 | 1063.00 | 5 | 190.00 |
DZ03A | Major Thoracic Procedures with CC | 3371.00 | 14 | 6985.00 | 39 | 190.00 |
AA20Z | Intracranial Procedures except Trauma with Muscular, Balance, Cranial or Peripheral Nerve Disorders or Epilepsy – Category 1 or 2 | 1957.00 | 10 | 3883.00 | 32 | 210.00 |
CZ07O | Exteriorisation of Trachea with Major CC | 8640.00 | 98 | 7363.00 | 95 | 250.00 |
PB01Z | Major Neonatal Diagnoses | 1511.00 | 16 | 1511.00 | 16 | 291.00 |
HB16B | Minor Hip Procedures for Non Trauma Category 1 with CC | 1267.00 | 33 | 1267.00 | 33 | 231.00 |
FZ05A | Major Stomach or Duodenum Procedures ≥ 2 Years with CC | 3591.00 | 16 | 6539.00 | 57 | 228.00 |
FZ05C | Major Stomach or Duodenum Procedures ≤ 1 Year | 5402.00 | 16 | 4582.00 | 11 | 228.00 |
PA44Z | Neoplasm Diagnoses with Length of Stay 0 Days | 541.00 | 5 | 527.00 | 5 | 291.00 |
FZ12A | General Abdominal – Very Major or Major Procedures with Major CC | 5070.00 | 29 | 6963.00 | 54 | 228.00 |
PA18A | Minor Infections with CC | 843.00 | 5 | 1204.00 | 8 | 291.00 |
AA09Z | Intracranial Procedures except Trauma with Other Diagnoses – Category 4 | 2396.00 | 5 | 8293.00 | 49 | 210.00 |
HB63Z | Minor Shoulder and Upper Arm Procedures for Non Trauma | 1401.00 | 5 | 1401.00 | 5 | 231.00 |
EA20Z | Other Complex Cardiac Surgery and Re-do’s | 10,511.00 | 26 | 12,806.00 | 57 | 205.00 |
EA14Z | Coronary Artery Bypass Graft (First Time) | 7358.00 | 16 | 9055.00 | 39 | 205.00 |
EA12Z | Implantation of Cardioverter – Defibrillator Only | 5556.00 | 5 | 7248.00 | 34 | 205.00 |
DZ02A | Complex Thoracic Procedures with Major CC | 8271.00 | 31 | 9426.00 | 54 | 190.00 |
VA11B | Multiple Trauma Diagnoses Score 24–32 with Interventions Score 1–8 | 3864.00 | 24 | 3864.00 | 24 | 232.00 |
PA26A | Other Gastrointestinal or Metabolic Disorders with CC | 1603.00 | 5 | 1076.00 | 5 | 291.00 |
PA59C | Major Congenital Conditions under 1 Year with CC | 2444.00 | 8 | 3609.00 | 31 | 291.00 |
PA59E | Major Congenital Conditions ≥ 1 Year with CC | 1148.00 | 5 | 3142.00 | 13 | 291.00 |
PA08B | Intermediate Injury without Intracranial Injury without CC | 790.00 | 5 | 757.00 | 5 | 291.00 |
PA28A | Feeding Difficulties and Vomiting with CC | 2136.00 | 10 | 1012.00 | 5 | 291.00 |
HB23B | Intermediate Knee Procedures for Non Trauma with CC | 2342.00 | 29 | 2342.00 | 29 | 231.00 |
PA19B | Viral Infections with Length of Stay ≥ 2 Days | 1255.00 | 5 | 1255.00 | 5 | 291.00 |
PA06Z | Head Injury with Intracranial Injury | 1689.00 | 9 | 1689.00 | 9 | 291.00 |
PA67Z | Diabetes Mellitus with Ketoacidosis or Coma | 954.00 | 6 | 954.00 | 6 | 291.00 |
PA45Z | Febrile Neutropenia with Malignancy | 8858.00 | 51 | 3894.00 | 13 | 291.00 |
LB10Z | Bladder Major Open Procedures/Reconstruction | 5348.00 | 24 | 7019.00 | 52 | 215.00 |
PA17A | Intermediate Infections with CC | 1067.00 | 5 | 1274.00 | 9 | 291.00 |
PA03B | Febrile Convulsions ≥ 1 Year | 705.00 | 5 | 595.00 | 5 | 291.00 |
QZ05A | Miscellaneous Vascular Procedures with CC | 1687.00 | 5 | 3733.00 | 30 | 227.00 |
VA13D | Multiple Trauma Diagnoses Score ≥ 51 with Interventions Score 19–29 | 8858.00 | 117 | 8858.00 | 117 | 232.00 |
AA10Z | Intracranial Procedures except Trauma with Non-Transient Stroke or Cerebrovascular Accident, Nervous System Infections or Encephalopathy – Category 3 | 7598.00 | 74 | 11,733.00 | 74 | 210.00 |
JC01A | Major Multiple Skin Procedures with Major CC | 9610.00 | 62 | 9608.00 | 79 | 223.00 |
GA05A | Hepatobiliary Procedures Category 5 with CC | 6767.00 | 26 | 7488.00 | 57 | 221.00 |
EA52Z | Repair or Replacement of More Than One Heart Valve | 12,196.00 | 31 | 15,633.00 | 84 | 205.00 |
DZ03B | Major Thoracic Procedures without CC | 2429.00 | 9 | 3884.00 | 20 | 190.00 |
QZ04Z | Extracranial or Upper Limb Arterial Surgery | 3567.00 | 7 | 5606.00 | 34 | 227.00 |
EA39Z | Pacemaker Procedure without Generator Implant (Includes Resiting and Removal of Cardiac Pacemaker System) | 2748.00 | 5 | 5302.00 | 33 | 205.00 |
PA38D | Renal Disease with Renal Failure with Length of Stay ≥ 1 Day | 3800.00 | 9 | 3184.00 | 15 | 291.00 |
PA07B | Head Injury without Intracranial Injury without CC | 539.00 | 5 | 506.00 | 5 | 291.00 |
VA10D | Multiple Trauma Diagnoses Score ≥ 51 with No Interventions | 3712.00 | 93 | 3712.00 | 93 | 232.00 |
PA21A | Infectious and Non-Infectious Gastroenteritis with CC | 1855.00 | 8 | 810.00 | 5 | 291.00 |
PA16B | Major Infections without CC | 696.00 | 5 | 1659.00 | 11 | 291.00 |
FZ01C | Complex Oesophageal Procedures ≤ 18 Years | 14,175.00 | 63 | 14,175.00 | 63 | 228.00 |
QZ01A | Aortic or Abdominal Surgery with CC | 6487.00 | 27 | 7307.00 | 53 | 227.00 |
HB13Z | Intermediate Hip Procedures for Non Trauma Category 2 | 5194.00 | 26 | 5194.00 | 26 | 231.00 |
HC08Z | Intradural Spine Major 1 | 4992.00 | 31 | 4992.00 | 31 | 231.00 |
AA11Z | Intracranial Procedures except Trauma with Haemorrhagic Cerebrovascular Disorders – Category 3 | 6166.00 | 50 | 8917.00 | 50 | 210.00 |
PA01A | Nervous System Disorders with CC | 1146.00 | 5 | 2368.00 | 15 | 291.00 |
PA34A | Musculoskeletal or Connective Tissue Disorders with CC | 1112.00 | 5 | 1246.00 | 8 | 291.00 |
LB02D | Kidney Major Open Procedure ≤ 18 Years | 4289.00 | 7 | 8972.00 | 36 | 215.00 |
HB16C | Minor Hip Procedures for Non Trauma Category 1 without CC | 969.00 | 5 | 969.00 | 5 | 231.00 |
VA12D | Multiple Trauma Diagnoses Score ≥ 51 with Interventions Score 9–18 | 7012.00 | 102 | 7012.00 | 102 | 232.00 |
CZ01S | Minor Mouth or Throat Procedures ≤ 18 Years with CC | 1551.00 | 5 | 3137.00 | 8 | 250.00 |
HB14B | Intermediate Hip Procedures for Non Trauma Category 1 with CC | 3509.00 | 61 | 3509.00 | 61 | 231.00 |
PA63A | Head, Neck and Ear Disorders with Length of Stay 0 Days | 540.00 | 5 | 383.00 | 5 | 291.00 |
PA60C | Other Congenital Conditions under 1 Year with CC | 945.00 | 5 | 1336.00 | 9 | 291.00 |
VA10C | Multiple Trauma Diagnoses Score 33–50 with No Interventions | 3453.00 | 47 | 3453.00 | 47 | 232.00 |
HB15F | Minor Hip Procedures for Non Trauma Category 2 ≤ 18 Years with CC | 1725.00 | 23 | 1725.00 | 23 | 231.00 |
FZ20C | Appendicectomy Procedures ≤ 18 Years | 2367.00 | 5 | 2292.00 | 7 | 228.00 |
FZ27D | Endoscopic or Intermediate General Abdominal Procedures ≤ 18 Years | 1216.00 | 5 | 1729.00 | 8 | 228.00 |
LA05Z | Renal Replacement Peritoneal Dialysis Associated Procedures | 1138.00 | 5 | 1195.00 | 5 | 215.00 |
LB05D | Kidney Intermediate, Endoscopic and Percutaneous Interventions ≤ 18 Years | 2372.00 | 5 | 4973.00 | 25 | 215.00 |
AA04Z | Intracranial Procedures except Trauma with Non-Transient Stroke or Cerebrovascular Accident, Nervous System Infections or Encephalopathy – Category 4 | 7936.00 | 74 | 11,733.00 | 74 | 210.00 |
PA19A | Viral Infections with Length of Stay ≤ 1 Day | 446.00 | 5 | 444.00 | 5 | 291.00 |
PA21B | Infectious and Non-Infectious Gastroenteritis without CC | 705.00 | 5 | 520.00 | 5 | 291.00 |
EA11Z | Percutaneous Congenital Interventions: Other Including Septostomy, Embolisations, Non-Coronary Stents and Energy Moderated Perforation | 1934.00 | 5 | 4417.00 | 33 | 205.00 |
FZ25B | Therapeutic Endoscopic or Intermediate Stomach or Duodenum Procedures ≤ 18 Years | 996.00 | 5 | 996.00 | 5 | 228.00 |
FZ04A | Very Major Stomach or Duodenum Procedures with Major CC | 8135.00 | 44 | 11,299.00 | 84 | 228.00 |
AB04Z | Major Pain Procedures | 570.00 | 5 | 2624.00 | 24 | 210.00 |
LB11B | Urinary Diversion without Cystectomy without Malignancy | 5913.00 | 30 | 9562.00 | 71 | 215.00 |
VA12C | Multiple Trauma Diagnoses Score 33–50 with Interventions, Score 9–18 | 6487.00 | 61 | 6487.00 | 61 | 232.00 |
VA11A | Multiple Trauma Diagnoses Score ≤ 23 with Interventions, Score 1–8 | 1965.00 | 10 | 1965.00 | 10 | 232.00 |
SA14Z | Plasma Exchanges 2–9 | 2385.00 | 13 | 7293.00 | 55 | 237.00 |
AA19Z | Intracranial Procedures except Trauma with Cerebral Degenerations or Miscellaneous Disorders of Nervous System – Category 1 or 2 | 1843.00 | 8 | 5014.00 | 25 | 210.00 |
VA11C | Multiple Trauma Diagnoses Score 33–50 with Interventions Score 1–8 | 4513.00 | 45 | 4513.00 | 45 | 232.00 |
PB03Z | Healthy Baby | 0.00 | 5 | 0.00 | 5 | 0.00 |
PA49Z | Coagulation Disorders | 666.00 | 5 | 691.00 | 5 | 291.00 |
PA35A | Skin Disorders with CC | 1103.00 | 5 | 1187.00 | 8 | 291.00 |
PA42Z | Brain Tumours with Length of Stay ≥ 1 Day | 2660.00 | 9 | 2830.00 | 9 | 291.00 |
GB03A | Endoscopic/Radiology Category 2 with CC | 1020.00 | 5 | 6235.00 | 53 | 221.00 |
GB01Z | Endoscopic/Radiology Category 4 | 2366.00 | 9 | 4813.00 | 58 | 221.00 |
PA68Z | Diabetes Mellitus without Ketoacidosis or Coma | 941.00 | 5 | 941.00 | 5 | 291.00 |
QZ15C | Therapeutic Endovascular Procedures without CC | 1178.00 | 5 | 3091.00 | 26 | 227.00 |
SA15Z | Plasma Exchanges 10–19 | 4892.00 | 13 | 7293.00 | 55 | 237.00 |
PA51Z | Child Safeguarding (Welfare and Protection) | 854.00 | 5 | 854.00 | 5 | 291.00 |
GA03A | Hepatobiliary Procedures Category 7 with CC | 10,784.00 | 49 | 14,235.00 | 71 | 221.00 |
DZ02B | Complex Thoracic Procedures with CC | 6356.00 | 18 | 6729.00 | 26 | 190.00 |
PA14D | Lower Respiratory Tract Disorders without Acute Bronchiolitis with Length of Stay ≥ 1 Day without CC | 2286.00 | 16 | 1035.00 | 6 | 291.00 |
CZ04Q | Complex Major Mouth or Throat Procedures without CC | 7565.00 | 39 | 7565.00 | 39 | 250.00 |
AA17Z | Intracranial Procedures except Trauma with Haemorrhagic Cerebrovascular Disorders – Category 1 or 2 | 2794.00 | 12 | 6852.00 | 64 | 210.00 |
QZ11B | Amputations without Major CC | 8011.00 | 53 | 10,771.00 | 95 | 227.00 |
HC11Z | Intradural Spine Minor 2 | 3138.00 | 18 | 3138.00 | 18 | 231.00 |
EA10Z | Percutaneous Congenital Interventions: Balloon Valve Intermediate Interventions and Arterial Duct Closure | 4111.00 | 6 | 8275.00 | 48 | 205.00 |
FZ02Z | Very Major Oesophageal Procedures | 3802.00 | 11 | 6158.00 | 39 | 228.00 |
FZ11B | Large Intestine – Major Procedures without Major CC | 2643.00 | 14 | 4640.00 | 32 | 228.00 |
AA15Z | Intracranial Procedures except Trauma with Other Diagnoses – Category 3 | 2396.00 | 5 | 8293.00 | 49 | 210.00 |
QZ05B | Miscellaneous Vascular Procedures without CC | 1035.00 | 5 | 2402.00 | 14 | 227.00 |
EA43Z | Implantation of Prosthetic Heart or Ventricular Assist Device | 42,583.00 | 90 | 42,583.00 | 90 | 205.00 |
FZ07B | Major Small Intestine Procedures without CC | 3134.00 | 15 | 4551.00 | 28 | 228.00 |
Treatment function | Treatment function name (outpatient) | WF01B First Attendance – Single Professional (£) | WF02B First Attendance – Multi Professional (£) | WF01A Follow Up Attendance – Single Professional (£) | WF02A Follow Up Attendance – Multi Professional (£) |
---|---|---|---|---|---|
100 | General Surgery | 191.00 | 207.00 | 101.00 | 101.00 |
101 | Urology | 177.00 | 196.00 | 96.00 | 99.00 |
103 | Breast Surgery | 154.00 | 154.00 | 84.00 | 85.00 |
104 | Colorectal Surgery | 131.00 | 157.00 | 72.00 | 105.00 |
105 | Hepatobiliary and Pancreatic Surgery | 166.00 | 166.00 | 102.00 | 102.00 |
106 | Upper Gastrointestinal Surgery | 140.00 | 140.00 | 82.00 | 82.00 |
107 | Vascular Surgery | 234.00 | 234.00 | 116.00 | 116.00 |
110 | Trauma and Orthopaedics | 137.00 | 137.00 | 83.00 | 83.00 |
120 | Ear, Nose and Throat | 114.00 | 141.00 | 63.00 | 73.00 |
130 | Ophthalmology | 115.00 | 138.00 | 67.00 | 75.00 |
140 | Oral Surgery | 130.00 | 185.00 | 80.00 | 126.00 |
143 | Orthodontics | 186.00 | 285.00 | 83.00 | 129.00 |
144 | Maxillo-Facial Surgery | 115.00 | 190.00 | 70.00 | 99.00 |
160 | Plastic Surgery | 117.00 | 131.00 | 67.00 | 85.00 |
170 | Cardiothoracic Surgery | 227.00 | 227.00 | 142.00 | 162.00 |
171 | Paediatric Surgery | 191.00 | 241.00 | 109.00 | 163.00 |
172 | Cardiac Surgery | 293.00 | 293.00 | 171.00 | 171.00 |
173 | Thoracic Surgery | 260.00 | 260.00 | 159.00 | 159.00 |
190 | Anaesthetics | 98.00 | 98.00 | 95.00 | 95.00 |
191 | Pain Management | 181.00 | 195.00 | 91.00 | 119.00 |
211 | Paediatric Urology | 182.00 | 196.00 | 111.00 | 111.00 |
214 | Paediatric Trauma and Orthopaedics | 154.00 | 163.00 | 100.00 | 113.00 |
215 | Paediatric Ear Nose and Throat | 116.00 | 141.00 | 74.00 | 74.00 |
216 | Paediatric Ophthalmology | 156.00 | 172.00 | 89.00 | 125.00 |
217 | Paediatric Maxillo-Facial Surgery | 154.00 | 190.00 | 116.00 | 116.00 |
219 | Paediatric Plastic Surgery | 182.00 | 182.00 | 98.00 | 98.00 |
251 | Paediatric Gastroenterology | 279.00 | 279.00 | 158.00 | 158.00 |
252 | Paediatric Endocrinology | 305.00 | 352.00 | 172.00 | 185.00 |
253 | Paediatric Clinical Haematology | 414.00 | 464.00 | 218.00 | 247.00 |
257 | Paediatric Dermatology | 49.00 | 168.00 | 107.00 | 108.00 |
258 | Paediatric Respiratory Medicine | 315.00 | 315.00 | 172.00 | 172.00 |
263 | Paediatric Diabetic Medicine | 353.00 | 353.00 | 119.00 | 119.00 |
300 | General Medicine | 210.00 | 251.00 | 105.00 | 121.00 |
301 | Gastroenterology | 265.00 | 265.00 | 83.00 | 116.00 |
302 | Endocrinology | 230.00 | 230.00 | 106.00 | 116.00 |
303 | Clinical Haematology | 268.00 | 288.00 | 106.00 | 106.00 |
306 | Hepatology | 224.00 | 290.00 | 139.00 | 151.00 |
307 | Diabetic Medicine | 242.00 | 321.00 | 99.00 | 147.00 |
320 | Cardiology | 210.00 | 251.00 | 105.00 | 121.00 |
321 | Paediatric Cardiology | 289.00 | 289.00 | 170.00 | 170.00 |
329 | Transient Ischaemic Attack | 477.00 | 477.00 | – | – |
330 | Dermatology | 112.00 | 168.00 | 69.00 | 108.00 |
340 | Respiratory Medicine | 223.00 | 244.00 | 105.00 | 128.00 |
341 | Respiratory Physiology | 189.00 | 189.00 | 122.00 | 122.00 |
350 | Infectious Diseases | 255.00 | 255.00 | 195.00 | 195.00 |
360 | Genitourinary Medicine | 133.00 | 148.00 | 82.00 | 82.00 |
361 | Nephrology | 299.00 | 454.00 | 124.00 | 219.00 |
370 | Medical Oncology | 228.00 | 290.00 | 98.00 | 115.00 |
410 | Rheumatology | 246.00 | 246.00 | 102.00 | 102.00 |
420 | Paediatrics | 231.00 | 288.00 | 129.00 | 159.00 |
430 | Geriatric Medicine | 303.00 | 303.00 | 139.00 | 139.00 |
501 | Obstetrics | 119.00 | 154.00 | 60.00 | 60.00 |
502 | Gynaecology | 138.00 | 142.00 | 81.00 | 99.00 |
503 | Gynaecological Oncology | 154.00 | 271.00 | 90.00 | 132.00 |
560 | Midwife Episode | 119.00 | 154.00 | 60.00 | 60.00 |
800 | Clinical Oncology | 228.00 | 290.00 | 98.00 | 115.00 |
812 | Diagnostic Imaging | 0.00 | 0.00 | 0.00 | 0.00 |
HRG code | HRG name (A&E) | Band | 24-hour A&E units tariff (£) | Non-24-hour A&E units and minor injury units tariff (£) |
---|---|---|---|---|
VB01Z | Any Investigation with Category 5 Treatment | 1 | 235.00 | 54.00 |
VB02Z | Category 3 Investigation with Category 4 Treatment | 1 | 235.00 | 54.00 |
VB03Z | Category 3 Investigation with Category 1–3 Treatment | 2 | 151.00 | 54.00 |
VB04Z | Category 2 Investigation with Category 4 Treatment | 2 | 151.00 | 54.00 |
VB05Z | Category 2 Investigation with Category 3 Treatment | 2 | 151.00 | 54.00 |
VB06Z | Category 1 Investigation with Category 3–4 Treatment | 3 | 81.00 | 54.00 |
VB07Z | Category 2 Investigation with Category 2 Treatment | 4 | 112.00 | 54.00 |
VB08Z | Category 2 Investigation with Category 1 Treatment | 4 | 112.00 | 54.00 |
VB09Z | Category 1 Investigation with Category 1–2 Treatment | 3 | 81.00 | 54.00 |
VB10Z | Dental Care | 5 | 54.00 | 54.00 |
VB11Z | No Investigation with No Significant Treatment | 5 | 54.00 | 54.00 |
Appendix 4 Generalisability study additional data
Predictive model identifying children most likely to require a central venous catheter in the paediatric intensive care unit
The PICANet database does not record insertion or removal of CVCs. However, through the use of CVC audit data from two PICUs, it was possible to create a predictive model to identify admissions in the PICANet data set most likely to have required a CVC.
Methods
Central venous catheter audit data
Central venous catheter audit data were obtained from four PICUs. Data from PICUs 1 and 2 consisted of individual-level information and data from PICUs 3 and 4 consisted of aggregate data. At PICU 1, the insertion and duration of insertion of CVCs were recorded for 6 months between July and December 2009. At PICU 2, the number of CVCs present for each patient was recorded on a daily basis between December 2005 and March 2012. At PICU 3, the total number of patients admitted and the number of patients with one or more CVCs was recorded by month between January 2011 and February 2012. At PICU 4, the total number of patients admitted, the number of patients with one or more CVC and the total number of CVCs in place were recorded each day between December 2009 and June 2012.
A predictive model for central venous catheter use
Central venous catheter use was identified within the PICANet data set using the PICANet ID and hospital number from the audit data. Multivariable logistic regression was then used to model the probability of CVC use dependent on a set of predictors:
where πi is the probability of CVC use for patient i, a is the constant term and β1. . . βj are the set of predictors. To identify the best-fitting set of predictors, all possible regression models were tested, ranging from the model including only the intercept to the model including all possible predictor variables. Models were compared using the BIC.
Evaluating the performance of the predictive model
To quantify the performance of a predictive model, two measures are typically used:83
-
discrimination (the ability of predicted probabilities to correctly classify children by CVC use)
-
calibration (agreement between observed CVC use and predicted probability of CVC use)
To measure the discrimination of the predictive model, the c-index [equivalent to the area under the receiver operating characteristic (ROC) curve] was calculated. The c-index corresponds to the chance that the predicted probability of CVC use in someone who did require a CVC is greater than the predicted probability of CVC use in someone who did not require a CVC. The greater the c-index, the more discriminative the model.
To measure the calibration of the predictive model, observed CVC use and predicted probabilities were compared using the calibration slope (or linear predictor), as described in Steyerberg et al. 83 The calibration slope is the regression coefficient β in the logistic regression of observed CVC use (binary variable) with predicted CVC use (probability) as the only predictor. Predicted CVC use is calculated as the linear combination of regression coefficients as estimated from the predictive model. In a perfectly calibrated model, the regression coefficient β in the following model would be equal to 1:
By definition, when the model is developed and tested in the same sample, the calibration slope will be equal to 1. However, when predictive models are tested with new data, the calibration slope is often < 1 as most models provide predictions that are too extreme. The closer the β coefficient to 1, the better the calibration of the model.
Internal validation
When predictive models are derived and tested within the same sample of data, measures of predictive ability (e.g. calibration/discrimination) are likely to be overoptimistic. This is because of model overfitting, in which the ‘apparent’ performance in the model derivation data set is likely to be better than the performance in a new set of data. Bootstrapping is an approach that addresses this problem and makes use of all available data, producing more stable results. 83,84 The method involves repeatedly sampling from the original data, a process that simulates sampling from the underlying population from which the original data were drawn. An estimate of the ‘optimism’ in the predictive ability of the model is made by comparing model performance in bootstrap samples with ‘apparent’ performance in the derivation sample.
Bootstrapping was used to estimate the optimism in the predictive ability of the model as measured in the derivation sample of data (i.e. the ‘apparent’ performance in the CVC audit data). The optimism reflects the difference between model performance in the derivation data set and model performance in a separate but similar data set drawn from the same underlying population. Optimism was estimated as the difference between the apparent performance of a model derived in a bootstrap sample and ‘test’ performance when the same model was applied to the derivation sample (CVC audit data).
Finally, apparent model performance (as measured in the derivation sample) was adjusted for optimism/overfitting by subtracting the estimate of optimism from the measure of predictive ability (calibration slope or c-index). The resulting measure of performance is said to be ‘internally validated’. 85
Choosing a probability cut-off
A probability cut-off is required to classify children as either requiring a CVC or not requiring a CVC. Higher probability cut-offs result in greater specificity; lower probability cut-offs results in greater sensitivity. A visualisation of the trade-off between sensitivity and specificity was provided by the ROC curve. Two main criteria are used for finding the optimal cut-off based on maximising the area under the ROC curve, and probability cut-offs according to both of these criteria were calculated:86,119
-
the minimum distance criterion assumes that the optimal cut-off minimises the distance between the point (0,1) and the ROC curve, that is, the minimal value of (17 – sensitivity)2 + (1 – specificity)2
-
the Youden Index criterion assumes that the optimal cut-off maximises the vertical distance between the ROC curve and the line of equality where sensitivity = 1 – specificity, that is, the maximum value of sensitivity + specificity – 1.
External validation
Aggregate CVC audit data from PICUs 3 and 4 were not used for development of the predictive model (individual-level data were not available) but could provide estimates of the average proportion of children requiring a CVC in the PICU. To externally validate the predictive model, the actual numbers of admissions and bed-days with CVCs in the audit data from PICUs 3 and 4 were compared with the results of the predictive model (with shrinkage factor applied).
Results
A predictive model for central venous catheter use
The best-fitting model included length of stay, vasoactive agent, admission from ward, renal support and invasive ventilation. No significant first-order interactions were found. The predicted probability of CVC use for each admission (πi) was derived from the logistic model:
where βxi was the linear predictor of the BIC model. Model coefficients are provided in Table 37.
Evaluating the performance of the predictive model
Discrimination
The c-index of the predictive model in the original sample was estimated as 0.778. The average c-index in 100 bootstrap samples was 0.778 and, on average, the c-index as measured in the derivation sample was 0.004 higher than when measured in the test sample. Subtracting this estimate of optimism from the apparent performance in the derivation sample produced an internally validated c-index of 0.778 – 0.004 = 0.774. This indicated that the model performed reasonably well at classifying children as requiring a CVC.
Calibration
By definition, the calibration slope (β coefficient) for the regression of observed CVC use and predicted CVC use in the original sample was 1, as the model was developed and tested in the same sample:
The average calibration slope in 100 bootstrap samples was 0.967 and, on average, the calibration slope in the derivation data set was 0.033 higher than when measured in the test data set. Subtracting this estimate of optimism from the apparent performance in the derivation sample produced an internally validated calibration slope of 1 – 0.033 = 0.967. This indicated close agreement between observed CVC use and CVC use as predicted in the model.
The coefficients in the original model were multiplied by the shrinkage factor of 0.967 to provide a final model, adjusted for overfitting. 85
Choosing a probability cut-off
The Youden Index indicated that the optimal probability cut-off was 0.57. With this cut-off, the sensitivity of the predictive model for capturing admissions requiring a CVC was 61%, the specificity was 82%, the positive predictive value was 82% and the negative predictive value was 61%.
External validation
Compared with the aggregate CVC data, the model predicted that 54.6% and 63.5% of admissions in the Newcastle PICU and Birmingham PICU, respectively, required a CVC, compared with true values of 49.4% and 54.6% respectively. The predictive model identified 80% of the CATCH admissions as having a CVC.
PICU trust | Type of CVC used prior to the CATCH 2009 survey | Admissions requiring a CVC, 2012 survey | ||
---|---|---|---|---|
Emergency admissions | Elective admissions | Emergency admissions (%) | Elective admissions (%) | |
1 | Not surveyed | Not surveyed | 75 | 25 |
2 | Standard/heparin | Standard/heparin | 57 | 93 |
3 | Not surveyed | Not surveyed | < 5 | < 5 |
4 | Standard/heparin | Standard | 80 | 90 |
5 | Standard | Standard | 85 | 50 |
6 | Standard | Standard | No response | No response |
7 | Standard/antibiotic | Standard | 60–100 | 60–90 |
8a | Standard | Standard | 80 | 80 |
9a | Heparin | Heparin | 60 | 30 |
10 | Standard | Standard | 30 | 50 |
11 | Standard | Standard | 50 | 50 |
12 | Standard | Standard | No response | No response |
13 | Standard | Standard | No response | No response |
14 | Standard | Standard | 75 | No response |
15 | Heparin | Heparin | 87 | 18 |
16 | Standard | Standard | 60 | 20 |
17 | Standard | Standard | 70 | 80 |
18 | Standard | Standard | No response | No response |
19 | No response | No response | No response | No response |
20 | Standard | Antibiotic | 50 | 50 |
21 | Heparin | Standard/heparin | 50 | 30 |
22a | Not surveyed | Not surveyed | No response | No response |
23a | Not surveyed | Not surveyed | 60 | 50 |
Average | 60 | 50 |
Predictor | Odds ratio (95% CI) | p-value |
---|---|---|
Length of stay (hours) | 1.003 (1.000 to 1.004) | < 0.0001 |
Vasoactive agent | 4.443 (3.600 to 5.513) | < 0.0001 |
Admission from ward | 1.428 (1.200 to 1.738) | < 0.0001 |
Renal support | 3.952 (2.000 to 7.822) | < 0.0001 |
No ventilation or non-invasive ventilation only | 1 | |
Invasive ventilation | 2.547 (1.900 to 3.350) | < 0.0001 |
Invasive and non-invasive ventilation | 2.278 (1.500 to 3.395) | < 0.0001 |
Characteristic | CATCH participantsa (n = 1398) | Admissions expected to require a CVC (n = 20,199) | All admissions during the trial period (n = 53,897) | |||
---|---|---|---|---|---|---|
n | % | n | % | n | % | |
Length of stay (hours) | ||||||
1 to < 4 | 3 | 0.2 | 186 | 0.9 | 1482 | 2.7 |
4 to < 12 | 18 | 1.3 | 438 | 2.2 | 3764 | 7.0 |
12 to < 24 | 101 | 7.2 | 1699 | 8.4 | 9647 | 17.9 |
24 to < 48 | 175 | 12.5 | 2959 | 14.6 | 10,919 | 20.3 |
48+ | 1101 | 78.8 | 14,917 | 73.9 | 28,085 | 52.1 |
Age (years) | ||||||
< 1 | 815 | 58.3 | 11,775 | 58.3 | 27,323 | 50.7 |
1–4 | 327 | 23.4 | 4473 | 22.1 | 13,405 | 24.9 |
5–10 | 144 | 10.3 | 2023 | 10.0 | 6837 | 12.7 |
11–15 | 112 | 8.0 | 1926 | 9.5 | 6328 | 11.7 |
Unknown | 0.0 | 2 | 0.0 | 4 | 0.0 | |
Vasoactive agents | 1054 | 75.4 | 17,081 | 84.6 | 18,792 | 34.9 |
Renal support | 148 | 10.6 | 1469 | 7.3 | 1684 | 3.1 |
PIM2 | ||||||
< 1% | 150 | 10.7 | 1857 | 9.2 | 13,855 | 25.7 |
1 to < 5% | 744 | 53.2 | 10,332 | 51.2 | 25,840 | 47.9 |
5 to < 15% | 354 | 25.3 | 5472 | 27.1 | 10,520 | 19.5 |
15 to < 30% | 103 | 7.4 | 1486 | 7.4 | 2290 | 4.2 |
30%+ | 47 | 3.4 | 1052 | 5.2 | 1392 | 2.6 |
Ventilation status | ||||||
Neither | 33 | 2.4 | 442 | 2.2 | 12,652 | 23.5 |
Non-invasive only | 10 | 0.7 | 159 | 0.8 | 2620 | 4.9 |
Invasive only | 1017 | 72.7 | 16,170 | 80.1 | 32,882 | 61.0 |
Both | 337 | 24.1 | 3424 | 17.0 | 5625 | 10.4 |
Unknown | 1 | 0.1 | 4 | 0.0 | 118 | 0.2 |
Type of admission | ||||||
Planned | 572 | 40.9 | 9015 | 44.6 | 21,844 | 40.5 |
Unplanned | 826 | 59.1 | 11,180 | 55.3 | 31,992 | 59.4 |
Unknown | 0.0 | 4 | 0.0 | 61 | 0.1 | |
Source of admission | ||||||
Same hospital | 729 | 52.1 | 11,713 | 58.0 | 32,966 | 61.2 |
Other hospital | 667 | 47.7 | 8374 | 41.5 | 20,210 | 37.5 |
Unknown | 2 | 0.1 | 112 | 0.6 | 721 | 1.3 |
Primary diagnosis at admission | ||||||
Cardiac | 707 | 50.6 | 10,687 | 52.9 | 16,818 | 31.2 |
Respiratory | 273 | 19.5 | 3751 | 18.6 | 14,295 | 26.5 |
Infection | 100 | 7.2 | 1078 | 5.3 | 2333 | 4.3 |
Other | 318 | 22.7 | 4683 | 23.2 | 20,451 | 37.9 |
Care area of admission | ||||||
A&E | 242 | 17.3 | 2379 | 11.8 | 9422 | 17.5 |
HDU | 72 | 5.2 | 878 | 4.3 | 2410 | 4.5 |
ICU/PICU/NICU | 222 | 15.9 | 3802 | 18.8 | 8112 | 15.1 |
Other intermediate care area | 8 | 0.6 | 466 | 2.3 | 1315 | 2.4 |
Recovery only | 3 | 0.2 | 39 | 0.2 | 155 | 0.3 |
Theatre and recovery | 565 | 40.4 | 8422 | 41.7 | 20,566 | 38.2 |
Unknown | 9 | 0.6 | 165 | 0.8 | 919 | 1.7 |
Ward | 273 | 19.5 | 3889 | 19.3 | 10,417 | 19.3 |
Radiography/endoscopy/CT | 4 | 0.3 | 159 | 0.8 | 581 | 1.1 |
Retrieval, yes | 596 | 42.6 | 7464 | 37.0 | 18,230 | 33.8 |
Retrieval team | ||||||
Non-specialist team | 19 | 1.4 | 721 | 3.6 | 2031 | 3.8 |
Other specialist team | 306 | 21.9 | 4358 | 21.6 | 9681 | 18.0 |
Own team | 263 | 18.8 | 2339 | 11.6 | 6388 | 11.9 |
Unknown | 8 | 0.6 | 46 | 0.2 | 130 | 0.2 |
Sex | ||||||
Male | 811 | 58.0 | 11,363 | 56.3 | 30,428 | 56.5 |
Female | 587 | 42.0 | 8830 | 43.7 | 23,449 | 43.5 |
Unknown | 0.0 | 6 | 0.0 | 20 | 0.0 | |
PICU type | ||||||
General | 59 | 4.2 | 2831 | 14.0 | 15,828 | 29.4 |
Mixed | 1286 | 92.0 | 16,997 | 84.1 | 37,386 | 69.4 |
Cardiac | 53 | 3.8 | 371 | 1.8 | 683 | 1.3 |
PICU size (admissions per year) | ||||||
< 650 | 59 | 4.2 | 2373 | 11.7 | 14,255 | 26.4 |
650–1000 | 620 | 44.3 | 3227 | 16.0 | 8731 | 16.2 |
> 1000 | 719 | 51.4 | 14,599 | 72.3 | 30,911 | 57.4 |
Appendix 5 Statistical analysis report
Glossary
- Bundled Healthcare Resource Group
- Healthcare Resource Group referring to a patient pathway of care such as a ward stay.
- Unbundled Healthcare Resource Group
- A high-cost or specialist service Healthcare Resource Group in addition to a patient pathway of care.
List of abbreviations
- A&E
- accident and emergency
- AIC
- Akaike information criterion
- BIC
- Bayesian information criterion
- BSI
- bloodstream infection
- CEAC
- cost-effectiveness acceptability curve
- CHiP
- Control of Hypoglycaemia in Paediatric Intensive Care
- CI
- confidence interval
- CR-BSI
- catheter-related bloodstream infection
- CRF
- case report form
- CVC
- central venous catheter
- DNA
- deoxyribonucleic acid
- HDU
- high-dependency unit
- HES
- Hospital Episode Statistics
- HQIP
- Healthcare Quality Improvement Partnership
- HR
- hazard ratio
- HRG
- Healthcare Resource Group
- HTA
- Health Technology Assessment
- ICER
- incremental cost-effectiveness ratio
- ICU
- intensive care unit
- IDSMC
- Independent Data and Safety Monitoring Committee
- ln
- natural logarithm
- NCA
- National Clinical Audit
- NICU
- neonatal intensive care unit
- ONS
- Office for National Statistics
- PAS
- Patient Administration System
- PCR
- polymerase chain reaction
- PHE
- Public Health England
- PICANet
- Paediatric Intensive Care Audit Network
- PICU
- paediatric intensive care unit
- PIM2
- Paediatric Index of Mortality
- QALY
- quality-adjusted life-year
- RCT
- randomised controlled trial
- ROC
- receiver operating characteristic
- RR
- relative risk
- rRNA
- ribosomal ribonucleic acid