Notes
Article history
The research reported in this issue of the journal was commissioned and funded by the Evidence Synthesis Programme on behalf of NICE as project number NIHR135564. The protocol was agreed in April 2022. The assessment report began editorial review in July 2022 and was accepted for publication in March 2023. The authors have been wholly responsible for all data collection, analysis and interpretation, and for writing up their work. The HTA editors and publisher have tried to ensure the accuracy of the authors’ report and would like to thank the reviewers for their constructive comments on the draft document. However, they do not accept liability for damages or losses arising from material published in this report.
Permissions
Copyright statement
Copyright © 2023 Metry et al. This work was produced by Metry et al. under the terms of a commissioning contract issued by the Secretary of State for Health and Social Care. This is an Open Access publication distributed under the terms of the Creative Commons Attribution CC BY 4.0 licence, which permits unrestricted use, distribution, reproduction and adaption in any medium and for any purpose provided that it is properly attributed. See: https://creativecommons.org/licenses/by/4.0/. For attribution the title, original author(s), the publication source – NIHR Journals Library, and the DOI of the publication must be cited.
2023 Metry et al.
Chapter 1 Background
Description of the underlying health problem
Severe acute respiratory syndrome coronavirus 2 (SARS-CoV-2) is the virus that causes coronavirus disease 2019 (COVID-19). At the time of writing (January 2023) there had been more than 660 million cases of COVID-19 worldwide and more than 6.5 million deaths; in the UK these values were more than 24 million cases and approaching 200,000 deaths. 1 In the UK, there have been waves of infections (peaking in late December 2021 and early January 2022), and waves of death (peaking in January 2021). 1
The ratio of notified infections to death in the UK has changed markedly over time, being approximately 5 to 1 in April 2020, 45 to 1 in January 2021 and 700 to 1 in January 2022 (authors’ calculations based on Worldometer data1). Factors associated with the change in ratio include:
-
better ascertainment of COVID-19 cases, which previously may have been left unobserved particularly early in the pandemic especially when mild or asymptomatic;
-
increasing level of protection in the population, both acquired from previous SARS-CoV-2 infection and vaccine-induced;
-
improved levels of treatment, such as the use of dexamethasone;
-
the likelihood of more frail people dying in earlier waves; and
-
the change in variants of SARS-CoV-2.
Should the risk of death following COVID-19 remain at low levels and SARS-CoV-2 becomes endemic in society, then treatments for patients with COVID-19 may no longer be treated differently to interventions for other conditions such as breast cancer or heart disease. If this were the case, then it could be considered logical and acceptable that pharmacological treatment for COVID-19 would be appraised by the National Institute for Health and Care Excellence (NICE) using its standard methods. 2 This is in line with the best practice recommendations for the assessment of diagnostics and therapeutics for COVID-19 published by HORIZON 2020. 3
The SARS-CoV-2 variants have changed noticeably throughout the COVID-19 pandemic. Between February 2021 and May 2021, the Alpha variant was predominant, but was replaced by the Delta variant which was the main variant until December 2021 when Omicron became established. Since then, there has been a period where Omicron BA2 has been the predominant variant and in July 2022, Omicron BA5 was estimated to be the cause of 75% of identified SARS-CoV-2 variants. 4
The NICE scope
In April 2022, NICE issued a scope5 for the assessment of therapeutics for people with COVID-19; the NICE website also hosts the final protocol written by the External Assessment Group (EAG). 6 This scope was revised and finalised in August 2022;7 the key changes being that lenzilumab was removed as an intervention and tixagevimab and cilgavimab was added as an intervention. The remit of the final scope was to appraise the clinical and cost-effectiveness of eight interventions for treating (1) people with mild COVID-19 at high risk of progressing to severe COVID-19 and (2) people with severe COVID-19. The comparators included the established management in clinical practice with or without corticosteroids and appropriate respiratory support, and the other interventions. The components of the decision problem are discussed more fully in The decision problem. The deadline for the original EAG report sent to stakeholders was 30 June 2022, allowing ˂3 months for the estimates of the clinical effectiveness of each intervention to be made, for the mathematical models to be adapted and run, the results to be interpreted and the report to be written.
The NICE scope7 did not include secondary infections to National Health Service (NHS) staff, or the wider population, which may be unfavourable to the interventions. The impact of transmission may be reduced as the modelled population are those with COVID-19 who are therefore symptomatic and who have been either hospitalised or referred for treatment. In this circumstance, it is likely that peak viral load has passed and that the modelled population would avoid unnecessary contact with other people. The scope also does not cover the potential benefits of interventions in maintaining the capacity for operations or in avoiding delays in patients’ treatment that could arise due to either a reduced number of patients in hospital with COVID-19, or reduced staff absence due to COVID-19. Were this benefit, which has been termed ‘enablement’, included in the model this would likely be favourable to the interventions.
Reinfections and readmission were not listed in the NICE scope and have not been considered in the modelling due to the lack of data and time constraints. It is uncertain whether this omission is favourable or unfavourable to particular interventions as subsequent adverse events could reduce the estimated quality-adjusted life-year (QALY) gains from avoiding adverse events in the first hospitalisation due to treatment, but the interventions could also confer additional protection from a secondary infection.
The scope focusses on treating patients with COVID-19. It does not include prophylactic treatment for patients who are at high risk but who do not have COVID-19.
Description of current service provision
Patients with severe COVID-19 are typically hospitalised with the intensity of treatment dependent on the severity of the condition. Patients may be treated in intensive care units, be provided with high-flow oxygen (HFO) or low-flow oxygen (LFO), and be treated with interventions, including those in the NICE scope and with corticosteroids.
The decision problem
This section has been subdivided into sections detailing the population, interventions, comparators, outcome measures and subgroups.
Population
The population considered within the EAG report has been divided into two broad groups. The first group consists of people who have been hospitalised due to COVID-19 and the second group consists of people who are at high risk of requiring hospital care due to COVID-19. Patients who were hospitalised for reasons other than COVID-19 and contracted COVID-19 in hospital and were at high risk of requiring hospital care for COVID-19 were categorised within the second group. For brevity, all patients not hospitalised due to COVID-19 who are at high risk of hospitalisation will be termed ‘non-hospitalised patients’ noting the aforementioned caveat regarding patients who contract COVID-19 in hospital, whereas patients who have been hospitalised directly because of COVID-19 are referred to as ‘hospitalised patients’.
Following discussions with NICE, the definition for patients at high risk was aligned to that considered within the Platform Adaptive trial of NOvel antiviRals for eArly treatMent of COVID-19 In the Community (PANORAMIC) clinical study,8 with the exception that being aged 50 years or over was not considered to be a high-risk factor.
The aim of treatment differs between both groups. For patients hospitalised due to severe or critical COVID-19, the aim of treatment is to reduce the immunoinflammatory response of the body and prevent clinical deterioration. For non-hospitalised patients, the aim of treatment is to prevent viral replication and damp inflammation, thus reduce the probability of the development of severe symptoms that could lead to hospitalisation or death.
Interventions
The interventions listed within the NICE scope7 are shown in Tables 1–3 based on marketing authorisation in the UK at the time of writing. Table 1 contains the interventions with marketing authorisation in the UK, Table 2 contains the interventions with conditional marketing authorisation in the UK and Table 3 contains the interventions with no marketing authorisation in the UK. Each table contains the generic name of the intervention, its branded name and the company manufacturing it, the class of intervention, the mode of administration and recommended dose. Table 1 provides the indication for the drug, while Tables 2 and 3 provide the population in key studies for the intervention.
Generic treatment name (branded name and company) | Class | Mode of administration (recommended dose) | Indication relevant to the decision problem |
---|---|---|---|
Casirivimab/imdevimab (Ronapreve, Regeneron and Roche) | mAb | i.v./s.c. (600 mg of both drugs administered together as one infusion. An s.c. injection is permitted if an i.v. approach would lead to a delay) | Treatment of acute COVID-19 infection |
Molnupiravir (Lagevrio, Ridgeback Biotherapeutics and Merck Sharp and Dohme) | Antiviral | Oral (800 mg twice daily for 5 days) | Treatment of mild to moderate COVID-19 in adults with a positive SARS-CoV-2 diagnostic test and who have at least one risk factor for developing severe illness |
Tocilizumab (RoActemra, Roche) | Immunomodulator | s.c./i.v. (8 mg/kg administered once i.v. with 0.9% sodium chloride over 1 hour) One additional infusion of tocilizumab 8 mg/kg may be administered. The interval between the two infusions should be at least 8 hours |
Treatment of COVID-19 in adults who are receiving systemic corticosteroids and require supplemental oxygen or mechanical ventilation |
Generic treatment name (branded name and company) | Class | Mode of administration (recommended dose) | Therapeutic indication in the SmPC relevant to the decision problem |
---|---|---|---|
Nirmatrelvir/ritonavir (Paxlovid, Pfizer) | Antiviral | Oral (300 mg (nirmatrelvir) and 100 mg (ritonavir) twice daily for 5 days) | Treatment of COVID-19 in adults who do not require supplemental oxygen and who are at increased risk for progression to severe COVID-19 |
Remdesivir (Veklury, Gilead) | Antiviral | i.v. (200 mg loading dose on day 1 for all patients, then dependent on patient characteristics).
|
Treatment of COVID-19 in:
|
Sotrovimab (Xevudy, GlaxoSmithKline and Vir Biotechnology) | mAb | i.v. (500 mg over 30 minutes) | Treatment of symptomatic adults and adolescents (aged 12 years and over and weighing at least 40 kg) with acute COVID-19 infection who do not require oxygen supplementation and who are at increased risk of progressing to severe COVID infection |
Tixagevimab/cilgavimaba (Evusheld, Astra Zeneca) | mAb | Intramuscular injection (single dose of 300 mg of tixagevimab and 300 mg of cilgavimab) | Treatment of COVID-19 in adults who do not require supplemental oxygen and who are at increased risk for progression to severe COVID-19 |
Generic treatment name (branded name and company) | Class | Mode of administration (recommended dose) | Population in key studies if no marketing authorisation or conditional marketing authorisation exists |
---|---|---|---|
Baricitinib (Olumiant, Eli Lilly) | Immunomodulator | Oral (4 mg daily, the optimal duration is currently unclear) | Studied in clinical trials, as a monotherapy, in people with COVID-19 |
Baricitinib (Olumiant, Eli Lilly) and remdesivir (Veklury, Gilead) | Immunomodulator and antiviral | As for the component drugs | Studied in clinical trials in people aged 18 years and older, hospitalised with COVID-19 |
Multiple interventions are indicated for the prevention of severe COVID-19. Severe disease in adults is defined as having clinical signs of pneumonia plus at least one of the following: respiratory rate > 30 breaths/minute, severe respiratory distress, or saturation of peripheral oxygen < 90% on room air and would require hospitalisation. 9
Comparators
The comparators within the decision problem include all of the interventions contained in Tables 1–3, when used in the same position as a particular intervention and additionally standard of care (SoC) which would be dependent on the severity of the patient’s illness. SoC is defined as any treatment widely accepted by the NHS, which is routinely funded by the NHS with no strong rationale to appraise it, for example supplemental oxygen and dexamethasone. SoC has evolved throughout the COVID-19 pandemic, which means that randomised controlled trials (RCTs) conducted comparing interventions against SoC may not be directly comparable as SoC has improved over time.
Outcome measures
The NICE scope7 lists nine possible outcomes to explore: mortality; requirement for respiratory support; time to recovery; hospitalisation (requirement and duration); time to return to normal activities; virological outcomes (viral shedding and viral load); post-COVID-19 symptoms; adverse effects of treatments; and health-related quality of life (HRQoL). All model outcomes, except virological outcomes were assessed; these were excluded as these would be of more relevance to decision problems that included transmission and due to the prioritisation of other endpoints given the limited time available.
The cost-effectiveness of the eight treatments was expressed in terms of incremental cost-effectiveness ratios (ICERs) which were reported in terms of cost per QALY gained. A patient lifetime horizon was used to take differential mortality between treatments into account.
Subgroups
Due to time constraints, the only subgrouping considered was related to whether oxygen was required upon admission to hospital entry. This was considered important as the licensed indication and the clinical outcomes for some of the appraised interventions depend on the level of oxygen support required. The EAG is aware that other possible criteria for selecting subgroups include but are not limited to: age; immune system competence; comorbidities; seroprevalence; vaccination status; and the predominant SARS-CoV-2 variant but did not have the time to explore the impact of these characteristics.
Amendments to the initial EAG report
In November 2022, NICE released an appraisal consultation document (ACD) related to Therapeutics for people with COVID-19. 10 Many comments were received by NICE in response to the ACD, some of which have direct implications to the population of the EAG’s model and therefore the results generated. Based on these responses and the publication of relevant papers the EAG made seven changes to the modelling which are detailed below. The results presented in this report include these changes and the text in this report has been amended to reflect these changes:
-
Change 1: A more recent data set from COVID-network meta-analyses (NMA)11,12 has been used.
-
Change 2: Efficacy measures have been capped such that no treatment is estimated to cause harm to a patient.
-
Change 3: Amending the percentage of high-risk patients with COVID-19 who are hospitalised to reflect more recent data in Patel et al. 13
-
Change 4: The cost of hospitalisation in ordinal scales 4 or 5 has been increased to consider multiple finished consultant episodes per admission.
-
Change 5: The average duration of long COVID has been increased to use more recent data. 14
-
Change 6: The annual cost of long COVID has been increased as a more appropriate source was identified. 15
-
Change 7: An error in the implementation of clinical improvement was corrected, although this had only a minor impact.
Additionally, two further scenario analyses have been conducted which incorporated data not contained in the COVID-NMA summaries. This was the inclusion of data from Solidarity16 for remdesivir and additional data for tixagevimab/cilgavimab when treatment was provided within 5 days of infection.
Chapter 2 Clinical effectiveness
Methods for the rapid evidence review
Given the timelines of the project, the EAG could not follow best practice for systematically reviewing the clinical evidence relevant to the decision problem. Following discussions with NICE, a pragmatic, alternative approach was undertaken relying on the use of data extracted by third parties which are referred to as ‘living systematic reviews’. This is in line with the best practice recommendations for the assessment of diagnostics and therapeutics for COVID-19 published by HORIZON 2020. 3 The methods used, assumptions taken and the summarised results are provided in this chapter.
Rationale for using living systematic reviews
COVID-19 clinical research has accelerated dramatically worldwide, with over 5000 registered trials investigating therapeutic interventions for COVID-19. 17 The need for rapid information on COVID-19 has resulted in a paradigm shift, especially in the communication of scientific results. Traditional systematic reviews can date quickly but ‘living’ systematic reviews search for evidence much more regularly than standard reviews and incorporate relevant new evidence as it becomes available. This is important in the context of COVID-19, in which the evidence-base is rapidly changing as new data emerge. The ability of a ‘living’ systematic review and NMA to regularly update and incorporate relevant new evidence as it becomes available makes it the best type of evidence synthesis, in the opinion of the EAG, to inform this pragmatic rapid evaluation. This approach has been recommended by best practice recommendations3 which stated that ‘HTA agencies should consider the use of existing “living” clinical evidence reviews and meta-analyses to inform their clinical effectiveness decisions’ as ‘Using these sources will reduce duplication of work and may allow for quicker assessments’.
The EAG did not have the time to attempt to untangle the impact of differences between studies in terms of aspects such as the dominant SARS-CoV-2 variant, SoC, vaccination status, outcome definition and age of participants and caution that the results may not be directly comparable between interventions. The EAG also did not have time to: validate the data within the living systematic reviews; to quality assess the component studies; or to remove studies that were not using the appropriate doses. To recognise this uncertainty the EAG has run ‘mean’, ‘high’ and ‘low’ efficacy scenarios in the cost-effectiveness analyses (see Analyses undertaken) to allow decision-makers an indication of how cost-effectiveness changes with different efficacy assumptions.
Selection criteria for the living systematic reviews
Several living systematic reviews that incorporate emerging trial data and allow for analysis of comparative effectiveness of multiple COVID-19 treatments have been robustly developed and published. 12,17–19 Two sources were selected as they provided detailed relevant outcome data from individual studies and up-to-date evidence synthesis to inform the model.
The first source is the COVID-NMA initiative,11,12 supported by the World Health Organization (WHO) and Cochrane which is a living systematic review of registered randomised trials, in which all available evidence related to COVID-19 is regularly collected, critically appraised and synthesised using pairwise comparisons and NMA methods. These analyses are updated every 2 weeks and results can be accessed via a web interface (https://covid-nma.com/).
The second source is the metaEvidence initiative,18 supported by the University Hospital of Lyon and the University of Lyon which is also a living meta-analysis and evidence synthesis of therapies for COVID-19 and is an emerging online resource that provides direct access to the efficacy and safety results reported in the studies for potential drugs for the treatment of COVID-19. The risk of bias, synthesised by meta-analysis, is also reported. The analyses are updated within a target time of ˂ 24 hours with results accessed through a web interface (www.metaevidence.org/COVID19.aspx).
Other sources of evidence, which primarily informed living guidelines,17,19 did not report the extracted outcome data from individual studies. As such, they precluded further synthesis and evaluation.
Assumption of transportability of relative treatment effects
A consequence of the need to use data from the living systematic reviews was that there was reduced scope for the EAG to undertake nuanced analyses with a key limitation being that the EAG had to assume that all relative treatment effects were generalisable to different settings. This meant that for each intervention, the same treatment effects, either hazard ratios (HRs) or relative risks (RRs), were assumed to be applicable regardless of study characteristics which include: the age, perceived severity, vaccination status and history of SARS-CoV-2 infection of patients; the SoC at that time; the geographical location; and the dosage of the intervention used. The EAG acknowledges that this assumption may be incorrect, which adds additional uncertainty to the clinical- and cost-effectiveness results.
Inclusion criteria and data extraction
Selected data were extracted for the interventions contained in Tables 1–3. Key model outcomes such as time to death, clinical improvement at day 28 or day 60 (defined as a hospital discharge or improvement on the scale used by trialists to evaluate clinical progression and recovery) and incidence of serious adverse events (SAEs) were initially extracted from the COVID-NMA living systematic review. 12 Where relevant outcome data were not available, these data were extracted from the metaEvidence living systematic review. 18 All data extractions (undertaken between 16 March and 18 May, updated between 25 and 31 May 2022 and updated again on 6 September 2022 and 19 December 2022) were undertaken by one reviewer (AS) and checked by a second reviewer (AP), with any discrepancies resolved by a third reviewer (SR). All data and evidence synthesis analyses were extracted from forest plots, tables and text generated by the COVID-NMA and metaEvidence web interface; checking of the extracted data by the EAG against the original RCT publications for accuracy could not be undertaken within the timescales of the project.
Adjustments made for changing SoC, SARS-CoV-2 variant, vaccination status and prior infection
The conditions under which each study was conducted were heterogeneous. Across time SoC has changed markedly, most particularly with reference to the widespread use of corticosteroids such as dexamethasone and change in SARS-CoV-2 variants. The vaccine roll-out in England has provided protection that was not available to patients recruited to early studies, similarly, there is likely to be an increased level of protection associated with prior infection. Ideally there would be attempts to establish the impact of different circumstances on the observed clinical effectiveness of interventions in studies, although this was not possible within the timescales of the project. As such, the EAG had to make a simplistic assumption that none of the changes were treatment effect modifiers, and that given this, the relative benefits observed in the studies were generalisable and could be applied to the estimated outcomes for patients with COVID-19 in England in Summer 2022. The EAG notes that this assumption is very unlikely to hold but believed that this approach was preferable to provide no estimates of effectiveness. The EAG further warns that comparing treatments, either in terms of clinical- or cost-effectiveness could be very misleading.
One notable comment is that there is a belief raised by stakeholders and confirmed by the clinical authors of this report that casirivimab/imdevimab does not work for the Omicron variant of SARS-CoV-2. Further guidance from the US Food and Drug Administration that ‘sotrovimab is not authorized in any US state or territory at this time’ (5 April 2022) as it is unlikely to be effective against the Omicron BA.2 subvariant. 20 Additionally, less than a fortnight before the report was completed, the WHO offered strong recommendations against the use of casirivimab/imdevimab in patients with COVID-19 and against the use of sotrovimab in patients with non-severe COVID-19. 21 As such, it is likely that the ‘low’ efficacy scenario described in Analyses undertaken may be the most appropriate scenario for casirivimab/imdevimab and for sotrovimab in patients with non-severe COVID-19, with the results from the ‘mean’ and ‘high’ efficacy scenarios reserved for consideration if there is a change in the SARS-CoV-2 variant. The robustness of any estimate of efficacy is uncertain.
Another stakeholder comment was the belief that monoclonal antibodies may have better effectiveness than antivirals in reducing supplemental oxygen use in patients treated in the community that are subsequently hospitalised. Clinical opinion provided to the EAG suggests that the effect of antivirals is uncertain. The EAG believes that the sensitivity analyses undertaken in conjunction with an incremental net monetary benefit approach, shortened to net monetary benefit (NMB) approach (see Analyses undertaken for further details) would allow the committee to consider alternative assumptions.
Results of the rapid evidence review
This section reports key results from the analyses described in Methods for the rapid evidence review. A brief description of each included RCT, reproduced from the COVID-NMA Initiative,12 is presented in Appendix 1 Table 22. Appendix 2 Table 23 also presents a summary of the extracted data for each intervention and relevant outcomes from the living systematic reviews. The assumed clinical effectiveness for each intervention in hospitalised patients is detailed in Table 4, and in Table 5 for patients at high risk of hospitalisation treated in the community. The interventions are listed in order of current marketing authorisation and alphabetical order. The values reported in Tables 4 and 5 are used to inform the economic evaluation. All measures of treatment effect, such as RRs and HRs and 95% confidence intervals (CIs) were taken directly from the living systematic reviews unless specified. The individual studies informing Tables 4 and 5 are detailed in Appendix 1 Table 22. Where data were not available for clinical improvement or time to discharge a value of 1.0 was used as the model results were not sensitive to these values within the observed range associated with other interventions.
Intervention | Estimated efficacy (95% CI) | Mean value from the log-normal distribution | Source of evidence (number of studies informing the estimate) |
---|---|---|---|
Time to death HR | |||
Casirivimab/imdevimab | 0.69 (0.50 to 0.93) | 0.70 | COVID-NMA12 (1 study) |
Tocilizumab | 0.76 (0.64 to 0.90) | 0.76 | COVID-NMA12 (9 studies) |
Remdesivir | 0.77 (0.57 to 1.04) | 0.78 | COVID-NMA12 (3 studies) |
Baricitinib | 0.61 (0.47 to 0.78) | 0.62 | COVID-NMA12 (2 studies) |
Baricitinib/remdesivir | 0.65 (0.39 to 1.09) | 0.67 | COVID-NMA12 (1 study) |
RR for clinical improvement at 28 days | |||
Casirivimab/imdevimab | 1.03 (0.98 to 1.09) | 1.03 | COVID-NMA12 (2 studies) |
Tocilizumab | 1.05 (1.00 to 1.11) | 1.05 | COVID-NMA12 (15 studies) |
Remdesivir | 1.04 (0.99 to 1.10) | 1.04 | COVID-NMA12 (4 studies) |
Baricitinib | 1.02 (1.00 to 1.05) | 1.02 | COVID-NMA12 (3 studies) |
Baricitinib/remdesivir | 1.08 (1.00 to 1.17) | 1.08 | COVID-NMA12 (1 study) |
Time to discharge HR | |||
Casirivimab/imdevimab | 1.24 (1.05 to 1.47) | 1.24 | metaEvidence18 (2 studies) |
Tocilizumab | 1.05 (0.88 to 1.25) | 1.05 | metaEvidence18 (2 studies) |
Intervention | Estimated efficacy (95% CI) | Mean value from the log-normal distribution | Source of evidence (number of studies informing the estimate) |
---|---|---|---|
Hospitalisation or death RR | |||
Casirivimab/imdevimab | 0.28 (0.18 to 0.44) | 0.29 | COVID-NMA12 (3 studies) |
Molnupiravir | 0.80 (0.56 to 1.15) | 0.81 | COVID-NMA12 (5 studies) |
Nirmatrelvir/ritonavir | 0.13 (0.07 to 0.27) | 0.14 | COVID-NMA12 (1 study) |
Remdesivir | 0.28 (0.10 to 0.74) | 0.32 | COVID-NMA12 (1 study) |
Sotrovimab | 0.20 (0.08 to 0.48) | 0.22 | COVID-NMA12 (1 study) |
Tixagevimab/cilgavimab | 0.50 (0.29 to 0.86)a | 0.52 | metaEvidence18 (1 study) |
All-cause mortality RR at 28 days | |||
Casirivimab/imdevimab | 0.51 (0.09 to 2.95) | 0.76 | COVID-NMA12 (4 studies) |
Molnupiravir | 0.27 (0.09 to 0.82) | 0.32 | COVID-NMA12 (7 studies) |
Nirmatrelvir/ritonavir | 0.04 (0.00 to 0.63) | 0.15 | COVID-NMA12 (1 study) |
Remdesivir | 1.00 (0.02 to 50.23)b | 7.36c | COVID-NMA12 (1 study) |
Sotrovimab | 0.20 (0.01 to 4.16) | 0.65 | COVID-NMA12 (1 study) |
Tixagevimab/cilgavimab | 1.00 (0.32 to 3.06) | 1.18c | COVID-NMA12 (1 study) |
To aid interpretation of the clinical efficacy data for interventions used to treat patients in hospital, plots of (1) the HR for death at 28 days, (2) the RR for clinical improvement at 28 days and (3) the HR associated with time to discharge are presented in Appendix 3 (see Figures 22–24).
The EAG simulated 5000 sets of draws for each intervention assuming that all distributions are independent (and not capped) and recorded the order of treatments from most efficacious to least efficacious. For each treatment, the proportion of simulations in which an intervention is in each rank position is shown in Appendix 3 (see Figure 25). There is considerable uncertainty in the results; for example, baricitinib is the intervention with the greatest estimated probability of being ranked first, yet has similar probabilities of being ranked second, or of being third, fourth and fifth combined. To add additional uncertainty, the assumption that the efficacy estimate is generalisable to different settings is likely to be incorrect due to differences in factors such as SoC, predominant SARS-CoV-2 variant and vaccination status.
To aid interpretation of the clinical efficacy data for interventions used to treat patients in the community, plots of the RR for avoiding hospitalisation or death at 28 days, and of the RR for avoiding death at 28 days, are shown in Appendix 3 (see Figures 26 and 27). The EAG simulated 5000 sets of draws for each intervention assuming that all distributions are independent and recording the order of treatments from most efficacious to least efficacious. For each treatment, the proportion of simulations in which an intervention is in each rank position is shown in Appendix 3 (see Figure 28). There is considerable uncertainty in the results; for example, while nirmatrelvir/ritonavir has a large, estimated probability (> 65%) of being ranked first, it has a 15% chance of being ranked third or lower. To add additional uncertainty, the assumption that the efficacy estimate is generalisable to conditions in October 2022 is likely to be incorrect.
The interventions should be reviewed for activity against future variants. If it is shown that these confer more or less protection than against the predominant variant in the key clinical studies, then decision-makers may choose to select the ‘high’ or ‘low’ efficacy results to guide estimates of cost-effectiveness.
Chapter 3 Methods for the cost-effectiveness analysis
A provisional working plan was available in the published NICE final scope. 5 The model framework for assessing the cost-effectiveness of treatments for people hospitalised due to COVID-19 is an adaptation of the approach taken by Rafia et al. 22 This decision was made for two principal reasons. Firstly, there is an overlap in the authors for both the Rafia et al. paper and this report, meaning that the model was available to the team reducing model construction time. Secondly, this model structure was used in a preliminary appraisal of remdesivir that was undertaken by a NICE panel meeting;23 while no formal documents related to this meeting have been released, an author of this report (MS) who was on the panel believes that no significant issues were raised relating to the model structure.
For non-hospitalised patients, the model structure was based on that outlined in an unpublished report by the NICE Decision Support Unit which provided an early economic evaluation of neutralising monoclonal antibodies and oral antivirals for treating COVID-19 prior to hospitalisation. 24 This consisted of a decision-tree approach where patients who ultimately required hospital admission were evaluated in the hospital-based structure, whereas those that didn’t, remained in the community.
This section initially describes the model structures briefly, with later sections providing detail on the population of the parameter values used to generate the results within this report. Cost data were expressed in Great British pounds, reflecting prices for the year 2022. Costs were estimated from an NHS and Personal Social Services perspective. The costs and consequences of each strategy were estimated for a lifetime horizon with an annual discount rate of 3.5% being applied for costs and benefits expressed in QALYs.
Due to the timescales of the project no systematic review was undertaken for inputs such as costs and utility values. The default values were taken from a mixture of Rafia et al.,22 data sourced from papers known to the authors, pragmatic, non-systematic searches and from suggestions made by stakeholders at consultation and following the publication of the ACD by NICE.
Model structures
General model structure for hospitalised patients
The economic model was developed in Microsoft Excel® (Microsoft Corporation, Redmond, WA, USA) and uses a partitioned survival approach (often referred to as area under the curve approach) with three mutually exclusive health states; (1) discharged from hospital and alive, (2) hospitalised with or without COVID-19 and (3) death from any cause (COVID-19 or due to other causes).
Movements between health states were not explicitly modelled. Instead, the partitioned model estimates health state occupancy at each time interval. Figure 1 shows a simplified schematic of the model structure. A daily cycle length is used until the end of parametric extrapolation, at day 70, after which a weekly cycle length is used. An initial daily cycle length was chosen to allow changes in treatment and/or hospitalisation and oxygen requirements that happen early in a patient’s stay to be modelled at a granular level. A cohort partitioned survival approach was used due to the limited time, the absence of individual patient-level data (IPD) that may allow a more complex model structure and the need to not explicitly model transitions between health states as would be required by a state transition model. A limitation of the partitioned survival approach is that it is not possible to track individual patients in the model which may have allowed a better representation of the patient experience.
FIGURE 1.
Simplified schematic of model structure (values are for illustration only).

While in hospital, the 8-point ordinal scale of clinical status (an inverted version of the scale originally developed for severe influenza requiring hospitalisation as recommended by the WHO) used in the Adaptive COVID-19 Treatment Trial (ACTT-1) RCT,25 and in the Remdesivir Effectiveness Evaluation Study26 is used. This ordinal scale is described in Table 6 and is used in the model to (1) define the population at baseline in terms of oxygen requirements at the start of treatment, and (2) estimate changes in hospital/oxygen requirements during the hospital stay.
Clinical status | |
---|---|
1 | Not hospitalised and no limitations of activities |
2 | Not hospitalised, with limitation of activities, home oxygen requirement, or both |
3 | Hospitalised, not requiring supplemental oxygen and no longer requiring ongoing medical care (used if hospitalisation was extended for infection-control or other non-medical reasons) |
4 | Hospitalised, not requiring supplemental oxygen but requiring ongoing medical care (related to COVID-19 or to other medical conditions) |
5 | Hospitalised, requiring any supplemental oxygen such as low-flow oxygen (LFO) |
6 | Hospitalised, requiring non-invasive ventilation (NIV) or use of high-flow oxygen (HFO) devices |
7 | Hospitalised, receiving invasive mechanical ventilation (IMV) or extracorporeal membrane oxygenation (ECMO) |
8 | Death |
When evaluating the interventions, patients enter the hospital model based on the marketing authorisation, where this has been granted, or in relation to the population in the key studies. Table 7 provides information of the ordinal scales within which the interventions can be used, in line with their marketing authorisation (or anticipated marketing authorisation) of each intervention as illustrated in Tables 1–3. Scale scales 1 and 2 describe patients with COVID-19 in the community while ordinal scales 3 or higher describe patients in hospital, although ordinal scale 3 does not require ongoing medical care. Only ordinal scales 3 or higher are relevant for the hospital model.
Ordinal scale | |||||||
---|---|---|---|---|---|---|---|
Intervention | 1 | 2 | 3 | 4 | 5 | 6 | 7 |
Cas and imd | |||||||
Molnupiravir | Δ | Δ | Δ | ||||
Tocilizumab | | | | ||||
Nirm and rit | Δ | Δ | Δ | ||||
Remdesivir | | | | | | | |
Sotrovimab | Δ | Δ | Δ | ||||
Tix and cilg | Δ | Δ | Δ | ||||
Baricitinib | |||||||
Bari and rem |
Movements (improvement or worsening) between the different hospitalisation/oxygen requirements over time are modelled with each scale being associated with cost and HRQoL implications. During their hospital stay, patients are distributed according to their hospital/oxygen requirement derived from the placebo arm of the ACTT-1 study and additional assumptions where necessary. Figure 2 provides an illustration of movement between ordinal scales for patients who needed supplemental oxygen on hospital entry and when treated with SoC. The area above Ordinal Scale 7 denotes patients who have died; the area below Ordinal Scale 3 signifies patients discharged from hospital.
FIGURE 2.
Illustration of ordinal scale occupancy during hospital stay of a cohort admitted to hospital requiring supplemental oxygen and receiving SoC treatment.
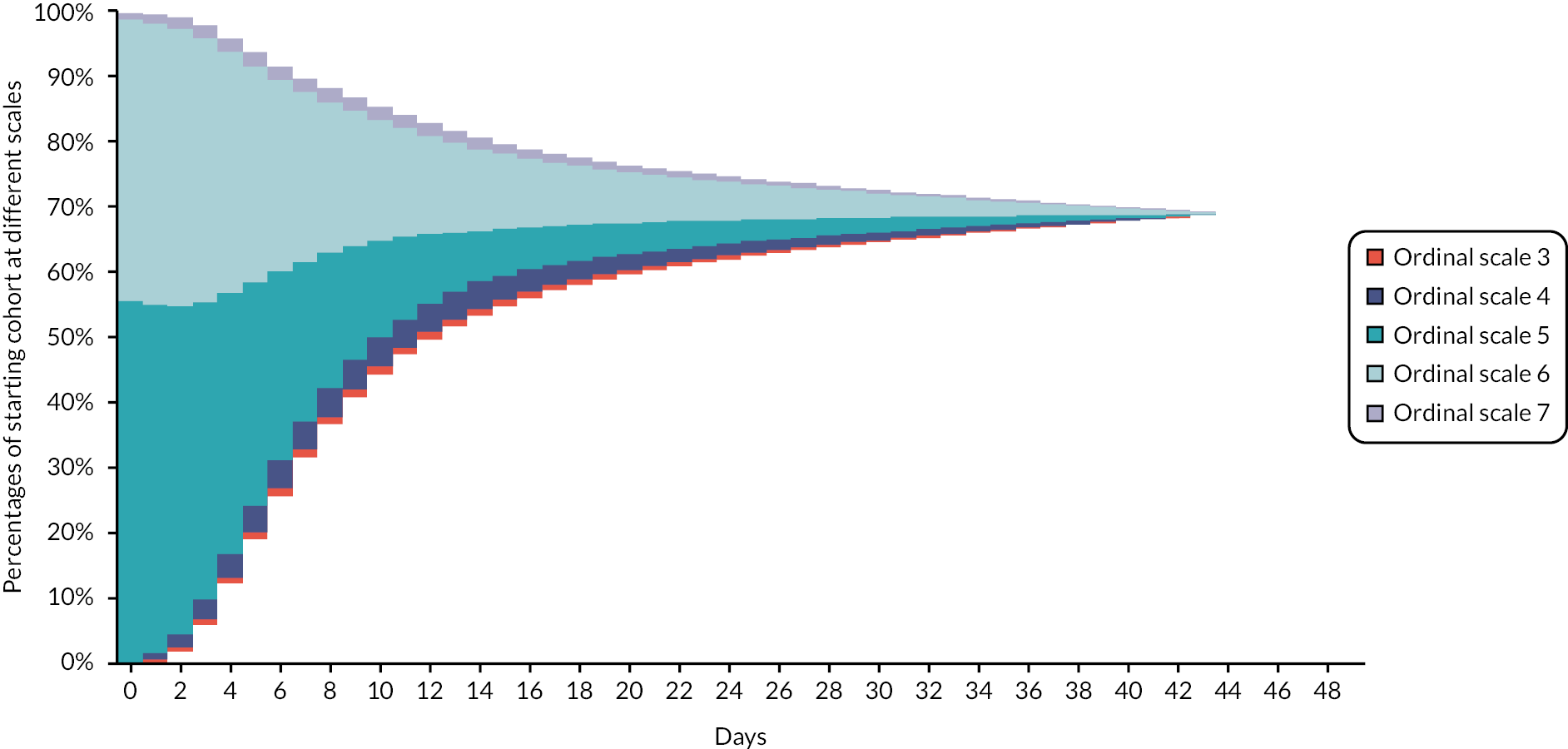
Following Rafia et al. 22 the model assumes that all patients are discharged at 70 days. This may underestimate the costs and QALY losses associated with hospital care for the most efficacious drugs, although this is not expected to be a large limitation as the proportions of patients estimated to be in hospital at day 70 is very small. For example, in the mean efficacy scenario this proportion was zero for all interventions.
Pivotal clinical trials/studies for treatments for COVID-19 used in this economic evaluation tend to follow patients and typically collect key clinical outcomes after 28 days of follow-up. It is, therefore, necessary to extrapolate beyond the duration of studies to capture the life expectancy and HRQoL following hospital discharge from COVID-19. Following discharge, patients who were hospitalised with COVID-19 are at an elevated risk of death;27 emerging evidence suggests that some patients discharged continue to experience symptoms and have a reduced quality of life,28–37 may require readmission due to COVID-19,25,38–42 and are at an elevated risk to experience multiorgan dysfunctions27 (such as respiratory diseases, diabetes, cardiovascular, liver and kidney diseases) and may require long-term management/monitoring. 43 Within the model, HRQoL reductions and additional costs associated with COVID-19 have been included; for brevity this has been termed ‘long COVID’. In addition, the possibility of patients having an increased risk of death following COVID-19 has been modelled using a standardised mortality rate (SMR) applied to the mortality rates for an age- and sex-matched population.
Consequently, a seven-step approach is employed:
-
Step 1: Use of a parametric function (hazard spline model with three knots) fitted to the relevant outcomes (time to death and time to discharge) for all patients on the SoC arm in Randomised Evaluation of COVid-19 thERapY (RECOVERY) study44 for the first 28 days, as used in Rafia et al. 22
-
Step 2: This parametric function is adjusted to reflect the outcomes at day 28 as reported in the literature to reflect the benefit of using corticosteroids, which represent the current SoC for patients in need of supplemental oxygen. 43 The model was calibrated as detailed in Time to hospital death in patients initiating SoC (with or without corticosteroids).
-
Step 3: Treatment effect in the form of HRs or RRs for the interventions were applied to the SoC curves. Data were missing for some interventions with respect to the HR for discharge and the HR for clinical improvement (see Results of the rapid evidence review). The EAG noted that these HRs were not large drivers of the cost-effectiveness results, and that there was no clear relationship between the two HRs and other results, such that an estimation could be made. As no values for interventions with data were markedly different from unity when compared with SOC, the EAG decided to use the values for SoC where data were missing, with a sensitivity analysis undertaken using a HR of 1.0 for all interventions which is likely to be favourable to casirivimab/imdevimab in relation to time of discharge and baricitinib/remdesivir in relation to clinical improvement.
-
Step 4: As shown in Figure 2, ordinal scale occupancy in hospital is assumed to last until the distribution for overall survival (OS) and the distribution for time to discharge intersect. It was assumed in the model that none of the hospitalised cohorts would remain in hospital after 70 days.
-
Step 5: Parametric extrapolation is employed to estimate the rates of death from day 28 until day 70 in the base case.
-
Step 6: Use of mortality rates from the general population, adjusted by an SMR for the assumed mean duration of long COVID to reflect the elevated risk of death in patients with COVID-19 discharged from hospital.
-
Step 7: Use of unadjusted mortality rate from the general population after the assumed mean duration of long COVID.
General model structure for non-hospitalised patients
The model structure used for assessing interventions for patients with COVID-19 and at high risk of hospitalisation is depicted in Figure 3. This is comprised of a decision tree which simulates whether hospitalisation is required, and for those patients who are hospitalised, whether supplemental oxygen is required on admission. Patients who are hospitalised were assumed to enter a partitioned survival model as described in General model structure for hospitalised patients.
FIGURE 3.
Structure of the decision tree used for the non-hospitalised cohort.
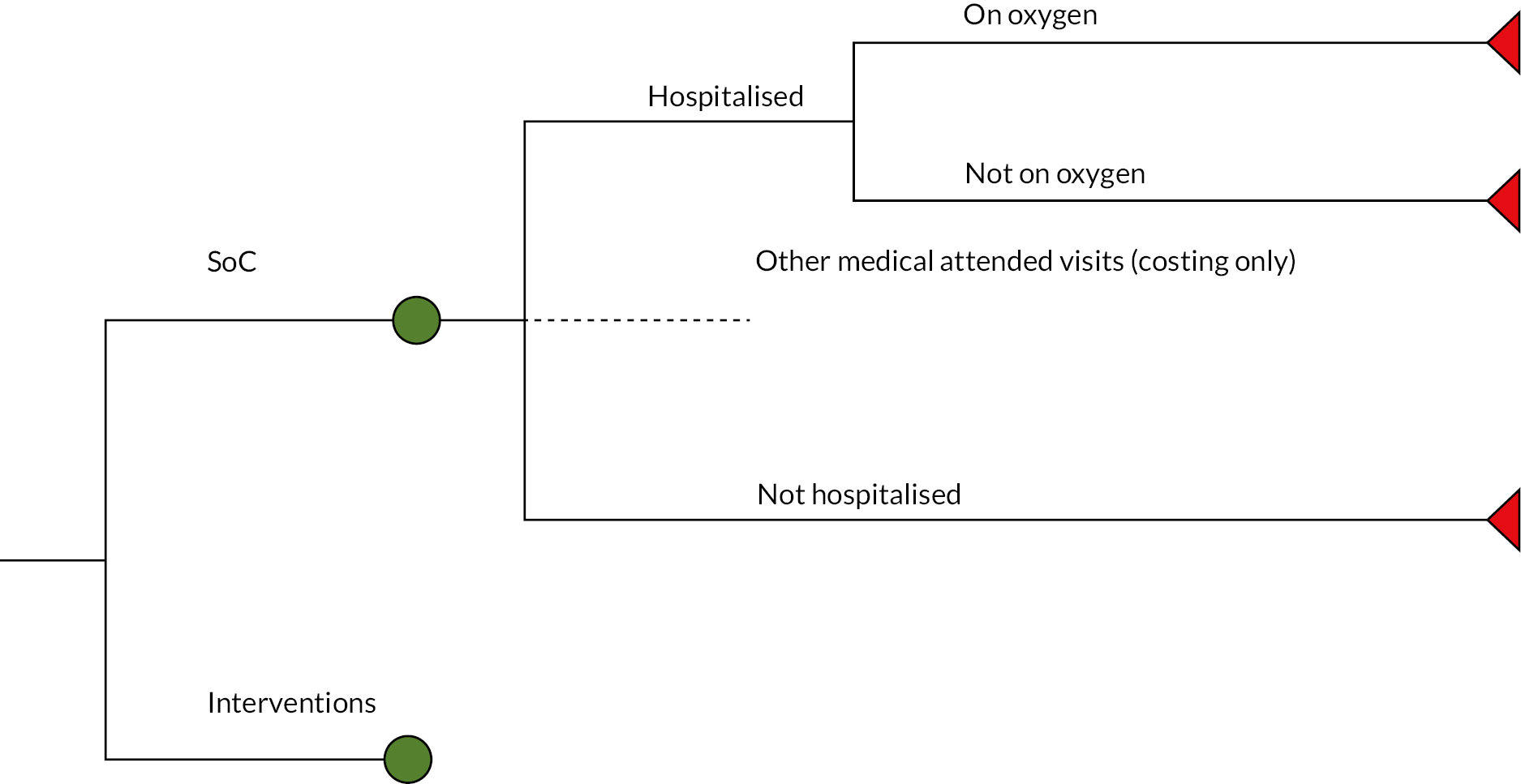
The EAG’s central estimate of the probability of patients at high risk of being hospitalised was taken from Patel et al. 13 which was a retrospective cohort study of non-hospitalised patients who received early treatment for, or were diagnosed with COVID-19 or had a positive polymerase chain reaction test between 1 December 2021 and 31 May 2022 using the Discover dataset in North-West London. Four thousand forty-four untreated patients did not receive treatment with a monoclonal antibody or an antiviral. The untreated cohort had a median age of 52 years and 86% had received two or more vaccinations. One hundred fourteen patients were hospitalised with a primary diagnosis of COVID-19 within 28 days of COVID-19 diagnosis equating to 2.82%. This value has been used in the base case. The EAG notes that this value aligns with the definition of high-risk detailed in a report45 produced for the Department of Health and Social Care, but believes that this, along with the sensitivity analyses conducted will be informative for decision-making.
Three additional sources for hospitalisation for high-risk patients in the community were also considered. These were: Hippisley-Cox et al.,46 the PANORAMIC study47 and Shields et al. 48
Data from Hippisley-Cox et al. 46 indicated an average risk of hospitalisation following a SARS-CoV-2 positive test was 1.45% based on approximately 1.3 million people in England, although the EAG notes that it would expect the value based on a positive SARS-CoV-2 test to be lower than based on having COVID-19 (which is people with SARS-CoV-2 who are symptomatic). The risk of hospitalisation was markedly increased in patients with Down syndrome, patients with kidney transplant, chemotherapy grade B or C and rare neurological conditions with midpoint HRs > 4. Many conditions were associated with increased risks of hospital admission, although vaccination and prior SARS-CoV-2 infection were associated with lower risks.
Data from the PANORAMIC study47 indicated a lower proportion of high-risk people were hospitalised with 96 of 12,525 (0.77%) untreated patients requiring hospitalisation. Stakeholders, however, stated that high-risk patients may not have been recruited to PANORAMIC and were instead treated by COVID Medicines Delivery Units (CMDU) and that the proportion of hospitalised patients would be underestimated.
Data reported by Shields et al. 48 indicated that the risk of hospitalisation for immunodeficient individuals who did not receive treatment was 15.9%.
In sensitivity analyses (see Analyses undertaken) the hospitalisation rate is changed from 2.82% to 1%, 5%, 10% and 20% to provide a comprehensive range for Committee discussion. These ranges would allow decision-makers to explore the cost-effectiveness of treatments in subgroups that are of greater, or lower, risk of hospitalisation.
The proportion of hospitalised patients requiring supplemental oxygen was estimated from an International Severe Acute Respiratory and Emerging Infection Consortium report49 where the requiring oxygen of any level on admission was calculated at 81% (55% HFO 16% non-invasive ventilation and 10% invasive ventilation). These proportions were assumed to be independent of treatment (intervention or SoC) due to lack of data, and it is plausible that if a person requires hospitalisation, then the intervention has not worked. The EAG ran a sensitivity analysis assuming that the proportion requiring supplemental oxygen was reduced to 75% [with 60% on HFO 10% NIV non-invasive ventilation and 5% invasive ventilation] for patients who have received an intervention.
The model applies an RR to account for other medical attended visits (MAVs) (i.e. visits other than hospital admission) compared to admissions. This RR was estimated from data in Nyberg et al. 50 and was equal to 1.37 (1.23% MAV rate divided by 0.9% hospitalisation rate). Only costs were considered for MAVs and incorporated a visit to an accident and emergency department.
Two key clinical outcomes were extracted from the living systematic reviews: RRs for hospitalisation or death, and RRs for day 28 all-cause mortality, which are shown in Figures 26 and 27, respectively. The RR for hospitalisation or death was assumed to apply for hospitalisations only due to the relatively low mortality rate compared to the admission rate. A separate RR was calculated for each intervention for deaths within hospital such that the overall RR for death at 28 days was consistent with the published estimate reported in Tables 4 and 5. This methodology assumes that there were no deaths among non-hospitalised patients in the first 28 days of the model. The EAG believes that this limitation would have a negligible impact on the ICER.
The EAG assumed that there would be no further active treatment in hospital for patients treated in the community, and thus patients receive SoC only. This decision was based on the following factors: the RRs for mortality for some of the interventions used in the community were substantially lower than the HRs for those treatments used in hospital. For example, the RR for death for nirmatrelvir/ritonavir was 0.04 while the median HR for death for baricitinib was 0.61, indicating that the residual effect of nirmatrelvir/ritonavir was larger than the impact of baricitinib, which had the most efficacious median value. Furthermore, there is no evidence for the synergistic effects (or not) of using multiple interventions.
In line with NICE’s final scope the model does not consider the impact of treatment on the transmission of SARS-CoV-2. 7 The community model may also slightly overestimate the costs associated with people in hospital at the time of catching COVID-19 as hospitalisation costs may be double-counted, although the EAG believes this will be of limited importance.
The modelling did not assess the logistical aspects of treatment in the community, but the EAG notes that this could be a large factor in deciding which treatments could be preferred, as oral treatments could be more acceptable to patients and healthcare systems than treatments that are given intravenously or subcutaneously.
Clinical parameters and inputs used un this rapid assessment
Baseline characteristics after discharge
The economic model uses age and gender distributions to estimate both the rate of mortality beyond the duration of clinical evidence and to estimate HRQoL values for patients discharged from hospital and patients at high risk remaining in the community. The baseline mean age for the modelled hospitalised cohort was calculated from weekly Office for National Statistics (ONS) data51 reported in the middle of May 2022. For patients with COVID-19, these data included rates of hospital admissions per 100,000 people and number of deaths, by age bands. These values were multiplied by population data obtained from the ONS52 to estimate the absolute number of admissions and deaths by age band. The estimated number of discharged patients was calculated by subtracting the number of deaths from the number of admissions. Table 8 presents the estimated numbers and percentages calculated for admission, death and discharge conditional on age band.
Age band (years) | Hospital admission, n (%) | Death, n (%) | Discharge, n (%) |
---|---|---|---|
0–14 | 196 (3.9) | 2 (0.3) | 194 (4.4) |
15–24 | 126 (2.5) | 0 (0.0) | 126 (2.9) |
25–44 | 478 (9.4) | 7 (1.0) | 471 (10.7) |
45–54 | 237 (4.7) | 6 (0.9) | 231 (5.3) |
55–64 | 545 (10.8) | 29 (4.3) | 516 (11.8) |
65–74 | 761 (15.0) | 97 (14.4) | 664 (15.1) |
75–84 | 983 (19.4) | 209 (31.0) | 774 (17.6) |
85+ | 1737 (34.3) | 324 (48.1) | 1413 (32.2) |
Overall | 5062 (100) | 674 (100) | 4388 (100) |
If the midpoint of each age band represented the entire band, mean ages for admission, death and discharge are estimated at 70.6, 82.8 and 68.7 years, respectively. Without data to accurately estimate the age for people with COVID-19 at high risk of hospitalisation who do not get hospitalised, the EAG assumed that this equalled the age of patients who had not been hospitalised in order to maintain the average starting age for all comparators, which was 55 years in the base case.
The distribution between sexes was taken from an Intensive Care National Audit and Research Centre report53 which reported that 38.3% of patients admitted to hospital from May 2021, in a critically ill state due to confirmed COVID-19, were female.
Time to hospital death in patients initiating SoC (with or without corticosteroids)
The EAG used the following steps to estimate the survival of patients admitted to hospital due to COVID-19 and receiving SoC.
The Kaplan–Meier (KM) estimate for OS was taken from the control arm of the RECOVERY study,44 and was digitised which allowed pseudo-IPD to be reconstructed based on the algorithm developed by Guyot et al. 54 A spline model (hazard scale) with 3 knots was subsequently fitted to the pseudo-IPD using the R package flexsurv and employing a natural cubic spline function. This model was selected over standard parametric functions (such as the exponential, Weibull, Gompertz, Log-Normal, Log-Logistic, Gamma and Generalised Gamma) to increase the accuracy in the estimate and because parametric extrapolation beyond the observed period of the trial was limited to a maximum of 70 days. This distribution was then calibrated to the current data such that 73.5% of patients were alive for the population in need of oxygen and 86.0% of patients were alive for the population admitted with no need of supplemental oxygen at 28 days. These values were taken from a NICE rapid guideline19 assuming that the outcomes for patients without corticosteroid use were generalisable to patients requiring supplemental oxygen and the outcomes for those patients with corticosteroids were generalisable to patients not requiring supplemental oxygen. This decision was made as corticosteroids were only seen to be efficacious in patients not requiring supplemental oxygen. For illustration, Figure 4 shows the OS curves used in the model for SoC and remdesivir by oxygen requirement at hospital admission; the remdesivir data was calculated by applying the HR shown in Table 4.
FIGURE 4.
Illustration of OS curves used for the hospitalised cohort for SoC and remdesivir by oxygen requirement at entry.

Time to discharge for patients initiating SoC
The methodology for calculating time to discharge for patients receiving SoC was similar to that for time to death [see Time to hospital death in patients initiating SoC (with or without corticosteroids)] with the following changes. The KM estimate for time to discharge was taken from the control arm of the RECOVERY study,44 and a spline model (hazard scale) with three knots selected. This distribution was then calibrated to the current data such that 64.0% of patients for the population in need of supplemental oxygen and 80.4% of patients with no need of supplemental oxygen were discharged at 28 days. These values were taken from a NICE rapid guideline19 assuming that the outcomes for patients without corticosteroid use were generalisable to patients requiring supplemental oxygen and the outcomes for patients using corticosteroids were generalisable to patients not requiring supplemental oxygen. For illustration, Figure 5 shows the time to discharge curves used in the model for SoC and casirivimab/imdevimab by oxygen requirement at hospital admission; the data was calculated applying the HR shown in Table 4.
FIGURE 5.
Illustration of time to discharge curves used for the hospitalised cohort for SoC and casirivimab/imdevimab by oxygen requirement at entry.

Redistribution of patients according to supplemental oxygen/hospitalisation requirements
In order to estimate costs and QALYs during an average hospital stay, it was necessary to model how patients move between the 8-point ordinal scale as each scale has different consequences in terms of the costs of treatment and the HRQoL of the patient. Hospitalised patients with COVID-19 may receive supplemental oxygen, defined as LFO, HFO and mechanical ventilation. However, during their hospital stay, patients may require more or less intensive management. Hospitalised patients are divided into five states, which correspond to ordinal scales 3 to 7.
Assumed distribution of patients on the 8-point ordinal scale on hospital entry
By definition, all patients admitted to hospital due to COVID-19 without the need for supplemental oxygen are in ordinal stage 4. For patients requiring supplemental oxygen, data from ACTT-125 which reported the distribution of ordinal score by treatment for placebo on admission to hospital were used. These data however do not reflect the distribution of current admissions as the percentage requiring invasive mechanical ventilation (IMV) or extracorporeal membrane oxygenation (ECMO) (ordinal stage 7) was 46%, however, a recent value suggests that this was only 1%. 53 The distribution from ACTT-1 was adjusted such that only 1% of patients resided in ordinal stage 7 with those patients reallocated from ordinal stage 7 being redistributed between ordinal stages 5 and 6, according to their relative weight in the ACTT-1 study. Table 9 shows the proportions of patients across the ordinal health stages at baseline for those requiring supplemental oxygen and those not requiring supplemental oxygen, respectively. The EAG is aware that remdesivir should not be used at ordinal scale 7 but considers this to be a minor limitation given the small proportion of patients in this scale.
Ordinal health scale | Not requiring supplemental oxygen | Requiring supplemental oxygen | ||
---|---|---|---|---|
Assumed proportion on entry to hospital (day 0), % | Assumed proportion of patients alive at day 14, % | Assumed proportion on entry to hospital (day 0), % | Assumed proportion of patients alive at day 14, % | |
3 | 0 | 21 | 0 | 4 |
4 | 100 | 36 | 0 | 15 |
5 | 0 | 26 | 56 | 28 |
6 | 0 | 14 | 43 | 46 |
7 | 0 | 3 | 1 | 7 |
Distribution of hospitalised patients between the ordinal stages on SoC at day 14
Beigel et al. 25 report data from the ACTT-1 study for the placebo arm which detailed the ordinal stage distribution at baseline and 14 days later. Because of small numbers, which would have meant that movement between some stages was impossible, a continuity correction was added for all possible transitions, splitting one new observation at day 14 equally over the five ordinal scales.
However, ACTT-1 was an early study and there have been many changes such as the vaccination programme, increased use of corticosteroids and changes in SARS-CoV-2 variants. These changes have meant that the results from this study are no longer generalisable to the UK, particularly in terms of the proportion of patients who reach ordinal scale 7 and require IMV or ECMO. In ACTT-1, the EAG calculated that the percentage of patients’ time spent in ordinal scale 7 was 48%; contrastingly, this value has been reported in May 2022 to be only 4.12%. 55 The ACTT-1 data was calibrated so that the percentage of time in ordinal stage 7 was equal to 4.12%, with the patients no longer allocated to ordinal scale 7 being allocated to ordinal stage 6 instead. The decision to allocate to ordinal stage 6 was to avoid a situation where the predicted outcomes for patients at stage 7 on hospital entry were better than those for patients admitted at ordinal stage 6. The estimated proportions of patients in hospital across the ordinal health stages at day 14 are shown in Table 9 divided by patients not requiring supplemental oxygen and those requiring supplemental oxygen.
Movement between ordinal scales between day 0 and day 14
We assumed that for patients in hospital, the distribution of patients changes linearly from the proportion in each ordinal health scale at baseline to the proportions in each ordinal scale assumed at day 14. For simplicity, these proportions were assumed to remain constant after day 14 until the end of hospitalisation (day 70). Figure 29 in Appendix 3 provides the assumed distribution between ordinal scales over a 28-day period for patients in hospital who required supplemental oxygen at baseline.
Treatment effects for interventions compared with SoC
The treatment effects for interventions are summarised in Tables 4 and 5. Where data were not available for clinical improvement or time to discharge a value of 1.0 was used as the model results were not sensitive to these values within the observed range associated with other interventions. A value of 1.0 indicates that the level of clinical improvement and time to discharge are the same for an intervention and for SoC. The RRs for clinical improvement were only applied for improvements of two ordinal scales or more as per the outcome definition in the early trials. 56
Duration of treatment/number of doses
The dosage information data were taken from the NICE COVID-19 rapid guideline. 19 Where either the dosage or the duration of treatment was not available, this information was taken from alternative sources. Table 10 summarises the dosage information used in the model.
Intervention | Dosing | Source |
---|---|---|
Casirivimab/imdevimab | 600 mg of both drugs administered together once | Marketing Authorisation |
Molnupiravir | 800 mg twice daily for 5 days | NICE guideline19 and Marketing Authorisation |
Tocilizumab | Single dose of 8 mg/kg with a maximum of 800 mg. Assumed 50% will receive the maximum dose with the rest getting 600 mg | NICE guideline,19 Marketing Authorisation and an assumption |
Nirmatrelvir/ritonavir | 300 mg of nirmatrelvir and 100 mg of ritonavir twice daily for 5 days | NICE guideline19 and Conditional Marketing Authorisation |
Remdesivir | 100 mg once daily for 5 days for the hospital setting, and 3 days for the community setting. A 200 mg loading dose on day 1 was used for both | NICE guideline19 |
Sotrovimab | 500 mg single infusion | Conditional Marketing Authorisation |
Tixagevimab/cilgavimab | 600 mg of both drugs administered together once | Montgomery et al. 202257 |
Baricitinib | 4 mg once daily for 14 days or discharge whichever earlier | Recommended dose and COVID-NMA Initiative12 |
Mortality rate assumed posthospitalisation and for those people who did not require hospital admission
The unadjusted rate of mortality for the general population is taken from the England and Wales life table 2018–20. 58 After discharge, patients hospitalised with COVID-19 were assumed to be at an elevated risk of death while they have long COVID. An SMR of 7.7 (7.2–8.3) was applied based on the RR reported by Ayoubkhani et al. 27 which was estimated from 47,780 patients treated for COVID-19 in NHS hospitals and discharged alive, using matched-controls and which had a median follow-up of 140 days. This SMR was also applied to patients at high risk in the community for the period in which they were simulated to have long COVID.
Serious adverse events
While the living systematic reviews allowed the RRs related to SAEs to be extracted, on inspection the ERG identified that these were not events related to the unwanted impacts of the interventions but were conditions related to severe COVID-19. As such, many interventions were associated with less SAEs than SoC, which is generally atypical for efficacious pharmacological treatments. As the model was explicitly tracking the severity of patients using the 8-point ordinal scale the EAG decided to omit SAEs from the model. The degree to which this is favourable, or unfavourable to specific treatments is unknown.
Long COVID
To facilitate modelling, the authors have not strictly adhered to previously defined definitions of long COVID but have taken a simplistic approach with sensitivity analyses undertaken to assess the uncertainty of the impact of long COVID in the base case.
The prevalence of long COVID within the wider community has been taken from an ONS report dated 6 May 2022,59 which in supplementary tables reports adjusted model estimates for long COVID of any severity and at any point since the last vaccine of: 8.7% of double-vaccinated patients and 8.0% of triple-vaccinated patients, who had the Omicron BA 1 variant; and 15.9% of double-vaccinated patients and 8.6% of triple-vaccinated patients, who had the Delta variant. Having noted the relatively wide CIs for the ONS estimates, the difference depending on vaccination status (with no data reported for unvaccinated patients) and the method it proposes to use for estimating the duration of long COVID (described below), the EAG assumed that 10% of patients in the community who were at high risk of severe COVID-19 but did not need hospitalisation would experience long COVID. The EAG was not aware of any evidence on the impact of community treatment on the incidence of long COVID and thus assumed that long COVID was independent of treatment. The degree to which this is favourable, or unfavourable to specific treatments is unknown. More recent data has been released (with a date of July 2022,60 although this did not influence the decision made by the EAG).
The ONS released an updated report in December 2022 on the prevalence of ongoing symptoms following COVID-19 in the UK. 14 This stated that of people with self-reported long COVID, defined as ‘symptoms continuing for more than four weeks after the first suspected coronavirus (COVID-19) infection that were not explained by something else’ 87% of people had been first infected by COVID-19 (or suspected they had) at least 12 weeks earlier, 55% were infected at least 1 year previously, and 27% at least 2 years previously.
The EAG fitted simple parametric distributions to the three reported estimates of at least 12 weeks duration (87% with long COVID at 12 weeks, 55% at 1 year and 27% at 2 years). A Gamma distribution (shape = 74.373, scale 1.089), a Weibull distribution (shape = 1.065, scale 82.049) and a log-normal distribution [mean = 3.998, standard deviation 1.212 (both on the log scale)] were observed to fit the data well. The mean survival times from these distributions were 81.0 weeks (Gamma), 80.1 weeks (Weibull) and 113.6 weeks (log-normal).
The plot of the log-normal and the Weibull parametric fits, which have the highest and lowest mean times of the distributions that fitted the data well are shown in Figure 6, with the log-normal used in the base-case. For the log-normal distribution, approximately 30% of people still have symptoms at 2 years, 10% at 5 years and 3% at 10 years.
FIGURE 6.
Assumed duration of long COVID.

For its base case, the EAG assumed the log-normal distribution was most appropriate as data may be administratively censored in that patients who had COVID-19 only 6 months ago could not report having symptoms at 2 years, and the EAG noted that the proportions of people reporting long COVID symptoms for at least 2 years had increased from 19% in a June report61 to 27% in the December report. The EAG undertook sensitivity analyses halving and doubling the mean duration, the range of which includes the mean from the Gamma distribution. The EAG notes that its analyses are simplistic as formal survival analysis methods were not used, and that it does not assume that all patients must have long COVID for at least 4 weeks, as used in some definitions but believes that the analyses undertaken are informative for decision-making despite this limitation.
From Evans et al. 62 it is estimated that at approximately 6 months, 51.7% of patients with non-missing data (n = 830) reported that they had not recovered from COVID-19; this value increases to 71.2% when patients stating they were not sure if they had recovered were included. The patients included in the study were hospitalised early in the pandemic (between March and November 2020) and it is unclear how generalisable this result is to patients hospitalised in 2022. The best-fitting log-normal and Weibull distributions shown in Figure 6 estimate the proportions of patients not recovered from long COVID to be 72.9% and 74.5% at 26 weeks which is similar to the value reported in Evans et al. 62 when those not sure if they have recovered are included. Given the uncertainty in patients who stated they were not sure if they had recovered, a simplistic assumption was made that all patients hospitalised due to COVID-19 would suffer long COVID. The EAG was not aware of any evidence on the impact of hospital treatment on the incidence of long COVID and thus it was assumed that this is independent of treatment. The degree to which this is favourable, or unfavourable to specific treatments is unknown.
While the simplistic approach used does not capture any potential differential severities of long-term effects based on initial severity of COVID-19,63 the impact of vaccination status, or the consequences of any organ damage caused by long COVID the authors believe that this method is still informative for decision-making.
Costs and health-related quality of life
Drug acquisition costs
While the EAG acknowledges that some stock may have already been acquired before this appraisal, recommendations are to inform future commissioning decisions and so the list price is used in this report. Drug acquisition costs, both list prices and prices with Patient Access Scheme (PAS) discounts applied were provided to the EAG by NICE. All analyses in this report have used list prices, with analyses using the PAS for baricitinib, sotrovimab and tocilizumab included in a confidential appendix. Table 11 summarises the list prices used in the model. Three drugs had list prices which were not publicly available: casirivimab/imdevimab, molnupiravir and tixagevimab/cilgavimab. No cost-effectiveness results are presented for these interventions in this report but will be contained in a confidential appendix; however, results related to QALYs gained through the use of these treatments will be presented. For corticosteroids, daily costs were assumed to be negligible compared to the in-hospital day cost and were not included for simplicity.
Intervention | List price | Notes |
---|---|---|
Tocilizumab | £512.00 £256.00 |
Price for 1 vial of 400 mg tocilizumab Price for 1 vial of 200 mg tocilizumab |
Nirmatrelvir/ritonavir | £829.00 | Price for 20 nirmatrelvir tablets and 10 ritonavir tablets |
Remdesivir | £340.00 | Price for 1 vial of 100 mg remdesivir |
Sotrovimab | £2209.00 | Price for 1 vial of 500 mg sotrovimab |
Baricitinib | £805.56 | Price for a pack of 28 tablets, each contains 4 mg baricitinib |
Baricitinib and remdesivir | As component interventions | As component interventions |
Administration costs
The EAG assumed that the costs associated with treatment administration while in hospital would be incorporated in the unit costs associated with hospitalisation (see Unit costs associated with hospitalisation). NICE provided the EAG with information from the CMDU relating to how indicative local tariffs were calculated. The costs included elements for: staffing, administrative support, dispensing, clinical consumables, couriering medicines, travel, stationery and hiring rooms, but excluded medical review to assess drug interactions and any changing in permanent staffing structures.
The costs associated with providing oral antivirals were estimated to be £410 per person, whereas the costs associated with intravenous (i.v.) infusions were estimated to be £820 per person. For simplicity, the costs associated with administering injections were assumed to be the same as oral antivirals. Within the analyses it has been assumed that there is likely to be a delay in patients receiving intravenous casirivimab/imdevimab and that a subcutaneous version would be used instead.
Stakeholders commented that due to drug interactions, additional time related to medication reviews would be required for some interventions and that these costs should be incorporated in the model. The need for additional time and the costs associated with this time are both uncertain so they have not been included in the model. However, the EAG comments that due to the NMB approach taken, any determined costs associated with additional medication review could be subtracted from the NMB values, allowing the NICE appraisal committee, and other stakeholders, to determine the relative cost-effectiveness of interventions.
Unit costs associated with hospitalisation
Following stakeholder comments some sources for costs have been updated and the latest version of the National Schedule of NHS costs (2020–21) has been used. 64 A stakeholder suggested alternative sources for the costs associated with ordinal scales 3, 4 and 5 which were as follows: for ordinal scale 3, a weighted average of currency codes DZ11R to DZ11V (Lobar, Atypical or Viral Pneumonia, without Interventions); for ordinal scale 4, a weighted average of currency codes DZ11N to DZ11Q (Lobar, Atypical or Viral Pneumonia, with Single Interventions); and for ordinal scale 5 a weighted average of DZ11K to DZ11M (Lobar, Atypical or Viral Pneumonia, with Multiple Interventions). These appeared plausible and the EAG estimated the cost per bed-day by dividing the average costs per currency code by the average length of stay per currency code taken from the 2017/2018 National Schedule of NHS cost65 as no later data on length of stay existed. However, the results lacked face validity as the estimated average cost per bed-day was less in ordinal scale 5 than in ordinal scales 3 and 4.
The EAG used an alternative approach which generated plausibly valid costs per bed-day for the ordinal scales. These costs were larger than those in the report sent out for stakeholder consultation, apart from ordinal scale 3 which is lower. In response to the consultation on the ACD a stakeholder indicated that there was a better way to calculate the costs of a bed day in hospital in ordinal scales 4 or 5 as the cost per finished cost episode (FCE) was used to approximate the cost per bed day and there can be multiple FCEs per admission. The EAG has reviewed these approaches and agrees that these are an improvement and preferred the approach where the costs of being in ordinal scale 5 were greater than in ordinal scale 4. The EAG calculated values using this approach (described below) and produced slightly higher cost values than the consultee; the higher costs were used in the model. The NHS currency codes used to estimate the costs for ordinal scales 4, 6 and 7 are detailed in Table 12.
Ordinal scale | Clinical status | Unit cost | Source | Utility decrement (unless stated) | Source |
---|---|---|---|---|---|
3 | Hospitalised, no longer requiring ongoing medical care | £248 | National Schedule of NHS costs 2020–2166 Using the weighted average of DZ11R to DZ11V (lobar, atypical or viral pneumonia, without interventions) for a regular day or night admission |
0.36 | Wilcox et al. (2017)67 |
4 | Hospitalised, not requiring supplemental oxygen | £759 | National Schedule of NHS costs 2020–2166 and NHS Digital, Hospital Episode Statistics for England. Admitted Patient Care statistics, 2020–2168 Using the weighted average cost of DZ19R – DZ19V (lobar, atypical or viral pneumonia, without interventions) for non-elective long stay multiplied by the mean number of FCEs and divided by the weighted mean length of stay associated with primary diagnosis codes U07.1 and U07.2. |
||
5 | Hospitalised, LFO | £1166 | National Schedule of NHS costs 2020–2166 and NHS Digital, Hospital Episode Statistics for England. Admitted Patient Care statistics, 2020–2166 Using the weighted average cost of DZ19N – DZ19Q (lobar, atypical or viral pneumonia, with single intervention) for non-elective long stay multiplied by the mean number of FCEs and divided by the weighted mean length of stay associated with primary diagnosis codes U07.1 and U07.2. |
0.58 | Hollmann et al. (2013)69 |
6 | Hospitalised, HFO or NIV | £1977 | National Schedule of NHS costs 2020–2166 Using XC07Z (Adult Critical Care, 0 organs supported) |
||
7 | Hospitalised, receiving IVM or ECMO | £2393 | National Schedule of NHS costs 2020–2166 Using the weighted average of XC01Z, XC02Z, XC03Z, XC04Z, XC05Z and XC06Z (Adult Critical care, one or more organs supported) |
Utility value of 0 | Assumption |
The mean length of stay associated with COVID-19 was estimated from NHS Digital, Hospital Episode Statistics for England. Admitted Patient Care statistics, 2020–2168 using primary diagnosis codes U07.1 and U07.2 which suggested a weighted average of 10.6 days. From the same source, the weighted mean number of FCEs per admission for U07.1 and U07.2 was 2.29. The cost per FCE for ordinal scale 4 was calculated using the National Schedule of NHS costs66 as the weighted average of HRG codes DZ11R, DZ11S, DZ11T, DZ11U and DZ11V (lobar, atypical or viral pneumonia, without interventions) for non-elective long stay which was a value of £3524. Using the same source, the cost per FCE for ordinal scale 5 was calculated as the weighted average of HRG codes DZ11N, DZ11P and DZ11Q (lobar, atypical or viral pneumonia, with single intervention) for non-elective long stay which was £5411. The use of DZ11 has previously been used as a proxy for COVID-19 costs in a published paper. 70
The costs for ordinal scale 4 and 5 were calculated as the average cost per FCE (£3524 and £5411, respectively), multiplied by the mean number of FCEs per admission (2.29) and divided by the mean length of stay (10.6 days); this results in a cost per day of £759.28 in ordinal scale 4 and £1165.70 in ordinal scale 5.
Costs associated with COVID-19 for outpatients or following discharge
Monitoring costs
For simplicity, monitoring/follow-up was assumed to occur in the first year only. Following discharge, patients were assumed to undergo two chest X-rays and 6 GP e-consultations on average related to their COVID-19 as in Rafia et al. 22 A one-off cost of £384 was applied to all patients assuming the cost of a chest X-ray was £44 (taken from Stroke et al. 71 and inflated to 2019/2020 prices using the NHS Cost Inflation Index (NHSCII) pay and prices indices72) and the cost associated with a GP e-consultation was £49. 72
Costs associated with long COVID
During the ACD consultation period, a stakeholder highlighted a report relating to the costs associated with chronic fatigue syndrome/myalgic encephalomyelitis,15 that could be a better source for the costs of long COVID than that used in the initial report provided to NICE (Vos-Vromans et al. 73). Having reviewed this document, the EAG believes that this is preferable and has now adopted this source. Table 2.4 of Vos-Vromans et al. reports an annual cost of £2095 (in 2014/15 prices) for total health care cost. The ERG has inflated this value to 2020/2021 prices using Jones and Burns72 to assume a cost of £2267 per annum for those with long COVID.
Health-related quality of life
NICE’s preferred measurement of HRQoL is the EQ-5D74 which asks participants to value five domains of health (mobility, self-care, usual activities, pain/discomfort and anxiety/depression) on either a three-level scoring system [EuroQol-5 Dimensions, three-level version (EQ-5D-3L)] or a five-level scoring system [EuroQol-5 Dimensions, three-level version (EQ-5D-5L)]. A value of 1.00 generated by this instrument indicates perfect health whereas a value of 0.00 indicates a state equivalent to death.
Unadjusted baseline utility value by age
Baseline utility values (prior to any decrements/adjustments) are taken from Ara and Brazier based on the age-sex utility values (EQ-5D-3L) in the UK. 75
Health-related quality of life during the hospitalisation episode
Due to the nature of this rapid assessment, no formal systematic review of the literature was conducted to identify the most appropriate utility values. Hence, utility values (or decrements) were sourced from Rafia et al. 22 which estimated the utility of patients not requiring supplemental oxygen using patients with clostridium difficile infection as proxies and estimated the utility of patients requiring supplemental oxygen using patients with influenza (H1N1) as proxies. A stakeholder highlighted some systematic reviews of utility in COVID-19 patients, but all had limitations. Both Nobari et al. 76 and Hay et al. 77 focussed on general populations rather than those admitted to hospital whereas Walle-Hansen et al. 78 reported changes in the EQ-5D-Visual Analogue Scale, and in the change in each domain of the EQ-5D between scores before COVID-19 infection and 6 months after hospitalisation.
Health-related quality of life for high-risk patients with COVID-19 in the community
People at high risk of requiring hospital care with COVID-19 but who remain in the community without long COVID were assumed to have the same utility as the general age- and sex-matched population as detailed in Unadjusted baseline utility value by age. This is a simplification but one that the authors of the report believe would have limited impact due to the short duration of the acute COVID episode.
Health-related quality of life related to long COVID
A paper by Evans et al. 62 reported the impact on HRQoL following hospitalisation due to COVID-19. The EQ-5D-5L prior to hospitalisation was observed to be 0.84 but was 0.71 after hospitalisation, suggesting a utility impact of long COVID of 0.13. This value is not dissimilar to a reported utility loss in patients following severe sepsis. 79 It was assumed that this disutility would apply to all patients for their duration of long COVID. While the data in Evans et al. 62 indicated that utility loss was correlated with WHO class, with more severe patients having more utility loss, the EAG’s simplistic approach used the average value for all patients.
Analyses undertaken
Probabilistic sensitivity analysis (PSA) is the most appropriate method for providing the most accurate estimation of the ICER, however, this could not be undertaken within the timescales of the project. This was because there was a need to use the SOLVER function within Excel to calculate the proportion of patients treated in the community who are admitted to hospital, and die within this episode, as the model assumed that deaths due to COVID-19 only occurred in the hospital (see General model structure for non-hospitalised patients). This calculation added considerable computational time for each new parameter set precluding PSA, although to approximate the results from a PSA, the mean values for clinical effectiveness were used rather than median values in a ‘mean efficacy’ scenario.
The authors also stress that in this decision problem where there is considerable uncertainty in the efficacy of treatments it is anticipated that the difference in the ICERs from PSA compared to the point estimate would be small compared with the change in the ICER between the mean efficacy and the low efficacy scenarios, which reduces the benefit from a formal PSA and the lack of PSA was deemed a small limitation.
Three ‘deterministic’ analyses were run, which were (1) using the mean value for clinical effectiveness data, (2) using the most favourable limit of the 95% CI for clinical effectiveness data and (3) using the least favourable limit of the 95% CI for clinical effectiveness data. For each of the three, the median (which is typically also the mean) value was used for all other parameters. For brevity, the analyses have been referred to as ‘mean efficacy’, ‘high efficacy’ and ‘low efficacy’, respectively.
For patients in hospital due to COVID-19, the EAG has amended the low efficacy scenario in order that the treatments evaluated do not, on balance, harm patients, that is, at the very worst, the treatments would produce identical QALYs to SoC. This means that in the low efficacy scenario, a HR of unity was used for time to death for remdesivir and for baricitinib/remdesivir and a RR of unity for clinical improvement for remdesivir. The mean values from the estimated distributions have been left unchanged. The EAG believed it plausible that other aspects such as time to discharge and clinical improvement could plausibly be worse for treatments as a by-product of preventing death, and only capped these values at unity if the treatment were shown to have no benefit on mortality. As such, the RR for clinical improvement at 28 days for remdesivir was set to unity.
For patients treated in the community at high risk of COVID-19, the EAG has set the RR for all-cause mortality at 28 days to be unity in the low efficacy scenario for casirivimab/imdevimab, remdesivir, sotrovimab and tixagevimab/cilgavimab. The RR for-all cause mortality in the mean efficacy scenario was set to unity for remdesivir and tixagevimab/cilgavimab. The EAG has capped the RR for hospitalisation or death for molnupiravir at unity in the low efficacy scenario as it was deemed implausible that molnupiravir would have a positive impact on mortality but would markedly increase the number of hospitalisations.
The mean efficacy would be the efficacy expected if the conditions were exactly the same as during the studies contained in COVID-NMA12 and metaEvidence. 18 However, the high and low efficacy scenarios were for reasons of transparency and not knowing the ‘true’ efficacy were set to the lower and upper 95% limits of the CIs of reported efficacy, respectively. The EAG has acknowledged that this approach is arbitrary, and that the CI is influenced by the number of observed events and the sample size, such that two identical treatments could have markedly different confident intervals purely due to the size of the pivotal study. The EAG does not have a preferred base case as the impact of changing variants, vaccination status, prior infection and SOC are likely to affect efficacy observed in RCTs.
These analyses were supplemented by sensitivity analyses and are believed to provide the NICE appraisal committee with pertinent information relating to the true uncertainty in the decision problem, which is believed by the authors to be much larger than any difference between the mean results from a PSA and from a deterministic analysis using the mean of the distribution. As the efficacy of treatments is assumed to be independent, then there is considerable uncertainty in the true treatment effect (see Appendix 3, Figures 25 and 28) and it is plausible that one intervention had its ‘low efficacy’ value while another had its ‘high efficacy’ value. In such scenarios the more cost-effective treatment can be ascertained by comparing the NMB for each intervention using the appropriate scenario.
Seven sensitivity analyses were performed, which explored the impact of changing: (1) the duration of long COVID (ranging from half to double that of the base case); (2) changing the rate of hospital admission in the community with people being at ‘high risk’ of hospitalisation from a value of 2.82% to 1.00%, 5.00%, 10.00% and 20.00%; (3) changing the average age of patients at high risk of hospitalisation in the community from 55 years to 50 and 60 years; (4) using a HR of unity for all interventions in relation to time to hospital discharge and time to clinical improvement; (5) changing the baseline distribution of supplemental oxygen requirements from that associated with SoC (19% no supplemental oxygen, 55% HFO 16% NIV and 10% invasive ventilation) to an arbitrarily less severe baseline distribution (25% no supplemental oxygen, 60% high-flow oxygen, 10% NIV and 5% invasive ventilation) for patients who have received an intervention in the community; (6) assuming a utility decrement of 0.02 per day for patients receiving i.v. treatment in the community; and (7) changing the SMR for people during the period of long COVID from 7.7 to 5.0 and 10.0.
Following comments from consultees, the EAG noted that the results from the WHO Solidarity study which reported the results of 8275 hospitalised patients randomly allocated to either remdesivir treatment or control were not included in COVID-NMA. The company supplied an exploratory meta-analysis on the efficacy of remdesivir on time to death including the results from Solidarity which the EAG used to run as a scenario for remdesivir in the hospital setting. This uses a HR for time to death of 0.85 (95% CI 0.76 to 0.95). The mean value used in the EAG analysis is 0.85 with the high and low efficacy scenarios using the 95% CI. This scenario was run twice dependent on the assumption made regarding time to discharge.
The first scenario followed the EAG’s main analyses assuming a RR for clinical improvement of 1.04 in the mean efficacy scenario (1.00 in the low efficacy scenario and 1.10 in the high efficacy scenario) and a HR for time to discharge of 1.00 for all efficacy scenarios. In the second scenario, a time to discharge of 1.27 was used for the main efficacy scenario (1.10 in the low efficacy scenario and 1.46 in the high efficacy scenario) based on the RR for discharge or National Early Warning Score ≤ 2 for 24 hours as reported in ACTT-1. 25 As these values incorporated clinical improvement but were assumed to apply to time to discharge only, a RR of unity was assumed for clinical improvement in all three efficacy scenarios to reduce the possibility of double counting.
Another scenario analysis was undertaken for tixagevimab/cilgavimab using efficacy data provided by the company for people treated within 5 days of symptom onset. Within their response to the consultation on the draft guidance, the company states that ‘Evusheld is more clinically effective and cost-effective when used within 5 days from symptom onset’ and that ‘selecting 5 days as a treatment cut-off for Evusheld aligns with how clinicians would seek to use Evusheld in clinical practice’. The company provided an unpublished set of outcomes for this set of patients from TACKLE80 which were an RR of hospitalisation or death of 0.31 (95% CI 0.15 to 0.64) with a calculated mean value of 0.33, and RR of all-cause mortality at 28 days of 0.33 (95% CI 0.03 to 3.15) with a calculated mean value of 0.67. Following the logic detailed earlier in this section, the mortality RR for the low efficacy scenario was capped at unity.
The results presented provide the ICER, measured in terms of cost per QALY gained, for each intervention compared to SoC and the efficiency frontier, which contains all interventions that are not dominated or extendedly dominated. For the efficiency frontier, the willingness to pay (WTP) at which the preferred treatment changes, presented in terms of cost per QALY thresholds, is provided. A full incremental analysis is an appropriate method for comparing interventions when all treatments would be considered for use in patients and where there is confidence that the relative treatment effects are comparable in terms of key factors (such as the same SoC, the same vaccination levels, and the same SARS-CoV-2 variant). In this report there are considerable differences between studies in key factors which could invalidate incremental analyses. As such, the results from incremental analyses should be treated with considerable caution.
To allow a broader view of the cost-effectiveness, the EAG has provided the ICER for each treatment compared with SoC and used an NMB approach. Within this framework, the largest NMB is associated with the most cost-effective strategy at the stated cost-per-QALY threshold, and multiple strategies can be compared simultaneously, as the absolute difference in strategies in terms of cost, and monetarised health differences, can be easily determined. The formula for calculating NMB is the increase in QALYs associated with an intervention multiplied by a stated cost per QALY threshold minus the additional costs associated with the intervention compared with the costs associated with SoC. If NMB is positive the intervention is cost-effective compared with SoC at the selected threshold; if the NMB is negative, then the intervention is not cost-effective compared with SoC at the selected threshold. When multiple interventions are considered, the intervention with the greatest NMB would be interpreted as the most cost-effective intervention. The advantage of the NMB approach is twofold. First, if an intervention is not appropriate for treating a group of patients, then this NMB can be ignored without affecting the other values. Second, interventions can be compared using different scenarios specific to an intervention, so for example, the NMBs could be compared between one intervention at high efficacy and one intervention at low efficacy, were this desired. NMB values have been presented using a threshold of £20,000 per QALY gained and a threshold of £30,000 per QALY gained.
For the sensitivity analysis, only NMB values were presented in order that many results can be shown simultaneously. For the sensitivity analyses presented in this report, cost per QALY thresholds of £20,000 per QALY and £30,000 per QALY have been used.
One limitation associated with the omission of PSA is that value of information analyses could not be conducted to assess the monetary implications of recommending an intervention that was not the most cost-effective and to put a ceiling on the expenditure of research addressing knowledge gaps. This is an area for future research.
The use of severity modifiers
From 31 January 2022, NICE Appraisal committees would consider the severity of a condition, defined as the future health lost by people with a condition receiving standard care2 and that a greater weight can be applied to QALYs if a condition is deemed to be severe. The guidance from NICE is that if there is an absolute discounted QALY shortfall of ˂12 and that the proportional shortfall in discounted QALYs is ˂85% then no severity modifier should be applied in the decision problem, and that the ICER remains unchanged.
For patients admitted to hospital, the mean age was assumed to be 70.6 years and with 38.3% being female. Using these characteristics, the EAG calculated that the discounted QALYs associated with the general population would be approximately 8.68. Based on the results presented in Cost-effectiveness results, SoC is associated with estimated discounted QALYs of 4.61 for patients who require supplemental oxygen on admission and 5.79 for patients who do not require supplemental oxygen on admission. For those requiring supplemental oxygen, the absolute shortfall was 4.44 discounted QALYs and the proportional shortfall was 49%; these numbers are lower for those who do not require supplemental oxygen. As such, no severity modifier is applied for patients who are hospitalised due to COVID-19.
For patients at high risk of hospitalisation in the community, the mean age in the base case was assumed to be 55 years. The 38.3% proportion of females used for hospitalised patients was assumed to be generalisable to patients at high risk in the community. Using these characteristics, the EAG calculated that the discounted QALYs associated with the general population would be approximately 13.93. Based on the results presented in Cost-effectiveness results, the absolute shortfall in discounted QALYs for patients at high risk of hospitalisation was ˂1, and the proportionate shortfall in discounted QALYs was ˂4%. Given these values, no severity modifier is applied for patients who are at high risk of hospitalisation due to COVID-19.
Model validation
The EAG validated its model by altering parameters and assumptions such that it replicated the model reported in Rafia et al. 22 Similar results for remdesivir were achieved in terms of costs, QALYs and the ICER. During the stakeholder consultation period two implementation errors were identified. The larger related to the outpatient setting and was unfavourable to treatments; the second related to the implementation of clinical deterioration and had a small impact on the results. These errors have been corrected to produce the results in this report.
Chapter 4 Cost-effectiveness results
The cost-effectiveness results have been divided into three subsections. The first provides the results for hospitalised patients who require supplemental oxygen on admission, the second provides the results for hospitalised patients who do not require supplemental oxygen on admission and the third provides the results for patients at high risk of hospitalisation in the community. Each of the three subsections is further divided to provide the results from the mean efficacy, high efficacy and low efficacy scenarios due to considerable uncertainty in the observed efficacy within pivotal studies and changes since the study relating to: the evolving nature of SoC; the impact of vaccination; the impact of previous SARS-CoV-2 infection; and the predominant SARS-CoV-2 variant.
The EAG stresses that this report only uses publicly available list prices. The PASs for tocilizumab, baricitinib and sotrovimab are not included which means that the results presented are not accurate representations of the true ICERs for these drugs. Furthermore, three drugs do not have publicly available list prices: casirivimab/imdevimab, molnupiravir and tixagevimab/cilgavimab. Results incorporating PASs and confidential list prices are contained in a confidential appendix that is seen by the NICE Appraisal Committee.
The results need to be interpreted with caution and in the context of external information. For example, in September 2022, the WHO offered strong recommendations against the use of casirivimab/imdevimab in patients with COVID-19 and against the use of sotrovimab in patients with non-severe COVID-19. As such, the efficacy values associated with these two drugs are highly likely to be nearer the low efficacy values rather than the mean efficacy values, and there is considerable uncertainty in the efficacy of the remaining treatments.
Incremental analyses will be particularly uncertain but have been included for completeness. A NMB approach has been used to allow results to be compared when different assumptions are made for each intervention (e.g. in relation to efficacy) or where some interventions are omitted as they would not be appropriate for a particular patient. Pairwise ICERs for the mean, high and low efficacy scenarios have been presented for each intervention compared with SoC in the non-confidential base case for each of the three populations.
Results for hospitalised patients who need supplemental oxygen on admission
Mean efficacy results for patients requiring supplemental oxygen on admission to hospital
The results of the mean efficacy analysis for patients requiring supplemental oxygen on admission to hospital are shown in Table 13. All interventions were estimated to have a cost per QALY gained compared to SoC below £12,000.
Intervention | Discounted costs (£) | Discounted QALYs | Cost per QALY compared with SoC (£) | NMB compared with SoCa (£) | NMB compared with SoCb (£) | Cost per QALY incremental analyses (£) |
---|---|---|---|---|---|---|
SoC | 22,127 | 4.61 | – | – | – | – |
Tocilizumab | 25,551 | 5.12 | 6728 | 6755 | 11,844 | 6728 |
Remdesivir | 27,773 | 5.08 | 11,989 | 3773 | 8484 | Dominated |
Baricitinib | 30,223 | 5.46 | 9519 | 8915 | 17,421 | 13,676 |
Baricitinib/remdesivir | 30,515 | 5.32 | 11,744 | 5897 | 13,040 | Dominated |
High efficacy results for patients requiring supplemental oxygen on admission to hospital
The results of the high efficacy analysis for patients requiring supplemental oxygen on admission to hospital are shown in Table 14. All interventions were estimated to have a cost per QALY gained compared to SoC below £12,000. The costs associated with tocilizumab are lower than for other drugs due to the assumed higher rate of discharge of patients as the remaining interventions do not have data and assumed to have the same discharge rate as SoC.
Intervention | Discounted costs (£) | Discounted QALYs | Cost per QALY compared with SoC (£) | NMB compared with SoCa (£) | NMB compared with SoCb (£) | Cost per QALY incremental analyses (£) |
---|---|---|---|---|---|---|
SoC | 22,127 | 4.61 | – | – | – | – |
Tocilizumab | 23,452 | 5.41 | 1661 | 14,635 | 22,745 | 1661 |
Remdesivir | 33,100 | 5.57 | 11,433 | 8223 | 17,727 | Extendedly dominated (Ext Dom) |
Baricitinib | 34,364 | 5.82 | 10,116 | 11,957 | 24,141 | Ext Dom |
Baricitinib/remdesivir | 38,517 | 6.03 | 11,559 | 11,968 | 26,025 | 24,302 |
Low efficacy results for patients requiring supplemental oxygen on admission to hospital
The results of the low efficacy analysis for patients requiring supplemental oxygen on admission to hospital are shown in Table 15. Remdesivir and baricitinib/remdesivir were dominated by SoC due to providing no additional QALYs at an increased price. The ICER for baricitinib was below £9000, that for tocilizumab was below £29,000.
Intervention | Discounted costs (£) | Discounted QALYs | Cost per QALY compared with SoC (£) | NMB compared with SoCa (£) | NMB compared with SoCb (£) | Cost per QALY incremental analyses (£) |
---|---|---|---|---|---|---|
SoC | 22,127 | 4.61 | – | – | – | - |
Remdesivir | 24,077 | 4.61 | Dominated | −2001 | −2002 | Dominated |
Baricitinib/remdesivir | 24,339 | 4.61 | Dominated | −2102 | −2102 | Dominated |
Baricitinib | 26,099 | 5.08 | 8470 | 5513 | 10,203 | 8470 |
Tocilizumab | 28,009 | 4.81 | 28,806 | −1687 | 354 | Dominated |
Results for hospitalised patients who do not need supplemental oxygen on admission
Mean efficacy results for patients requiring no supplemental oxygen on admission to hospital
The results of the mean efficacy analysis for patients not requiring supplemental oxygen on admission to hospital are shown in Table 16. All interventions were estimated to have a cost per QALY gained compared to SoC below £12,000.
Intervention | Discounted costs (£) | Discounted QALYs | Cost per QALY compared with SoC (£) | NMB compared with SoCa (£) | NMB compared with SoCb (£) | Cost per QALY incremental analyses (£) |
---|---|---|---|---|---|---|
SoC | 13,316 | 5.79 | – | – | – | – |
Baricitinib | 16,073 | 6.29 | 5499 | 7271 | 12,284 | 5499 |
Remdesivir | 16,487 | 6.07 | 11,214 | 2485 | 5313 | Dominated |
Baricitinib/remdesivir | 17,509 | 6.21 | 9895 | 4282 | 8519 | Dominated |
High efficacy results for patients requiring no supplemental oxygen on admission to hospital
The results of the high efficacy analysis for patients not requiring supplemental oxygen on admission to hospital are shown in Table 17. All interventions were estimated to have a cost per QALY gained compared to SoC below £9000.
Intervention | Discounted costs (£) | Discounted QALYs | Cost per QALY compared with SoC (£) | NMB compared with SoCa (£) | NMB compared with SoCb (£) | Cost per QALY incremental analyses (£) |
---|---|---|---|---|---|---|
SoC | 13,316 | 5.79 | – | – | – | – |
Baricitinib | 17,534 | 6.49 | 6019 | 9799 | 16,808 | 6019 |
Remdesivir | 18,182 | 6.35 | 8648 | 6389 | 12,017 | Dominated |
Baricitinib/remdesivir | 20,308 | 6.60 | 8595 | 9278 | 17,413 | 24,628 |
Low efficacy results for patients requiring no supplemental oxygen on admission to hospital
The results of the low efficacy analysis for patients not requiring supplemental oxygen on admission to hospital are shown in Table 18. Remdesivir and baricitinib/remdesivir were estimated to be dominated by SoC due to producing no additional QALYs at an additional cost. Baricitinib had a cost per QALY below £6000.
Intervention | Discounted costs (£) | Discounted QALYs | Cost per QALY compared with SoC (£) | NMB compared with SoCa (£) | NMB compared with SoCb (£) | Cost per QALY incremental analyses (£) |
---|---|---|---|---|---|---|
SoC | 13,316 | 5.79 | – | – | – | – |
Baricitinib | 14,797 | 6.07 | 5259 | 4279 | 7466 | 5259 |
Remdesivir | 15,239 | 5.79 | Dominated | −1924 | −1924 | Dominated |
Baricitinib/remdesivir | 15,477 | 5.79 | Dominated | −2037 | −2037 | Dominated |
Results for patients at high risk of hospitalisation treated in the community
Mean efficacy results for patients at high risk of hospitalisation
The results of the mean efficacy analysis for patients at high risk of hospitalisation are shown in Table 19. Nirmatrelvir/ritonavir was estimated to have a cost per QALY compared to SOC of below £7000 with the remaining interventions having an ICER above £30,000.
Intervention | Discounted costs (£) | Discounted QALYs | Cost per QALY compared with SoC (£) | NMB compared with SoCa (£) | NMB compared with SoCb (£) | Cost per QALY incremental analyses (£) |
---|---|---|---|---|---|---|
SoC | 1053 | 13.41 | – | – | – | – |
Nirmatrelvir/ritonavir | 1805 | 13.53 | 6168 | 1687 | 2907 | 6168 |
Sotrovimab | 3580 | 13.48 | 34,999 | −1083 | −361 | Dominated |
Remdesivir | 4390 | 13.45 | 90,850 | −2602 | −2235 | Dominated |
High efficacy results for patients at high risk of hospitalisation
The results of the high efficacy analysis for patients at high risk of hospitalisation are shown in Table 20. Nirmatrelvir/ritonavir was estimated to have a cost per QALY compared to SOC of below £6000, sotrovimab was estimated to have a cost per QALY compared to SOC of below £19,000 and remdesivir was estimated to have a cost per QALY compared to SOC of below £25,000.
Intervention | Discounted costs (£) | Discounted QALYs | Cost per QALY compared with SoC (£) | NMB compared with SoCa (£) | NMB compared with SoCb (£) | Cost per QALY incremental analyses (£) |
---|---|---|---|---|---|---|
SoC | 1053 | 13.41 | – | – | – | – |
Nirmatrelvir/ritonavir | 1817 | 13.55 | 5420 | 2055 | 3464 | 5420 |
Sotrovimab | 3613 | 13.55 | 18,336 | 232 | 1628 | Dominated |
Remdesivir | 4421 | 13.55 | 24,431 | −611 | 768 | Dominated |
Low efficacy results for patients at high risk of hospitalisation
The results of the low efficacy analysis for patients at high risk of hospitalisation are shown in Table 21. Nirmatrelvir/ritonavir was estimated to have a cost per QALY compared to SOC of below £12,000 while remdesivir and sotrovimab both had ICERs of over £100,000 compared with SoC.
Intervention | Discounted costs (£) | Discounted QALYs | Cost per QALY compared with SoC (£) | NMB compared with SoCa (£) | NMB compared with SoCb (£) | Cost per QALY incremental analyses (£) |
---|---|---|---|---|---|---|
SoC | 1053 | 13.41 | – | – | – | – |
Nirmatrelvir/ritonavir | 1817 | 13.48 | 11,009 | 623 | 1317 | 11,009 |
Sotrovimab | 3686 | 13.44 | 116,505 | −1673 | −1447 | Dominated |
Remdesivir | 4651 | 13.42 | 373,256 | −3405 | −3309 | Dominated |
Sensitivity analysis results
The eight sets of sensitivity analyses described in Analyses undertaken were run. For reference, the NMBs of each intervention, using a WTP of £20,000 per QALY gained are shown in Figures 7–9 for patients who are hospitalised and require supplemental oxygen, patients who are hospitalised but do not require supplemental oxygen, and patients with COVID-19 in the community who are at high risk of hospitalisation respectively. To comply with regulations on the number of tables and figures in HTA monographs, corresponding figures when using a WTP of £30,000 are shown in Appendix 4 (see Figures 32–34, respectively). The patterns of NMB are the same at both the £20,000 and £30,000 WTP values with few changes in the sign associated with the NMB. The sign of the NMB changed for:
-
Tocilizumab at low efficacy in patients admitted to hospital who require supplemental oxygen which had a negative NMB at a WTP of £20,000 but a positive NMB at a WTP of £30,000.
-
Remdesivir at high efficacy in patients at high risk in the community which had a negative NMB at a WTP of £20,000 but a positive NMB at a WTP of £30,000.
FIGURE 7.
Base case net monetary benefits for patients admitted to hospital who require supplemental oxygen assuming a threshold of £20,000 per QALY gained.

FIGURE 8.
Base case net monetary benefits for patients admitted to hospital who do not require supplemental oxygen assuming a threshold of £20,000 per QALY gained.
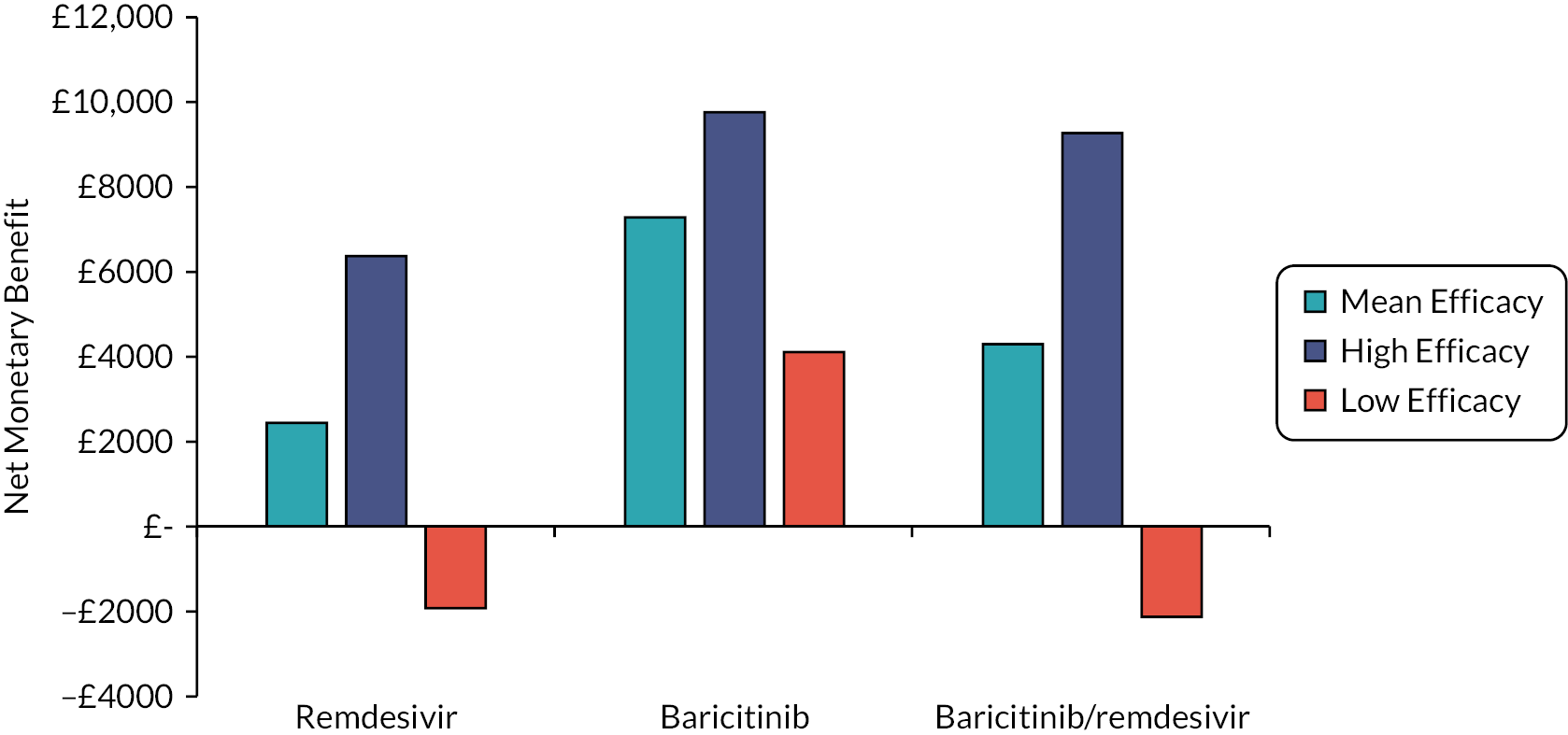
FIGURE 9.
Base case net monetary benefits for patients with COVID-19 in the community and high risk of hospitalisation assuming a threshold of £20,000 per QALY gained.

Amending the duration of long COVID
The NMB results when the duration of long COVID is doubled (to 227.6 weeks) and halved (to 56.8 weeks) are shown in Figures 10–12 for people admitted to hospital requiring supplemental oxygen, those admitted to hospital with no need for supplemental oxygen and those treated in the community at high risk of hospitalisation, respectively, using a WTP of £20,000 per QALY. Corresponding data using a WTP of £30,000 per QALY are shown in Appendix 4 (see Figures 35–37).
FIGURE 10.
The NMB results for patients admitted to hospital who require supplemental oxygen when the duration of long COVID is halved and doubled. Assuming a WTP of £20,000.

FIGURE 11.
The NMB results for patients admitted to hospital who do not require supplemental oxygen when the duration of long COVID is halved and doubled. Assuming a WTP of £20,000.
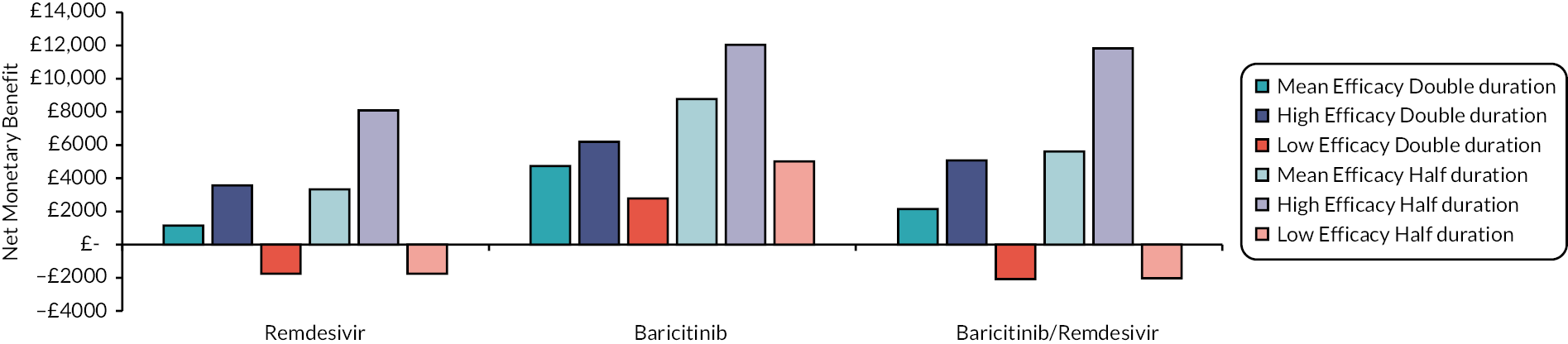
FIGURE 12.
The NMB results for patients in the community with COVID-19 who are at high risk of hospitalisation when the duration of long COVID is halved and doubled. Assuming a WTP of £20,000.
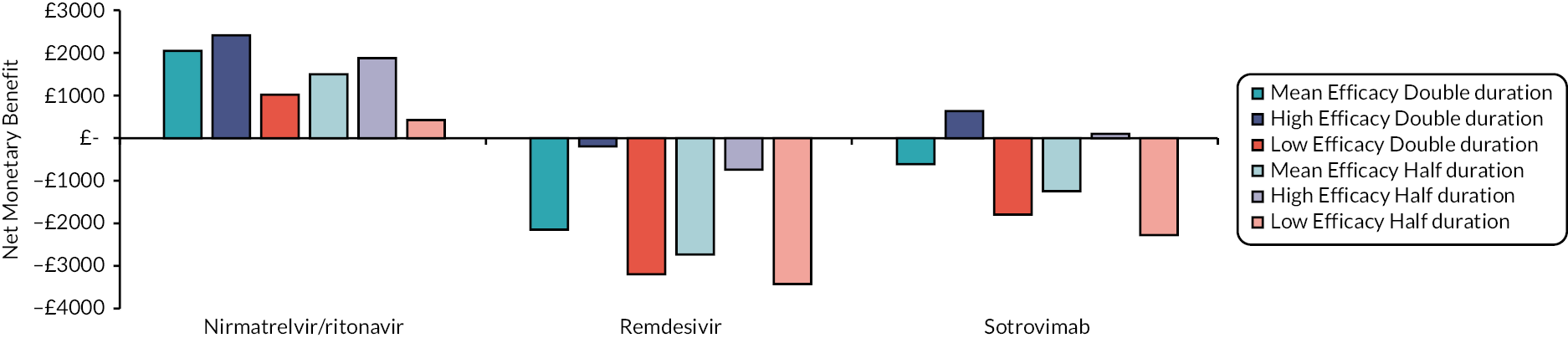
For patients in hospital, longer durations of COVID reduced NMBs, as there were more survivors with long COVID when treatment was beneficial. In contrast, NMBs were increased in patients at high risk in the community as treatments stopped patients being hospitalised and therefore reduced the numbers assumed to have long COVID. There were one instances where the NMB had a different sign compared with the base case when the duration of COVID was changed which was for sotrovimab in the mean efficacy scenario when the duration of long COVID was doubled. There was a moderate impact on the ICERs generated for hospitalised patients in the mean scenario typically changing between +/– £2000 per QALY. The impact was greater for remdesivir when used in the community although in this instance the initial ICERs were large.
Amending the hospital admission percentage for people with COVID-19 in the community at high risk of hospitalisation treated with SoC
The NMB results when the hospitalisation admission percentage for people with COVID-19 in the community at high risk of hospitalisation treated with SoC was changed from 2.82% to 1%, 5%, 10% and 20% are shown in Figure 13 assuming a WTP of £20,000 per QALY and Appendix 4 (see Figure 38) assuming a WTP of £30,000 per QALY. The proportion of patients with COVID-19 at high risk of being hospitalised being admitted to hospital makes a large difference to the NMB with values increasing as the admission proportion increases. All interventions had a positive NMB when the proportion of patients hospitalised was increased to 10.00% and the mean efficacy scenario was used independent of the WTP assumed. Although remdesivir and sotrovimab had negative NMBs when the low efficacy scenario was used even when the admission percentage was increased to 20%. The ICERs versus SoC changed considerably based on the proportion of high-risk patients hospitalised. The ICERs when assuming 1%, 10% and 20% and the mean efficacy were: nirmatrelvir/ritonavir (£24,647, dominant and dominant), remdesivir (£280,819, £16,170 and £1512) and sotrovimab (£111,318, £4870 and dominant).
FIGURE 13.
The NMB results for patients in the community with COVID-19 who are at high risk of hospitalisation when the hospital admission percentage was changed. Assuming a WTP of £20,000.

Amending the age of people with COVID-19 in the community at high risk of hospitalisation treated with SoC
The NMB results when the age assumed for people with COVID-19 in the community at high risk of hospitalisation treated with SoC was changed from 55 years to 50 and 60 years are shown in Figure 14, assuming a WTP of £20,000 per QALY, Appendix 4 (see Figure 39) shows results using a WTP of £30,000 per QALY. Where the RR of day 28 mortality is lower than one the NMBs decrease as the age of the patients increases because less QALYs are gained when a death is prevented. However, the EAG notes that there is no explicit link between risks of poor outcomes and age, and it is likely that all other things being equal, older patients are at a higher risk and that the results could be misleading. However, there was only a moderate impact on the ICERs generated for hospitalised patients in the mean scenario typically changing between +/– £2000 per QALY.
FIGURE 14.
The NMB results for patients in the community with COVID-19 who are at high risk of hospitalisation when the age was changed from 55 years to 50 and 60 years. Assuming a WTP of £20,000 per QALY.

Using a HR of unity for all interventions in relation to time to hospital discharge and time to clinical improvement
The NMB results when all interventions and SoC had the same impact on time to hospital discharge and time to clinical improvement are shown in Figure 15 for patients requiring supplemental oxygen and in Figure 16 for patients not requiring supplemental oxygen, assuming a WTP of £20,000 per QALY. Figures 40 and 41 in Appendix 4 use a WTP of £30,000 per QALY. This sensitivity analysis did not change the patterns or the sign of the NMBs. These parameters were not large drivers of the ICER. The only noticeable change in the ICER was that for tocilizumab which increased by about £3000 compared with SoC.
FIGURE 15.
The NMB when a HR of unity was used for all interventions in relation to time to hospital discharge and time to clinical improvement for patients requiring supplemental oxygen. Assuming a WTP of £20,000 per QALY.

FIGURE 16.
The NMB when a HR of unity was used for all interventions in relation to time to hospital discharge and time to clinical improvement for patients not requiring supplemental oxygen. Assuming a WTP of £20,000 per QALY.
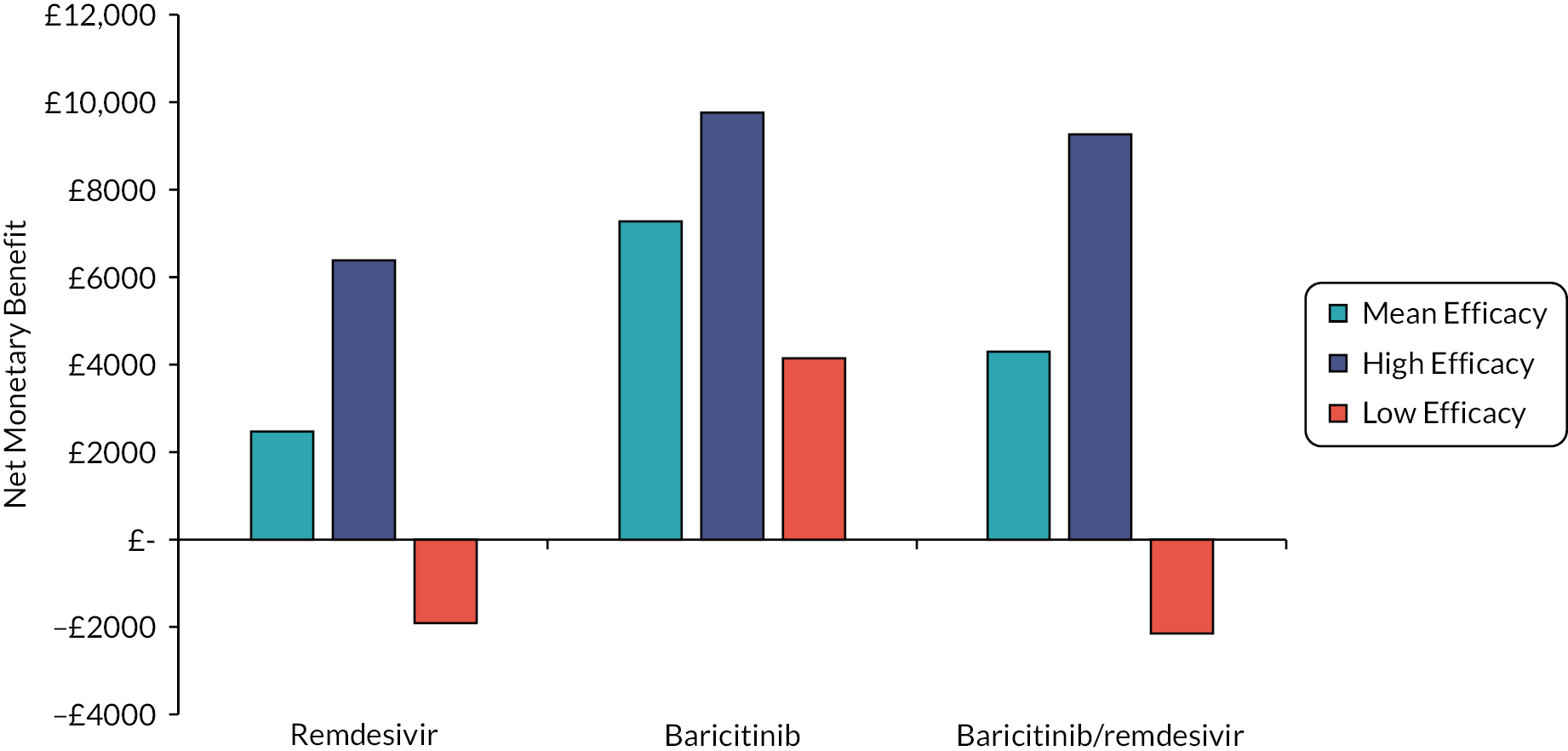
Changing the baseline distribution of supplemental oxygen requirements for people with COVID-19 in the community upon hospitalisation
The NMB results when the interventions were assumed to have a less severe distribution following treatment in the community are shown in Figure 17 assuming a WTP of £20,000 per QALY. Figure 42 in Appendix 4 provides NMBs assuming a WTP of £30,000 per QALY. This sensitivity analysis had a minor impact on the ICERs and did not change whether any NMBs were positive and negative.
FIGURE 17.
The NMB results when treatment in the community for high-risk patients was associated with less supplemental oxygen on admission to hospital. Assuming a WTP of £20,000 per QALY.

Applying a utility decrement of 0.02 per day for people in the community receiving i.v. treatment
The NMB results when a disutility of 0.02 per day for those receiving i.v. treatment in the community are shown in Figure 18 assuming a WTP of £20,000 per QALY and in Appendix 4 (see Figure 43) assuming a WTP of £30,000. This sensitivity analysis made no discernible change to the NMBs or ICERs.
FIGURE 18.
The NMB results when a disutility of 0.02 per day is assumed for patients receiving intravenous (i.v.) treatment in the community. Assuming a WTP of £20,000 per QALY.

Changing the SMR for people with long COVID
The NMB results when the SMR associated with long COVID is changed from 7.7 to 5.0 and 10.0 are shown in Figures 19–21 assuming a WTP of £20,000 per QALY. Figures 44–46 in Appendix 4 provide these data assuming a WTP of £30,000 per QALY. The change in the SMR for people with long COVID had little impact on the ICERs for hospital treatments. There was a greater impact for treatments in the community, but this did not change whether a NMBs was positive or negative.
FIGURE 19.
The NMB results for patients admitted to hospital who require supplemental oxygen when the SMR associated with long COVID is changed. Assuming a WTP of £20,000 per QALY.
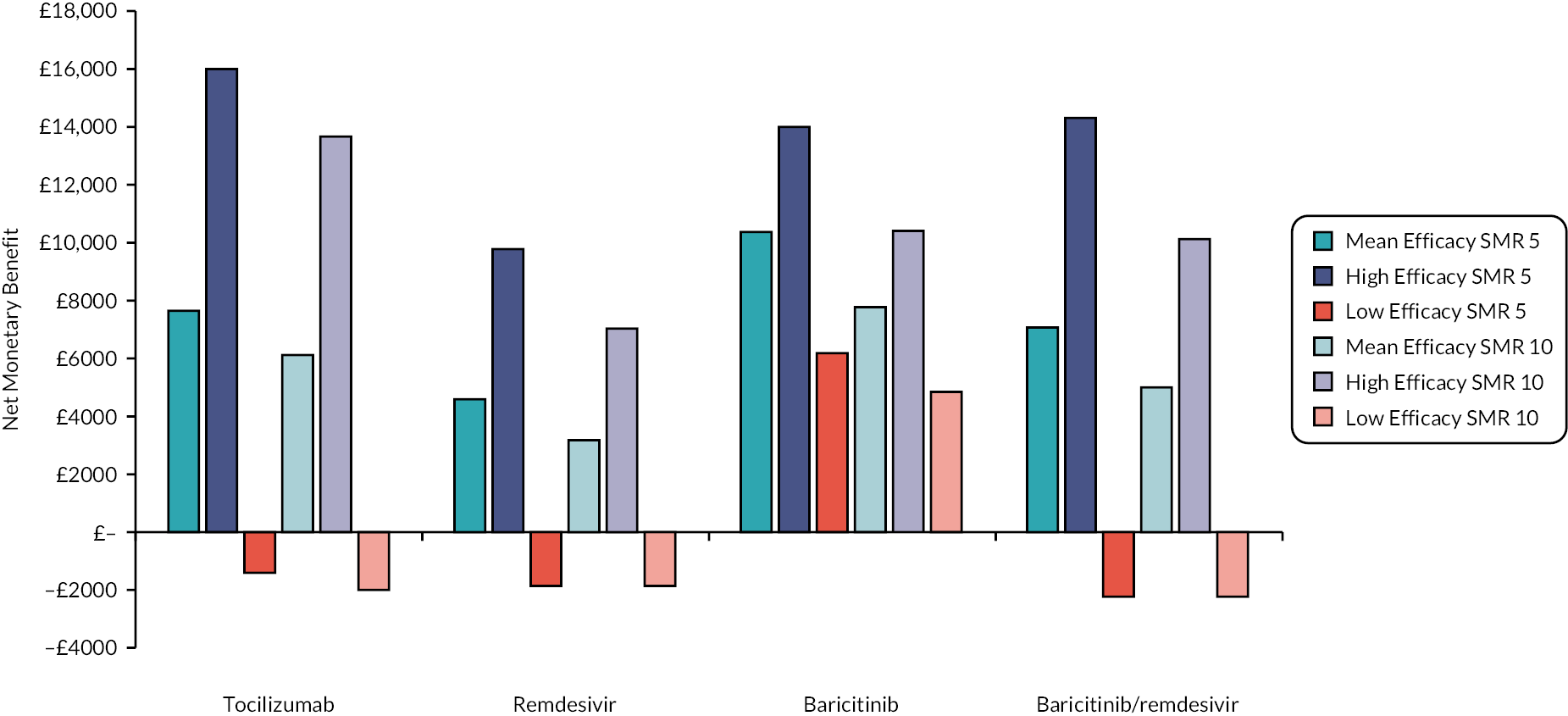
FIGURE 20.
The NMB results for patients admitted to hospital who do not require supplemental oxygen when the SMR associated with long COVID is changed. Assuming a WTP of £20,000 per QALY.

FIGURE 21.
The NMB results for high-risk patients in the community when the SMR associated with long COVID is changed. Assuming a WTP of £20,000 per QALY.

Scenario analyses results
The NMB results when HR of time to death estimated from meta-analysis include data from Solidarity with and without RR of time to discharge from ACTT-1 are shown in Figure 20 for those who require supplemental oxygen and in Figure 21 for those that do not require supplemental oxygen assuming a WTP of £20,000 per QALY. Figures 47 and 48 in Appendix 4 provide these data assuming a WTP of £30,000 per QALY. At the mean and high efficacy scenario, when data from Solidarity were used remdesivir had a positive NMB regardless of the WTP and oxygen status assumed. In the low efficacy scenario, remdesivir had a positive NMB regardless of the WTP and oxygen status assumed if Solidarity data and the HR for time to discharge from ACTT-1 were used. For patients requiring supplemental oxygen the ICER was £25,903 in the low efficacy scenario when only Solidarity data were used; the corresponding ICER was £34,550 for those not requiring supplementary oxygen.
The use of 5-day outcome measures for tixagevimab/cilgavimab increased total discounted QALYs from: 13.42 to 13.43 in the low efficacy scenario; from 13.43 to 13.47 in the mean efficacy scenario; and from 13.51 to 13.55 in the high efficacy scenario.
Summary of cost-effectiveness analyses
The results provided in this report provide an indication of plausible ICERs for each intervention although the results for casirivimab/imdevimab, molnupiravir and tixagevimab/cilgavimab could not be presented due to confidential list prices, and the PAS prices for baricitinib, sotrovimab and tocilizumab could not be incorporated in this report. There were two key drivers of the ICERs: these were the efficacy of intervention and the proportion of high-risk patients in the community that needed hospitalisation. Other variables impacted on the ICERs to much lesser degrees.
The ICERs were more favourable to treatments when efficacy was assumed to be high, with ICERs routinely below £12,000 in interventions used in hospital, however, ICERs were much higher where efficacy was assumed to be low, with some interventions dominated due to providing no additional QALYs. In the community, a similar pattern was seen, with all interventions having ICERs below £25,000 assuming high efficacy, but with remdesivir and sotrovimab having ICERs above £100,000 at low efficacy. The EAG stresses the considerable uncertainty in the efficacy of each intervention due to changes in factors such as the SARS-CoV-2 variant, changed SoC and vaccination status. This is demonstrated by sotrovimab having favourable median and mean efficacies in preventing hospitalisation, but this drug is not authorised in the USA, as it is unlikely to be effective against the Omicron BA.2 subvariant. Further the WHO has made strong recommendations against the use of sotrovimab. Given potential further changes in the variant, the results presented in this report, and within the confidential appendix, should be treated with caution.
The EAG provided the committee with three efficacy scenarios for each treatment: mean efficacy, high efficacy and low efficacy. The mean efficacy would be the efficacy expected if the conditions were exactly the same as during the studies contained in COVID-NMA12 and metaEvidence. 18 However, the high and low efficacy scenarios were, for reasons of transparency and not knowing the ‘true’ efficacy, set to the lower and upper 95% limits of the CIs of reported efficacy, respectively. The EAG has acknowledged a limitation that the CI is influenced by the number of observed events and the sample size, such that two identical treatments could have markedly different confident intervals purely due to the size of the pivotal study. The EAG does not have a preferred base case as the impact of changing variants, vaccination status, prior infection and SOC are likely to affect the efficacy observed in RCTs.
For interventions used in the community, the proportion of high-risk patients in the community that needed hospitalisation greatly changed the ICER with large differences between those generated using a 1% proportion and those generated using 20%. These were: nirmatrelvir/ritonavir (£24,647 and dominant), remdesivir (£280,819 and £1512) and sotrovimab (£111,318 and dominant). The estimate reported by Patel et al.,13 2.82%, was assumed to be the base case value in this report but the EAG acknowledges that there will be identifiable subgroups with higher risks than this and has provided analyses at different values for the information of the Appraisal Committee. It is possible that some interventions that are not believed to be cost-effective by the Appraisal Committee using a value of 2.82% would be deemed cost-effective at higher hospitalisation rates.
Chapter 5 Discussion and conclusions
Summary of clinical-effectiveness data
For time reasons, the EAG used data from two living systematic reviews and had to assume that the reported efficacy of treatments was generalisable to other settings. This assumption may not be correct due to: the evolving nature of SoC; the impact of vaccination; the impact of previous SARS-CoV-2 infection; and the predominant SARS-CoV-2 variant. In addition, patient age, ethnicity, sex and immune system competence may be treatment effect modifiers. This point is proven in the case of sotrovimab which had beneficial mean efficacy values but where guidance from the US Food and Drug Administration that ‘sotrovimab is not authorized in any US state or territory at this time’ (5 April 2022) as it is unlikely to be effective against the Omicron BA.2 subvariant. 20
All treatments were associated with a midpoint beneficial effect on preventing mortality, except for remdesivir for patients at high risk in the community where there were no deaths in either arm. Noting the caveats associated with assuming transportability of treatment effects and the relatively wide CIs associated with preventing mortality, the EAG did not feel confident that it could robustly identify a treatment that was more efficacious than others or, potentially, SoC.
The interventions should be reviewed for activity against current and future variants. If it is shown that these confer more or less protection than against the predominant variant in the key clinical studies, then decision-makers may choose to select the ‘high’ or ‘low’ efficacy results to guide estimates of cost-effectiveness.
Summary of cost-effectiveness analyses
For patients who have been hospitalised due to COVID-19, all treatments had scenarios where the ICER was below £20,000 compared with SoC, however, in the low efficacy scenario only baricitinib and tocilizumab had ICERs under £30,000 compared with SoC. For patients with COVID-19 in the community at high risk of hospitalisation, nirmatrelvir/ritonavir was estimated to have an ICER below £8000 compared with SoC, with sotrovimab and remdesivir having ICERs above £30,000. The EAG stresses that, for all interventions in all settings, if the drug does not work well against current or future variants the ICER could be markedly higher than that estimated in the low efficacy scenario.
The analyses in this report are more favourable to remdesivir treatment in hospital than previous estimates reported by Rafia et al. 22 The primary reasons for this are differing assumptions in the models. In Rafia et al. 22 remdesivir was associated with an odds ratio for clinical improvement that indicated that remdesivir was harmful, compared with SoC. to a patient who did not die in hospital and the proportion of patients in ordinal scale 7 receiving SoC was large (22% at day 14). In our analyses, remdesivir is now associated with improved outcomes for patients who do not die in hospital but also the proportion of patients in ordinal scale 7 who receive SoC was significantly reduced (9% at day 14). These changes result in a considerable saving in hospital costs, which results in a lower ICER in our work.
The analyses did not look at the logistical aspects of providing treatment. For patients in hospital this is unlikely to be a significant issue, however, it could be for patients in the community. Local decision-makers would need to ascertain whether i.v. treatment for patients with COVID-19, if that were the patient’s preference, is possible. The analysis did also not consider the impact of patient preference of route of administration on utility.
Strengths of the economic analysis include
-
The use of effectiveness data from living systematic reviews.
-
An attempt by the EAG to align the results of SoC produced by the model with data observed in mid-2022.
-
Uncertainty in the model inputs and assumptions has been explored in sensitivity analyses.
-
The modelling attempts to capture movement between the 8-point ordinal scale to consider the costs and consequences of patient improvement and patient decline.
-
The modelling explicitly attempts to take the impact of the longer-term implications of COVID-19 into consideration.
-
The development of the model allows for a relatively quick evaluation of the treatments should more contemporary data become available.
Limitations of the analysis include
-
The characteristics of the decision problem may have changed considerably since the pivotal trials for each intervention was conducted. Such changes include the emergence of new SARS-CoV-2 variants, the introduction of a vaccination programme, proportion of people with a history of prior SARS-CoV-2 infection and the widespread use of corticosteroids in SoC. The EAG assumed that none of these were treatment effect modifiers and that the treatment effects were generalisable which is likely to be incorrect for a proportion of interventions.
-
No recent studies were identified using the Omicron BA5 most prevalent in England in the Summer of 2022.
-
No head-to-head studies of interventions were identified that could be used in the modelling and the uncertainty regarding the most efficacious treatment is large.
-
List prices are used for all interventions; results including PASs could not be provided in a publicly available document.
-
For some interventions list prices were not publicly available. As such, no ICERs have been presented for these drugs.
-
Uncertainty remains in the underlying rates of hospitalisation in patients with COVID-19 at high risk of hospitalisation under SoC.
-
Uncertainty remains in the underlying rates of death in patients hospitalised due to COVID-19 who receive SoC.
-
All deaths associated with people at high risk in the community are assumed to occur in hospital.
-
SoC only was assumed to be provided to patients in hospital if they had been treated with an intervention in the community as the residual effects of some treatments used in the hospital were larger than treatments used in hospital.
-
Treatments used in hospital were not assumed to affect the proportion of discharged people with long COVID and treatments used in the community were not assumed to affect the proportion of people not admitted to hospital with long COVID.
-
All patients were assumed to be discharged from hospital at day 70, which could favour the more efficacious treatments in reducing hospital costs.
-
No prior beliefs were incorporated relating to the clinical efficacy of the interventions. It may be clinically implausible that treatments that have a statistically significant beneficial HR relating to hospitalisation or death would be associated with increased RR of death at 28 days.
-
The model did not consider secondary infections, which is likely to be unfavourable to the interventions.
-
The model did not consider reinfections. It is unknown if this is favourable or unfavourable to the interventions.
-
The model did not consider enablement benefits such as maintaining the capacity for operations or in avoiding delays in patients’ treatment that could arise due to either a reduced number of patients in hospital with COVID-19, or reduced staff absence due to COVID-19.
-
No value of information analysis was conducted. This would allow funders to estimate the relative benefits of investing in future research.
-
No analysis was conducted on whether it is logistically possible to treat patients in the community with COVID-19 and a high risk of hospitalisation with i.v. drugs.
Areas of future research
There is considerable uncertainty related to many aspects of this evaluation which hinders forming an accurate estimate of the ICER. A key uncertainty is the clinical effectiveness of interventions in conditions that do not replicate those in the pivotal studies. Contemporary research assessing the relative clinical effectiveness of interventions (and SoC) within head-to-head studies at current levels of vaccination, against the current predominant SARS-CoV-2 variant would be beneficial if the results could be obtained in a timely manner. Further data related to the probability of hospital admission and death for patients at high risk in the community would also improve the precision of the estimated ICERs as would ascertaining the average age of this population. If possible, analysing efficacy data by previously specified risk groups, such as age band, and underlying risk category, may allow more granular results to be obtained. The impacts of long COVID in terms of morbidity and mortality are currently uncertain and further research is required in this area. Value of information analyses could be undertaken to efficiently direct future research although it is clear that the efficacy of the interventions will be a key driver of any cost-effectiveness results.
Given current knowledge the EAG is happy that the results produced using relatively simplistic techniques supported with sensitivity analyses are informative to decision-makers. If data become available that show that the sum of the consequences for a cohort of homogenous people is not equal to the sum from a same-sized cohort of heterogeneous people then more complex modelling techniques, such as individual patient models may be required. More complex modelling could explore the benefits associated with the possibility of secondary infection and reinfection, and with wider aspects such as enablement benefit.
The use of patient and public involvement
There was no patient and public involvement in producing this report. This was not considered possible within the timescales of the project. However, the EAG is aware that at the NICE Technology Appraisal Committee that will discuss this topic, there will be patient and public involvement and representation, and this may result in the EAG changing model parameters and generating revised results.
Equality, diversity and inclusion
As this report is secondary research, no patient participation was involved and the EAG did not need to consider the equality, diversity and inclusion of participants. The primary research team was part of the ScHARR Technology Assessment Group contracted by the Department of Health, and this team is a diverse group representing a wide range of protected characteristics, consisting of seniority, ages, ethnicity and religious beliefs, and including both males and females. The clinical team represents experts within their field who have successfully worked with the ScHARR Technology Assessment Group on previous projects. The lead author is not the most senior member of the team.
Acknowledgements
Contributions of authors
Andrew Metry (https://orcid.org/0000-0001-7412-6093) is a Research Associate at ScHARR, University of Sheffield. He participated in all aspects of the project.
Abdullah Pandor (https://orcid.org/0000-0003-2552-5260) is a Senior Research Fellow at ScHARR, University of Sheffield. He extracted, reviewed and summarised the studies related to the clinical evidence used in the model.
Shijie Ren (https://orcid.org/0000-0003-3568-7124) is a Senior Research Fellow at ScHARR, University of Sheffield. She extracted and reviewed the evidence to populate the model.
Andrea Shippam (https://orcid.org/0000-0003-3866-0939) is a Programme Administrator at ScHARR, University of Sheffield. She extracted and reviewed the evidence to populate the model.
Mark Clowes (https://orcid.org/0000-0002-5582-9946) is an Information Specialist at ScHARR, University of Sheffield. He provided guidance on the preliminary searches undertaken by the team.
Paul Dark (https://orcid.org/0000-0003-3309-0164) is a Professor of Critical Care Medicine at the University of Manchester. He provided clinical advice throughout the project.
Ronan McMullan (https://orcid.org/0000-0001-6760-6072) is a Professor of Medical Microbiology at Queen’s University, Belfast. He provided clinical advice throughout the project.
Matt Stevenson (https://orcid.org/0000-0002-3099-9877) is a Professor of Health Technology Assessment at ScHARR, University of Sheffield. He led the project and advised on all aspects of the research.
All authors commented on the final monograph.
Data-sharing statement
All data requests should be submitted to the corresponding author for consideration. Access to anonymised data may be granted following review.
Ethics statement
No ethical approval was needed as all included data were from secondary published sources.
Disclaimers
This report presents independent research funded by the National Institute for Health and Care Research (NIHR). The views and opinions expressed by authors in this publication are those of the authors and do not necessarily reflect those of the NHS, the NIHR, the HTA programme or the Department of Health and Social Care. If there are verbatim quotations included in this publication the views and opinions expressed by the interviewees are those of the interviewees and do not necessarily reflect those of the authors, those of the NHS, the NIHR, the HTA programme or the Department of Health and Social Care.
References
Appendix 1 Summary of clinical studies used to inform the economic model
Author, year | Design | Population | Severity | Sample size (n) | Intervention | Comparator | Follow-up | Funding | Overall risk of bias |
---|---|---|---|---|---|---|---|---|---|
Baricitinib | |||||||||
Marconi et al. 202181 (status: published) COV-BARRIER (NCT04421027) |
RCT, single blind | Patients with confirmed COVID-19 admitted to 101 centres in Argentina, Brazil, Germany, India, Italy, Japan, Mexico, Russia, South Korea, Spain, UK and the USA (including Puerto Rico) | Mild to severe Mean age: NR but includes adults aged ≥ 18 years |
1525 | Baricitinib, 4 mg/day (n = 764) (delivered orally) |
Placebo (n = 761) | 60 days | Private | Some concerns |
Horby et al. 202282 (status: published) RECOVERY (NCT04381936) |
RCT, unblinded | Patients with suspected or confirmed COVID-19 admitted to 159 centres in the UK | Mild to critical Mean age: NR but includes adults aged ≥ 2 years |
8156 | Baricitinib, 4 mg/day (n = 4148) (delivered orally) |
Standard care (n = 4008) | 28 days | Public/non-profit | Some concerns |
Ely et al. 202283 (status: published) COV-BARRIER (NCT04421027) |
RCT, double blind | Patients with confirmed COVID-19 admitted to 18 centres in Argentina, Brazil, Mexico and the USA | Critical Mean age: NR but includes adults aged ≥ 18 years |
101 | Baricitinib, 4 mg/day (n = 51) (delivered by nasogastric tube or orally) |
Placebo (n = 50) | 60 days | Private | Low RoB |
Baricitinib/remdesivir | |||||||||
Kalil et al. 202084 (status: published) ACTT-2 (NCT04401579) |
RCT, double blind | Patients with confirmed COVID-19 admitted to 67 centres in Denmark, Japan, Mexico, Singapore, South Korea, Spain, UK and the USA | Mild to critical Mean age: NR but includes adults aged ≥ 18 years |
1033 | Baricitinib, 4 mg/day plus remdesivir, 100 mg/daya (n = 515) (baricitinib delivered by nasogastric tube or orally; remdesivir delivered intravenously) | Placebo plus remdesivir, 100 mg/daya (n = 518) (remdesivir delivered intravenously) | 29 days | Public/non-profit | Low RoB |
Casirivimab/imdevimab | |||||||||
Horby et al. 202285 (status: published) RECOVERY-REGEN (NCT04381936) |
RCT, unblinded | Hospitalised patients with suspected or confirmed COVID-19 at 127 centres in the UK | Mild to critical Mean age: NR but includes patients ≥ 12 years |
9785 | REGN-COV2, 8 g (n = 4839) (casirivimab, 4 g and imdevimab 4 g delivered intravenously) |
Standard care (n = 4946) | 28 days | Mixed (Public/private) | Some concerns |
Somersan-Karakaya et al., 202286 (status: published) (NCT04426695) |
RCT, double blind | Hospitalised patients with confirmed COVID-19 at 103 centres across USA, Brazil, Chile, Mexico, Moldova and Romania | Mild to moderate Mean age: NR but includes adults aged ≥ 18 years |
1364 (multiarm trial) |
REGN-COV2, 2.4 g (n = 457) (casirivimab, 1.2 g and imdevimab 1.2 g delivered intravenously) REGN-COV2, 8 g (n = 455) (casirivimab, 4 g and imdevimab 4 g delivered intravenously) |
Placebo (n = 452) | 56 days | Mixed (Public/private) | Some concerns |
Portal-Celhay et al. 202287 (status: published) | RCT, double blind | Outpatients with confirmed COVID-19 (asymptomatic-mild) treated at 47 centres in the USA | Mild outpatients Mean age: NR but includes adults aged ≥ 18 years |
413 (multiarm trial) |
REGN-COV2, 2.4 g (n = 166) (casirivimab, 1.2 g and imdevimab 1.2 g delivered intravenously) REGN-COV2, 1.2 g (n = 165) (casirivimab, 0.6 g and imdevimab 0.6 g delivered intravenously) |
Placebo (n = 82) | 169 days | Private | Low RoB |
O’Brien et al. 202288 (status: published) (NCT04452318) |
RCT, double blind | Outpatients with confirmed COVID-19 (asymptomatic) treated at 112 centres in Moldova, Romania and the USA | Mild outpatients Mean age: NR but includes adults aged ≥ 18 years and adolescents aged ≥ 12 to < 18 years |
314 | REGN-COV2, 1200 mg (n = 156) (delivered subcutaneously once-off) |
Placebo (n = 158) | 226 days | Mixed (Public/private) | Some concerns |
Weinreich et al. 202189 (status: published) (NCT04425629) |
RCT, double blind | Outpatients with COVID-19 (mild) treated at 82 centres in Mexico and the USA | Mild outpatients Mean age: NR but includes adults aged ≥ 18 years |
1678 (Amended phase 3 portion only of trial) |
REGN-COV2, 1200 mg (n = 838) (delivered intravenously once-off) |
Placebo (n = 840) | 28 days | Mixed (Public/private) | Some concerns |
3029 (original and amended phase 3 portion of trial) |
REGN-COV2, 2400 mg (n = 1529) (delivered intravenously once-off) |
Placebo (n = 1500) | |||||||
Molnupiravir | |||||||||
Butler et al. 202290 (status: preprint) PANORAMIC (ISRCTN 30448031) |
RCT, unblinded | Outpatients with confirmed COVID-19 (mild-ambulatory) treated by multiple centres in the UK | Mild outpatients Mean age: NR but includes adults aged ≥ 50 years or ≥ 18 years with comorbidities |
25,783 | Molnupiravir, 1600 mg/day (n = 12,821) (delivered orally) |
Standard care (n = 12,962) | 28 days | Public/non-profit | High RoB |
Caraco et al. 202191 (status: published) MOVe-OUT (NCT04575597) |
RCT, double blind | Outpatients with confirmed COVID-19 (asymptomatic, mild) treated by 82 centres in 14 countries | Mild outpatients Mean age: NR (no further details provided) |
302 (4 arm trial) | Molnupiravir, 1600 mg/day (n = 76) (delivery method NR) | Placebo (n = 74) | 210 days | Private | Low RoB |
Fischer et al. 202192 (status: published) (NCT04405570) |
RCT, double blind | Outpatients with confirmed COVID-19 (mild) treated by 10 centres in the USA | Mild outpatients Mean age: NR but includes adults aged ≥ 18 years |
202 (4 arm trial) |
Molnupiravir, 1600 mg/day (n = 55) (delivered orally) |
Placebo (n = 62) | 28 days | Mixed (Public/ Private) |
High RoB |
Jayk Bernal et al. 202193 (status: published) | RCT, double blind | Outpatients with confirmed COVID-19 (mild-moderate) treated by 107 sites in 20 countries | Mild-moderate outpatients Mean age: NR (no further details provided) |
1433 | Molnupiravir, 1600 mg/day (n = 716) (delivered orally) |
Placebo (n = 717) | 28 days | Private | Low RoB |
Khoo et al. 202294 (status: preprint) AGILE CST-2 (NCT04746183) |
RCT, double blind | Outpatients with confirmed COVID-19 (mild) treated by 5 centres in the UK | Mild outpatients Mean age: NR but includes adults aged ≥ 18 years |
180 | Molnupiravir, 1600 mg/day (n = 90) (delivered orally) |
Placebo (n = 90) | 28 days | Mixed (Public/private) | Some concerns |
Tippabhotla et al. 202295 (status: preprint) (CTRI/2021/07/034588) |
RCT, unblinded | Outpatients with confirmed COVID-19 (mild) treated at 16 centres in India | Mild outpatients Mean age: NR but includes adults aged ≥ 18 years and ≤ 60 years |
1220 | Molnupiravir, 1600 mg/day (n = 610) (delivered orally) |
Standard care (n = 610) | 28 days | Private | Some concerns |
Zou et al. 202296 (status: published) (ChiCTR 2200056817) |
RCT, blinding NR | Patients with confirmed COVID-19 (mild) isolated and treated by a single centre in China | Mild outpatients Mean age: NR but includes adults aged ≥ 18 years and ≤ 80 years |
116 | Molnupiravir, 1600 mg/day (n = 80) (delivered orally) |
Standard care (n = 36) | 21 days | Mixed (Public/private) | Some concerns |
Nirmatrelvir/ritonavir | |||||||||
Hammond et al. 202297 (status: published) EPIC-HR (NCT04960202) |
RCT, double blind | Outpatients with confirmed COVID-19 (mild) treated by 343 centres in 21 countries | Mild outpatients Mean age: NR but includes adults aged ≥ 18 years |
2246 | Nirmatrelvir, 600 mg/day plus ritonavir, 200 mg/day (n = 1120) (delivered orally) | Placebo (n = 1126) | 34 days | Private | Some concerns |
Remdesivir | |||||||||
Ader et al. 202298 (status: published) DisCoVeRy (NCT04315948) |
RCT, unblinded | Patients with confirmed COVID-19 admitted to 48 centres in France, Belgium, Portugal, Austria and Luxembourg | Mild to critical Mean age: NR but includes adults aged ≥ 18 years |
857 | Remdesivir 100 mg/daya (n = 429) (delivered intravenously) |
Standard care (n = 428) | 90 days | Public/non-profit | Some concerns |
Biegel et al. 202025 (status: published) (NCT04280705) |
RCT, double blind | Patients with confirmed COVID-19 admitted to 60 centres in 10 countries | Mild to critical Mean age: NR (no further details provided) |
1062 | Remdesivir 100 mg/daya (n = 541) (delivered intravenously) |
Placebo (n = 521) | 28 days | Public/on-profit | Some concerns |
Mahajan et al. 202199 (status: published) (NR) |
RCT, unblinded | Patients with confirmed COVID-19 admitted to a single centre in India | Moderate to severe Mean age: NR but includes adults aged between 18 and 60 years |
82 | Remdesivir 100 mg/daya (n = 41) (delivered intravenously) |
Standard care (n = 41) | 24 days | None | High RoB |
Spinner et al. 2020100 (status: published) (NCT04292730) |
RCT, unblinded | Patients with COVID-19 admitted to 105 centres in the USA, Europe and Asia | Mild to severe Mean age: NR but includes patients ≥ 12 years |
596 | Remdesivir 100 mg/daya (5 and 10 arms days merged) (n = 396) (delivered intravenously) |
Standard care (n = 200) | 28 days | Private | Some concerns |
Wang et al. 2020101 (status: published) (NCT04257656) |
RCT, double blind | Patients with confirmed COVID-19 admitted to 10 centres in China | Severe Mean age: NR but includes adults aged ≥ 18 years |
237 | Remdesivir 100 mg/day a (n = 158) (delivered intravenously) |
Placebo (n = 79) | 28 days | Mixed (Public/ Private) |
Some concerns |
Gottlieb et al. 2021102 (status: published) PINETREE (NCT04501952) |
RCT, double blind | Outpatients with confirmed COVID-19 (mild) treated at 64 centres in Denmark, Spain, UK and USA. | Mild outpatients Mean age: NR but includes patients ≥ 12 years |
584 | Remdesivir 100 mg/day a (n = 292) (delivered intravenously) |
Placebo (n = 292) | 28 days | Private | Some concerns |
Sotrovimab | |||||||||
Gupta et al. 2022103 (status: published) COMET-ICE (NCT04545060) |
RCT, double blind | Outpatients with confirmed COVID-19 (mild) and at high risk for Covid-19 progression, treated at 57 centres in the USA, Canada, Brazil, Spain and Peru | Mild outpatients Mean age: NR but includes adults aged ≥ 18 years |
1057 | Sotrovimab, 500 mg once-off (n = 528) (delivered intravenously) |
Placebo (n = 529) | 168 days | Private | Some concerns |
Tixagevimab/cilgavimab | |||||||||
Montgomery et al. 2022 (status: published)57 TACKLE (NCT04723394) |
RCT, triple blind | Outpatients with confirmed COVID-19 (mild) treated at 95 centres in Argentina, Brazil, Czech Republic, Germany, Italy, Japan, Mexico, Poland, Spain, Russian Federation, the UK, Ukraine and the USA | Mild to moderate Mean age: NR but includes adults aged ≥ 18 years |
910 | Tixagevimab, 300 mg plus cilgavimab, 300 mg intramuscular injection (n = 456) | Placebo (n = 454) |
28 days | Private | Some concerns |
Tocilizumab | |||||||||
ARCHITECTS, 2021104 (status: unpublished) (NCT04412772) |
RCT, double blind | Patients with confirmed COVID-19 admitted to a single centre in the USA | Critical Mean age: NR (no further details provided) |
21 | Tocilizumab 8 mg/kg once-off (n = 10) (delivered intravenously) |
Placebo (n = 11) | 90 days | Public/non-profit | Low RoB |
Broman et al. 2022105 (status: published) COVIDSTORM (NCT04577534) |
RCT, unblinded | Patients with confirmed COVID-19 admitted to a single centre in Finland | Moderate to-severe Mean age: NR but includes adults aged ≥ 18 years |
88 | Tocilizumab 400 to 800 mg once-off, depending on weight (n = 59) (delivered intravenously) |
Standard care (n = 29) | 90 days | No specific funding | Some concerns |
COVIDOSE-2, 2021 (status: unpublished) (NCT04479358) |
RCT, unblinded | Patients with confirmed COVID-19 admitted to multiple centres in the USA | Moderate to severe Mean age: NR but includes adults aged ≥ 18 years |
28 | Tocilizumab 40 mg or 120 mg once-off (n = 20) (delivery method NR) |
Standard care (n = 8) | 28 days | Public/non-profit | Low RoB |
Declercq et al. 2021106 (status: published) COV-AID, 2021 (NCT04330638) |
RCT, unblinded | Patients with suspected or confirmed COVID-19 admitted to 16 centres in Belgium | Moderate to critical Mean age: NR but includes adults aged > 18 years |
342 (multiarm trial) | Tocilizumab 8 mg/kg once-off (n = 82) (delivered intravenously) |
Standard care (n = 72) | 90 days | Public/non-profit | Some concerns |
Derde et al. 2021107 (status: preprint) REMAP-CAP (NCT02735707) |
RCT, unblinded | Patients with suspected or confirmed COVID-19 admitted to 133 centres in 9 countries (UK, Netherlands, Ireland, Australia, New Zealand, Canada, Finland, Italy and Saudi Arabia) |
Severe to critical Mean age: NR but includes adults aged > 18 years |
2253 (multiarm trial) | Tocilizumab, 8 mg/kg once-off (n = 972) (delivered intravenously) |
Standard care (n = 418) | 90 days | Mixed | Some concerns |
Hermine et al. 2020108 (status: published) CORIMUNO-TOCI 1 (NCT04331808) |
RCT, unblinded | Patients with COVID-19 admitted to 9 centres in France | Moderate to severe Mean age: NR (no further details provided) |
131 | Tocilizumab 8 mg/kg (n = 64) (delivered intravenously) | Standard care (n = 67) | 60 days | Public/non-profit | Some concerns |
Hermine et al. 2022109 (status: published) CORIMUNO-TOCI-2 (NCT04331808) |
RCT, unblinded | Patients with suspected or confirmed COVID-19 admitted to 12 centres in France | Severe to critical Mean age: NR (no further details provided) |
97 | Tocilizumab 8 mg/kg once-off (n = 51) (delivery method NR) |
Standard care (n = 46) | 90 days | Public/non-profit | Some concerns |
HMO-0224-20, 2021 (status: unpublished) | RCT, unblinded | Patients with confirmed COVID-19 admitted to multiple centres in Israel | Severe-critical Mean age: NR but includes adults aged ≥ 18 years |
54 | Tocilizumab 8 mg/kg once-off (n = 37) (delivered intravenously) | Placebo (n = 17) | 90 days | Public/non-profit | High RoB |
Horby et al. 2021110 (status: published) RECOVERY (TCZ) (NCT04381936) |
RCT, unblinded | Patients with suspected or confirmed COVID-19 admitted to 131 centres in the UK | Moderate to critical Mean age: NR (no further details provided) |
4116 | Tocilizumab 400 to 800 mg, depending on weight (n = 2022) (delivered intravenously) | Standard care (n = 2094) | 28 days | Public/non-profit | Some concerns |
IMMCOVA, 2021 (status: unpublished) (NCT04412291) |
RCT, unblinded | Patients with confirmed COVID-19 admitted to multiple centres in Sweden | Moderate to severe Mean age: NR but includes adults aged ≥ 18 years |
49 | Tocilizumab, 8 mg/kg once-off (n = 22) (delivered intravenously) | Standard care (n = 27) | 28 days | Public/non-profit | Low RoB |
Rosas et al. 2022111 (status: published) COVACTA (NCT04320615) |
RCT, double blind | Patients with confirmed COVID-19 admitted to multiple centres across 9 countries (Canada, Denmark, France, Germany, Italy, Netherlands, Spain, the UK and USA) | Mild to critical Mean age: NR but includes adults aged ≥ 18 years |
452 | Tocilizumab, 8 mg/kg (n = 301) (delivered intravenously) | Placebo (n = 151) | 60 days | Mixed | Some concerns |
Rosas et al. 2021112 (status: published) REMDACTA (NCT04409262)b |
RCT, double blind | Patients with confirmed COVID-19 admitted to multiple centres in Spain, USA, Brazil and Russia | Severe to critical Mean age: NR (no further details provided) |
649 | Tocilizumab 8 mg/kg once-off or twice (n = 434) (delivery method NR) |
Placebo (n = 215) | 60 days | Private | Some concerns |
Rutgers et al. 2021113 (status: published) (Trial NL8504) |
RCT, unblinded | Patients with confirmed COVID-19 admitted to 11 centres in the Netherlands | Moderate to critical Mean age: NR but includes adults aged ≥ 18 years |
354 | Tocilizumab, 8 mg/kg once-off (n = 174) (delivered intravenously) |
Standard care (n = 180) | 90 days | Mixed | Some concerns |
Salama et al. 2020114 (status: published) EMPACTA (NCT04372186) |
RCT, double blind | Patients with confirmed COVID-19 admitted to 65 centres in Brazil, Kenya, Mexico, Peru, South Africa and the USA | Mild to severe Mean age: NR but includes adults aged ≥ 18 years |
388 | Tocilizumab, 8 mg/kg (n = 259) (delivered intravenously) |
Placebo (n = 129) | 60 days | Private | Some concerns |
Salvarani et al. 2020114 (status: published) (NCT04346355) |
RCT, unblinded | Patients with confirmed COVID-19 admitted to 24 centres in Italy | Severe Mean age: NR but includes adults aged ≥ 18 years |
126 | Tocilizumab, 8 mg/kg (n = 60) (delivered intravenously) |
Standard care (n = 66) | 30 days | Mixed | Some concerns |
Stone et al. 2020116 (status: published) (NCT04356937) |
RCT, double blind | Patients with COVID-19 admitted to 7 centres in the USA | Mild to severe Mean age: NR but includes adults aged 19 to 85 years |
243 | Tocilizumab, 8 mg/kg once-off (n = 161) (delivered intravenously) |
Placebo (n = 82) | 28 days | Private | Low RoB |
Talaschian et al. 2021117 (status: preprint) IRCT200810 27001411N4 |
RCT, double blind | Patients with confirmed COVID-19 admitted to a single centre in Iran | Moderate to severe Mean age: NR (no further details provided) |
40 | Tocilizumab, 8 mg/kg (n = 20) (delivered intravenously) |
Standard care (n = 20) | 28 days | Public/non-profit | High RoB |
Veiga et al. 2021118 (status: published) TOCIBRAS (NCT04403685) |
RCT, unblinded | Patients with confirmed COVID-19 admitted to 9 centres in Brazil | Moderate to critical Mean age: NR but includes adults aged ≥ 18 years |
129 | Tocilizumab, 8 mg/kg once off (n = 65) (delivered intravenously) |
Standard care (n = 64) | 29 days | Mixed | Some concerns |
Appendix 2 Summary of evidence from clinical studies and pooled effects used to inform the economic model
Appendix 3 Graphical representation of the clinical effectiveness results
As seen in Figure 22, all treatments have a beneficial mean estimate for the HR associated with death. The CIs of each treatment overlap showing that there is considerable uncertainty in the ranked order of clinical effectiveness. A similar conclusion related to the ranking of interventions for clinical improvement can be drawn from Figure 23, and for the ranking of treatments in relation to time to discharge from Figure 24, although only two interventions reported data on this measure. Figures 22–24 consist of two horizontal lines for each intervention which sit on a vertical line. The vertical line shows the lower and upper 95% CIs while the lower horizontal line provides the median value, and the upper horizontal line provides the mean value from the distribution. When the mean and the median values are close these become indistinguishable in the figures.
FIGURE 22.
The NMB for patients admitted to hospital who require supplemental oxygen when Solidarity data on time to death is used assuming a WTP of £20,000 per QALY.

FIGURE 23.
The NMB for patients admitted to hospital who do not require supplemental oxygen when Solidarity data on time to death is used assuming a WTP of £20,000 per QALY.

FIGURE 24.
The hazard ratio of death for interventions used to treat patients in hospital.

FIGURE 25.
The relative risk of clinical improvement at 28 days for interventions used to treat patients in hospital.
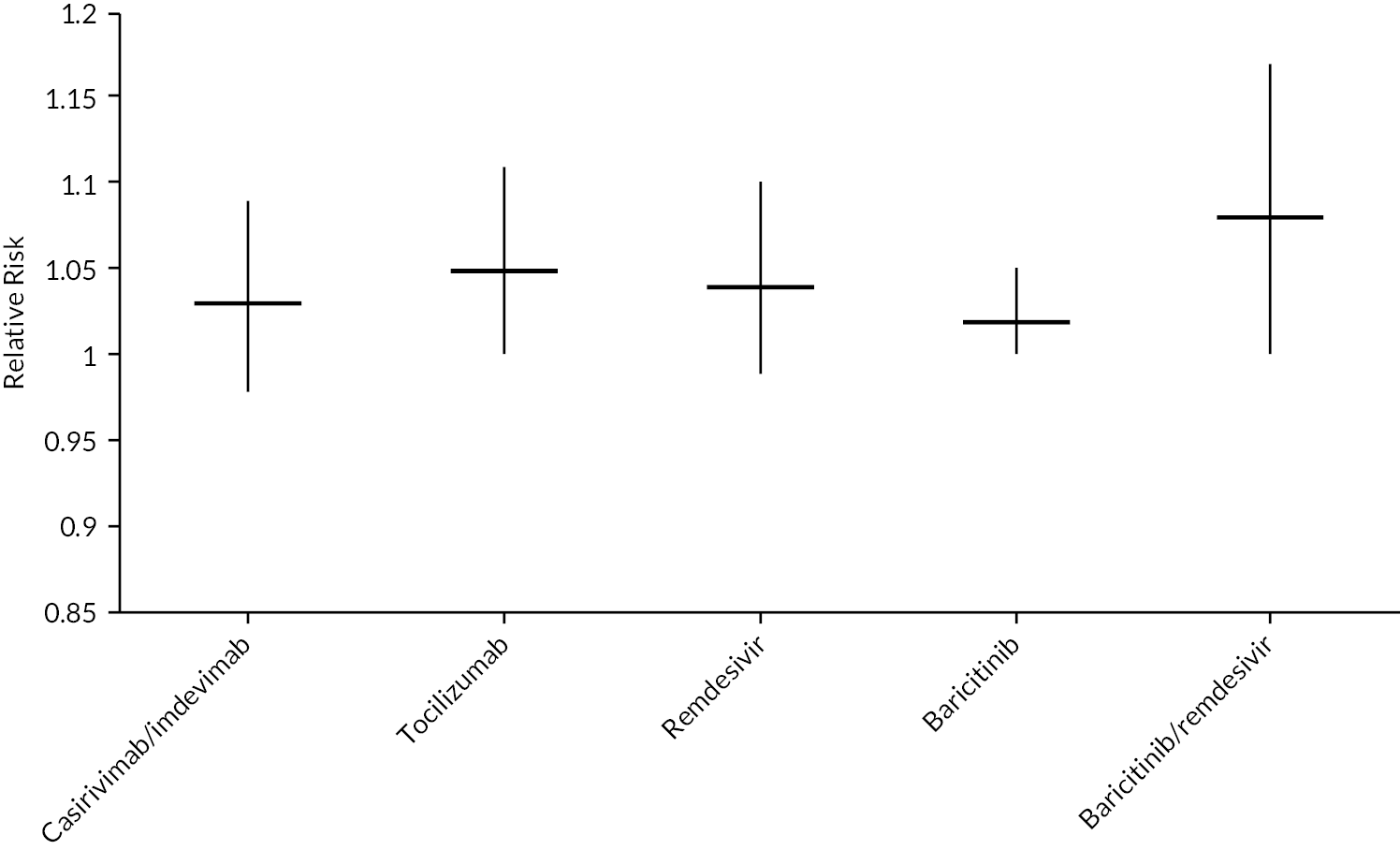
Figure 26 shows that no CI crosses unity, although the widths of the CIs differ, with that of nirmatrelvir/ritonavir having most precision, although the CI associated with this intervention overlaps with that of casirivimab/imdevimab, remdesivir and sotrovimab indicating considerable uncertainty in the most clinically effective intervention even if the assumption of generalisable efficacy holds. Figures 26 and 27 consist of two horizontal lines for each intervention which sit on a vertical line. The vertical line shows the lower and upper 95% CIs while the lower horizontal line provides the median value, and the upper horizontal line provides the mean value from the distribution. When the mean and the median values are close these become indistinguishable in the figures.
FIGURE 26.
The hazard ratio of discharge for interventions used to treat patients in hospital.

FIGURE 27.
The estimated probability that each intervention is ranked first through to fifth for hazard ratio for mortality.
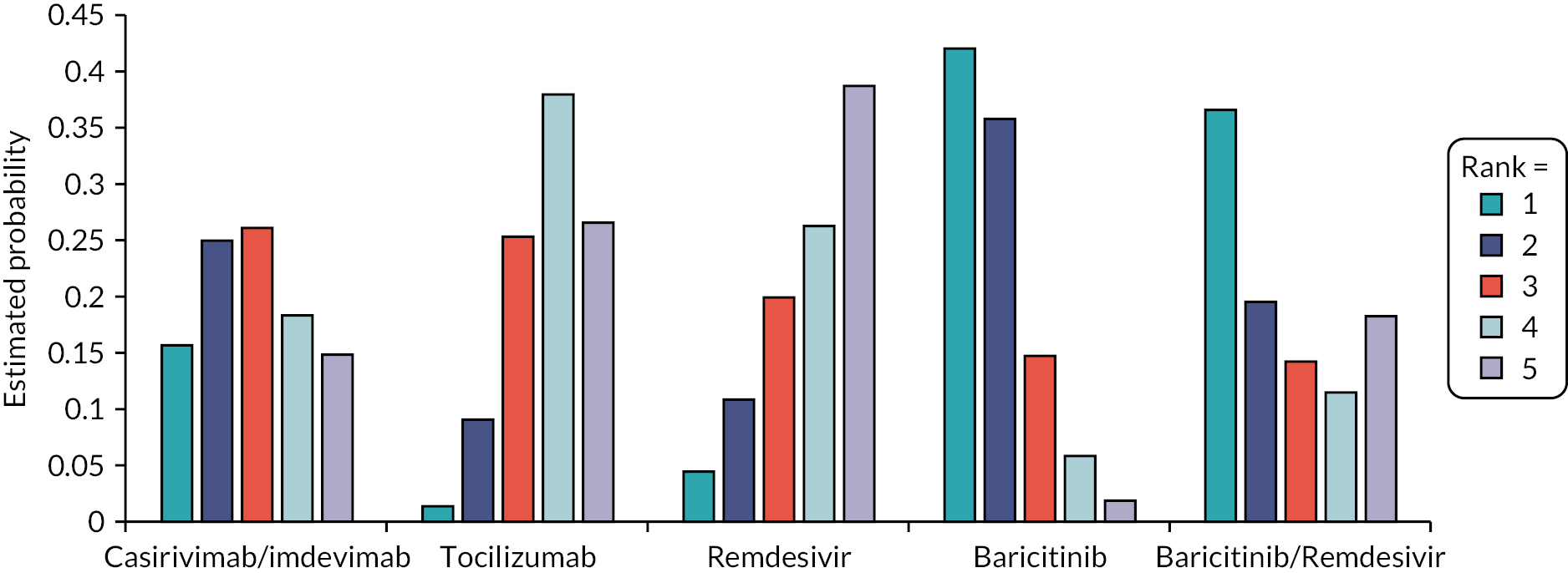
FIGURE 28.
The relative risk of hospitalisation or death at 28 days for interventions used to treat patients in the community.

FIGURE 29.
The relative risk of death at 28 days for interventions used to treat patients in the community.

FIGURE 30.
The estimated probability that each intervention is ranked first through to sixth for preventing mortality at 28 days when treating high-risk patients in the community.

FIGURE 31.
Linear assumptions for distribution across the five ordinal scales during hospital stay.

For the risk of death at 28 days, Figure 27 shows wide CIs for all treatments excluding molnupiravir and nirmatrelvir/ritonavir, in which the upper confidence limits do not exceed 1.0. The wide CIs are primarily related to the sample size and the small number of observed events in each arm.
Appendix 4 Additional analyses
FIGURE 32.
Base case net monetary benefits for patients admitted to hospital who require supplemental oxygen assuming a threshold of £30,000 per QALY gained.

FIGURE 33.
Base case net monetary benefits for patients admitted to hospital who do not require supplemental oxygen assuming a threshold of £30,000 per QALY gained.
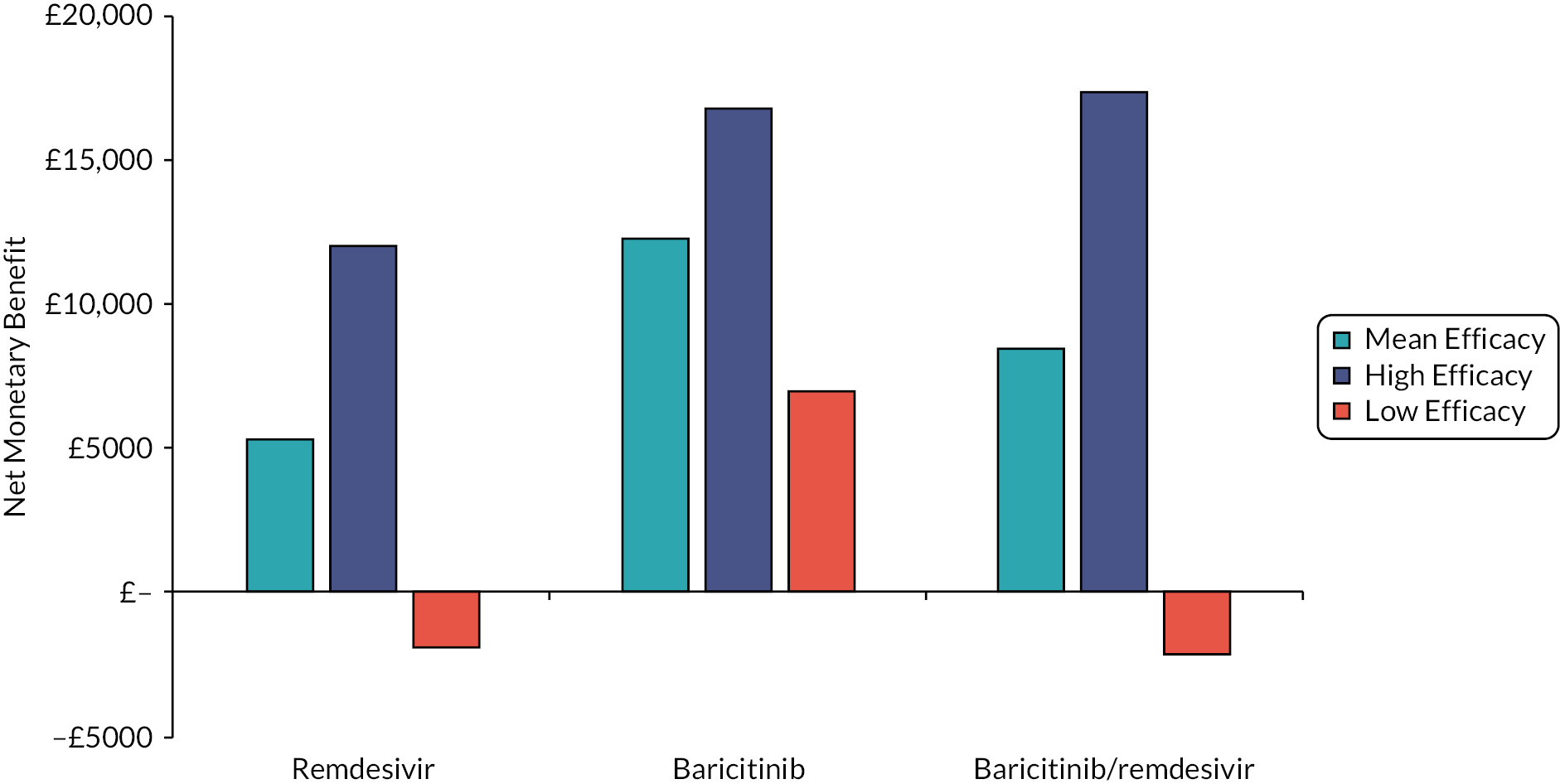
FIGURE 34.
Base case net monetary benefits for patients with COVID-19 in the community and high risk of hospitalisation assuming a threshold of £30,000 per QALY gained.

FIGURE 35.
The NMB results for patients admitted to hospital who require supplemental oxygen when the duration of long COVID is halved and doubled. Assuming a WTP of £30,000.
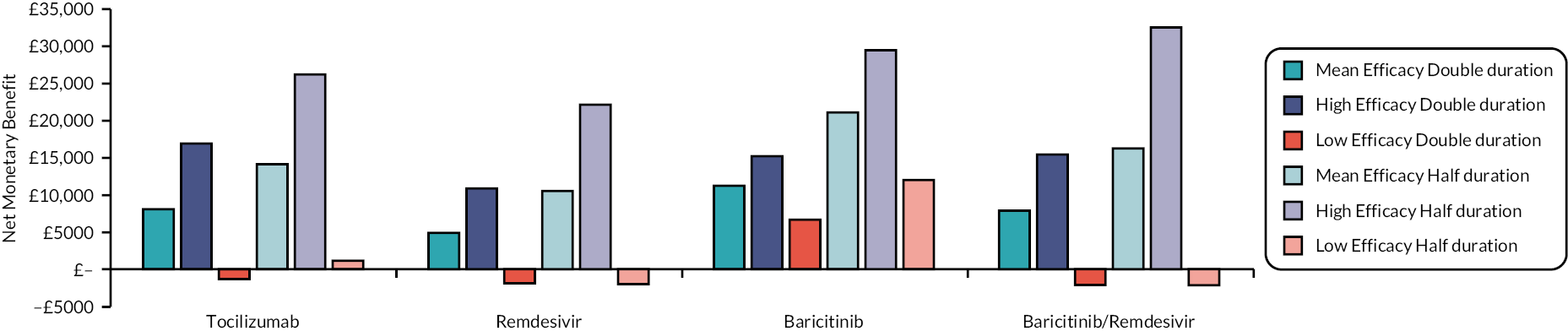
FIGURE 36.
The NMB results for patients admitted to hospital who do not require supplemental oxygen when the duration of long COVID is halved and doubled. Assuming a WTP of £30,000.

FIGURE 37.
The NMB results for patients in the community with COVID-19 who are at high risk of hospitalisation when the duration of long COVID is halved and doubled. Assuming a WTP of £30,000.

FIGURE 38.
The NMB results for patients in the community with COVID-19 who are at high risk of hospitalisation when the hospital admission percentage was changed. Assuming a WTP of £30,000.

FIGURE 39.
The NMB results for patients in the community with COVID-19 who are at high risk of hospitalisation when the age was changed from 55 years to 50 and 60 years. Assuming a WTP of £30,000 per QALY.
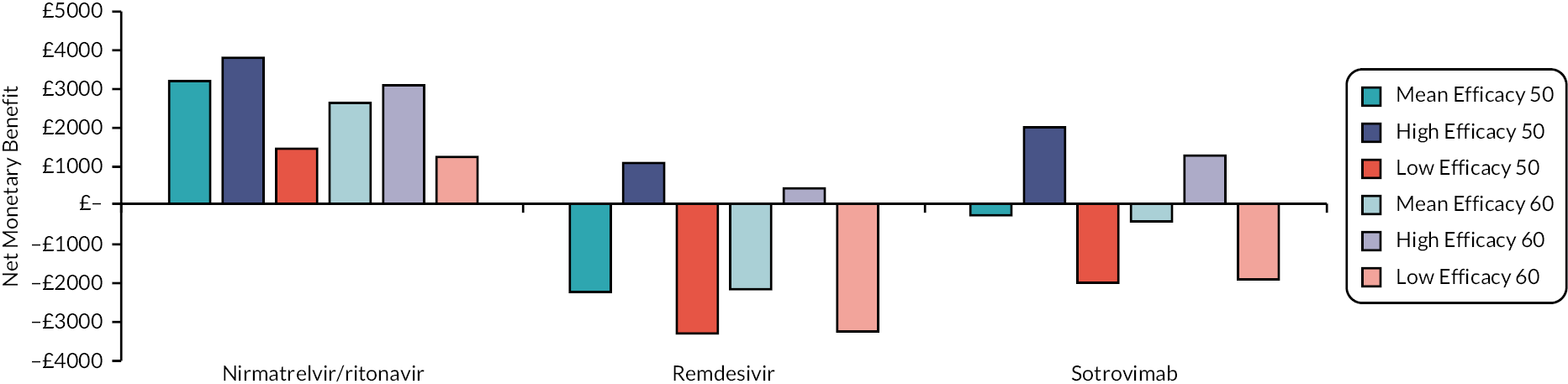
FIGURE 40.
The NMB when a HR of unity was used for all interventions in relation to time to hospital discharge and time to clinical improvement for patients requiring supplemental oxygen. Assuming a WTP of £30,000 per QALY.
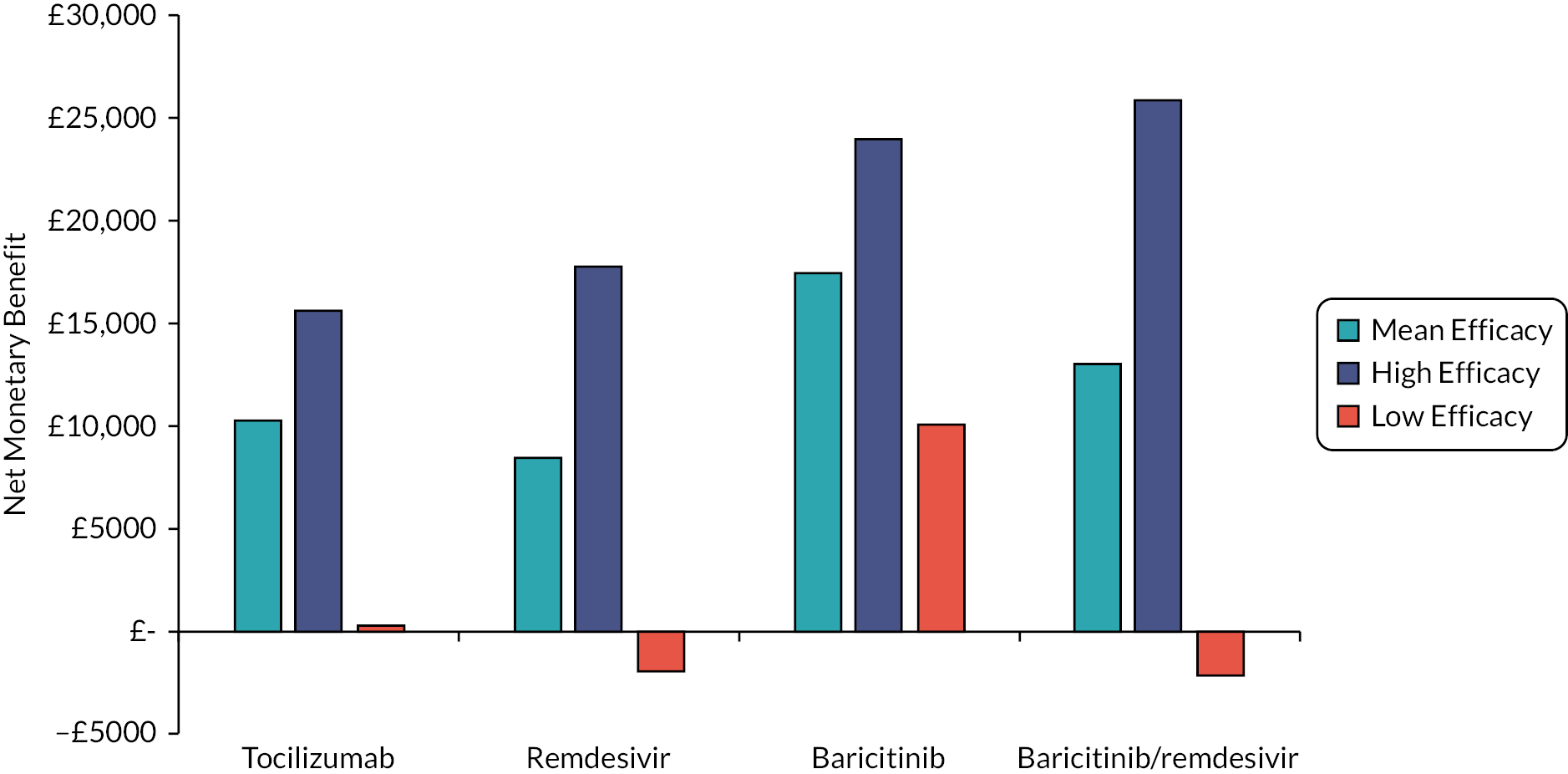
FIGURE 41.
The NMB when a HR of unity was used for all interventions in relation to time to hospital discharge and time to clinical improvement for patients not requiring supplemental oxygen. Assuming a WTP of £30,000 per QALY.
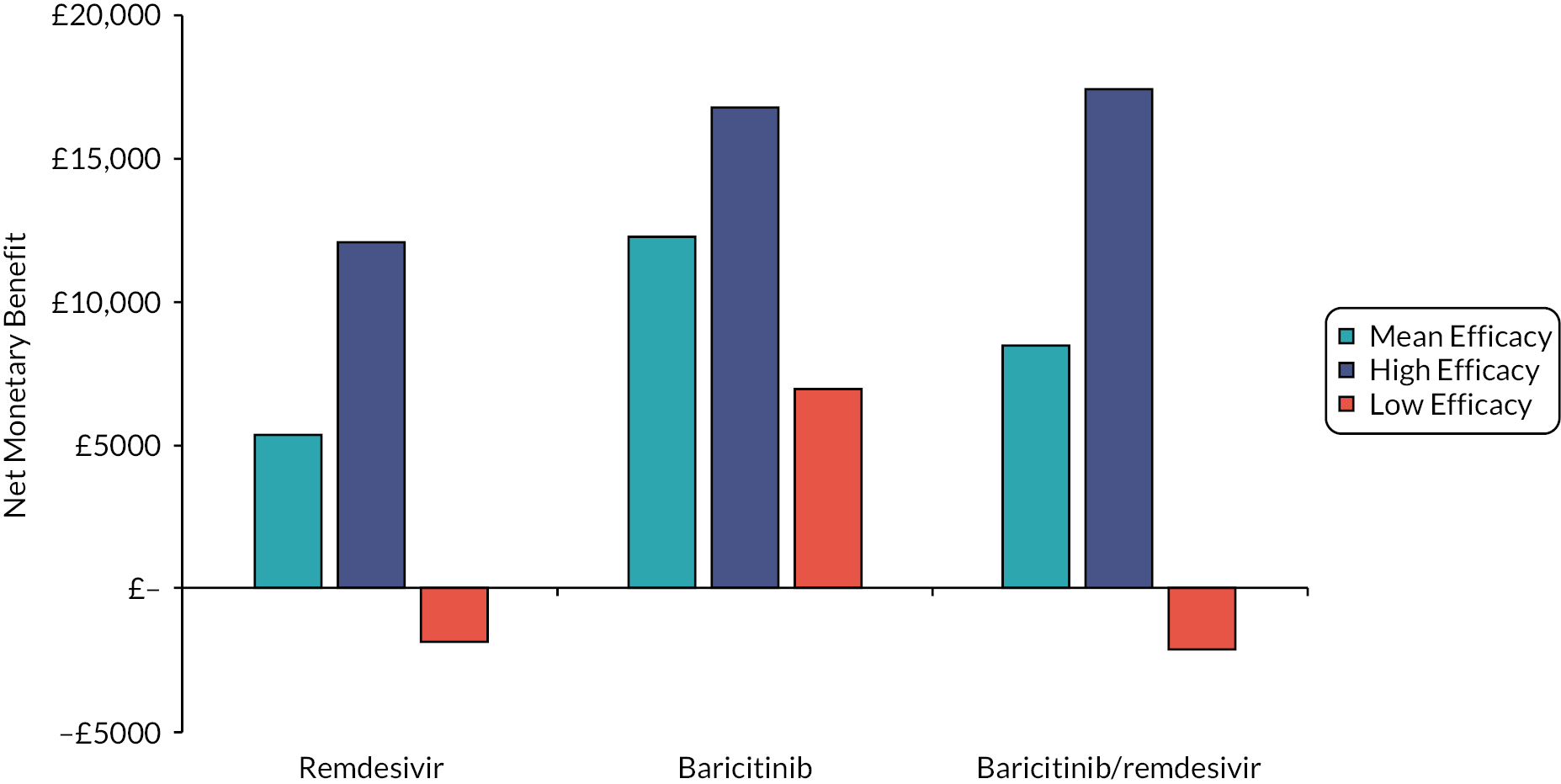
FIGURE 42.
The NMB results when treatment in the community for high-risk patients was associated with less supplemental oxygen on admission to hospital. Assuming a WTP of £30,000 per QALY.
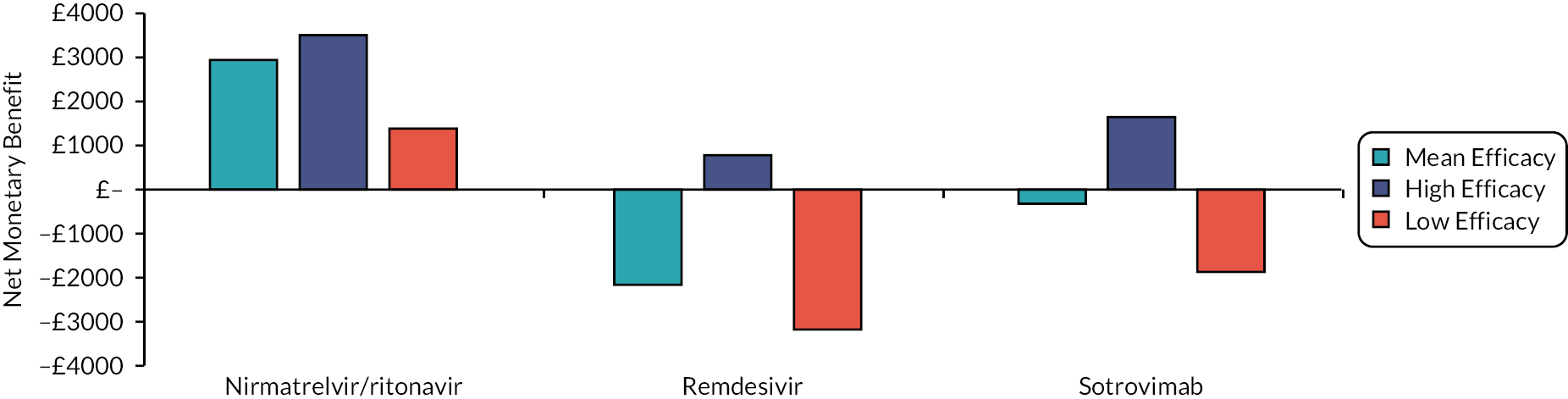
FIGURE 43.
The NMB results when a disutility of 0.02 per day is assumed for patients receiving intravenous (i.v.) treatment in the community. Assuming a WTP of £30,000 per QALY.
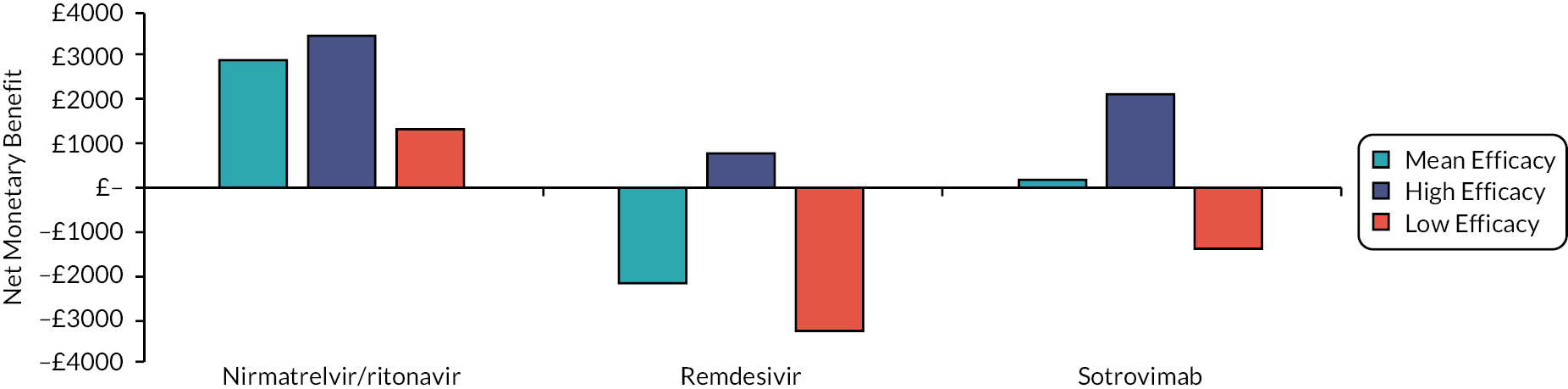
FIGURE 44.
The NMB results for patients admitted to hospital who require supplemental oxygen when the SMR associated with long COVID is changed. Assuming a WTP of £30,000 per QALY.

FIGURE 45.
The NMB results for patients admitted to hospital who do not require supplemental oxygen when the SMR associated with long COVID is changed. Assuming a WTP of £30,000 per QALY.
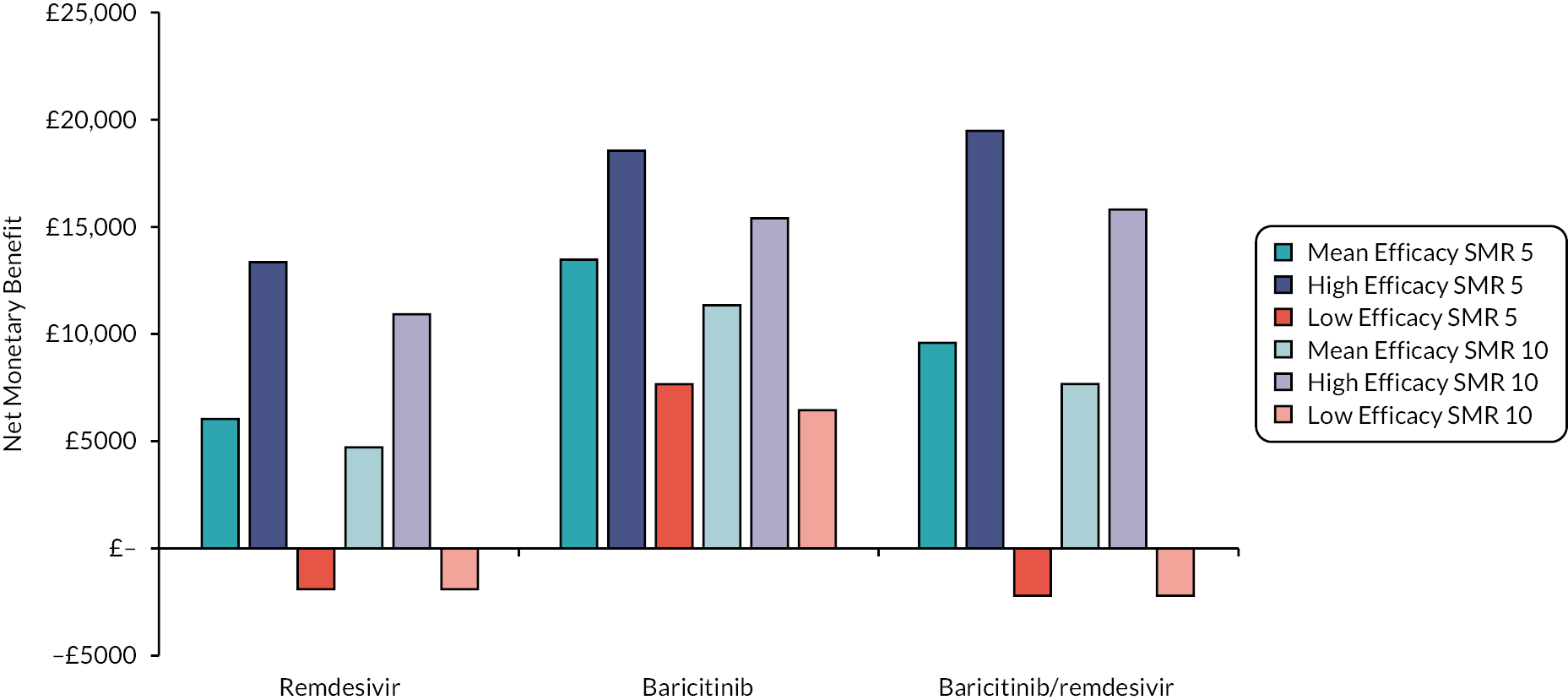
FIGURE 46.
The NMB results for high-risk patients in the community when the SMR associated with long COVID is changed. Assuming a WTP of £30,000 per QALY.
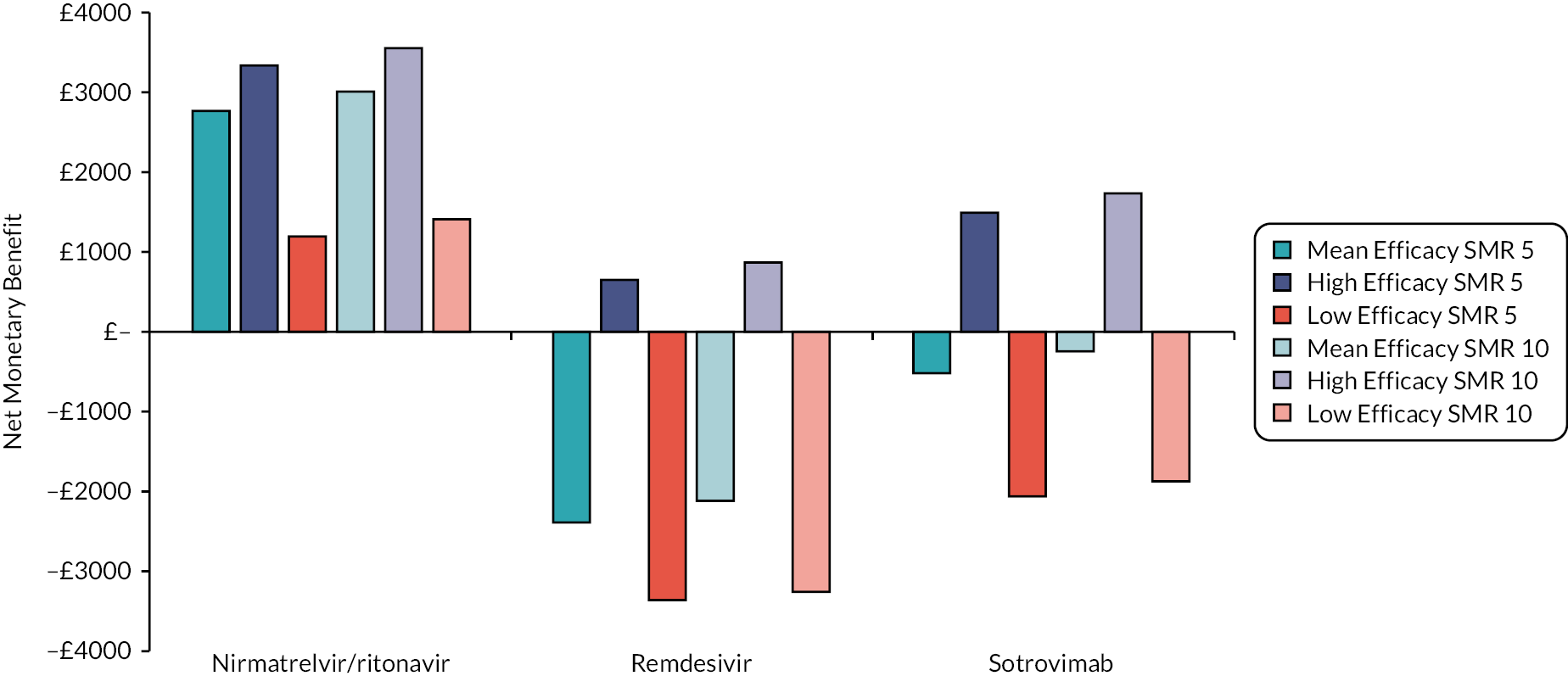
FIGURE 47.
The NMB for patients admitted to hospital who require supplemental oxygen when Solidarity data on time to death is used assuming a WTP of £30,000 per QALY.

FIGURE 48.
The NMB for patients admitted to hospital who do not require supplemental oxygen when Solidarity data on time to death is used assuming a WTP of £30,000 per QALY.

List of abbreviations
- ACTT-1
- Adaptive COVID-19 Treatment Trial
- CI
- confidence interval
- CMDU
- COVID Medicines Delivery Units
- COVID-19
- coronavirus disease 2019
- EAG
- External Assessment Group
- ECMO
- extracorporeal membrane oxygenation
- EQ-5D-3L
- EuroQol-5 Dimensions, three-level version
- EQ-5D-5L
- EuroQol-5 Dimensions, five-level version
- FCE
- finished cost episode
- HFO
- high-flow oxygen
- HR
- hazard ratio
- HRQoL
- health-related quality of life
- ICER
- incremental cost-effectiveness ratio
- IMV
- invasive mechanical ventilation
- IPD
- individual patient-level data
- i.v.
- intravenous
- KM
- Kaplan–Meier
- LFO
- low-flow oxygen
- MAVs
- medical attended visits
- NHS
- National Health Service
- NICE
- National Institute for Health and Care Excellence
- NIV
- non-invasive ventilation
- NMA
- network meta-analyses
- NMB
- net monetary benefit
- ONS
- Office for National Statistics
- OS
- overall survival
- PANORAMIC
- Platform Adaptive trial of NOvel antiviRals for eArly treatMent of COVID-19 In the Community clinical study
- PSA
- probabilistic sensitivity analysis
- QALY
- quality-adjusted life-year
- RCT
- randomised controlled trial
- RECOVERY
- Randomised Evaluation of COVid-19 thERapY
- RR
- relative risk
- SAE
- serious adverse events
- SARS-CoV-2
- severe acute respiratory syndrome coronavirus 2
- SMR
- standardised mortality ratio
- SoC
- standard of care
- WHO
- World Health Organization
- WTP
- willingness to pay