Notes
Article history
The research reported in this issue of the journal was funded by the HTA programme as award number 17/148/03. The contractual start date was in April 2019. The draft manuscript began editorial review in April 2023 and was accepted for publication in September 2023. The authors have been wholly responsible for all data collection, analysis and interpretation, and for writing up their work. The HTA editors and publisher have tried to ensure the accuracy of the authors’ manuscript and would like to thank the reviewers for their constructive comments on the draft document. However, they do not accept liability for damages or losses arising from material published in this article.
Permissions
Copyright statement
Copyright © 2024 Feng et al. This work was produced by Feng et al. under the terms of a commissioning contract issued by the Secretary of State for Health and Social Care. This is an Open Access publication distributed under the terms of the Creative Commons Attribution CC BY 4.0 licence, which permits unrestricted use, distribution, reproduction and adaptation in any medium and for any purpose provided that it is properly attributed. See: https://creativecommons.org/licenses/by/4.0/. For attribution the title, original author(s), the publication source – NIHR Journals Library, and the DOI of the publication must be cited.
2024 Feng et al.
Chapter 1 Background
Introduction
Streptococcus pneumoniae (pneumococcus) causes severe diseases, including bacterial pneumonia, meningitis and sepsis, leading to substantial morbidity and mortality worldwide, with the highest disease burden being in young children and older adults. 1,2 There have been more than 100 serotypes of pneumococcus documented as of 2020, not all of which cause severe diseases, and the distribution of these serotypes varies substantially between countries. 1,2 Three pneumococcal conjugate vaccines (PCVs) have been widely deployed worldwide in the past two decades: PCV7 (Prevnar; Pfizer), PCV10 (Synflorix; GlaxoSmithKline) and PCV13 (Prevenar 13; Pfizer), resulting in substantial reduction in disease. 1,3 New PCVs such as PCV15, PCV20 and PCV10-SII have been licensed in some countries but have yet to be widely deployed.
Between 2009 and 2011, PCV7 was gradually replaced by PCV13 and PCV10 and is no longer available. Currently, three PCVs are recommended by the World Health Organization (WHO) for infants worldwide: PCV13, PCV10 and a new 10-valent PCV manufactured by Serum Institute of India (PCV10-SII, Pneumosil) which was prequalified by WHO in December 2019. 3,4 PCV13 provides additional serotypes (3, 6A and 19A) to the 10 serotypes included in PCV10 (serotype 1, 4, 5, 6B, 7F, 9V, 14, 18C, 19F and 23F). PCV10-SII covers serotypes 1, 4, 5, 6B, 7F, 9V, 14, 18C, 19F and 23F. The licensure of PCVs is benchmarked against anticapsular immunoglobulin G (IgG) antibody responses above a threshold of 0.35 mcg/ml for all vaccine serotypes, which was established using data from three randomised controlled efficacy trials. 5 Real-world evidence suggests that correlates of protection and effectiveness against invasive pneumococcal disease vary across serotypes. 6
The WHO does not preferentially endorse one PCV over another. Both PCV13 and PCV10 have been shown to provide both direct and indirect protection against pneumococcal pneumonia, invasive pneumococcal disease and nasopharyngeal carriage. 3,7 Although there are 10 common serotypes in these two vaccines, the content of the vaccines differs, with different carrier proteins used in the conjugation process, as well as different amounts of polysaccharide, and these differences may contribute to differences in protection. In 2017, a systematic review of studies directly comparing PCV10 with PCV13 showed differences in anti-pneumococcal IgG responses between vaccines. However, no meta-analysis has been included in this review, and there remains uncertainty over whether one vaccine is consistently more immunogenic and whether differences in immunogenicity result in clinically important differences in protection. Large randomised controlled trials (RCTs) directly comparing different PCVs with invasive pneumococcal disease as the primary outcome are not feasible. Studies that assessed the impact of different PCVs on nasopharyngeal carriage have reported very few or no differences. 8,9 Episodes of nasopharyngeal carriage often last only a few days or weeks, and therefore cross-sectional swabbing studies may misclassify participants when swabs are not taken at the time of infection, resulting in underpowered comparisons. We previously used ‘seroefficacy’ as an outcome for estimating correlates of protection for PCVs against pneumococcal carriage,10 where seroinfection is defined as an increase in antibody levels between the primary vaccination series (typically complete at 5–7 months of age) and the booster dose (typically administered at 9–18 months of age). Seroinfection can be regarded as evidence of exposure to the pathogen and a resultant subclinical infection, given antibody responses wane rapidly during this period otherwise. 10
In this study, we meta-analysed individual participant data from studies of PCVs to compare the immunogenicity and seroefficacy of PCV10 with PCV13 for each serotype. We aimed to determine if serotype-specific immune responses were higher for either vaccine and whether this resulted in greater protection again carriage (seroefficacy) for the same serotypes. In addition, we explored the overall relationship between the higher immune response and protection against carriage in infants.
Chapter 2 Methods
Our systematic review is reported in line with the recommendations from the Preferred Reporting Items for Systematic Reviews and Meta-Analyses statement plus the extension statements for network and individual patient data systematic reviews. 11–13
Primary and secondary objectives
The primary objective was to compare the immunogenicity of PCV10 versus PCV13 for each serotype contained in the vaccines.
The secondary objectives were:
-
4. to compare the seroefficacy of PCV10 versus PCV13 for each serotype contained in the vaccines
-
5. for PCV10 and PCV13 separately, to estimate immunogenicity and seroefficacy in comparison to the older PCV7 vaccine
-
6. to determine how the comparisons of immunogenicity and efficacy of PCV10 to PCV13 are affected by the co-administration of different routine vaccines.
Outcomes
The primary outcome was serotype-specific anticapsular pneumococcal IgG antibodies measured at three time points: (1) 1 month after the primary series of one to three doses of vaccine in infancy, (2) prior to a booster dose and (3) 1 month after a booster dose.
The outcome for seroefficacy analyses was a binary variable for seroinfection defined as a rise in anti-serotype-specific IgG between the post-primary time point and the booster dose. As a binary variable, seroinfection was equivalent to 1 if antibody levels increased by any amount during this period, or 0 otherwise. This outcome was only able to be derived if individual participant data were available at both time points.
Systematic review
We conducted a systematic review identifying studies that compared the immunogenicity of licensed PCVs for infants or children in randomised trials. The PCVs included in the systematic review were:
-
7-valent pneumococcal conjugate vaccine (PCV7: Prevnar; Pfizer), containing serotypes 4, 6B, 9V, 14, 18C, 19F and 23F, each conjugated to diphtheria cross-reacting material (CRM).
-
13-valent pneumococcal conjugate vaccine (PCV13: Prevenar 13; Pfizer), containing serotypes 1, 3, 4, 5, 6A, 6B, 7F, 9V, 14, 18C, 19A, 19F and 23F, each conjugated to diphtheria CRM.
-
10-valent pneumococcal conjugate vaccine (PCV10: Synflorix; GlaxoSmithKline), containing serotypes 1, 4, 5, 6B, 7F, 9V, 14, 18C, 19F and 23F, conjugated to non-typeable Haemophilus influenzae protein D, for eight serotypes, or tetanus or diphtheria protein (serotypes 18C and 19F, respectively).
PCV7 was included even though no longer available, so that we could compare PCV13 and PCV10 indirectly through them each being compared with PCV7 for the same serotypes.
The search strategy was devised and conducted by an information specialist (NR). Five databases and two trial registers were searched from database inception to 27 July 2022. The original search was run in June 2019, with an update search run in July 2022. The databases searched were Cochrane Database of Systematic Reviews and Cochrane Central Register of Controlled Trials (CENTRAL; Cochrane Library, Wiley) (Issue 7 of 12, July 2022), EMBASE (OvidSP) (1974–present), Global Health (OvidSP) (1973–2022 Week 29) and MEDLINE (OvidSP) (1946–present). The trial registers searched were ClinicalTrials.gov (https://clinicaltrials.gov/) and WHO International Clinical Trials Registry Platform (https://trialsearch.who.int/). The search comprised title/abstract keywords and subject headings for pneumococcal vaccines and children. A methodological search filter for RCTs taken from the Cochrane Handbook was used to limit to RCTs. 10,11 Pharmaceutical company websites (GlaxoSmithKline and Pfizer) were also hand-searched for relevant studies. A full list of search terms for each database is summarised in Appendix 1. No date or language limits were applied. References were exported to EndNote 20 for de-duplication.
Study selection
Two reviewers (JM, NP) independently reviewed the title and abstract of each reference and identified potentially relevant references. Two reviewers (JM, NP) independently selected studies to be included in the review from retrieved full-text papers using pre-determined inclusion criteria. Disagreements about study inclusion were resolved by a third reviewer (MV).
Randomised controlled trials were included if they provided direct comparisons of either PCV7, PCV10 or PCV13 among infants and children ˂ 2 years of age and if they provided estimates on antibody responses (serotype-specific anti-pneumococcal IgG) to PCVs for at least one time point of 1 between 4 and 6 weeks after the primary vaccination series and/or 1 month after a booster vaccination. Trials were eligible only if they included at least one of the three currently licensed (PCV10 and PCV13) or previously licensed (PCV7) vaccines.
Trials were excluded if they did not contain a randomised comparison of eligible vaccines, contained only a single vaccine or enrolled immunocompromised (e.g. HIV) children.
Data retrieval
For all eligible trials, the publication authors/data owners were approached for trial and individual participant-level data. Baseline characteristics and potential effect modifiers were extracted on participants’ age, sex, country, immunogenicity assays, co-administered study vaccines and vaccine schedules. The following study-level data were extracted from trial registries/published studies:
-
trial registration number/study identifier;
-
study country;
-
PCV vaccination schedule, for example, two priming doses followed by a booster (2 + 1) or three priming doses followed by a booster (3 + 1).
Individual participant-level data were retrieved if available for following variables:
-
vaccines administered (both study vaccines and vaccines administered concomitantly as part of the routine immunisation schedule)
-
vaccination dates
-
details of laboratory assays conducted, including where assays were run, units of measurement and the lower limit of quantification
-
participants’ age at enrolment
-
participants’ sex
-
serotype-specific anti-pneumococcal IgG measured by enzyme-linked immunosorbent assay at all time points.
Aggregate data from publications were extracted if individual participant data were not available. Data extraction of published results and individual participant-level data were independently completed by SF and MV.
Statistical analysis
Immunogenicity
Each trial that had individual participant-level data available was analysed to obtain the log of the ratio of geometric means (log-GMR) and its standard error (SE) for each serotype and time point of interest. If individual participant data were unavailable, published geometric mean ratio (GMR) estimates and confidence intervals (CIs) were used. The estimates combined from individual participant data and aggregate data formed the input data for data synthesis. Sensitivity analyses for immunogenicity results were conducted by restricting analyses to only those studies providing data for all three time points of interest.
Seroefficacy
The relative risk (RR) of seroinfection was estimated by comparing the proportion of participants with seroinfection between vaccine groups. When no seroinfection occurred in any group (numerator of absolute risk was 0), a small non-zero value (0.5) was added to both numerator and denominator to allow estimation of the RR. The log-RRs and their SEs were then the input data for evidence synthesis. Only trials supplying individual participant data were included in seroefficacy analyses.
Data synthesis by network meta-analysis and meta-analysis
Serotypes 4, 6B, 9V, 14, 18C, 19F and 23F were contained in all three vaccines; therefore, evidence could be synthesised using a network meta-analysis (NMA) of all comparisons between PCVs, including PCV7. Serotypes 1, 5, 7F, 3, 6A and 19A are only included in PCV10 and PCV13 vaccines; therefore, for these serotypes, evidence was synthesised by meta-analysing studies that directly compared PCV13 versus PCV10.
For the analysis of immunogenicity, we synthesised evidence for all PCV13 serotypes. However, seroefficacy could only be assessed in situations where the serotypes of interest were included in both vaccines being compared (PCV10 and PCV13), and, therefore, seroefficacy of serotypes 3, 6A and 19A could not be assessed as these are only included in one vaccine (PCV13).
Association between ratios of immunogenicity and seroefficacy
To estimate separate serotype-specific relationships between the GMRs and RRs, study-level data were combined regressing the RR of seroinfection on the GMR using linear regression models weighted by the sample size of the study. Weighted Pearson’s correlation coefficients were calculated.
To estimate the overall association between antibody GMR and RR across all serotypes, we fitted a mixed-effect model regressing study-level RRs of seroinfection on GMRs across serotypes, weighted by the sample size of each study. Fixed effects included GMR, serotype and interactions between GMR and serotype (allowing serotype-specific association), while study was included as a random effect. As a sensitivity analysis, we reversed both RRs and GMRs estimated (i.e. PCV13 vs. PCV7 was changed to PCV7 vs. PCV13). By shifting comparators, we aimed to evaluate the stability of the association estimates.
Model fit was evaluated through a comparison of fixed-effects and mixed-effects models, as well as between models with and without interactions between GMR and serotype. The final model was selected based on the Akaike information criterion (AIC), with preference given to the model yielding the lowest AIC score, thus indicating the best fit.
Pneumococcal conjugate vaccine-10 and PCV13 are manufactured slightly differently, with different carrier proteins, conjugation process, polysaccharide concentrations and sources. To evaluate if these differences between two products change the relationship between antibody levels and protection against seroinfection, we assessed the association between immunogenicity and seroefficacy restricting to studies that compared PCV13 versus PCV10 and PCV7 versus PCV10 only (comparisons between PCV13 and PCV7 were removed from analysis, as these vaccines are from the same manufacturer). We examined whether PCVs of different manufacturers that produce equivalent levels of antibody (GMR = 1) also provide comparable seroefficacy (RR = 1).
All analyses were performed in R version 4.2.2. NMA and meta-analysis were conducted using the netmeta and metafor packages. 14,15 Code for performing NMA using the ‘netmeta’ function from the netmeta package can be found in Appendix 7.
Assessment of risk of bias in included studies
Risk of bias in results of the included studies was assessed independently by two reviewers (JM, NP) using the Cochrane RoB2. 16 This considers the risk of bias (RoB) in five domains (randomisation process, deviations from the intended interventions, missing outcome data, measurement of the outcome and selection of the reported result) and generates an overall RoB. Assessments were undertaken for immunogenicity of PCV7, PCV10 and PCV13 for each serotype contained in the vaccines. RoBs for seroefficacy outcomes are assumed to be identical because the data came from the same blood samples and were analysed in similar ways. The possible RoB judgements for each domain, and overall, are ‘low risk of bias’, ‘some concerns’ and ‘high risk of bias’. Disagreements between reviewers were resolved by consensus. Results for the RoB assessment were presented using robvis (visualisation tool). 17
Assessment of heterogeneity and inconsistency of network meta-analysis
To assess the statistical heterogeneity and inconsistency of NMA, we evaluated the transitivity assumption by visually comparing the distribution of the baseline characteristics and potential effect modifiers across the different pairwise comparisons. We assessed the presence of heterogeneity using estimated values of the heterogeneity variance parameters (τ2) and the I2 statistic and its 95% CI that measures the percentage of variability in point estimates that cannot be attributed to random error. We evaluated the inconsistency, that is, coherence between direct and indirect evidence, using a Q statistic,15 which measures the deviation from consistency. The random-effects model was fitted following the graph-theoretical approach and using the GMR and RR as effect estimate with 95% CI. 14
Some individual participant-level data were missing due to laboratory errors, insufficient blood sample volume or participant withdrawal. Data were not imputed and missing data were considered missing completely at random. Individual participant-level data were analysed according to the vaccine received.
Chapter 3 Results
Search results
Database registry and hand searches identified 4697 publication records (see Appendix 2, Figure 19), of which 47 studies (78 publication reports) satisfied our eligibility criteria. 8,9,17–93 Nineteen studies (24 publication reports) were excluded from the analysis: 6 studies did not provide individual patient or aggregate data,70–73 and 13 studies (18 publication reports) were studies directly comparing the vaccines of interest, but it was not possible to form a loop within the NMA to provide indirect evidence (Figure 1). 17,74–88 Of these 13 studies, 8 reported results from different, mainly unlicensed PCVs, including a new Cuban PCV7, PCV10-SII, PCV11, PCV12, a Chinese PCV13, PCV14, PCV15, PCV20, PCV24 and PCV SP0202-VI.
FIGURE 1.
Network of studies included for (a) all eligible cohorts, (b) immunogenicity analysis cohorts and (c) seroefficacy analysis cohorts. Studies with no closed loop to enable use of indirect evidence (n = 13), or where data were unavailable (n = 6), are included in panel a but not in panel b or c. PCV7 Cuba, a Cuban PCV7; PCV13 CNY, a Chinese PCV13.
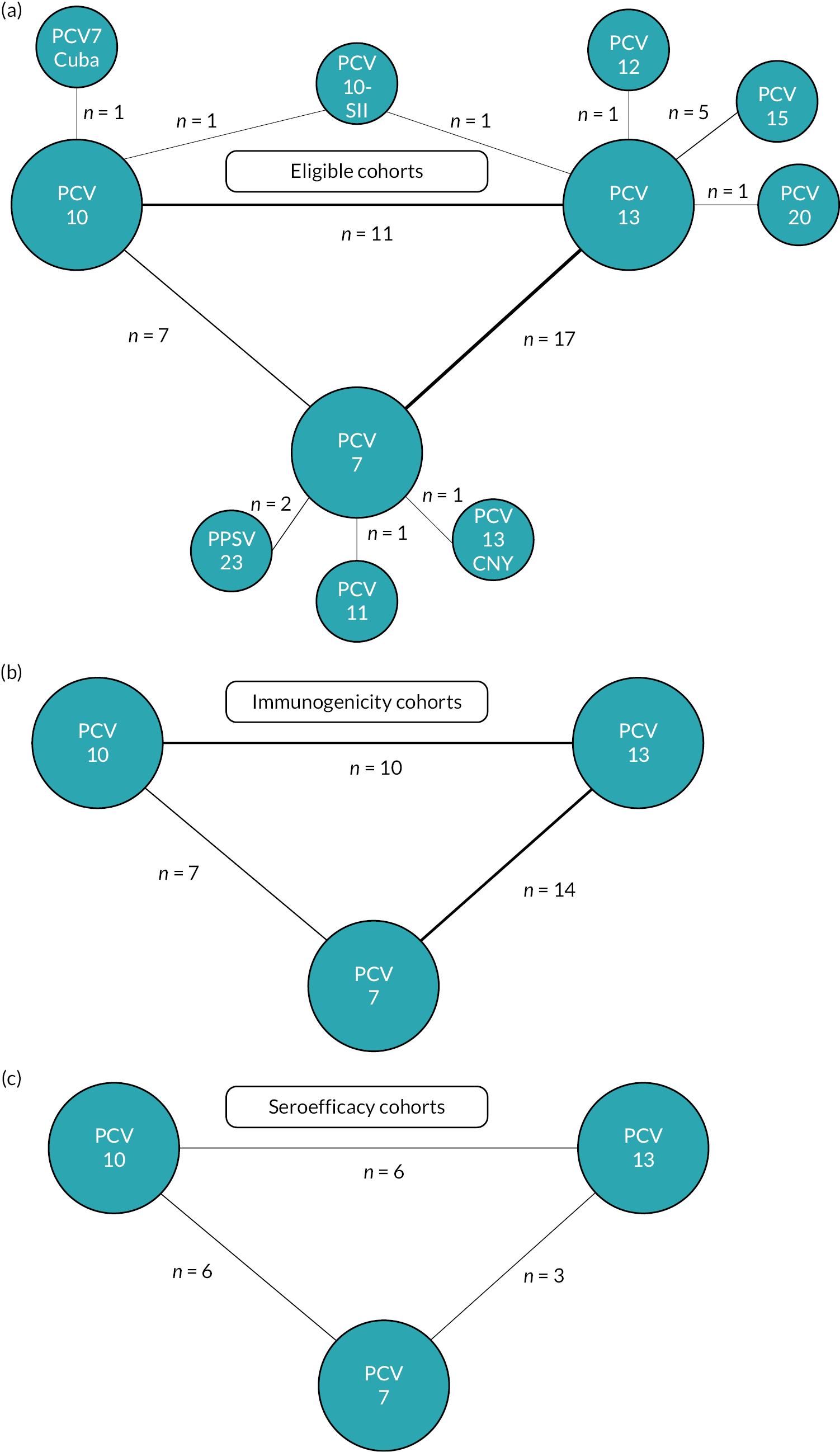
The remaining 28 studies (54 publication records) from 2009 to 2023 were included in the NMAs. 8,9,18–69,89 Twenty-two studies provided individual participant data with a further six studies reporting aggregate data (see Appendix 3, Tables 3 and 4).
The 28 included studies comprised 31 cohorts of children as 1 study conducted in 2 countries reported results separately,21,22 and 1 study included comparisons of 3 vaccination schedules19,48 (see Appendix 3, Table 3). Studies with multiple National Clinical Trial (NCT) numbers or publications, but the same population, were counted as one cohort. These 31 cohorts were representative of 38 countries in 6 continents: Europe (n = 11 cohorts), Asia (n = 9 cohorts), North America (n = 3 cohorts), Africa (n = 3 cohorts), Oceania (n = 4 cohorts) and South America (n = 1 cohort). Four cohorts were from studies conducted in multiple countries in Europe, and analyses were combined across sites. 24,53–55,64,66
There were 7 studies comparing PCV10 versus PCV7, 14 studies comparing PCV13 versus PCV7 and 8 studies comparing PCV13 versus PCV10. Two cohorts used a single prime, single boost (1 + 1) schedule with the first dose administered at either 6 or 14 weeks of age to South African infants and compared PCV13 with PCV10. 48 Five cohorts used a 2 + 1 prime-boost schedule: one study in Vietnam comparing PCV13 versus PCV10,57 one in South Africa comparing PCV13 versus PCV10 with additional comparisons with 1 + 1 schedules48 and two from studies conducted in Europe comparing PCV13 versus PCV7. 31,56 Three cohorts used a 3 + 0 schedule: one in the Gambia comparing PCV13 versus PCV10,50 one in the USA comparing PCV13 versus PCV767 and one in Germany comparing PCV10 versus PCV7. 39 The remaining 20 cohorts tested a 3 + 1 schedule, with most cohorts receiving a primary series at 2–4–6 months (n = 9) and a booster at around 12 months (n = 18).
Infants’ age at receipt of the first dose ranged from 1 to 3.5 months, and the age of the booster dose ranged from 9 to 18 months, resulting in an interval between primary and booster dose (used for the calculation of seroefficacy) of between 6 and 12 months. Most cohorts reported or cited types of co-administered vaccines (n = 24), and PCVs were commonly co-administered with routine childhood vaccine including diphtheria, tetanus and acellular/whole-cell pertussis vaccines (n = 23), Haemophilus influenzae type b tetanus toxoid (TT) conjugate vaccine (n = 23), hepatitis B vaccine (n = 20), inactivated/oral polio vaccine (n = 22) and group C meningococcal vaccine (n = 3) (see Appendix 3, Table 3). Serotype-specific IgG antibody responses were defined as primary outcomes in all studies. Studies comparing PCV10 versus PCV7 (n = 7) assessed serotypes included in PCV10, while all other studies assessed all serotypes included in PCV13. Geometric mean concentrations (GMCs) were reported at 28 days post-primary series (n = 29 cohorts), prior to a booster (n = 17 cohorts) and 28 days post booster (n = 25 cohorts). Fourteen cohorts (46.7%) reported GMC at all three time points. Individual participant data were available from 25 of 30 (83.3%) cohorts.
Assessment of risk of bias
Risk of bias assessments for the 28 included studies are summarised in Appendix 4, Figure 20. Results of 10 studies31,33,36,38,51,55,56,65,67,69 were assessed to be at ‘low risk of bias’ across all domains and overall. Two studies23,66 had results judged to be at ‘high risk of bias’ due to problems identified in one domain each: Wysocki (2009)66 only analysed immunogenicity for a subset of participants and Bryant (2010)23 did not report whether participants or staff delivering the intervention were blinded to the vaccine received. Lack of information was reported in Bryant (2010)23 for the analysis, raising concerns on appropriateness of the analysis for the aggregate data obtained from this study. The remaining 16 studies18,21,24,30,34,37,39,42,48,49,52,57,59,60,64 were judged to have ‘some concerns’ over RoB. These concerns predominantly arose because the randomisation process was not described and/or the study did not report if the participants or staff delivering the vaccines were blinded to which vaccines were given.
Immunogenicity
Figure 2 shows the number of study cohorts included in each analysis and the estimated GMR for each serotype and time point from the NMAs/meta-analyses, and Appendix 5, Tables 5 and 6 summarise the heterogeneity statistics and inconsistency of the networks. Substantial heterogeneity and network inconsistency were present for most serotypes at all three time points.
FIGURE 2.
Geometric mean ratios from meta-analyses at (a) 28 days post-primary vaccination series, (b) pre booster and (c) 28 days post booster. Each line in the figure shows the output from NMAs (PCV7 serotypes) or direct meta-analyses (PCV13 but non-PCV7 serotypes). Blue boxes and blue lines show the point estimates and CIs for GMRs comparing PCV13 versus PCV10. Points to the right of the vertical line are those with higher antibody responses in the PCV13 arm of the study, and points to the left are those with higher antibody responses in the PCV10 arm. The direct evidence column shows the percentage of evidence from studies directly comparing PCV13 versus PCV10 that contributes to the estimates presented in the figure in blue (PCV13 vs. PCV10). GMR of PCV13 versus PCV10 for PCV10 and PCV13 serotypes are from a meta-analysis of only studies which directly compared PCV13 with PCV10.


Geometric mean ratios for comparisons between PCV13 and PCV10 for any primary series schedule were higher for PCV13 for serotypes 4, 7F, 9V and 23F at 1 month after primary vaccination series, with 1.14- to 1.54-fold higher IgG responses with PCV13. Additional serotypes contained only in the PCV13 vaccine (3, 6A and 19A) also favoured PCV13 as expected. GMRs were similar for the remaining serotypes (1, 5, 6B, 14, 18C, 19F; Figure 2A). GMRs favoured PCV7 over either PCV13 or PCV10 for serotypes 4, 6B, 9V, 14 and 23F. There was no difference in GMRs for serotypes 18C and 19F across three vaccines (see Figure 2A).
Direct evidence was available from studies comparing PCV10 and PCV13, and indirect evidence for the difference between PCV13 and PCV10 was provided from studies comparing either PCV10 or PCV13 with PCV7 (see Figure 3). GMRs from direct and indirect comparisons were very similar across time points for most serotypes. However, there were statistical inconsistencies between direct and indirect evidence identified (p-value for inconsistency < 0.05) for serotype 6B, 14, 18C and 19F (see Appendix 5, Table 5).
FIGURE 3.
Direct and indirect evidence on GMRs comparing PCV13 vs. PCV10 at (a) 28 days post-primary vaccination series, (b) pre booster and (c) 28 days post booster. Each line in the figure shows the output from NMAs. Dark grey diamonds and lines show the point estimates and CIs for GMRs from studies directly comparing PCV13 versus PCV10. Light grey diamonds and lines show the point estimates and CIs for GMRs from studies comparing PCV13 versus PCV10 through PCV7. Black boxes and lines show the point estimates and CIs incorporating both direct and indirect evidence.
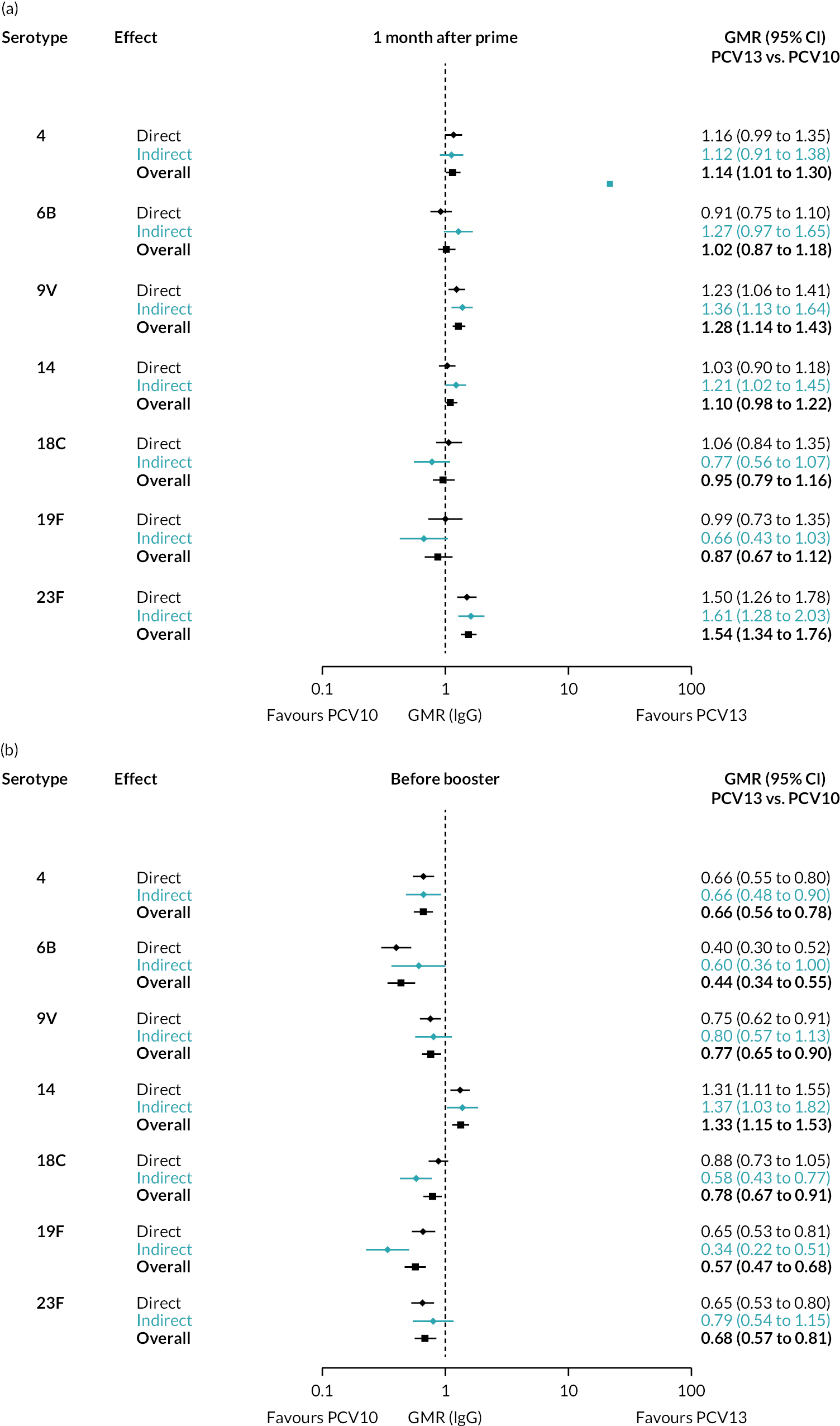
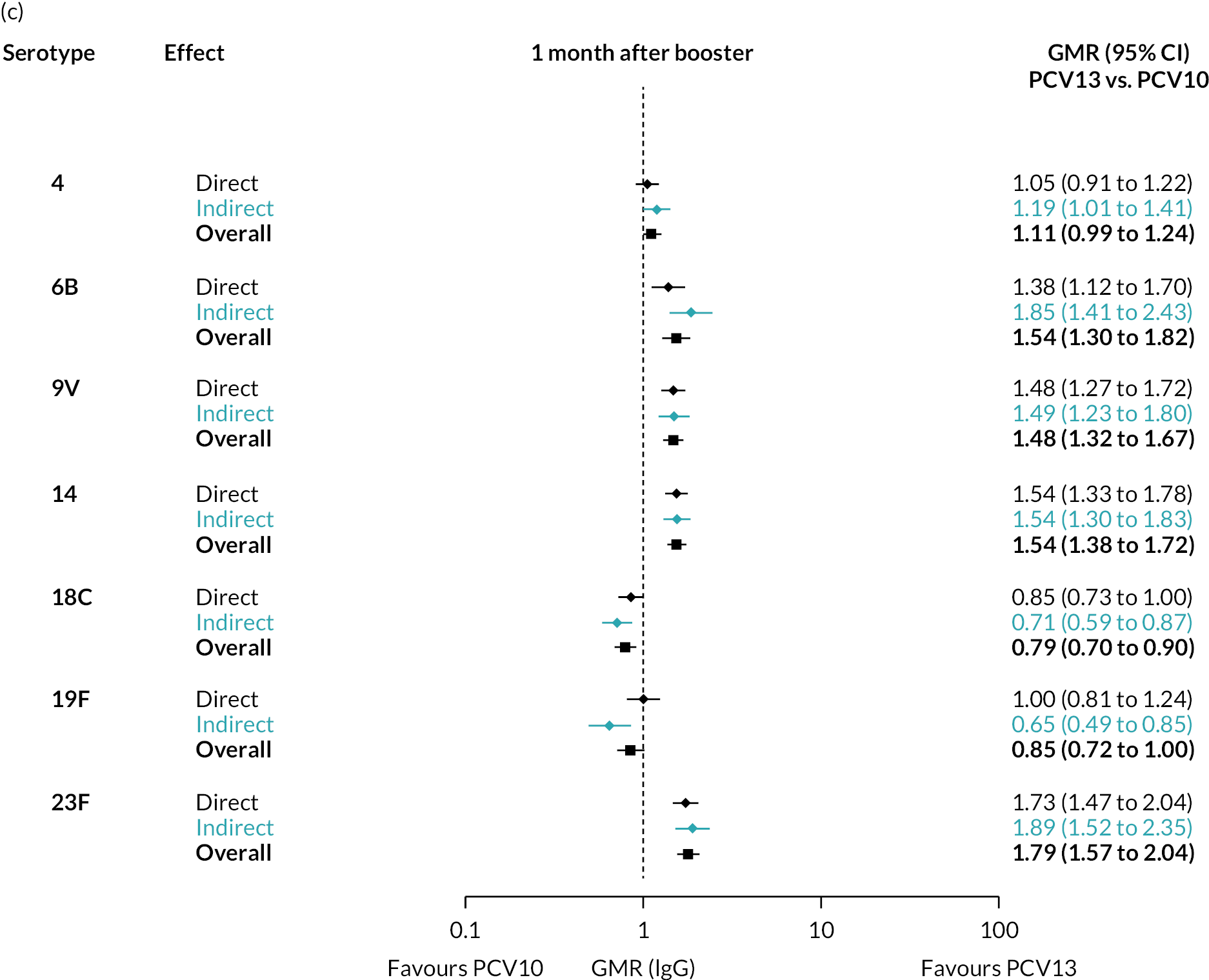
At the pre-booster time point, data were available from 18 cohorts. IgG responses were lower with PCV13 compared with PCV10 for all PCV7 serotypes except for serotype 14, with the point estimates of GMRs comparing PCV13 versus PCV10 ranging from 0.44 to 0.78. IgG responses were higher for PCV13 for serotypes 1, 5 and 7F. GMRs comparing PCV13 versus PCV7 showed higher IgG with PCV7 for serotypes 4, 6B, 9V, 14 and 23F, and higher IgG with PCV13 for serotype 19F (see Figure 2B).
At 28 days post booster, data were available from 26 cohorts. GMRs favoured PCV13 over PCV10 for serotype 6B, 9V, 14 and 23F and favoured PCV10 over PCV13 for serotype 18C (see Figure 2C). For serotype 1, 5 and 7F, antibody responses were higher in PCV13 compared with PCV10. PCV7 recipients had higher GMCs compared with PCV13 for all PCV7 serotypes except 6B, for which there was no difference, and 19F, which favoured PCV13. For PCV13-only serotypes (3, 6A and 19A), GMRs favour PCV13 at all three time points. Inconsistencies were found for serotype 4 and 6B between direct and indirect evidence (see Appendix 5, Table 5 and Figure 3).
To explore potential reasons for the observed heterogeneity, we summarised cohort-level GMRs and RRs for each vaccine comparison and present these with concomitant vaccines and vaccine schedules at all three time points in Appendix 5, Figures 21–59 (GMRs) and Figures 60–69 (RRs). These descriptive analyses revealed a lack of consistency in the direction of study-level estimates within each vaccine comparison, resulting in the significant heterogenicity. There was also no observable pattern in any trial-level variable (region, co-administered vaccines, vaccine schedule), from which one might propose a mechanism that would adequately explain this variation in GMRs, although studies which compared vaccines with the same carrier protein seemed to have more consistent estimates. In sensitivity analysis, we restricted to 11 cohorts providing IgG results for all the three time points and observed similar results (see Appendix 6, Figure 70). Additional sensitivity analyses stratified by region and vaccine schedule demonstrated reduced heterogeneity for some serotypes and similar patterns compared with main analysis (see Appendix 6, Figures 71–73). Sensitivity analyses excluding the two studies having overall ‘high risk of bias’ did not provide different results. Excluding the one study comparing PCV13 and PCV10 with a 1 + 1 schedule did not affect the results.
Seroefficacy
There were 12 studies (15 cohorts) with available individual participant antibody data at both post-primary and prior to the booster dose, allowing serotype-specific estimation of seroefficacy from a total of 5152 participants. Of these 15 cohorts, 6 compared PCV10 versus PCV7, 3 compared PCV13 versus PCV7 and 6 compared PCV13 versus PCV10 (see Figure 1).
The RR of seroinfection from the NMA for each serotype is summarised in Figure 4, and a summary of direct and indirect evidence is given in Figure 5. The I2 and p-value indicate some heterogeneity for all PCV7 serotypes except for serotype 4 and 19F (see Appendix 5, Table 6).
FIGURE 4.
Meta-analyses of the RR of seroinfection. Each line in the figure shows the output from NMAs (PCV7 serotypes) or direct meta-analyses (PCV10 serotypes). Blue boxes and blue lines show the point estimates and CIs of RR of seroinfection comparing PCV13 versus PCV10. The direct evidence column shows the percentage of evidence from studies directly comparing PCV13 versus PCV10. Results for PCV10 serotypes are from a meta-analysis of only studies comparing PCV13 with PCV10; therefore, estimates of PCV7 versus PCV10 and PCV13 versus PCV7 were not available.

FIGURE 5.
Direct and indirect estimates of the RR of seroinfection. Each line in the figure shows the output from NMAs. Dark grey diamonds and lines show the point estimates and CIs RRs from studies directly comparing PCV13 versus PCV10. Light grey diamonds and lines show the point estimates and CIs for RRs from studies comparing PCV13 versus PCV10 through PCV7. Black boxes and lines show the point estimates and CIs incorporating both direct and indirect evidence.

Among PCV7 serotypes, the risk of seroinfection was lower with PCV13 than PCV10 for serotypes 4, 6B, 9V, 18C and 23F, while no difference was seen for serotype 14 and 19F (see Figure 4). The RRs of seroinfection (PCV13 vs. PCV10) for PCV7 serotypes ranged from 0.32 (95% CI 0.19 to 0.52) for serotype 4 to 1.28 (95% CI 0.95 to 1.74) for serotype 14. The direct evidence contributed to around 80–95% of total evidence, and we found no inconsistency between direct and indirect evidence for all but serotype 19F (p > 0.05; see Appendix 5, Table 6 and Figures 60–69).
For serotypes 1, 5 and 7F, evidence was summarised from six studies directly comparing PCV13 with PCV10. Heterogeneity was observed for serotype 5, and all CIs overlapped 1.0. Comparisons between PCV13 and PCV7 favoured neither vaccine over the other, whereas comparisons between PCV7 and PCV10 favoured PCV7 for serotypes 5, 6B, 9V, 18C and 23F.
Sensitivity analyses of studies conducted in Europe and, using 3 + 1 schedule, showed similar RRs as estimated from the main analysis (see Appendix 6, Figures 74 and 75). The seroefficacy analysis results remained consistent after removing one ‘high risk of bias’ study from the analysis.
Association between ratios of immunogenicity and seroefficacy
Figure 6 shows the serotype-specific relationships between trial-level immunogenicity (GMRs) and seroefficacy (RRs). Log-GMRs and log-RRs were highly or moderately correlated for all PCV7 serotypes [with weighted Pearson’s correlation coefficients (r) ranging from –0.76 to –0.60, all p < 0.05] except for serotype 14 (r = –0.30, p = 0.26).
FIGURE 6.
Association between study-level GMRs and RRs of seroinfection. Each point shows results of a serotype-specific comparison between two vaccines from one study. Solid line shows the relationship between RR predicted from the crude model and GMR. Dashed line shows the CIs of predicted RR. Reference lines show GMR equivalent to one (vertical) and RR equivalent to one (horizontal) which represent values associated with no difference between vaccines. Points sizes represent sample size of the trial. Each panel shows one PCV7 serotype (4, 6B, 9V, 14, 18C, 19F and 23F).

In the combined analysis across all serotypes, vaccines that produced the same amount of antibody (GMR = 1) had very similar protection (adjusted RR 0.80, 95% CI 0.41 to 1.58; see Figure 7). The model estimate indicates that for each twofold increase in antibody response, the risk of seroinfection was halved (GMR of 2.0; RR 0.46, 95% CI 0.23 to 0.96; see Figure 7A and B). The estimates were stable when estimates of PCV13 versus PCV7 were analysed in reverse as PCV7 versus PCV13 (GMR of 2.0; RR 0.51, 95% CI 0.23 to 1.15; see Figure 7C).
FIGURE 7.
Overall association between trial-level GMRs and RRs across all serotypes included in PCV10. Each point shows results of a serotype-specific comparison between two vaccines from one study. Solid line shows the relationship between RR predicted from the model and GMR. Dashed line shows the CIs of predicted RR. Reference lines show GMR equivalent to one (vertical) and RR equivalent to one (horizontal) which represent values associated with no difference between vaccines. Points sizes represent sample size of the trial. Panel (a) shows the relationship by 13 serotypes covered by PCV13; (b) shows the same data as panel a classified by vaccine comparison groups; (c) shows the same data as panel B; however, studies comparing PCV13 versus PCV7 are analysed and displayed as PCV7 versus PCV13 as a sensitivity analysis; and (d) shows a further sensitivity analysis that excludes studies of PCV13 versus PCV7 and only shows studies that compared vaccines from two different manufacturers.

When analyses were restricted to comparison between products from different manufacturers, the relationship between immunogenicity and seroprotection remained similar to the main analysis with a CI that incorporates 1.0 (GMR 1.0; RR 0.73, 95% CI 0.36 to 1.47; see Figure 7D).
Chapter 4 Mathematical modelling of differential impact of PCV10 and PCV13 on invasive pneumococcal diseases
To illustrate the use of serotype-specific estimates of seroefficacy in modelling vaccine impact and cost-effectiveness, we developed a mathematical model of pneumococcal transmission dynamics to compare the differential impact of PCV10 and PCV13 introduction on invasive pneumococcal disease cases with vaccine serotypes in England and Wales. The model estimated the impact over a 25-year time period from 2005–6 to 2029–30.
Model structure and inputs
The mathematical model is a compartmental, deterministic, serotype-specific and realistic age structure model to describe the pneumococcal transmission dynamics with two PCV programmes. The model is a susceptible–infectious–susceptible model which assumes no natural immunity. When susceptibles acquire carriage infection, they become infectious for the duration of carriage. The duration of carriage for all serotypes is assumed to be age dependent, and the values were obtained from Melegaro et al. 94 After the duration of carriage, infectious people become susceptible again. For simplicity, invasive pneumococcal disease was assumed to occur at the time of carriage infection.
Serotypes
The systematic review described in Chapters 1–3 provided estimates of the RR of seroinfection by the time of the booster dose which is interpreted as the relative reduction in vaccine efficacy of PCV10 against carriage acquisition compared with PCV13. The systematic review found no significant difference in RRs for serotypes 1, 5, 7F, 15 and 19F among 10 serotypes common in PCV10 and PCV13. Hence, we have only included five serotypes (18C, 23F, 4, 6B and 9V) for this modelling study. For each serotype, 100 random draws were taken from the CI around the estimates of the RR of seroinfection from Chapter 3, assuming a normal distribution around the log-RR (see Figure 4).
Carriage
Data for the pre-PCV equilibrium were obtained from carriage prevalence estimates from the 10-month longitudinal nasopharyngeal swab study with 3869 swabs conducted in 2001–2 in England. 95 Due to the detailed stratification for age groups and serotypes needed, there were some categories in the swabbing study with no positive samples. For simplicity, we substituted 0.5 positive sample in these groups. The carriage prevalence for the five serotypes is presented in Figure 8.
FIGURE 8.
Carriage prevalence of five serotypes (18C, 23F, 4, 6B and 9V) in seven age groups (data from Hussain et al. 95).

Invasive disease cases
Data on the invasive pneumococcal disease cases for the five serotypes and 16 age groups were obtained for England and Wales in 2005–6 (see Figure 9). Similar to the carriage data, there were some groups with no positive samples, and for these groups, one positive sample was substituted to enable calculation of incidence.
FIGURE 9.
Invasive pneumococcal disease cases for five serotypes (18C, 23F, 4, 6B and 9V) and 16 age groups in 2005–6 in England and Wales. M, months; Y, years.
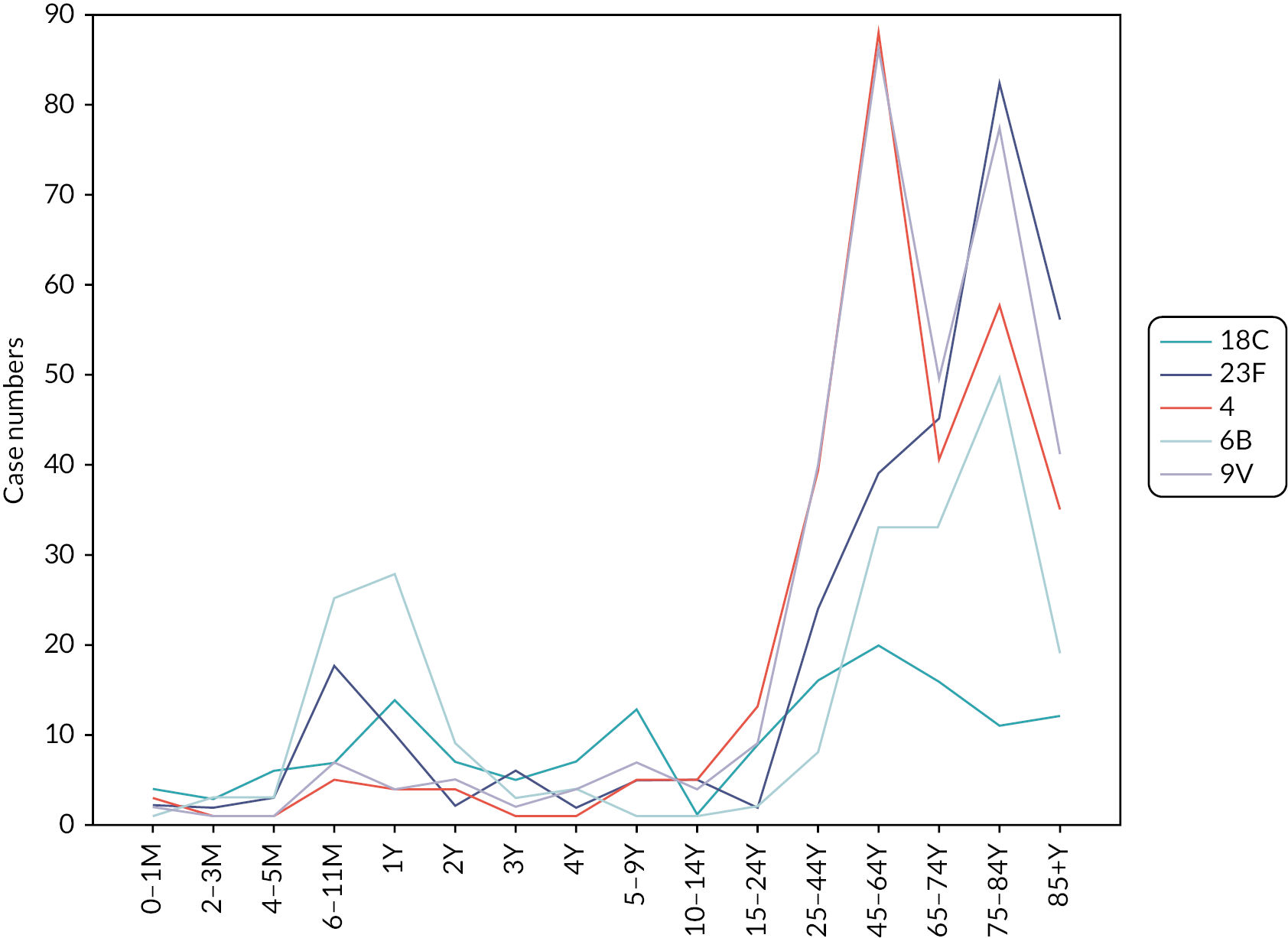
Contact patterns
The contact patterns for the seven age groups for the forces of infection were obtained by combining the GB POLYMOD close contact matrix,96 and additional data on under-1-year-olds. 97
Parameter estimations
Using a static model, we fitted the force of infection by age groups for each target serotype (see Figure 10) using the carriage prevalence in Figure 8. Upon these forces of infection values, we calculate the transmission probabilities per contact in seven age groups (see Figure 11) using the 2005 England and Wales population and the contact pattern matrix.
FIGURE 10.
Force of pneumococcal infection for seven age groups and five serotypes in 2005–6 obtained using the pre-PCV carriage prevalence and individual patient data cases in 2005–6 in England and Wales. 98
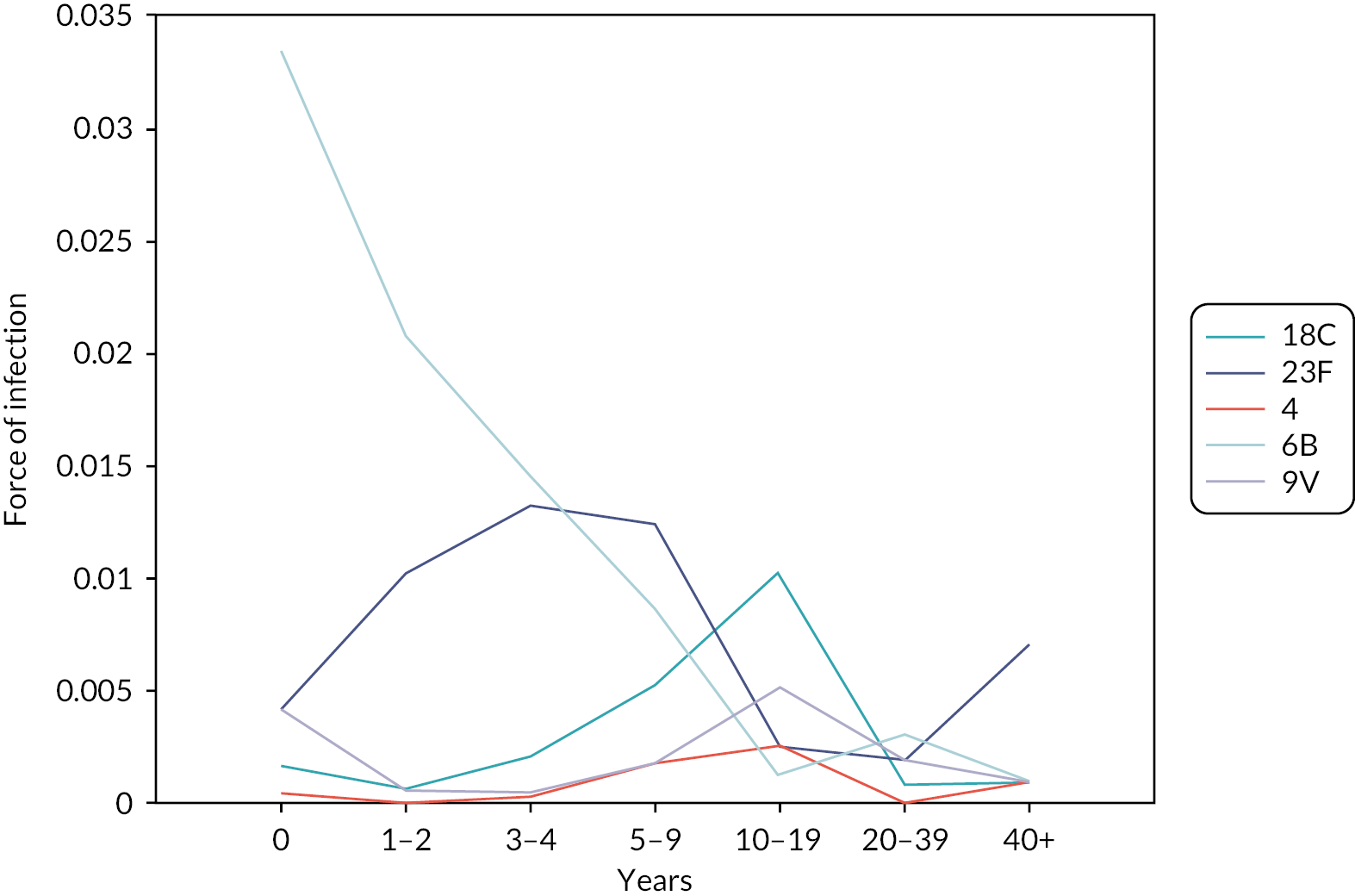
FIGURE 11.
Pneumococcal transmission probabilities per contact for seven age groups and five serotypes in 2005–6 obtained using the pre-PCV carriage prevalence and individual patient data cases in 2005–6 in England and Wales. 98

We calculated case : carrier ratios (CCRs) (see Figure 12) by age groups for each serotype by dividing the number of invasive disease cases for the corresponding age group and serotype with the number of new infections during the baseline year. 98 As a sensitivity analysis, we tested an alternative assumption and used the number of infectious people instead of new infections to calculate the CCRs. Both assumptions produced almost identical results.
FIGURE 12.
Case : carrier ratios by 16 age groups for five pneumococcal serotypes using the pre-PCV carriage prevalence and individual patient data cases in 2005–6 in England and Wales. M, months; Y, years. 98
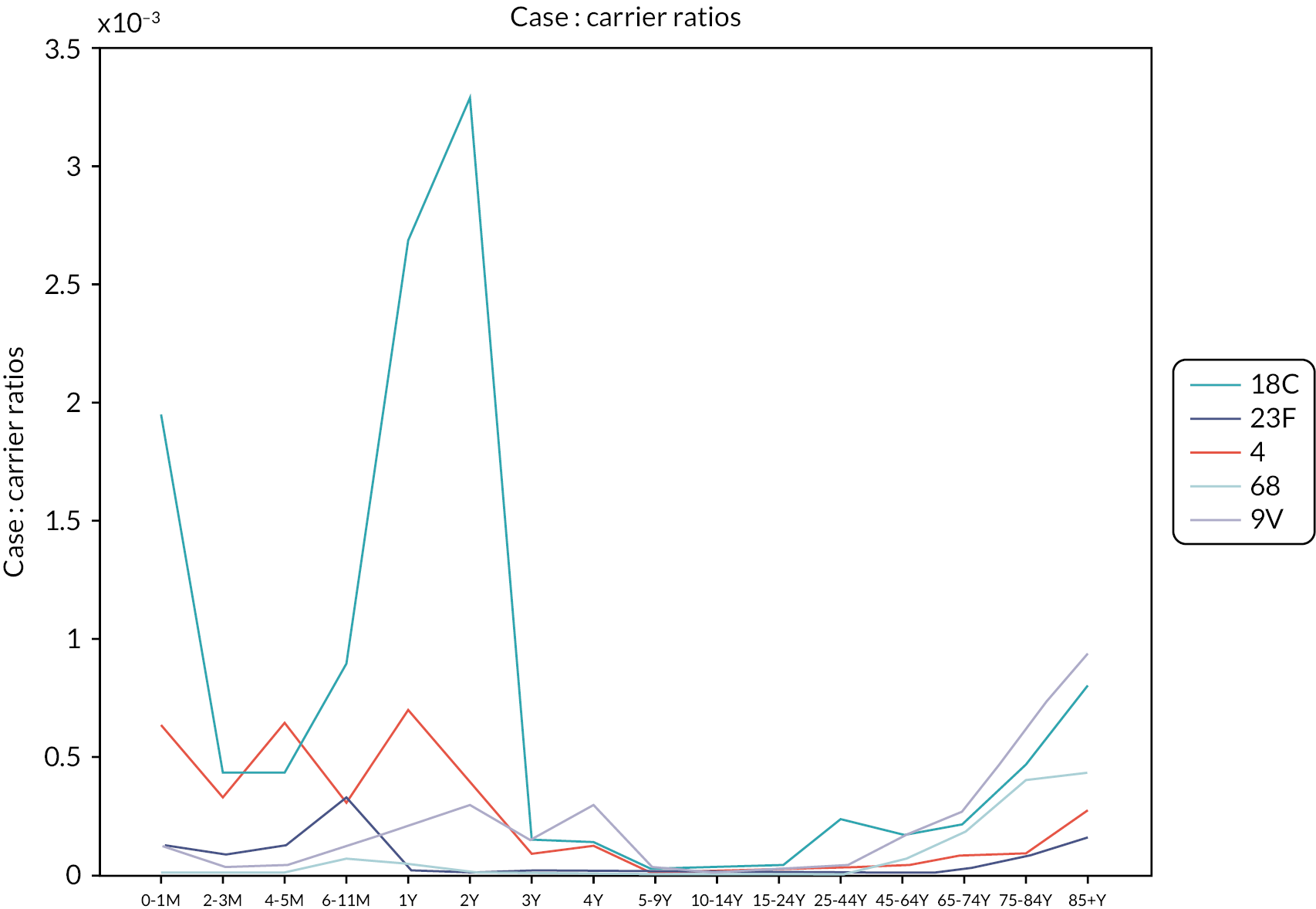
Population
We used the England and Wales population data from 2006/7 until 2030/1 for the long-term impact of two PCV programmes for 25 years from 2006 to 2007 (see Figure 13).
FIGURE 13.
Annual population sizes by age groups between 2005 and 2030 in England and Wales (projections obtained from the Office of National Statistics). Y, years.
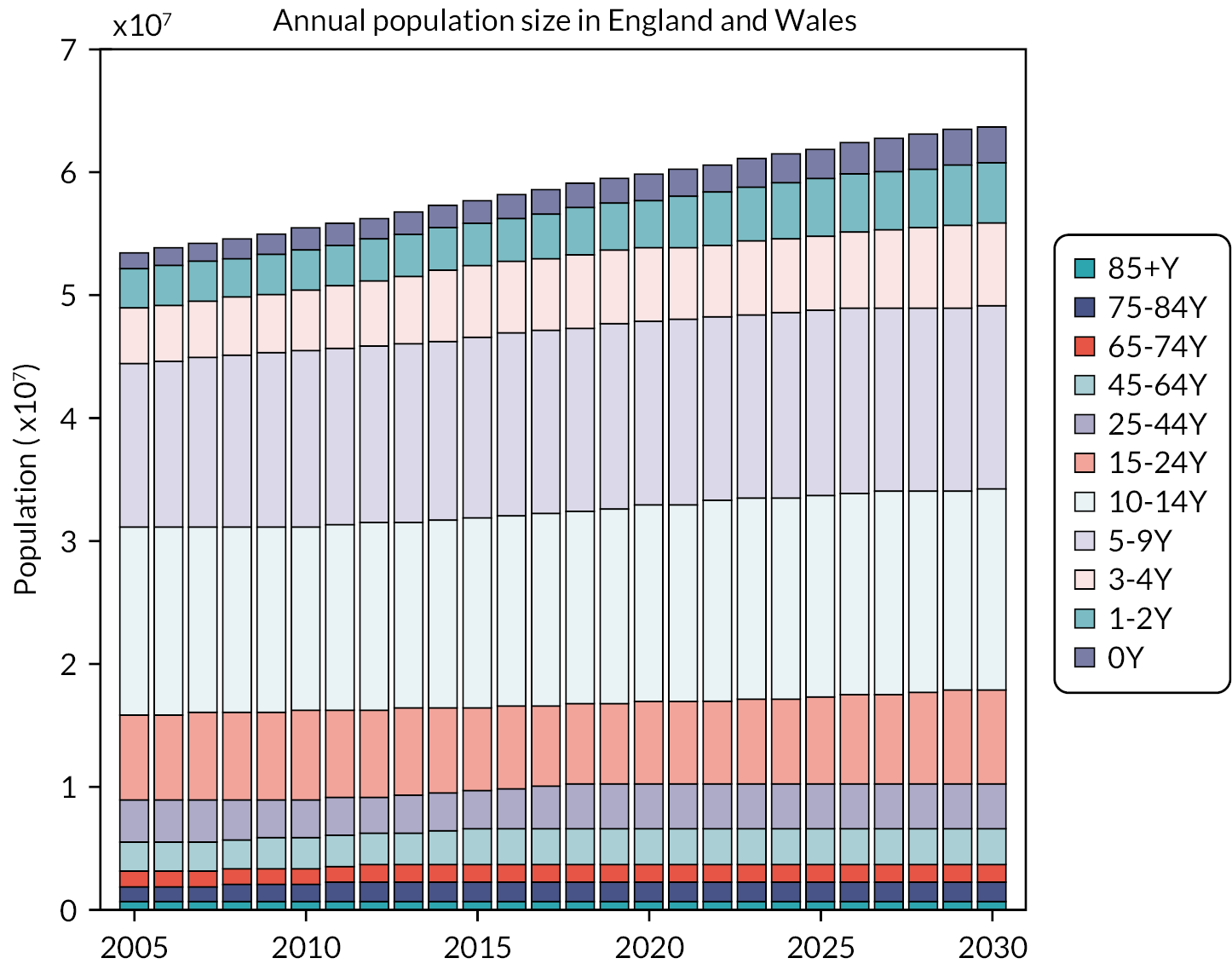
Baseline projections for invasive pneumococcal disease cases without PCV programmes would be increased due to these increasing population size in Figure 13.
Vaccination programme
The PCV programme schedule was assumed to be introduced in the beginning of 2006–7 (first week of June 2006) with 2 + 1 at 2, 4 and 12 months of age in the model. The coverage for the first dose was fixed at 90%, 95% for the second dose among the first-dosed infants and 95% of the second-dosed infants for third-dose coverage. There was no catch-up programme assumed in the model. The vaccine efficacy against carriage for PCV13 was assumed to be 55% for the fully vaccinated compartments, and half this value for those partially protected. The duration of partial and full protection is assumed to be 5 years. 98 Those losing partial or full protection move to waned and partially protected compartments of the model, respectively. The average duration of full vaccine protection is assumed to be 10 years. Those in the waned and partially protected compartments move to fully protected after receiving their booster dose. The flow diagram in Figure 14 describes the movement between compartments due to vaccine doses and waning vaccine protection.
FIGURE 14.
Flow diagram showing how vaccination status of individuals is tracked in the model, based on the number of doses received and waning of vaccine protection.

Results
The model showed that in the absence of any vaccine programme, an increase in invasive pneumococcal disease cases caused by all five serotypes would be seen over the 25-year time frame (Figure 15). With the introduction of either PCV13 or PCV10 vaccine programmes in 2006, case counts decreased, achieving near eradication of all serotypes within the time frame modelled. The decrease in cases was most rapid for serotype 6B and least rapid for serotype 4. The decrease in cases was less rapid for PCV10 than for PCV13.
FIGURE 15.
Modelled long-term yearly number of invasive pneumococcal disease cases in England and Wales after introduction of PCV10, or PCV13 in 2006, compared with no PCV programme.
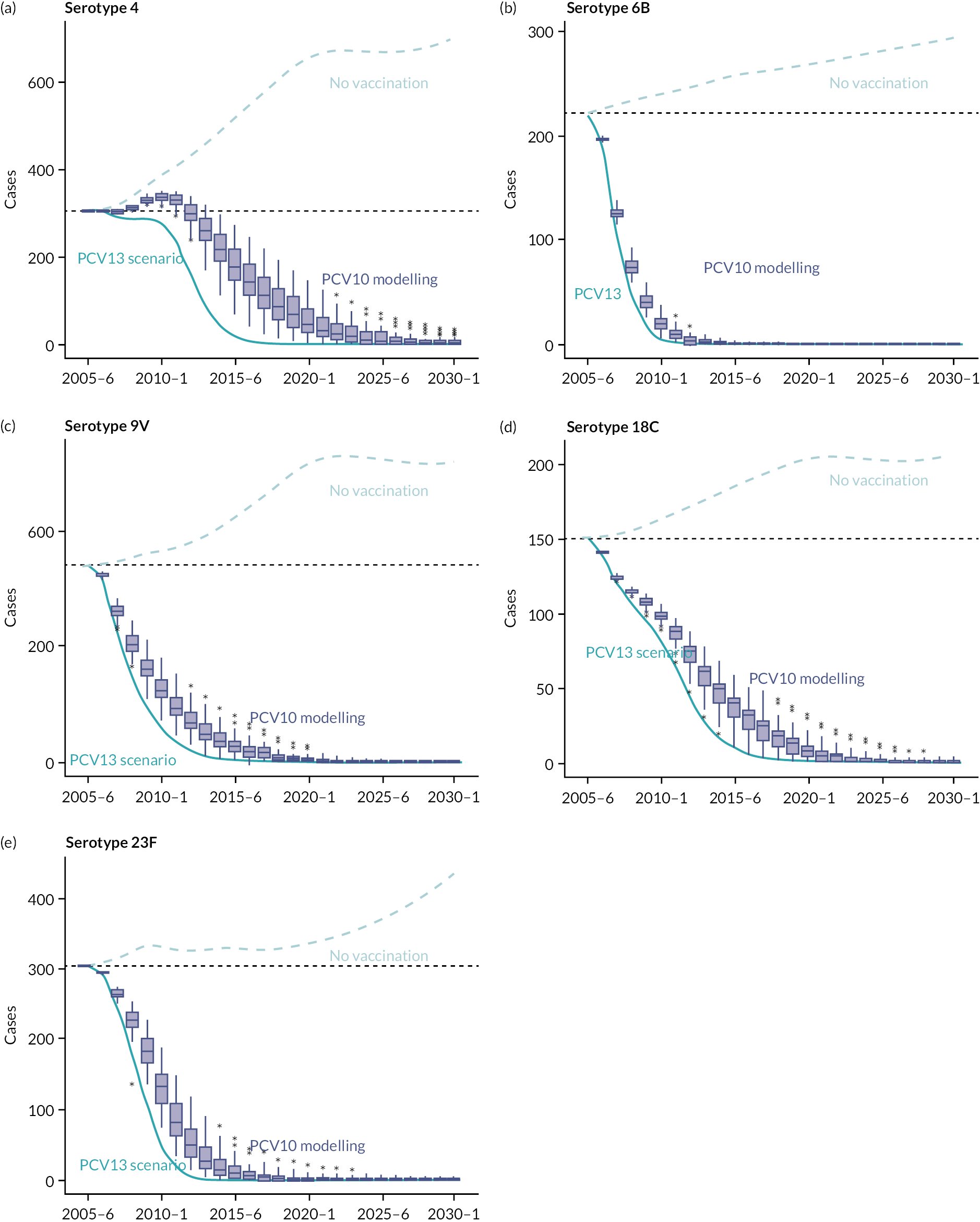
The PCV programme is assumed to be introduced in the beginning of June 2006 in the 2006–7 epidemiological year. a–e present results for five serotypes (4, 6B, 9V, 18C and 23F). Box plots show the modelled long-term impact of PCV10 on invasive pneumococcal disease cases. The dotted blue line shows the trajectory of invasive pneumococcal disease cases in the absence of any PCV programme (general increase reflects increasing population over years and age-dependent CCR by serotypes). The dark blue line shows the long-term impact of PCV13.
Chapter 5 Retrospective economic evaluation of vaccinating infants with PCV13 compared to vaccination with PCV10 to prevent pneumococcal disease in England and Wales
Aims
We aimed to assess the cost-effectiveness of introducing infant vaccination with PCV13 compared to introducing PCV10 from a healthcare payer perspective in England and Wales. More specifically, to retrospectively estimate the additional threshold price per dose below which PCV13 would be more cost-effective than PCV10 had they both been available at the time of introduction of the PCV vaccine programme in England and Wales in 2006.
While these vaccines were not actually available in 2006, the eventual switch in England and Wales from PCV7 to PCV13 in 2010 was informed by a transmission dynamic model99 combined with a health economic evaluation. 100 For those analyses, the assumption was made that PCV7 and PCV13 each fully protected against the serotypes in the vaccine, while PCV10 was not evaluated. The completion of the NMA (see Chapters 1–3) allows a retrospective comparison of PCV10 and PCV13 using serotype-specific efficacy results for the first time.
Methods
A previously published health economic model100 of the costs and health outcomes associated with invasive pneumococcal disease was combined with estimates of the differential number of age-specific cases of invasive pneumococcal disease following introduction of PCV10 versus PCV13 vaccination from the dynamic transmission model described in Chapter 4. Differences in the impact of the two vaccines on the number of cases of invasive pneumococcal disease were based on the serotype-specific RR of seroinfection with PCV13 versus PCV10 from Chapter 3 (see Figure 4).
Vaccination programme
The impact of a 2 + 1 infant vaccination programme (with doses given at 2, 4 and 12 months of age) was modelled from introduction in 2006 over a 25-year time horizon, by which time, the impact of PCV10 and PCV13 programmes is predicted to have reached a steady state with almost complete eradication of these serotypes (see Figure 15). The number of infants eligible for vaccination each year was based on the annual (historical and projected) population of children in England and Wales aged under 1 year of age. 101,102 The coverage of the first dose was assumed to be 90%, followed by 95% uptake among those eligible for the second and third doses.
Disease outcomes
The risks of different invasive pneumococcal disease presentations for each case were based on the age-specific distributions presented graphically in the supplementary material of van Hoek et al. 100 To incorporate these distributions into the current analysis, the figures were digitised using the R package metaDigitise and the data were fitted to either a beta distribution (for probabilities) or gamma distributions (length of stay or non-invasive disease outcome multipliers) using the R package fitDistrPlus. Fitted distributions are summarised in Table 1.
Parameter description | Age group | Parameter distribution |
---|---|---|
Invasive disease parameters | ||
Probability of meningitis | Under 2 years | Beta (mean = 0.44, SD = 0.017) |
2–14 years | Beta (mean = 0.28, SD = 0.022) | |
15–64 years | Beta (mean = 0.15, SD = 0.0077) | |
65–74 years | Beta (mean = 0.098, SD = 0.01) | |
Over 75 years | Beta (mean = 0.048, SD = 0.0047) | |
Probability of pneumonia | Under 2 years | Beta (mean = 0.31, SD = 0.017) |
2–14 years | Beta (mean = 0.44, SD = 0.024) | |
15–64 years | Beta (mean = 0.61, SD = 0.01) | |
65–74 years | Beta (mean = 0.64, SD = 0.016) | |
Over 75 years | Beta (mean = 0.69, SD = 0.011) | |
Probability of empyema | Under 2 years | Beta (mean = 0.028, SD = 0.0063) |
2–14 years | Beta (mean = 0.05, SD = 0.0097) | |
15–64 years | Beta (mean = 0.024, SD = 0.0034) | |
65–74 years | Beta (mean = 0.018, SD = 0.0045) | |
Over 75 years | Beta (mean = 0.0093, SD = 0.0021) | |
Probability of sepsis | Under 2 years | Beta (mean = 0.15, SD = 0.013) |
2–14 years | Beta (mean = 0.15, SD = 0.017) | |
15–64 years | Beta (mean = 0.19, SD = 0.0086) | |
65–74 years | Beta (mean = 0.22, SD = 0.014) | |
Over 75 years | Beta (mean = 0.23, SD = 0.0096) | |
Probability of other foci | Under 2 years | Beta (mean = 0.07, SD = 0.0092) |
2–14 years | Beta (mean = 0.077, SD = 0.012) | |
15–64 years | Beta (mean = 0.032, SD = 0.0039) | |
65–74 years | Beta (mean = 0.03, SD = 0.0061) | |
Over 75 years | Beta (mean = 0.015, SD = 0.0027) | |
Case fatality risk for meningitis | Under 2 years | Beta (mean = 0.048, SD = 0.0078) |
2–14 years | Beta (mean = 0.068, SD = 0.015) | |
15–64 years | Beta (mean = 0.11, SD = 0.011) | |
65–74 years | Beta (mean = 0.17, SD = 0.024) | |
Over 75 years | Beta (mean = 0.33, SD = 0.031) | |
Case fatality risk for pneumonia | Under 2 years | Beta (mean = 0.0053, SD = 0.0036) |
2–14 years | Beta (mean = 0.0083, SD = 0.0037) | |
15–64 years | Beta (mean = 0.051, SD = 0.0034) | |
65–74 years | Beta (mean = 0.13, SD = 0.0086) | |
Over 75 years | Beta (mean = 0.32, SD = 0.012) | |
Case fatality risk for empyema | Under 2 years | Beta (mean = 9.5e-08, SD = 3.6e-08) |
2–14 years | Beta (mean = 1.1e–07, SD = 5.8e-08) | |
15–64 years | Beta (mean = 0.027, SD = 0.014) | |
65–74 years | Beta (mean = 0.077, SD = 0.04) | |
Over 75 years | Beta (mean = 0.22, SD = 0.069) | |
Case fatality risk for sepsis | Under 2 years | Beta (mean = 0.041, SD = 0.012) |
2–14 years | Beta (mean = 0.032, SD = 0.013) | |
15–64 years | Beta (mean = 0.15, SD = 0.012) | |
65–74 years | Beta (mean = 0.24, SD = 0.018) | |
Over 75 years | Beta (mean = 0.41, SD = 0.018) | |
Case fatality risk for other foci | Under 2 years | Beta (mean = 0.011, SD = 0.0087) |
2–14 years | Beta (mean = 0.012, SD = 0.011) | |
15–64 years | Beta (mean = 0.12, SD = 0.027) | |
65–74 years | Beta (mean = 0.14, SD = 0.039) | |
Over 75 years | Beta (mean = 0.15, SD = 0.038) | |
Length of stay (days) for meningitis | Under 2 years | Gamma (mean = 12, SD = 0.58) |
2–14 years | Gamma (mean = 11, SD = 1.7) | |
15–64 years | Gamma (mean = 26, SD = 2.4) | |
65–74 years | Gamma (mean = 29, SD = 5) | |
Over 75 years | Gamma (mean = 23, SD = 2.8) | |
Length of stay (days) for pneumonia | Under 2 years | Gamma (mean = 5, SD = 0.48) |
2–14 years | Gamma (mean = 4.8, SD = 0.56) | |
15–64 years | Gamma (mean = 10, SD = 0.43) | |
65–74 years | Gamma (mean = 15, SD = 0.82) | |
Over 75 years | Gamma (mean = 18, SD = 0.68) | |
Length of stay (days) for empyema | Under 2 years | Gamma (mean = 17, SD = 2.7) |
2–14 years | Gamma (mean = 16, SD = 1.6) | |
15–64 years | Gamma (mean = 32, SD = 6.1) | |
65–74 years | Gamma (mean = 24, SD = 4.7) | |
Over 75 years | Gamma (mean = 39, SD = 8.6) | |
Length of stay (days) for sepsis | Under 2 years | Gamma (mean = 9.9, SD = 2.9) |
2–14 years | Gamma (mean = 7.4, SD = 1.1) | |
15–64 years | Gamma (mean = 17, SD = 1.5) | |
65–74 years | Gamma (mean = 15, SD = 1.3) | |
Over 75 years | Gamma (mean = 20, SD = 1.3) | |
Length of stay (days) for other foci | Under 2 years | Gamma (mean = 6.5, SD = 1.2) |
2–14 years | Gamma (mean = 5.2, SD = 0.92) | |
15–64 years | Gamma (mean = 17, SD = 3.1) | |
65–74 years | Gamma (mean = 13, SD = 2.1) | |
Over 75 years | Gamma (mean = 33, SD = 6.4) | |
Non-invasive disease multipliers | ||
Cases of non-invasive pneumonia (GP) per case of invasive pneumococcal disease | Under 1 year | Gamma (mean = 0.88, SD = 0.32) |
1 year | Gamma (mean = 2.3, SD = 0.85) | |
2–4 years | Gamma (mean = 3.6, SD = 1.4) | |
5–9 years | Gamma (mean = 6.2, SD = 2.3) | |
Over 10 years | Gamma (mean = 4.6, SD = 1.7) | |
Cases of non-invasive pneumonia (hospital) per case of invasive pneumococcal disease | Under 1 year | Gamma (mean = 1.1, SD = 0.41) |
1 year | Gamma (mean = 2.9, SD = 1) | |
2–4 years | Gamma (mean = 4.1, SD = 1.5) | |
5–9 years | Gamma (mean = 2.3, SD = 0.86) | |
Over 10 years | Gamma (mean = 6.7, SD = 2.5) |
Hospitalised cases are stratified according to disease focus (meningitis, bacteraemia, empyema, pneumonia or other), which are associated with a corresponding hospital length-of-stay distribution and mortality risk. For meningitis survivors, the risk of developing different long-term sequelae was based on the results of a systematic review of sequelae due to pneumococcal meningitis in high-income countries. 103
In a scenario analysis, the difference in impact of PCV10 versus PCV13 on non-invasive pneumonia was also included, assuming this is proportional to the impact on invasive pneumococcal disease for each age group. The number of cases of invasive pneumococcal disease averted was multiplied by age-specific factors estimated by van Hoek and co-workers to calculate the number of cases of non-invasive pneumonia due to S. pneumoniae.
Quality-adjusted life-years
For deaths following invasive pneumococcal disease, we estimated the quality-adjusted life-year (QALY) loss as the average discounted remaining life expectancy for each age group in the model. The discounted life expectancy for each age group was calculated using the life table method and weighted according to the size of the population for each year of age with each age group. UK life tables for each year were based on medium scenario of the 2022 update of the United Nations World Population Prospects estimates,104 which account for expected changes in underlying population mortality over the period 2006–30.
For non-fatal outcomes, the QALY loss for different outcomes of the acute episode was based on the QALY loss used by van Hoek and co-workers. For meningitis sequelae, a reduced health state utility was assumed to apply for each year of remaining life based on the values reported by Oostenbrink et al. 105 Conservatively, it was assumed that meningitis survivors with sequelae did not experience any reduction in life expectancy.
In the base-case analysis, QALYs were discounted to the year 2006 at an annual discount rate of 3.5%. As a sensitivity analysis, an annual discount rate of 1.5% was also used.
Costs
Healthcare costs were parameterised using distributions reported by van Hoek et al. 100 after adjustment to 2006 prices (see Table 2). This adjustment was performed using the Hospital and Community Health Services Index. 106 All costs were discounted annually at 3.5%.
Parameter description | Mean value | Parameter distribution |
---|---|---|
Healthcare costs (£2010) a | ||
Primary care consultation | 35 | Fixed |
Prescription (pneumonia) | 10.74 | Fixed |
Prescription (sepsis or meningitis) | 2.14 | Fixed |
Hospital bed-day (sepsis) | 308 | Log-normal (mean = 5.73, SD = 0.40) |
Hospital bed-day (meningitis) | 261 | Log-normal (mean = 5.56, SD = 0.29) |
Hospital bed-day (pneumonia) | 270 | Log-normal (mean = 5.60, SD = 0.26) |
Outpatient follow-up (meningitis) | 346 | Log-normal (mean = 5.85, SD = 0.44) |
Annual cost of meningitis sequelae (first year) | 6080 | Log-normal (mean = 8.71, SD = 0.36) |
Annual cost of meningitis sequelae (subsequent year) | 185 | Log-normal (mean = 5.22, SD = 0.44) |
QALY loss per case | ||
Hospitalisation (sepsis or pneumonia) | 0.079 | Beta (mean = 0.079, SD = 0.0031) |
Hospitalisation (meningitis) | 0.023 | Beta (mean = 0.023, SD = 0.0083) |
Pneumonia (outpatient visit) | 0.0037 | Beta (mean = 0.037, SD = 0.0012) |
Health state utility of meningitis sequelae | ||
Deafness | 0.81 | Beta (mean = 0.81, SD = 0.0028) |
Mild hearing loss | 0.91 | Beta (mean = 0.91, SD = 0.0015) |
Epilepsy | 0.83 | Beta (mean = 0.83, SD = 0.0015) |
Mild mental retardation | 0.62 | Beta (mean = 0.62, SD = 0.0021) |
Paresis | 0.67 | Beta (mean = 0.67, SE = 0.0023) |
For hospitalised cases, the cost per case was calculated by multiplying the length of stay by the corresponding cost per bed-day based on NHS reference costs. Cases were assumed to incur the cost of one primary care consultation along with the cost of an antibiotic prescription prior to hospitalisation. Survivors of meningitis with no long-term sequelae were assumed to incur the cost of one follow-up outpatient appointment, whereas the annual healthcare costs for those with meningitis sequelae were based on previous estimates from Melegaro and Edmunds. 107
For non-invasive disease costs, it was assumed that 1% of otitis media cases would require an outpatient appointment. For non-invasive pneumonia resulting in hospitalisation, in the absence of length-of-stay data, the cost was based on the reference cost for an admission.
The delivery costs for each dose of vaccine were assumed to be the same for PCV10 and PCV13 and, therefore, would not impact the threshold vaccine price.
Threshold price analysis
To estimate the additional price per dose below which PCV13 becomes more cost-effective than PCV10, the additional QALY gain with PCV13 was monetised by valuing each QALY according to different cost-effectiveness threshold (CET) values. This was then used to calculate the incremental net monetary benefit (iNMB) combining the value of the QALY gain with the healthcare savings minus any extra costs of the PCV13 vaccine programme. The vaccine threshold price is then the additional cost per dose at which the iNMB equals zero.
In the base case, the additional threshold price was calculated using a CET of £20,000 per QALY, and a CET of £30,000 per QALY was used as a sensitivity analysis.
Results
The introduction of an infant PCV13 programme was predicted to avoid an additional 2808 (95% CI 2690 to 2925) cases of invasive pneumococcal disease compared with PCV10 introduction between 2006 and 2030. This includes an estimated 326 cases of meningitis, 578 cases of sepsis, 1770 cases of invasive pneumonia and 30,680 cases of non-invasive pneumonia. Under base-case assumptions, this resulted in discounted healthcare savings of £13 million (95% CI £12 to £14 million). Including non-invasive pneumonia increased the savings to £27 million (95% CI £25 to £29 million). A breakdown of healthcare cost savings by disease presentation is shown in Figure 16.
FIGURE 16.
Discounted healthcare costs averted (£2006) by an infant PCV13 introduction compared with a PCV10 programme between 2006 and 2030, stratified by disease presentation.

In the base case, averting additional cases of invasive pneumococcal disease resulted in a gain of an additional 4545 (95% CI 4465 to 4724) discounted QALYs, which increased to a gain of 6625 (95% CI 6352 to 6899) QALYs when using a discount rate of 1.5%. Again, inclusion of non-invasive disease had only a small impact (see Figure 17).
FIGURE 17.
Discounted QALYs gained by an infant PCV13 programme introduction compared to PCV10 introduction between 2006 and 2030 stratified by disease presentation under different scenario assumptions.
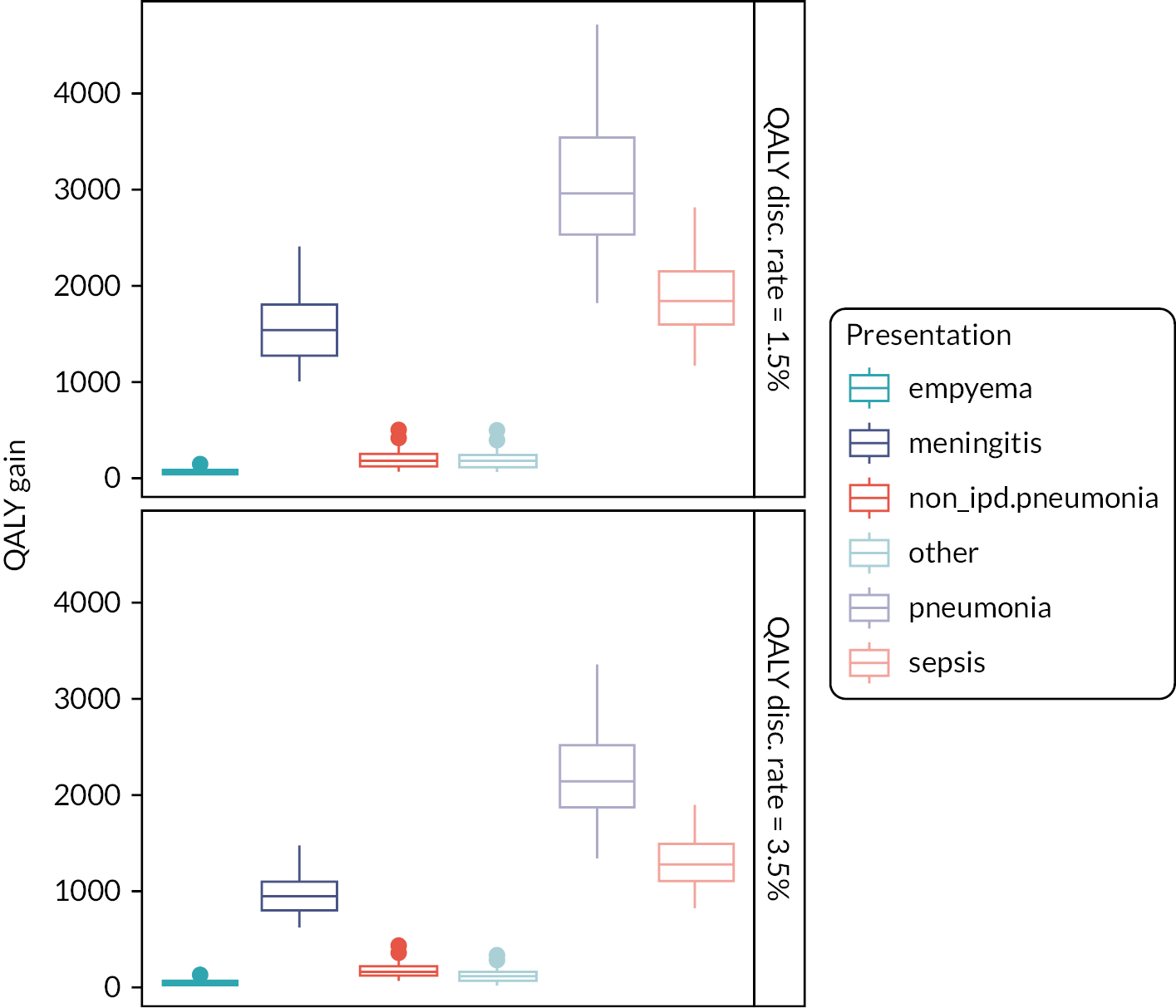
Figure 18 shows the maximum additional price that should be paid for a PCV13 dose under different scenarios. In the base-case analysis, introduction of PCV13 instead of PCV10 in 2006 would have been cost-effective in England and Wales provided the price of PCV13 was no more than an additional £3.57 (95% CI £3.42 to £3.71) per dose higher than PCV10, at a CET of £20,000 per QALY. Using a higher CET of £30,000 per QALY, additional threshold price increased to £5.13 (95% CI £4.92 to £5.33).
FIGURE 18.
Additional threshold price per dose in £2006 at which PCV13 becomes cost-effective compared to PCV10 under different scenario assumptions.
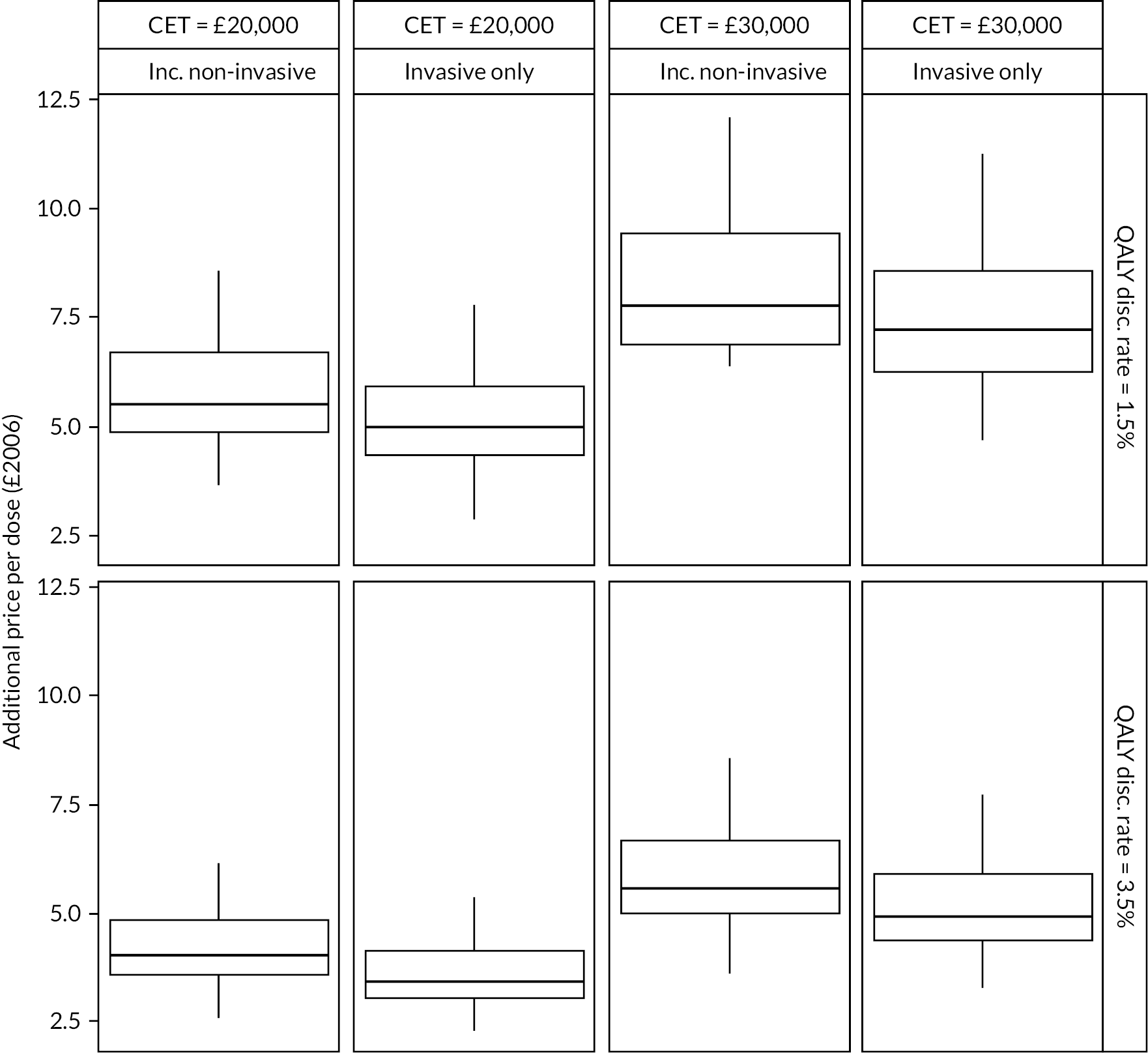
Chapter 6 Discussion
In our study, we used a novel methodology to define seroinfection from immunogenicity data to compare the relative efficacy of PCVs in preventing infection. Our results using individual-level data from a global meta-analysis provide the first estimates of the comparative protection afforded by different pneumococcal vaccines and show that for many serotypes, carriage events are less common after PCV13 than PCV10, likely due to a higher antibody response. In addition, we quantify the relationship between the immune response to vaccination and protection against infection, measured serologically, and show that higher antibody responses in infants are associated with greater protection from infection.
The observed heterogenicity in immune responses was unexpected. We assumed that if one vaccine is able to induce more antibody than another, then it would do so with some degree of consistency across all trials. However, this was not what was observed. Comparisons of the same vaccines in different studies gave widely varying estimates, and although we have reported the summary GMR estimates in our immunogenicity meta-analyses, the large degree of between-study heterogeneity in these models means these overall estimates are difficult to interpret. In some settings, PCV13 performed better; yet, in others, PCV10 was the more immunogenic vaccine. Although there was no single study-level factor that could be identified that might explain the variation in estimates, only three candidate factors could be considered (location, schedule and co-administered vaccines) and data reporting on co-administered vaccines were not always comprehensive. The assays used have been WHO standardised and unlikely to cause this variation, and additionally, only studies directly comparing the two vaccines were included.
Of note, comparisons between vaccines from the same manufacturer (PCV13 vs. PCV7) were more consistent than comparisons between vaccines from different manufacturers. Immune interference (‘bystander effects’) has been noted when vaccines with similar components are co-administered,108 and this may affect the responses to one vaccine over another. It is interesting that 18C and 19F were serotypes that showed a very large degree of between-study heterogeneity. These two serotypes in PCV10 have different carrier proteins (18C is conjugated to TT and 19F is conjugated to diphtheria toxoid) and may be more susceptible than other serotypes to the co-administration of vaccines containing tetanus or diphtheria components. An additional potential confounder that is unmeasured in these studies is the degree of exposure to circulating serotypes of pneumococcus in each setting, which also has the potential to influence the immune response to vaccines.
These diverse immunogenicity findings from studies of the same vaccines raise the question of whether such differences in immunogenicity lead to meaningful differences in protection. If so, it may be important to know which vaccine performs better in which setting and further investigation into the predictors of the immune response to vaccines may be warranted. We addressed this question by modelling the relationship between seroefficacy estimates and immunogenicity comparisons (GMRs), analysed at the trial level across all serotypes and studies. This method capitalises on the observed between-study heterogenicity rather than being hindered by it. In our model, vaccines with higher antibody levels were also those with greater protection against subclinical infections in general. A vaccine with twice the antibody production was predicted to halve the rate at which carriage occurred.
Licensure of new vaccines is based on non-inferiority comparisons with current vaccines and the proportion of antibody responses above the agreed threshold as a minimum requirement. Once a vaccine meets this ‘at-least-as-good-as’ immunogenicity criteria, it has previously not been clear whether exceeding it is of benefit, and the WHO position paper states ‘It is unknown whether a lower serotype-specific GMC of antibody indicates less efficacy’. 3 Our results show that lower protection against subclinical infection does indeed follow from lower antibody production and that two vaccines that produce a similar level of antibody will provide similar levels of protection, even if they are from different manufacturers.
The implications of these findings are of greatest importance when a new vaccine roll-out is being considered. Lower antibody production or lower seroefficacy for one vaccine product does not necessarily imply limited effectiveness against invasive pneumococcal diseases when considering vaccines such as PCV10 and PCV13 which are highly effective vaccines in many settings. Instead, lower antibody responses lead to less rapidly observed indirect protection after implementation into a national programme as a smaller proportion of transmission events are blocked by the vaccine. This is evident in the mathematical modelling in Chapter 4 which showed less rapid decreases in the number of cases of invasive disease when introducing PCV10 compared with PCV13.
For serotypes where protective impact has not been observed (serotype 3), new vaccines with substantially higher antibody responses may be needed. A Phase II clinical trial of PCV15 compared with PCV13 reported almost twice the antibody level for serotype 3 at 28 days post-primary series for PCV15 (GMR 1.93, 95% CI 1.71 to 2.18). 82 Based on our modelled association between GMR and RR, the RR of seroinfection with PCV15 versus PCV13 would be 0.48 (95% CI 0.21 to 0.87). Previously reported vaccine effectiveness estimates against nasopharyngeal carriage of serotype 3 include –27% (95% CI –180 to 44) and 1% (95% CI –106 to 52),30,109 and these translate to point estimates of 39% (95% CI –16% to 66%) and 52% (95% CI 9% to 79%) vaccine effectiveness against carriage of this serotype with PCV15 based on the relationship: (VE(pcv15) = (1 − RR(pcv15 vs pcv13) × (1 − VE(pcv13)/100%)) × 100%).
Implications for practice
This evidence of differences in serotype-specific protection can be incorporated into cost-effectiveness models used to compare vaccine products. 16 Cost-effectiveness studies have highlighted the lack of direct evidence of comparative efficacy of different PCVs, resulting in previous cost-effectiveness models that ignore serotype-specific differences and assume equivalent efficacy for all serotypes covered by different PCVs. 110–112 Our study fills this evidence gap and allows researchers and policy-makers to use more accurate vaccine-specific models in decision-making.
Our cost-effectiveness analysis of a hypothetical scenario showed that introducing infant PCV13 was predicted to avert a higher burden of pneumococcal disease compared to PCV10. This would have realised a small saving of £13 million discounted over 24 years.
When considering the introduction of new pneumococcal vaccines into the routine immunisation schedule, we recommend that differences in antibody responses for different vaccines be considered in modelling scenarios as higher antibody responses result in reduced transmission and greater impact on invasive diseases. Vaccine-specific threshold prices can then be determined for cost-effective vaccines. Our analysis showed that due to its higher efficacy against some serotypes, a higher threshold price per dose could be paid for PCV13 while remaining cost-effective. Seroefficacy estimates can also be determined for new pneumococcal vaccines and could contribute to licensing or policy decisions in the future.
Strength and limitations
Seroefficacy analyses need to be restricted to serotypes contained in both vaccines. Comparing a vaccinated cohort to a cohort that is unvaccinated, or receives a vaccine that does not contain the serotype of interest, will result in biased estimates as the immune response after exposure to a pathogen will differ in children whose immune system is primed for that pathogen, when compared with a naïve population. For this reason, we restricted our seroefficacy analysis to shared serotypes between vaccines. While seroinfection is most likely an indicator of nasopharyngeal carriage, it may also represent cases of asymptomatic bacteraemia.
Our analyses are based on a large set of studies conducted in infants for the most commonly used vaccines and make use of data originally collected for a different purpose than ours. We used the time points available in these studies. However, the time points one might use if designing a study for the purpose of calculating seroefficacy may differ and would likely include an additional time point 6–9 months after the booster dose. Without this time point, we are extrapolating pre-booster efficacy to post-booster time periods, and the impact of this assumption is unknown.
The mathematical and economic models used were based on outputs from our NMA models which contained significant heterogeneity. The potential for bias when using inconsistent data for modelling scenarios in this situation is hard to quantify but needs to be considered.
Public and patient involvement
The Oxford Vaccine Group public and patient involvement (PPI) group was involved at the design stage in the development of the plain language summary for the submitted grant proposal. Due to the nature of the project being a reanalysis of data from previously completely studies, there was no involvement of the PPI group in the data collection or analysis. Dissemination of results was discussed with the PPI lead, but as the conclusions and recommendations are relevant only to policy-makers and academics and as decision on vaccine product choice and purchases are made by the Department of Health for the whole of England, not by individuals, the PPI lead felt that further PPI review for the project would not be a good use of the PPI volunteer time.
Equality, diversity and inclusivity
The research team for the project was small but reflected contributions from under-represented groups. In particular, a large proportion of the key contributors were female, including first, second and senior authors, including the principal investigator. Junior members of the team are fully acknowledged with authorship on the final report and all publications arising from the project.
Data from individual studies included in the systematic review were from a wide range of countries and regions.
Conclusions
In conclusion, we estimated serotype-specific difference in both seroefficacy and immunogenicity between PCV10 and PCV13. Higher IgG antibody levels confer better protection against seroinfection. This methodology can be further used to compare novel high-valent PCVs and to inform cost-effectiveness models.
Additional information
Contributions of authors
Shuo Feng (https://orcid.org/0000-0001-7855-0991) (Statistician/Epidemiologist) contributed to the study methods, obtained the individual participant data, extracted the aggregated data, analysed the data and wrote the first draft of the manuscript.
Julie McLellan (https://orcid.org/0000-0002-2868-8631) (Senior Systematic Reviewer) contributed to the study methods, conducted the study selection and assessment of bias and contributed to data interpretation and manuscript development.
Nicola Pidduck (https://orcid.org/0000-0002-6491-1159) (Research Assistant) conducted the study selection and assessment of bias.
Nia Roberts (https://orcid.org/0000-0002-1142-6440) (Senior Outreach Librarian) contributed to the study methods, devised and conducted the search strategy.
Julian PT Higgins (https://orcid.org/0000-0002-8323-2514) (Professor of Evidence Synthesis) contributed to the study methods, data interpretation and reviewed the final version of the manuscript.
Yoon Choi (https://orcid.org/0000-0001-5561-4366) (Infectious disease modeller) conducted the mathematical modelling and manuscript development.
Alane Izu (https://orcid.org/0000-0002-5547-7223) (Senior Statistician) contributed to data collection and interpretation and reviewed the final version of the manuscript.
Mark Jit (https://orcid.org/0000-0001-6658-8255) (Professor of Vaccine Epidemiology) contributed to the study methods, conducted health economic analyses and manuscript development.
Shabir A Madhi (https://orcid.org/0000-0002-7629-0636) (Professor of Vaccinology) contributed to data collection and interpretation and reviewed the final version of the manuscript.
Kim Mulholland (https://orcid.org/0000-0001-7947-680X) (Professor of Child Health) contributed to data collection and interpretation and reviewed the final version of the manuscript.
Andrew J Pollard (https://orcid.org/0000-0001-7361-719X) (Ashall Professor of Infection and Immunity) contributed to data interpretation and reviewed the final version of the manuscript.
Simon Procter (https://orcid.org/0000-0002-0380-1503) (Research Fellow, Department of Infectious Disease Epidemiology) conducted health economic analyses, data interpretation and manuscript development.
Beth Temple (https://orcid.org/0000-0002-4885-9848) (Epidemiologist) contributed to data collection and interpretation and reviewed the final version of the manuscript.
Merryn Voysey (https://orcid.org/0000-0001-6324-6559) (Associate Professor of Statistics in Vaccinology) conceived and designed the study, obtained study funding, obtained the individual participant data and extracted the aggregated data and provided study oversight.
Data-sharing statement
Data were obtained through third-party resources (Pfizer data through Vivli Inc. and data from GlaxoSmithKline through clinicalstudydatarequest.com) and were made available for a limited period of time to conduct these analyses. The authors do not have continuing access to the data sets. Vivli, clinicalstudydatarequest.com, Pfizer Inc. and GlaxoSmithKline have not contributed to or approved, and are not in any way responsible for, the contents of this publication. All other queries should be addressed to the corresponding author.
Ethics statement
This is a meta-analysis of anonymised data from previously conducted clinical trials and as such does not require review by an ethics committee. Each individual study included in the meta-analysis had ethical approval in place. Data-sharing agreements were entered into with entities providing anonymised individual-level data.
Information governance statement
There was no personal information handled as part of this work.
Disclosure of interests
Full disclosure of interests: Completed ICMJE forms for all authors, including all related interests, or available in the toolkit on the NIHR Journals Library report publication page at https://doi.org/10.3310/YWHA3079.
Primary conflicts of interest: Andrew J Pollard is Chair of the UK DHSC Joint on Vaccination and Immunisation and was a member of the Strategic Advisory Group of Experts on Immunization to the World Health Organization (WHO) until 2022. Andrew J Pollard, Merryn Voysey and Shuo Feng are contributors to COVID-19 vaccine intellectual property licensed by Oxford University Innovation to AstraZeneca. Shabir A Madhi reports grants to his institution from the Bill and Melinda Gates Foundation, Pfizer and GlaxoSmithKline and is member of the Strategic Advisory Group of Experts on Immunization to the WHO. Kim Mulholland is an investigator on grants from MSD and Pfizer and reports grants to his institution from the Bill and Melinda Gates Foundation and the WHO. Mark Jit reports grants to his institution from Bill and Melinda Gates Foundation, Gavi the Vaccine Alliance, NIHR, RCUK, European Commission and Wellcome Trust. All other authors declare no competing interests. The remaining authors declare no competing interests. The views expressed in this article do not necessarily represent the views of the DHSC, JCVI, NIHR or WHO.
Disclaimers
This article presents independent research funded by the National Institute for Health and Care Research (NIHR). The views and opinions expressed by authors in this publication are those of the authors and do not necessarily reflect those of the NHS, the NIHR, the HTA programme or the Department of Health and Social Care. If there are verbatim quotations included in this publication the views and opinions expressed by the interviewees are those of the interviewees and do not necessarily reflect those of the authors, those of the NHS, the NIHR, the HTA programme or the Department of Health and Social Care.
References
- Wahl B, O’Brien KL, Greenbaum A, Majumder A, Liu L, Chu Y, et al. Burden of Streptococcus pneumoniae and Haemophilus influenzae type b disease in children in the era of conjugate vaccines: global, regional, and national estimates for 2000–15. Lancet Glob Health 2018;6:e744-57.
- Johnson HL, Deloria-Knoll M, Levine OS, Stoszek SK, Hance LF, Reithinger R, et al. Systematic evaluation of serotypes causing invasive pneumococcal disease among children under five: the pneumococcal global serotype project. PLOS Med 2010;7.
- Shiri T, Datta S, Madan J, Tsertsvadze A, Royle P, Keeling MJ, et al. Indirect effects of childhood pneumococcal conjugate vaccination on invasive pneumococcal disease: a systematic review and meta-analysis. Lancet Glob Health 2017;5:e51-9.
- Alderson MR, Sethna V, Newhouse LC, Lamola S, Dhere R. Development strategy and lessons learned for a 10-valent pneumococcal conjugate vaccine (PNEUMOSIL®). Hum Vaccin Immunother 2021;17:2670-7.
- Siber GR, Chang I, Baker S, Fernsten P, O’Brien KL, Santosham M, et al. Estimating the protective concentration of anti-pneumococcal capsular polysaccharide antibodies. Vaccine 2007;25:3816-26.
- Andrews NJ, Waight PA, Burbidge P, Pearce E, Roalfe L, Zancolli M, et al. Serotype-specific effectiveness and correlates of protection for the 13-valent pneumococcal conjugate vaccine: a postlicensure indirect cohort study. Lancet Infect Dis 2014;14:839-46.
- Berman-Rosa M, O’Donnell S, Barker M, Quach C. Efficacy and effectiveness of the PCV-10 and PCV-13 vaccines against invasive pneumococcal disease. Pediatrics 2020;145.
- Temple B, Nation ML, Dai VTT, Beissbarth J, Bright K, Dunne EM, et al. Effect of a 2 + 1 schedule of ten-valent versus 13-valent pneumococcal conjugate vaccine on pneumococcal carriage: results from a randomised controlled trial in Vietnam. Vaccine 2021;39:2303-10.
- Pomat WS, van den Biggelaar AHJ, Wana S, Francis JP, Solomon V, Greenhill AR, et al. 10v13v PCV Trial Team . Safety and immunogenicity of pneumococcal conjugate vaccines in a high-risk population: a randomized controlled trial of 10-valent and 13-valent pneumococcal conjugate vaccine in Papua New Guinean infants. Clin Infect Dis 2019;68:1472-81.
- Voysey M, Fanshawe TR, Kelly DF, O’Brien KL, Kandasamy R, Shrestha S, et al. Serotype-specific correlates of protection for pneumococcal carriage: an analysis of immunity in 19 countries. Clin Infect Dis 2018;66:913-20.
- Liberati A, Altman DG, Tetzlaff J, Mulrow C, Gøtzsche PC, Ioannidis JPA, et al. The PRISMA statement for reporting systematic reviews and meta-analyses of studies that evaluate healthcare interventions: explanation and elaboration. BMJ 2009;339.
- Stewart LA, Clarke M, Rovers M, Riley RD, Simmonds M, Stewart G, et al. PRISMA-IPD Development Group . Preferred Reporting Items for Systematic Review and Meta-Analyses of individual participant data: the PRISMA-IPD statement. JAMA 2015;313:1657-65.
- Hutton B, Salanti G, Caldwell DM, Chaimani A, Schmid CH, Cameron C, et al. The PRISMA extension statement for reporting of systematic reviews incorporating network meta-analyses of health care interventions: checklist and explanations. Ann Intern Med 2015;162:777-84.
- Rücker G. Network meta-analysis, electrical networks and graph theory. Res Synth Methods 2012;3:312-24.
- Schwarzer G, Carpenter JR, Rücker G. Network Meta-Analysis. Meta-Analysis with R. Cham: Springer Publishing; 2015.
- Sterne JAC, Savović J, Page MJ, Elbers RG, Blencowe NS, Boutron I, et al. RoB 2: a revised tool for assessing risk of bias in randomised trials. BMJ 2019;366.
- McGuinness LA, Higgins JPT. Risk-of-bias VISualization (robvis): an R package and Shiny web app for visualizing risk-of-bias assessments. Res Synth Methods 2021;12:55-61.
- Amdekar YK, Lalwani SK, Bavdekar A, Balasubramanian S, Chhatwal J, Bhat SR, et al. Immunogenicity and safety of a 13-valent pneumococcal conjugate vaccine in healthy infants and toddlers given with routine vaccines in India. Pediatr Infect Dis J 2013;32:509-16.
- Anonymous. Correction to Lancet Infect Dis. Lancet Infect Dis 2020;20. https://doi.org/10.1016/S1473-3099(20)30289-9.
- Beissbarth J, Wilson N, Arrowsmith B, Binks MJ, Oguoma VM, Lawrence K, et al. Nasopharyngeal carriage of otitis media pathogens in infants receiving 10-valent non-typeable Haemophilus influenzae protein D conjugate vaccine (PHiD-CV10), 13-valent pneumococcal conjugate vaccine (PCV13) or a mixed primary schedule of both vaccines: a randomised controlled trial. Vaccine 2021;39:2264-73.
- Bermal N, Szenborn L, Chrobot A, Alberto E, Lommel P, Gatchalian S, et al. The 10-valent pneumococcal non-typeable Haemophilus influenzae protein D conjugate vaccine (PHiD-CV) coadministered with DTPw-HBV/Hib and poliovirus vaccines: assessment of immunogenicity. Pediatr Infect Dis J 2009;28:S89-96.
- Bermal N, Szenborn L, Edison A, Hernandez M, Pejcz J, Majda-Stanislawska E, et al. Safety and immunogenicity of a booster dose of the 10-valent pneumococcal nontypeable Haemophilus influenzae protein D conjugate vaccine coadministered with DTPw-HBV/Hib and poliovirus vaccines. Pediatr Infect Dis J 2011;30:69-72.
- Bryant KA, Block SL, Baker SA, Gruber WC, Scott DA, Group PCVIS. Safety and immunogenicity of a 13-valent pneumococcal conjugate vaccine. Pediatrics 2010;125:866-75.
- Carmona Martinez A, Prymula R, Miranda Valdivieso M, Otero Reigada MDC, Merino Arribas JM, Brzostek J, et al. Immunogenicity and safety of 11- and 12-valent pneumococcal non-typeable Haemophilus influenzae protein D-conjugate vaccines (11vPHiD-CV, 12vPHiD-CV) in infants: results from a phase II, randomised, multicentre study. Vaccine 2019;37:176-86.
- Cooper D, Yu X, Sidhu M, Nahm MH, Fernsten P, Jansen KU. The 13-valent pneumococcal conjugate vaccine (PCV13) elicits cross-functional opsonophagocytic killing responses in humans to Streptococcus pneumoniae serotypes 6C and 7A. Vaccine 2011;29:7207-11.
- Dagan R, Jiang Q, Juergens C, Trammel J, Gruber WC, Scott DA. Carrier-induced hyporesponsiveness to pneumococcal conjugate vaccines: unraveling the influence of serotypes, timing, and previous vaccine dose. Clin Infect Dis 2021;72:448-54.
- Dagan R, Juergens C, Trammel J, Patterson S, Greenberg D, Givon-Lavi N, et al. Modeling pneumococcal nasopharyngeal acquisition as a function of anticapsular serum antibody concentrations after pneumococcal conjugate vaccine administration. Vaccine 2016;34:4313-20.
- Dagan R, Juergens C, Trammel J, Patterson S, Greenberg D, Givon-Lavi N, et al. PCV13-vaccinated children still carrying PCV13 additional serotypes show similar carriage density to a control group of PCV7-vaccinated children. Vaccine 2017;35:945-50.
- Dagan R, Juergens C, Trammel J, Patterson S, Greenberg D, Givon-Lavi N, et al. Efficacy of 13-valent pneumococcal conjugate vaccine (PCV13) versus that of 7-valent PCV (PCV7) against nasopharyngeal colonization of antibiotic-nonsusceptible Streptococcus pneumoniae. J Infect Dis 2015;211:1144-53.
- Dagan R, Patterson S, Juergens C, Greenberg D, Givon-Lavi N, Porat N, et al. Comparative immunogenicity and efficacy of 13-valent and 7-valent pneumococcal conjugate vaccines in reducing nasopharyngeal colonization: a randomized double-blind trial. Clin Infect Dis 2013;57:952-62.
- Esposito S, Tansey S, Thompson A, Razmpour A, Liang J, Jones TR, et al. Safety and immunogenicity of a 13-valent pneumococcal conjugate vaccine compared to those of a 7-valent pneumococcal conjugate vaccine given as a three-dose series with routine vaccines in healthy infants and toddlers. Clin Vaccine Immunol 2010;17:1017-26.
- Gimenez-Sanchez F, Kieninger DM, Kueper K, Martinon-Torres F, Bernaola E, Diez-Domingo J, et al. 501 and 006 study groups . Immunogenicity of a combination vaccine containing diphtheria toxoid, tetanus toxoid, three-component acellular pertussis, hepatitis B, inactivated polio virus, and Haemophilus influenzae type b when given concomitantly with 13-valent pneumococcal conjugate vaccine. Vaccine 2011;29:6042-8.
- Grimprel E, Laudat F, Patterson S, Baker SA, Sidhu MS, Gruber WC, et al. Immunogenicity and safety of a 13-valent pneumococcal conjugate vaccine (PCV13) when given as a toddler dose to children immunized with PCV7 as infants. Vaccine 2011;29:9675-83.
- Huang LM, Lin TY, Juergens C. Immunogenicity and safety of a 13-valent pneumococcal conjugate vaccine given with routine pediatric vaccines in Taiwan. Vaccine 2012;30:2054-9.
- Juergens C, Patterson S, Trammel J, Greenberg D, Givon-Lavi N, Cooper D, et al. Post hoc analysis of a randomized double-blind trial of the correlation of functional and binding antibody responses elicited by 13-valent and 7-valent pneumococcal conjugate vaccines and association with nasopharyngeal colonization. Clin Vaccine Immunol 2014;21:1277-81.
- Kieninger DM, Kueper K, Steul K, Juergens C, Ahlers N, Baker S, et al. 006 study group . Safety, tolerability, and immunologic noninferiority of a 13-valent pneumococcal conjugate vaccine compared to a 7-valent pneumococcal conjugate vaccine given with routine pediatric vaccinations in Germany. Vaccine 2010;28:4192-203.
- Kim CH, Kim JS, Cha SH, Kim KN, Kim JD, Lee KY, et al. Response to primary and booster vaccination with 10-valent pneumococcal nontypeable Haemophilus influenzae protein D conjugate vaccine in Korean infants. Pediatr Infect Dis J 2011;30:e235-43.
- Kim DS, Shin SH, Lee HJ, Hong YJ, Lee SY, Choi KM, et al. Immunogenicity and safety of 13-valent pneumococcal conjugate vaccine given to Korean children receiving routine pediatric vaccines. Pediatr Infect Dis J 2013;32:266-73.
- Knuf M, Pankow-Culot H, Grunert D, Rapp M, Panzer F, Köllges R, et al. Induction of immunologic memory following primary vaccination with the 10-valent pneumococcal nontypeable Haemophilus influenzae protein D conjugate vaccine in infants. Pediatr Infect Dis J 2012;31:e31-6.
- Leach AJ, Mulholland EK, Santosham M, Torzillo PJ, Brown NJ, McIntyre P, et al. Pneumococcal conjugate vaccines PREVenar13 and SynflorIX in sequence or alone in high-risk Indigenous infants (PREV-IX_COMBO): protocol of a randomised controlled trial. BMJ Open 2015;5.
- Leach AJ, Mulholland EK, Santosham M, Torzillo PJ, McIntyre P, Smith-Vaughan H, et al. Otitis media outcomes of a combined 10-valent pneumococcal Haemophilus influenzae protein D conjugate vaccine and 13-valent pneumococcal conjugate vaccine schedule at 1-2-4-6 months: PREVIX_COMBO, a 3-arm randomised controlled trial. BMC Pediatr 2021;21.
- Leach AJ, Mulholland EK, Santosham M, Torzillo PJ, McIntyre P, Smith-Vaughan H, et al. Interchangeability, immunogenicity and safety of a combined 10-valent pneumococcal Haemophilus influenzae protein D conjugate vaccine (Synflorix) and 13-valent-PCV (Prevenar13) schedule at 1-2-4-6 months: PREVIX_COMBO, a 3-arm randomised controlled trial. Vaccine X 2021;7.
- Leach AJ, Wilson N, Arrowsmith B, Beissbarth J, Mulholland EK, Santosham M, et al. Immunogenicity, otitis media, hearing impairment, and nasopharyngeal carriage 6-months after 13-valent or ten-valent booster pneumococcal conjugate vaccines, stratified by mixed priming schedules: PREVIX_COMBO and PREVIX_BOOST randomised controlled trials. Lancet Infect Dis 2022;22:1374-87.
- Lee HJ, Shin SH, Kim DS, . Immunogenicity and safety of 13-valent pneumococcal conjugate vaccine given with routine pediatric vaccines to infants in Korea. Acta Paediatr Int J Paediatr 2010;99.
- Lehmann D, Kirarock W, van den Biggelaar AHJ, Passey M, Jacoby P, Saleu G, et al. 10v13v PCV trial team . Rationale and methods of a randomized controlled trial of immunogenicity, safety and impact on carriage of pneumococcal conjugate and polysaccharide vaccines in infants in Papua New Guinea. Pneumonia (Nathan) 2017;9.
- Licciardi P, Phan T, Toh ZQ, . Immunogenicity and memory B cell response following alternative pneumococcal vaccination strategies in Vietnam. Eur J Immunol 2016;46.
- Licciardi PV, Temple B, Dai VTT, Toan NT, Uyen D, Nguyen CD, et al. Immunogenicity of alternative ten-valent pneumococcal conjugate vaccine schedules in infants in Ho Chi Minh City, Vietnam: results from a single-blind, parallel-group, open-label, randomised, controlled trial. Lancet Infect Dis 2021;21:1415-28.
- Madhi SA, Mutsaerts EA, Izu A, Boyce W, Bhikha S, Ikulinda BT, et al. Immunogenicity of a single-dose compared with a two-dose primary series followed by a booster dose of ten-valent or 13-valent pneumococcal conjugate vaccine in South African children: an open-label, randomised, non-inferiority trial. Lancet Infect Dis 2020;20:1426-36.
- Odutola A, Ota MOC, Antonio M, Ogundare EO, Saidu Y, Foster-Nyarko E, et al. Efficacy of a novel, protein-based pneumococcal vaccine against nasopharyngeal carriage of Streptococcus pneumoniae in infants: a phase 2, randomized, controlled, observer-blind study. Vaccine 2017;35:2531-42.
- Odutola A, Ota MOC, Antonio M, Ogundare EO, Saidu Y, Owiafe PK, et al. Immunogenicity of pneumococcal conjugate vaccine formulations containing pneumococcal proteins, and immunogenicity and reactogenicity of co-administered routine vaccines: a phase II, randomised, observer-blind study in Gambian infants. Vaccine 2019;37:2586-99.
- Payton T, Girgenti D, Frenck RW, Patterson S, Love J, Razmpour A, et al. Immunogenicity, safety and tolerability of 3 lots of 13-valent pneumococcal conjugate vaccine given with routine pediatric vaccinations in the United States. Pediatr Infect Dis J 2013;32:871-80.
- Pomat WS, van den Biggelaar AHJ, Wana S, Francis JP, Solomon V, Greenhill AR, et al. Safety and immunogenicity of pneumococcal conjugate vaccines in a high-risk population: a randomised controlled trial of 10-valent and 13-valent PCV in Papua New Guinean infants. Clin Infect Dis 2018;68:1472-81.
- Prymula R, Szenborn L, Silfverdal SA, Wysocki J, Albrecht P, Traskine M, et al. Immunogenicity of the booster dose of 2 investigational protein- based pneumococcal vaccine formulations in toddlers: a phase ii randomized trial. Open Forum Infect Dis 2016;3.
- Prymula R, Szenborn L, Silfverdal SA, Wysocki J, Albrecht P, Traskine M, et al. Safety and reactogenicity of the booster dose of 2 investigational protein-based pneumococcal vaccine formulations in toddlers: a phase II randomized trial. Open Forum Infect Disk 2016;3.
- Prymula R, Szenborn L, Silfverdal SA, Wysocki J, Albrecht P, Traskine M, et al. Safety, reactogenicity and immunogenicity of two investigational pneumococcal protein-based vaccines: results from a randomized phase II study in infants. Vaccine 2017;35:4603-11.
- Snape MD, Klinger CL, Daniels ED, John TM, Layton H, Rollinson L, et al. Immunogenicity and reactogenicity of a 13-valent-pneumococcal conjugate vaccine administered at 2, 4, and 12 months of age: a double-blind randomized active-controlled trial. Pediatr Infect Dis J 2010;29:e80-90.
- Temple B, Toan NT, Dai VTT, Bright K, Licciardi PV, Marimla RA, et al. Immunogenicity and reactogenicity of ten-valent versus 13-valent pneumococcal conjugate vaccines among infants in Ho Chi Minh City, Vietnam: a randomised controlled trial. Lancet Infect Dis 2019;19:497-509.
- Temple B, Toan NT, Uyen DY, Balloch A, Bright K, Cheung YB, et al. Evaluation of different infant vaccination schedules incorporating pneumococcal vaccination (The Vietnam Pneumococcal Project): protocol of a randomised controlled trial. BMJ Open 2018;8.
- Togashi T, Okada K, Yamaji M, Thompson A, Gurtman A, Cutler M, et al. Immunogenicity and safety of a 13-valent pneumococcal conjugate vaccine given with DTaP vaccine in healthy infants in Japan. Pediatr Infect Dis J 2015;34:1096-104.
- van den Bergh MR, Spijkerman J, Francois N, Swinnen K, Borys D, Schuerman L, et al. Immunogenicity, safety, and reactogenicity of the 10-valent pneumococcal nontypeable Haemophilus influenzae protein D conjugate vaccine and DTPa-IPV-Hib when coadministered as a 3-dose primary vaccination schedule in The Netherlands: a randomized controlled trial. Pediatr Infect Dis J 2011;30:e170-8.
- van den Bergh MR, Spijkerman J, Francois N, Swinnen K, Borys D, Schuerman L, et al. Immunogenicity, safety and reactogenicity of a booster dose of the 10-valent pneumococcal nontypeable H. influenzae protein D conjugate baccine coadministered with DTPa-IPV-Hib in Dutch children: a randomized controlled trial. Pediatr Infect Dis J 2016;35:e206-19.
- van den Bergh MR, Spijkerman J, Swinnen KM, François NA, Pascal TG, Borys D, et al. Effects of the 10-valent pneumococcal nontypeable Haemophilus influenzae protein D-conjugate vaccine on nasopharyngeal bacterial colonization in young children: a randomized controlled trial. Clin Infect Dis 2013;56:e30-9.
- van den Biggelaar AHJ, Pomat WS, Masiria G, Wana S, Nivio B, Francis J, et al. Immunogenicity and immune memory after a pneumococcal polysaccharide vaccine booster in a high-risk population primed with 10-valent or 13-valent pneumococcal conjugate vaccine: a randomized controlled trial in Papua New Guinean children. Vaccines 2019;7.
- Vesikari T, Wysocki J, Chevallier B, Karvonen A, Czajka H, Arsène JP, et al. Immunogenicity of the 10-valent pneumococcal non-typeable Haemophilus influenzae protein D conjugate vaccine (PHiD-CV) compared to the licensed 7vCRM vaccine. Pediatr Infect Dis J 2009;28:S66-76.
- Weckx LY, Thompson A, Berezin EN, de Faria SM, da Cunha CA, Pride M, et al. 012 Study Group . A phase 3, randomized, double-blind trial comparing the safety and immunogenicity of the 7-valent and 13-valent pneumococcal conjugate vaccines, given with routine pediatric vaccinations, in healthy infants in Brazil. Vaccine 2012;30:7566-72.
- Wysocki J, Tejedor JC, Grunert D, Konior R, Garcia-Sicilia J, Knuf M, et al. Immunogenicity of the 10-valent pneumococcal non-typeable Haemophilus influenzae protein D conjugate vaccine (PHiD-CV) when coadministered with different neisseria meningitidis serogroup C conjugate vaccines. Pediatr Infect Dis J 2009;28:S77-88.
- Yeh SH, Gurtman A, Hurley DC, Block SL, Schwartz RH, Patterson S, et al. 004 Study Group . Immunogenicity and safety of 13-valent pneumococcal conjugate vaccine in infants and toddlers. Pediatrics 2010;126:e493-505.
- Zhu F, Hu Y, Li J, Ye Q, Young MM, Liang JZ, et al. Immunogenicity and safety of the 13-valent pneumococcal conjugate vaccine administered in a 3 + 1 versus 2 + 1 dose schedule among infants in China. Pediatr Infect Dis J 2019;38:1150-8.
- Zhu F, Hu Y, Li J, Ye Q, Young MM, Zhou X, et al. Immunogenicity and safety of 13-valent pneumococcal conjugate vaccine compared with 7-valent pneumococcal conjugate vaccine among healthy infants in China. Pediatr Infect Dis J 2016;35:999-1010.
- De Los Santos AM, Rodriguez-Weber MA, Sanchez-Marquez P, . Immunogenicity of a 2 + 1 infant vaccination series with 13-valent pneumococcal conjugate vaccine (PCV13) followed by pneumococcal non-typeable Haemophilus influenzae protein d conjugate vaccine (PHID-CV): a randomized trial exploring interchangeability of PCVS. Open Forum Infect Dis 2017;4:S538-9.
- Diez-Domingo J, Gurtman A, Bernaola E, Gimenez-Sanchez F, Martinon-Torres F, Pineda-Solas V, et al. Evaluation of 13-valent pneumococcal conjugate vaccine and concomitant meningococcal group C conjugate vaccine in healthy infants and toddlers in Spain. Vaccine 2013;31:5486-94.
- Martinon-Torres F, Gimenez-Sanchez F, Gurtman A, Bernaola E, Diez-Domingo J, Carmona A, et al. 3007 Study Group . 13-valent pneumococcal conjugate vaccine given with meningococcal C-tetanus toxoid conjugate and other routine pediatric vaccinations: immunogenicity and safety. Pediatr Infect Dis J 2012;31:392-9.
- Vanderkooi OG, Scheifele DW, Girgenti D, Halperin SA, Patterson SD, Gruber WC, et al. Canadian PCV13 Study Group . Safety and immunogenicity of a 13-valent pneumococcal conjugate vaccine in healthy infants and toddlers given with routine pediatric vaccinations in Canada. Pediatr Infect Dis J 2012;31:72-7.
- Banniettis N, Wysocki J, Szenborn L, Phongsamart W, Pitisuttithum P, Rämet M, et al. Phase 3 study to evaluate the safety, tolerability, and immunogenicity of catch-up vaccination regimens of V114 in healthy infants, children, and adolescents (PNEU-PLAN). Open Forum Infect Dis 2021;8.
- Bili A, Dobson S, Quinones J, Phongsamart W, Oberdorfer P, Kosalaraksa P, et al. A Phase 3, multicenter, randomized, double-blind study to evaluate the interchangeability of V114 and Prevnar 13TM with respect to safety, tolerability, and immunogenicity in healthy infants (PNEU-DIRECTION). Open Forum Infect Dis 2021;8.
- Chen JJ, Yuan L, Huang Z, Shi NM, Zhao YL, Xia SL, et al. Safety and immunogenicity of a new 13-valent pneumococcal conjugate vaccine versus a licensed 7-valent pneumococcal conjugate vaccine: a study protocol of a randomised non-inferiority trial in China. BMJ Open 2016;6.
- Clarke E, Bashorun A, Adigweme I, Badjie Hydara M, Umesi A, Futa A, et al. Immunogenicity and safety of a novel ten-valent pneumococcal conjugate vaccine in healthy infants in the Gambia: a phase 3, randomised, double-blind, non-inferiority trial. Lancet Infect Dis 2021;21:834-46.
- Clarke E, Bashorun AO, Okoye M, Umesi A, Badjie Hydara M, Adigweme I, et al. Safety and immunogenicity of a novel 10-valent pneumococcal conjugate vaccine candidate in adults, toddlers, and infants in the Gambia: results of a phase 1/2 randomized, double-blinded, controlled trial. Vaccine 2020;38:399-410.
- Dagan R, Melamed R, Muallem M, Piglansky L, Greenberg D, Abramson O, et al. Reduction of nasopharyngeal carriage of pneumococci during the second year of life by a heptavalent conjugate pneumococcal vaccine. J Infect Dis 1996;174:1271-8.
- Greenberg D, Hoover PA, Vesikari T, Peltier C, Hurley DC, McFetridge RD, et al. Safety and immunogenicity of 15-valent pneumococcal conjugate vaccine (PCV15) in healthy infants. Vaccine 2018;36:6883-91.
- Martinez CPD, Linares-Perez N, Toledo-Romani ME, Delgado YR, Gómez RP, Moreno BP, et al. Havana-Pneumococci Clinical Group . Safety and immunogenicity of the Cuban heptavalent pneumococcal conjugate vaccine in healthy infants: results from a double-blind randomized control trial Phase I. Vaccine 2018;36:4944-51.
- Platt HL, Greenberg D, Tapiero B, Clifford RA, Klein NP, Hurley DC, et al. V114-008 Study Group . A Phase II trial of safety, tolerability and immunogenicity of V114, a 15-valent pneumococcal conjugate vaccine, compared with 13-valent pneumococcal conjugate vaccine in healthy infants. Pediatr Infect Dis J 2020;39:763-70.
- Rupp R, Hurley D, Grayson S, Li J, Nolan K, McFetridge RD, et al. A dose ranging study of 2 different formulations of 15-valent pneumococcal conjugate vaccine (PCV15) in healthy infants. Hum Vaccin Immunotherap 2019;15:549-59.
- Senders S, Klein NP, Lamberth E, Thompson A, Drozd J, Trammel J, et al. Safety and immunogenicity of a 20-valent pneumococcal conjugate vaccine (PCV20) in healthy infants in the United States. Open Forum Infect Dis 2020;7.
- Senders S, Klein NP, Lamberth E, Thompson A, Drozd J, Trammel J, et al. Safety and immunogenicity of a 20-valent pneumococcal conjugate vaccine in healthy infants in the United States. Pediatr Infect Dis J 2021;40:944-51.
- Shin J, Teeratakulpisarn J, Puthanakit T, Theerawit T, Ryu JH, Shin J, et al. Immunogenicity and safety of a 12-valent pneumococcal conjugate vaccine in infants aged 6–10 weeks: a randomized double-blind active-controlled trial. Clin Exp Pediatr 2020;63:265-71.
- Thisyakorn U, Chokephaibulkit K, Kosalaraksa P, Benjaponpitak S, Pancharoen C, Chuenkitmongkol S. Immunogenicity and safety of 23-valent pneumococcal polysaccharide vaccine as a booster dose in 12- to 18-month-old children primed with 3 doses of 7-valent pneumococcal conjugate vaccine. Hum Vaccin Immunotherap 2014;10:1859-65.
- Zhao Y, Li G, Xia S, Ye Q, Yuan L, Li H, et al. Immunogenicity and safety of a novel 13-valent pneumococcal vaccine in healthy Chinese infants and toddlers. Front Microbiol 2022;13.
- Adigweme I, Futa A, Saidy-Jah E, Edem B, Akpalu E, Dibbasey T, et al. Immunogenicity and safety of a 10-valent pneumococcal conjugate vaccine administered as a 2 + 1 schedule to healthy infants in the Gambia: a single-centre, double-blind, active-controlled, randomised, phase 3 trial. Lancet Infect Dis 2023;23:609-20.
- Bili A, Dobson S, Quinones J, Phongsamart W, Oberdorfer P, Kosalaraksa P, et al. V114-027 PNEU-DIRECTION study group . A phase 3, multicenter, randomized, double-blind study to evaluate the interchangeability of V114, a 15-valent pneumococcal conjugate vaccine, and PCV13 with respect to safety, tolerability, and immunogenicity in healthy infants (PNEU-DIRECTION). Vaccine 2023;41:657-65.
- Banniettis N, Wysocki J, Szenborn L, Phongsamart W, Pitisuttithum P, Rämet M, et al. V114-024 PNEU-PLAN study group . A phase III, multicenter, randomized, double-blind, active comparator-controlled study to evaluate the safety, tolerability, and immunogenicity of catch-up vaccination regimens of V114, a 15-valent pneumococcal conjugate vaccine, in healthy infants, children, and adolescents (PNEU-PLAN). Vaccine 2022;40:6315-25.
- Lupinacci R, Rupp R, Wittawatmongkol O, Jones J, Quinones J, Ulukol B, et al. A Phase 3, multicenter, randomised, doubleblind, active-comparator-controlled study to evaluate the safety, tolerability, and immunogenicity of a 4-dose regimen of v114 in healthy infants (PNEU-PED). Arch Dis Child 2022;107.
- Lupinacci R, Rupp R, Wittawatmongkol O, Jones J, Quinones J, Ulukol B, et al. V114-029 PNEU-PED study group . A phase 3, multicenter, randomized, double-blind, active-comparator-controlled study to evaluate the safety, tolerability, and immunogenicity of a 4-dose regimen of V114, a 15-valent pneumococcal conjugate vaccine, in healthy infants (PNEU-PED). Vaccine 2023;41:1142-52.
- Melegaro A, Choi YH, George R, Edmunds WJ, Miller E, Gay NJ. Dynamic models of pneumococcal carriage and the impact of the heptavalent pneumococcal conjugate vaccine on invasive pneumococcal disease. BMC Infect Dis 2010;10:1-15.
- Hussain M, Melegaro A, Pebody RG, George R, Edmunds WJ, Talukdar R, et al. A longitudinal household study of Streptococcus pneumoniae nasopharyngeal carriage in a UK setting. Epidemiol Infect 2005;133:891-8.
- Mossong J, Hens N, Jit M, Beutels P, Auranen K, Mikolajczyk R, et al. Social contacts and mixing patterns relevant to the spread of infectious diseases. PLOS Med 2008;5.
- van Hoek AJ, Andrews N, Campbell H, Amirthalingam G, Edmunds WJ, Miller E. The social life of infants in the context of infectious disease transmission; social contacts and mixing patterns of the very young. PLOS ONE 2013;8.
- Choi YH, Andrews N, Miller E. Estimated impact of revising the 13-valent pneumococcal conjugate vaccine schedule from 2 + 1 to 1 + 1 in England and Wales: a modelling study. PLOS Med 2019;16.
- Choi YH, Jit M, Flasche S, Gay N, Miller E. Mathematical modelling long-term effects of replacing Prevnar7 with Prevnar13 on invasive pneumococcal diseases in England and Wales. PLOS ONE 2012;7.
- van Hoek AJ, Choi YH, Trotter C, Miller E, Jit M. The cost-effectiveness of a 13-valent pneumococcal conjugate vaccination for infants in England. Vaccine 2012;30:7205-13.
- Office for National Statistics . Population Estimates for the UK, England and Wales, Scotland and Northern Ireland: Mid-2016 2017. www.ons.gov.uk/peoplepopulationandcommunity/populationandmigration/populationestimates/bulletins/annualmidyearpopulationestimates/mid2016 (accessed 26 August 2018).
- Office for National Statistics . National Life Tables, UK n.d. www.ons.gov.uk/peoplepopulationandcommunity/birthsdeathsandmarriages/lifeexpectancies/bulletins/nationallifetablesunitedkingdom/2014to2016 (accessed 29 August 2018).
- Jit M. The risk of sequelae due to pneumococcal meningitis in high-income countries: a systematic review and meta-analysis. J Infect 2010;61:114-24.
- United Nations Department of Economic and Social Affairs . World Population Prospects 2022 n.d. https://population.un.org/wpp/ (accessed 12 April 2023).
- Oostenbrink R, Moll HA, Essink-Bot ML. The EQ-5D and the Health Utilities Index for permanent sequelae after meningitis: a head-to-head comparison. J Clin Epidemiol 2002;55:791-9.
- Curtis LA. Unit Costs of Health and Social Care 2009. Kent: Personal Social Services Research Unit, University of Kent; 2009.
- Melegaro A, Edmunds WJ. Cost-effectiveness analysis of pneumococcal conjugate vaccination in England and Wales. Vaccine 2004;22:4203-14.
- Voysey M, Sadarangani M, Clutterbuck E, Bolgiano B, Pollard AJ. The impact of administration of conjugate vaccines containing cross reacting material on Haemophilus influenzae type b antibody responses in infants: a systematic review and meta-analysis of randomised controlled trials. Vaccine 2016;34:3986-92.
- Pichichero M, Kaur R, Scott DA, Gruber WC, Trammel J, Almudevar A, et al. Effectiveness of 13-valent pneumococcal conjugate vaccination for protection against acute otitis media caused by Streptococcus pneumoniae in healthy young children: a prospective observational study. Lancet Child Adolesc Health 2018;2:561-8.
- Castañeda-Orjuela C, De la Hoz-Restrepo F. How cost effective is switching universal vaccination from PCV10 to PCV13? A case study from a developing country. Vaccine 2018;36:5766-73.
- Urueña A, Pippo T, Betelu MS, Virgilio F, Giglio N, Gentile A, et al. Cost-effectiveness analysis of the 10- and 13-valent pneumococcal conjugate vaccines in Argentina. Vaccine 2011;29:4963-72.
- Dilokthornsakul P, Kengkla K, Saokaew S, Permsuwan U, Techasaensiri C, Chotpitayasunondh T, et al. An updated cost-effectiveness analysis of pneumococcal conjugate vaccine among children in Thailand. Vaccine 2019;37:4551-60.
- Ferreira DM, Neill DR, Bangert M, Gritzfeld JF, Green N, Wright AKA, et al. Controlled human infection and rechallenge with Streptococcus pneumoniae reveals the protective efficacy of carriage in healthy adults. Am J Respir Crit Care Med 2013;187:855-64.
Appendix 1 Search strategy
MEDLINE (Ovid MEDLINE Epub Ahead of Print, In-Process and Other Non-Indexed Citations, Ovid MEDLINE Daily and Ovid MEDLINE) 1946–present.
Streptococcus pneumoniae/ |
(pneumococc* or s pneumoniae or strep* pneumoniae or strep* p).ti,ab. |
1 or 2 |
vaccines/ or bacterial vaccines/ or streptococcal vaccines/ or Vaccines, Conjugate/ |
(vaccin* or immuni?ation? or immuni?e? or inoculat* or conjugate).ti. |
(conjugate adj2 vaccin*).ti,ab. |
(7 valent or 7valent or seven valent or heptavalent or hepta-valent).ti,ab. |
(9 valent or 9valent or nine valent or nonavalent or nona-valent).ti,ab. |
(10 valent or 10valent or ten valent or decavalent or deca-valent).ti,ab. |
(13 valent or 13valent or thirteen valent).ti,ab. |
4 or 5 or 6 or 7 or 8 or 9 or 10 |
3 and 11 |
exp Pneumococcal Vaccines/ |
((pneumococc* or s pneumoniae or strep* pneumoniae or strep* p) adj5 (vaccin* or immuni?ation? or immuni?e? or inoculat* or conjugate)).ti,ab. |
(pcv7 or pcv 7 or pncrm* or pnccrm* or 7vpnc or 7vcrm).ti,ab. |
(pcv9 or pcv 9).ti,ab. |
(pcv10 or pcv 10 or phidcv or phid cv).ti,ab. |
(pcv13 or pcv 13 or 13vcrm).ti,ab. |
(pneumovax or pneumopur or streptopur or streptorix or prevnar or prevenar or synflorix or gsk 1024850a or gsk1024850a).ti,ab. |
12 or 13 or 14 or 15 or 16 or 17 or 18 or 19 |
exp child/ or infant/ |
(child* or infan* or baby or babies or toddler? or preschool* or pre-school* or p?ediatric?).ti,ab. |
21 or 22 |
20 and 23 |
randomized controlled trial.pt. |
controlled clinical trial.pt. |
randomized.ab. |
placebo.ab. |
drug therapy.fs. |
randomly.ab. |
trial.ab. |
groups.ab. |
25 or 26 or 27 or 28 or 29 or 30 or 31 or 32 |
exp animals/ not humans.sh. |
33 not 34 |
24 and 35 |
limit 24 to (‘reviews (maximizes specificity)’ or ‘systematic review’) |
36 or 37 |
(2019* or 2020* or 2021* or 2022*).ed,ez,yr. |
38 and 39 |
EMBASE 1974–present
Streptococcus pneumoniae/ |
(pneumococc* or s pneumoniae or strep* pneumoniae or strep* p).ti,ab. |
1 or 2 |
vaccine/ or bacterial vaccine/ or streptococcus vaccine/ |
(vaccin* or immuni?ation? or immuni?e? or inoculat* or conjugate).ti. |
(conjugate adj2 vaccin*).ti,ab. |
(7 valent or 7valent or seven valent or heptavalent or hepta-valent).ti,ab. |
(9 valent or 9valent or nine valent or nonavalent or nona-valent).ti,ab. |
(10 valent or 10valent or ten valent or decavalent or deca-valent).ti,ab. |
(13 valent or 13valent or thirteen valent).ti,ab. |
4 or 5 or 6 or 7 or 8 or 9 or 10 |
3 and 11 |
pneumococcus vaccine/ |
((pneumococc* or s pneumoniae or strep* pneumoniae or strep* p) adj5 (vaccin* or immuni?ation? or immuni?e? or inoculat* or conjugate)).ti,ab. |
(pcv7 or pcv 7 or pncrm* or pnccrm* or 7vpnc or 7vcrm).ti,ab. |
(pcv9 or pcv 9).ti,ab. |
(pcv10 or pcv 10 or phidcv or phid cv).ti,ab. |
(pcv13 or pcv 13 or 13vcrm).ti,ab. |
(pneumovax or pneumopur or streptopur or streptorix or prevnar or prevenar or synflorix or gsk 1024850a or gsk1024850a).ti,ab. |
12 or 13 or 14 or 15 or 16 or 17 or 18 or 19 |
exp child/ |
(child* or infan* or baby or babies or toddler? or preschool* or pre-school* or p?ediatric?).ti,ab. |
21 or 22 |
20 and 23 |
randomized controlled trial/ |
single blind procedure/ or double blind procedure/ |
crossover procedure/ |
random*.tw. |
(((singl* or doubl*) adj (blind* or mask*)) or crossover or cross over or factorial* or latin square or assign* or allocat* or volunteer*).ti,ab. |
25 or 26 or 27 or 28 or 29 |
(exp animals/ or nonhuman/) not human/ |
30 not 31 |
24 and 32 |
limit 24 to (‘systematic review’ or ‘reviews (maximizes specificity)’) |
33 or 34 |
(2019* or 2020* or 2021* or 2022*).yr,dd,dc. |
35 and 36 |
Global Health <1973 to 2022 Week 29>
streptococcus pneumoniae/ |
(pneumococc* or s pneumoniae or strep* pneumoniae or strep* p).ti,ab. |
1 or 2 |
vaccines/ or conjugate vaccines/ |
(vaccin* or immuni?ation? or immuni?e? or inoculat* or conjugate).ti. |
(conjugate adj2 vaccin*).ti,ab. |
(7 valent or 7valent or seven valent or heptavalent or hepta-valent).ti,ab. |
(9 valent or 9valent or nine valent or nonavalent or nona-valent).ti,ab. |
(10 valent or 10valent or ten valent or decavalent or deca-valent).ti,ab. |
(13 valent or 13valent or thirteen valent).ti,ab. |
4 or 5 or 6 or 7 or 8 or 9 or 10 |
3 and 11 |
((pneumococc* or s pneumoniae or strep* pneumoniae or strep* p) adj5 (vaccin* or immuni?ation? or immuni?e? or inoculat* or conjugate)).ti,ab. |
(pcv7 or pcv 7 or pncrm* or pnccrm* or 7vpnc or 7vcrm).ti,ab. |
(pcv9 or pcv 9).ti,ab. |
(pcv10 or pcv 10 or phidcv or phid cv).ti,ab. |
(pcv13 or pcv 13 or 13vcrm).ti,ab. |
(pneumovax or pneumopur or streptopur or streptorix or prevnar or prevenar or synflorix or gsk 1024850a or gsk1024850a).ti,ab. |
12 or 13 or 14 or 15 or 16 or 17 or 18 |
exp children/ or infants/ |
(child* or infan* or baby or babies or toddler? or preschool* or pre-school* or p?ediatric?).ti,ab. |
20 or 21 |
19 and 22 |
(random* or blind* or allocat* or assign* or trial* or placebo* or crossover* or cross-over*).mp. |
23 and 24 |
(2019* or 2020* or 2021* or 2022*).yr. |
25 and 26 |
ClinicalTrials.gov – 1 June 2019
(pneumococcal OR pneumococcus OR ‘streptococcus pneumoniae’ OR ‘streptococcal pneumoniae’ OR ‘streptococcus p’ OR ‘streptococcal p’) AND (vaccine OR vaccines OR vaccination OR immunisation OR immunization OR immunise OR immunisation OR inoculate) | Child |
(pneumococcal OR pneumococcus OR ‘streptococcus pneumoniae’ OR ‘streptococcal pneumoniae’ OR ‘streptococcus p’ OR ‘sterptococcal p’) AND (conjugate OR valent) | Child |
pcv7 or pcv 7 or pncrm or pnccrm or 7vpnc or 7vcrm or pcv9 or pcv 9 or pcv10 or pcv 10 or phidcv or phid cv or pcv13 or pcv 13 or 13vcrm |
pneumovax or pneumopur or streptopur or streptorix or prevnar or prevenar or synflorix or gsk 1024850a or gsk1024850a | Child |
ClinicalTrials.gov – 2022 – Added since 1 June 2019 |
Other terms = (pneumococcal OR pneumococcus OR ‘streptococcus pneumoniae’ OR ‘streptococcal pneumoniae’ OR ‘streptococcus p’ OR ‘streptococcal p’) AND (vaccine OR vaccines OR vaccination OR immunisation OR immunization OR immunise OR immunisation OR inoculate) | Child | First posted from 06/01/2019 to 01/01/2024 |
Title = (pneumococcal OR pneumococcus OR ‘streptococcus pneumoniae’ OR ‘streptococcal pneumoniae’ OR ‘streptococcus p’ OR ‘streptococcal p’) AND (vaccine OR vaccines OR vaccination OR immunisation OR immunization OR immunise OR immunisation OR inoculate) | Child | First posted from 06/01/2019 to 01/01/2024 |
Condition = (pneumococcal OR pneumococcus OR ‘streptococcus pneumoniae’ OR ‘streptococcal pneumoniae’ OR ‘streptococcus p’ OR ‘streptococcal p’) AND Intervention = (vaccine OR vaccines OR vaccination OR immunisation OR immunization OR immunise OR immunisation OR inoculate) | Child | First posted from 06/01/2019 to 01/01/2024 |
Other terms = (pneumococcal OR pneumococcus OR ‘streptococcus pneumoniae’ OR ‘streptococcal pneumoniae’ OR ‘streptococcus p’ OR ‘streptococcal p’) AND (conjugate OR valent) | Child |
Title = (pneumococcal OR pneumococcus OR ‘streptococcus pneumoniae’ OR ‘streptococcal pneumoniae’ OR ‘streptococcus p’ OR ‘streptococcal p’) AND (conjugate OR valent) | Child |
Condition = (pneumococcal OR pneumococcus OR ‘streptococcus pneumoniae’ OR ‘streptococcal pneumoniae’ OR ‘streptococcus p’ OR ‘streptococcal p’) AND Intervention = (conjugate OR valent) | Child |
Other terms = pcv7 or pcv 7 or pncrm or pnccrm or 7vpnc or 7vcrm or pcv9 or pcv 9 or pcv10 or pcv 10 or phidcv or phid cv or pcv13 or pcv 13 or 13vcrm | Child |
Title = pcv7 or pcv 7 or pncrm or pnccrm or 7vpnc or 7vcrm or pcv9 or pcv 9 or pcv10 or pcv 10 or phidcv or phid cv or pcv13 or pcv 13 or 13vcrm | Child |
Intervention = pcv7 or pcv 7 or pncrm or pnccrm or 7vpnc or 7vcrm or pcv9 or pcv 9 or pcv10 or pcv 10 or phidcv or phid cv or pcv13 or pcv 13 or 13vcrm | Child |
Other terms = pneumovax or pneumopur or streptopur or streptorix or prevnar or prevenar or synflorix or gsk 1024850a or gsk1024850a | Child |
Title = pneumovax or pneumopur or streptopur or streptorix or prevnar or prevenar or synflorix or gsk 1024850a or gsk1024850a | Child |
Intervention = pneumovax or pneumopur or streptopur or streptorix or prevnar or prevenar or synflorix or gsk 1024850a or | Child |
WHO ICTRP
pneumococcal vaccine OR pneumococcus vaccine OR pneumococcal conjugate OR pneumococcus conjugate OR pcv OR pneumovax OR pneumopur OR streptopur OR streptorix OR prevnar OR prevenar OR synflorix OR gsk 1024850a OR gsk1024850a - TRIALS IN CHILDREN |
Cochrane (CDSR – limited to publication date: 1 June 2019–27 July 2022, CENTRAL – limited to added to database date: 1 June 2019–27 July 2022)
ID | Search |
---|---|
#1 | MeSH descriptor: [Streptococcus pneumoniae] explode all trees |
#2 | (pneumococc* or ‘s pneumoniae’ or ‘streptococcus pneumoniae’ or ‘streptococcus p’ or ‘streptococcal pneumoniae’ or ‘streptococcal p’) |
#3 | #1 or #2 |
#4 | MeSH descriptor: [Vaccines] this term only |
#5 | MeSH descriptor: [Bacterial Vaccines] this term only |
#6 | ((vaccin* or immunization* or immunize* or immunisation* or immunise* or inoculat* or conjugate or valent)):ti,ab,kw |
#7 | #4 or #5 or #6 |
#8 | #3 and #7 |
#9 | MeSH descriptor: [Pneumococcal Vaccines] explode all trees |
#10 | ((pcv7 or ‘pcv 7’ or pncrm* or pnccrm* or 7vpnc or 7vcrm)):ti,ab,kw |
#11 | ((pcv9 or ‘pcv 9’)):ti,ab,kw |
#12 | (pcv10 or‘pcv 10’ or phidcv or ‘phid cv’):ti,ab,kw |
#13 | (pcv13 or ‘pcv 13’ or 13vcrm):ti,ab,kw |
#14 | pneumovax or pneumopur or streptopur or streptorix or prevnar or prevenar or synflorix or gsk 1024850a or gsk1024850a |
#15 | #8 or #9 or #10 or #11 or #13 or #14 |
#16 | MeSH descriptor: [Child] explode all trees |
#17 | MeSH descriptor: [Infant] this term only |
#18 | (child* or infan* or baby or babies or toddler* or preschool* or pre-school* or pediatric* or paediatric*):ti,ab,kw |
#19 | #16 or #17 or #18 |
#20 | #15 and #19 |
Appendix 2 PRISMA flow diagram
FIGURE 19.
PRISMA flow diagram. CDSR, Cochrane Database of Systematic Reviews; CENTRAL, Cochrane Central Register of Controlled Trials; ICTRP, International Clinical Trials Registry Platform.

Appendix 3 Study characteristics
Cohort IDa | Author and yeara | NCT | Individual participant data available/aggregate data | Comparison | Country/region | Continent | Schedule | Schedule primary series | Schedule booster | Co-administered vaccine(s) | Assay |
---|---|---|---|---|---|---|---|---|---|---|---|
121,22 | Bermal et al. 200921 | NCT00344318 NCT00547248 |
Individual | PCV10 vs. PCV7 | Philippines | Asia | 3 + 1 | 6–10–24 weeks | 12–18 months | DTPw-HBV-Hib-TT + OPV | 22F-ELISA |
121,22 | Bermal et al. 200921 | NCT00344318 NCT00547248 |
Individual | PCV10 vs. PCV7 | Poland | Europe | 3 + 1 | 2–4–6 months | 12–18 months | DTPw-HBV-Hib-TT + IPV | 22F-ELISA |
237 | Kim et al. 201137 | NCT00680914 | Individual | PCV10 vs. PCV7 | Korea | Asia | 3 + 1 | 2–4–6 months | 12–18 months | Hib-TT | 22F-ELISA |
339 | Knuf et al. 201239 | NCT00307541 NCT00333450 |
Individual | PCV10 vs. PCV7 | Germany | Europe | 3 + 0 | 2–3–4 months | N/A | DTPa-HBV-Hib-TT/IPV | 22F-ELISA |
453–55 | Prymula et al. 201755 | NCT01204658 | Individual | PCV13 vs. PCV10 | Czech Republic, Germany, Poland and Sweden | Europe | 3 + 1 | 2–3–4 months | 12–15 months | DTPa-HBV-Hib-TT/IPV | 22F-ELISA |
524 | Carmona Martinez et al. 201924 | NCT01616459 | Individual | PCV13 vs. PCV10 | Czech Republic, Germany, Poland and Spain | Europe | 3 + 1 | 2–3–4 months | 12–15 months | DTPa-HBV-Hib-TT/IPV + MenC-TT (SP) | 22F-ELISA |
68,46,47,57,58 | Temple et al. 201957 | NCT01953510 | Individual | PCV13 vs. PCV10 | Vietnam | Asia | 2 + 1 | 2–4 months | 9.5 months | DTPa-HBV-Hib-TT/IPV | Modified third-generation standardised ELISA |
760–62 | van den Bergh et al. 201160 | NCT00652951 | Individual | PCV10 vs. PCV7 | Netherland | Europe | 3 + 1 | 2–3–4 months | 11–13 months | DTPa-(HBV)-Hib-TT/IPV | 22F-ELISA |
864 | Vesikari et al. 200964 | NCT00307554 NCT00370396 |
Individual | PCV10 vs. PCV7 | Finland, France and Poland | Europe | 3 + 1 | 2–3–4 months | 12–18 months | DTPa-(HBV)-Hib-TT/IPV | 22F-ELISA |
966 | Wysocki et al. 200966 | NCT00334334 NCT00463437 |
Individual | PCV10 vs. PCV7 | Germany, Poland and Spain |
Europe | 3 + 1 | 2–4–6 months | 11–18 months | DTPa-(HBV)-Hib-TT/IPV + Hib MenC-TT | 22F-ELISA |
1018 | Amdekar et al. 201318 | NCT00452790 | Individual | PCV13 vs. PCV7 | India | Asia | 3 + 1 | 6–10–14 weeks | 12 months | DTwP-Hib-HBV + OPV | Standardised ELISA |
1126–30,35 | Dagan et al. 201330 | NCT00508742 | Aggregate | PCV13 vs. PCV7 | Israel | Asia | 3 + 1 | 2–4–6 months | 12 months | N/A | Standardised ELISA |
1231 | Esposito et al. 201031 | NCT00366899 | Individual | PCV13 vs. PCV7 | Italy | Europe | 2 + 1 | 3–5 months | 11 months | DTPa-HBV-Hib-TT/IPV | Standardised ELISA |
1333 | Grimprel et al. 201133 | NCT00366678 | Individual | PCV13 vs. PCV7 | France | Europe | 3 + 1 | 2–3–4 months | 12 months | DTPa-Hib-TT/IPV | Standardised ELISA |
1434 | Huang et al. 201234 | NCT00688870 | Individual | PCV13 vs. PCV7 | Taiwan | Asia | 3 + 1 | 2–4–6 months | 15 months | DTPa-(HBV)-Hib-TT/IPV | Standardised ELISA |
1525,32,36 | Kieninger et al. 201036 | NCT00366340 | Individual | PCV13 vs. PCV7 | Germany | Europe | 3 + 1 | 2–3–4 months | 11–12 months | DTPa-HBV-Hib-TT/IPV | Standardised ELISA |
1638,44 | Kim et al. 201338 | NCT00689351 | Individual | PCV13 vs. PCV7 | Korea | Asia | 3 + 1 | 2–4–6 months | 12 months | DTPa-HBV-Hib-TT/IPV | Standardised ELISA |
1751 | Payton et al. 201351 | NCT00444457 | Individual | PCV13 vs. PCV7 | USA | North America | 3 + 1 | 2–4–6 months | 12 months | DTPa-HBV-Hib-TT/IPV | Standardised ELISA |
189,45,52,63 | Pomat et al. 201852 | NCT01619462 | Aggregate | PCV13 vs. PCV10 | Papua New Guinea | Oceania | 3 + 1 | 1–2–3 months | 9 months | DTPw-HBV-Hib-TT + OPV | WHO standardised ELISA |
1956 | Snape et al. 201056 | NCT00384059 | Individual | PCV13 vs. PCV7 | UK | Europe | 2 + 1 | 2–4 months | 12–13 months | DTPa-Hib-TT/IPV/MenC + Hib-MenC-TT | Standardised ELISA |
2059 | Togashi et al. 201559 | NCT01200368 | Individual | PCV13 vs. PCV7 | Japan | Asia | 3 + 1 | enr 3–6 m, 4–8 w int | 12–15 months | DTPa | Standardised ELISA |
2165 | Weckx et al. 201265 | NCT00676091 | Individual | PCV13 vs. PCV7 | Brazil | South America | 3 + 1 | 2–4–6 months | 12 months | HBV-DTwP-Hib/OPV/Rotavirus | Standardised ELISA |
2267 | Yeh et al. 201067 | NCT00373958 | Individual | PCV13 vs. PCV7 | USA | North America | 3 + 1 | 2–4–6 months | 12–15 months | DTPa-HBV-Hib-TT/IPV | Standardised ELISA |
2368,69 | Zhu et al. 201669 | NCT01692886 | Individual | PCV13 vs. PCV7 | China | Asia | 3 + 1 | 3–4–5 months | 12 months | N/A | Standardised ELISA |
2423 | Bryant et al. 201023 | NCT00205803 | Aggregate | PCV13 vs. PCV7 | USA | North America | 3 + 0 | 2–4–6 months | N/A | DTPa-HBV-Hib-TT/IPV | Standardised ELISA |
2549,50 | Odutola et al. 201749 | NCT01262872 | Aggregate | PCV13 vs. PCV10 | Gambia | Africa | 3 + 0 | 2–3–4 months | N/A | DTPw-HBV-Hib-TT + OPV | GlaxoSmithKline in-house ELISA |
2620,40–43 | Leach et al. 202142 | NCT01174849 | Aggregate | PCV13 vs. PCV10 | Australia | Oceania | 3 + 1 | 2–4–6 months | 12 months | DTPa-HBV-Hib-TT/IPV/Rotavirus | Modified third-generation ELISA |
2719,48 | Madhi et al. 202048 | NCT02943902 | Individual | PCV13 vs. PCV10 | South Africa | Africa | 1 + 1 | 6 weeks | 40 weeks | DTPa-HBV-Hib-TT/IPV/Rotavirus/Measles | In-house ELISA according to the standardised WHO protocol |
2719,48 | Madhi et al. 202048 | NCT02943902 | Individual | PCV13 vs. PCV10 | South Africa | Africa | 1 + 1 | 14 weeks | 40 weeks | DTPa-HBV-Hib-TT/IPV/Rotavirus/Measles | In-house ELISA according to the standardised WHO protocol |
2719,48 | Madhi et al. 202048 | NCT02943902 | Individual | PCV13 vs. PCV10 | South Africa | Africa | 1 + 1 | 6–14 weeks | 40 weeks | DTPa-HBV-Hib-TT/IPV/Rotavirus/Measles | In-house ELISA according to the standardised WHO protocol |
2889 | Adigweme et al. 2023 | NCT03896477 | Aggregate | PCV13 vs. PCV10 | Gambia | Africa | 2 + 1 | 6–8 and 14–16 weeks | 9–18 months | DTwP-Hib-HBV/bOPV/Rotavirus | Validated ELISA by the WHO Pneumococcal Serology Reference Laboratory |
Author and yeara | NCT | Comparison |
---|---|---|
Studies with no closed loop in the network | ||
GlaxoSmithKline113 | NCT00169481 | PCV7 vs. PCV11 |
Dagan et al. 199679 | Not found | PCV7 vs. PPV23 |
Greenberg et al. 201880 | NCT01215188 | PCV13 vs. PCV15 |
Rupp et al. 201983 | NCT0251373 NCT02037984 |
PCV13 vs. PCV15 |
Thisyakorn et al. 201487 | NCT00594347 | PCV7 vs. PPV23 |
Martinez et al. 201881 | RPCE00000173 | Cuban PCV7 vs. PCV10 |
Platt et al. 202082 | NCT02987972 | PCV15 vs. PCV13 |
Bili et al. 2021;75 Bili et al. 202390 |
NCT03620162 | PCV15 vs. PCV13 |
Shin et al. 202086 | NCTR20170109002 | PCV12 vs. PCV13 |
Chen et al. 2016;76 Zhao et al. 202288 |
NCT02736240 | PCV7 vs. Chinese PCV13 |
Senders et al. 2021;85 Senders et al. 202084 | NCT03512288 | PCV13 vs. PCV20 |
Banniettis et al. 2021;74 Banniettis et al. 202291 |
NCT03885934 | PCV15 vs. PCV13 |
Lupinacci et al. 2022;92 Lupinacci et al. 202390 |
NCT03893448 | PCV15 vs. PCV13 |
Studies with data unavailable | ||
Martinon-Torres et al. 201272 | NCT00474539 | PCV7 vs. PCV13 |
Vanderkooi et al. 201273 | NCT00475033 | PCV7 vs. PCV13 |
De Los Santos et al. 201770 | NCT01641133 | PCV10 vs. PCV13 |
Diez-Domingo et al. 201371 | NCT00368966 | PCV7 vs. PCV13 |
Clarke et al. 202078,a | NCT02308540 | PCV10-SII vs. PCV13 |
Clarke et al. 202177,a | NCT03197376 | PCV10 vs. PCV10-SII |
Appendix 4 Risk of bias
FIGURE 20.
Assessment of RoB for included studies.

Appendix 5 Heterogeneity and inconsistency assessments
Serotype | Time point | Number of study | Number and proportion of study providing direct evidence | Number of participants providing direct evidence | Number of participants providing indirect evidence | Tau (τ) | I2 (%) | p-value (Q of heterogeneity and inconsistency; df) | p-value (Q of heterogeneity; df) | p-value (Q of inconsistency; df) | |
---|---|---|---|---|---|---|---|---|---|---|---|
4 | Post prime | 28 | 9 | 47.9% | 2356 | 9258 | 0.089 | 86.8 (82.0–90.4) | 0.000 (197.440; 26) | 0.000 (196.733; 25) | 0.400 (0.707; 1) |
6B | Post prime | 28 | 9 | 59.7% | 2356 | 9194 | 0.109 | 82.2 (75.0–87.3) | 0.000 (145.913; 26) | 0.000 (108.043; 25) | 0.000 (37.870; 1) |
9V | Post prime | 28 | 9 | 48.0% | 2360 | 9241 | 0.078 | 83.8 (77.5–88.4) | 0.000 (160.708; 26) | 0.000 (158.678; 25) | 0.154 (2.030; 1) |
14 | Post prime | 28 | 9 | 48.6% | 2358 | 9186 | 0.069 | 71.1 (57.4–80.4) | 0.000 (89.983; 26) | 0.000 (83.435; 25) | 0.010 (6.548; 1) |
18C | Post prime | 28 | 9 | 50.9% | 2358 | 9258 | 0.147 | 93.7 (91.9–95.1) | 0.000 (413.924; 26) | 0.000 (405.782; 25) | 0.004 (8.142; 1) |
19F | Post prime | 28 | 9 | 51.8% | 2358 | 9235 | 0.199 | 96.2 (95.3–96.9) | 0.000 (679.122; 26) | 0.000 (675.147; 25) | 0.046 (3.975; 1) |
23F | Post prime | 28 | 9 | 55.1% | 2358 | 9211 | 0.095 | 80.6 (72.5–86.3) | 0.000 (133.979; 26) | 0.000 (131.123; 25) | 0.091 (2.856; 1) |
1 | Post prime | 9 | 9 | 100% | 2357 | 0.177 | 93.8 (90.4–96.1) | 0.000 (130.029; 8) | 0.000 (130.029; 8) | ||
5 | Post prime | 9 | 9 | 100% | 2356 | 0.193 | 95.1 (92.5–96.7) | 0.000 (161.837; 8) | 0.000 (161.837; 8) | ||
7F | Post prime | 9 | 9 | 100% | 2358 | 0.056 | 63.6 (25.3–82.3) | 0.005 (21.980; 8) | 0.005 (21.980; 8) | ||
3 | Post prime | 9 | 9 | 100% | 2354 | 0.495 | 99.2 (99.0–99.4) | 0.000 (1006.362; 8) | 0.000 (1006.362; 8) | ||
6A | Post prime | 9 | 9 | 100% | 2354 | 0.514 | 99.0 (98.8–99.2) | 0.000 (825.778; 8) | 0.000 (825.778; 8) | ||
19A | Post prime | 9 | 9 | 100% | 2356 | 0.381 | 98.1 (97.5–98.6) | 0.000 (428.259; 8) | 0.000 (428.259; 8) | ||
4 | Prior booster | 17 | 7 | 68.9% | 1816 | 4174 | 0.098 | 83.7 (74.8–89.4) | 0.000 (92.071; 15) | 0.000 (90.267; 14) | 0.179 (1.805; 1) |
6B | Prior booster | 18 | 8 | 78.7% | 2220 | 4140 | 0.161 | 90.8 (86.8–93.6) | 0.000 (173.775; 16) | 0.000 (171.746; 15) | 0.154 (2.029; 1) |
9V | Prior booster | 18 | 8 | 72.7% | 2218 | 4175 | 0.108 | 87.9 (82.2–91.8) | 0.000 (132.395; 16) | 0.000 (131.952; 15) | 0.506 (0.443; 1) |
14 | Prior booster | 18 | 8 | 69.3% | 2224 | 4178 | 0.082 | 70.1 (50.9–81.8) | 0.000 (53.432; 16) | 0.000 (53.136; 15) | 0.586 (0.296; 1) |
18C | Prior booster | 17 | 7 | 65.6% | 1806 | 4177 | 0.089 | 82.0 (71.8–88.5) | 0.000 (83.259; 15) | 0.000 (68.689; 14) | 0.000 (14.571; 1) |
19F | Prior booster | 18 | 8 | 82.0% | 2215 | 4151 | 0.12 | 83.2 (74.3–89.0) | 0.000 (95.125; 16) | 0.000 (69.247; 15) | 0.000 (25.878; 1) |
23F | Prior booster | 18 | 8 | 76.9% | 2216 | 4170 | 0.115 | 82.8 (73.5–88.8) | 0.000 (92.759; 16) | 0.000 (92.450; 15) | 0.578 (0.309; 1) |
1 | Prior booster | 8 | 8 | 100.0% | 2228 | 0.127 | 90.1 (83.0–94.3) | 0.000 (71.032; 7) | 0.000 (71.032) | ||
5 | Prior booster | 8 | 8 | 100.0% | 2221 | 0.077 | 77.2 (54.7–88.5) | 0.000 (30.664; 7) | 0.000 (30.664) | ||
7F | Prior booster | 8 | 8 | 100.0% | 2220 | 0.083 | 79.4 (59.8–89.4) | 0.000 (33.968; 7) | 0.000 (33.968) | ||
3 | Prior booster | 7 | 7 | 100.0% | 1813 | 0.236 | 95.0 (91.8–96.9) | 0.000 (119.248; 6) | 0.000 (119.248) | ||
6A | Prior booster | 8 | 8 | 100.0% | 2213 | 0.169 | 92.2 (87.0–95.3) | 0.000 (89.441; 7) | 0.000 (89.441) | ||
19A | Prior booster | 8 | 8 | 100.0% | 2219 | 0.132 | 84.8 (71.9–91.8) | 0.000 (46.063; 7) | 0.000 (46.063) | ||
4 | Post booster | 25 | 6 | 53.1% | 1706 | 8457 | 0.063 | 72.9 (59.3–81.9) | 0.000 (84.813; 23) | 0.000 (75.091; 22) | 0.002 (9.722; 1) |
6B | Post booster | 26 | 7 | 60.0% | 2102 | 8434 | 0.11 | 86.5 (81.3–90.3) | 0.000 (178.002; 24) | 0.000 (176.999; 23) | 0.317 (1.003; 1) |
9V | Post booster | 26 | 7 | 57.9% | 2101 | 8457 | 0.076 | 82.5 (75.1–87.7) |
0.000 (136.818; 24) | 0.000 (135.005; 23) | 0.178 (1.812; 1) |
14 | Post booster | 26 | 7 | 51.0% | 2100 | 8446 | 0.063 | 71.7 (57.8–81.1) | 0.000 (84.914; 24) | 0.000 (84.381; 23) | 0.466 (0.532; 1) |
18C | Post booster | 25 | 6 | 54.0% | 1705 | 8453 | 0.074 | 79.6 (70.3–86.0) | 0.000 (112.643; 23) | 0.000 (112.118; 22) | 0.469 (0.525; 1) |
19F | Post booster | 26 | 7 | 58.1% | 2102 | 8443 | 0.111 | 87.4 (82.6–90.8) | 0.000 (190.038; 24) | 0.000 (186.332; 23) | 0.054 (3.706; 1) |
23F | Post booster | 26 | 7 | 64.3% | 2100 | 8437 | 0.08 | 76.4 (65.5–83.9) | 0.000 (101.839; 24) | 0.000 (101.839; 23) | 1.000 (0.000; 1) |
1 | Post booster | 7 | 7 | 100.0% | 2100 | 0.048 | 54.6 (0.0–80.6) | 0.040 (13.216; 6) | 0.040 (13.216) | ||
5 | Post booster | 7 | 7 | 100.0% | 2101 | 0.092 | 83.4 (67.2–91.6) | 0.000 (36.074; 6) | 0.000 (36.074) | ||
7F | Post booster | 7 | 7 | 100.0% | 2100 | 0.055 | 70.0 (34.2–86.3) | 0.003 (19.981; 6) | 0.003 (19.981) | ||
3 | Post booster | 6 | 6 | 100.0% | 1701 | 0.299 | 96.9 (95.2–98.0) | 0.000 (162.413; 5) | 0.000 (162.413) | ||
6A | Post booster | 7 | 7 | 100.0% | 2099 | 0.232 | 94.2 (90.4–96.5) | 0.000 (103.568; 6) | 0.000 (103.568) | ||
19A | Post booster | 7 | 7 | 100.0% | 2100 | 0.147 | 86.4 (74.0–92.8) | 0.000 (43.957; 6) | 0.000 (43.957) |
Serotype | Number of study | Number and proportion of study providing direct evidence | Number of participants providing direct evidence | Number of participants providing indirect evidence | tau | I2 (%) | p-value (Q of heterogeneity and inconsistency; df) | p-value (Q of heterogeneity; df) | p-value (Q of inconsistency; df) | |
---|---|---|---|---|---|---|---|---|---|---|
4 | 15 | 6 | 89.0% | 1577 | 3531 | 0.383 | 23.7 (0–59.5) | 0.198 (17.036; 13) | 0.178 (16.286; 12) | 0.386 (0.750; 1) |
6B | 15 | 6 | 91.6% | 1573 | 3466 | 0.249 | 74.4 (56.7–84.9) | 0.000 (50.756; 13) | 0.000 (48.136; 12) | 0.105 (2.621; 1) |
9V | 15 | 6 | 90.7% | 1574 | 3518 | 0.309 | 43.0 (0–69.6) | 0.044 (22.801; 13) | 0.049 (21.065; 12) | 0.188 (1.736; 1) |
14 | 15 | 6 | 81.3% | 1578 | 3524 | 0.361 | 69.6 (47.4–82.5) | 0.000 (42.834; 13) | 0.000 (40.642; 12) | 0.139 (2.192; 1) |
18C | 15 | 6 | 95.1% | 1568 | 3537 | 0.474 | 49.9 (7.4–72.9) | 0.017 (25.961; 13) | 0.017 (24.657; 12) | 0.254 (1.304; 1) |
19F | 15 | 6 | 80.2% | 1569 | 3497 | 0.327 | 49.1 (5.7–72.5) | 0.020 (25.541; 13) | 0.104 (18.415; 12) | 0.008 (7.126; 1) |
23F | 15 | 6 | 93.6% | 1570 | 3498 | 0.557 | 83.8 (74.3–89.9) | 0.000 (80.480; 13) | 0.000 (78.982; 12) | 0.221 (1.498; 1) |
1 | 6 | 6 | 100% | 1581 | 0.403 | 42.7 (0–77.3) | 0.121 (8.724; 5) | 0.121 (8.724; 5) | ||
5 | 6 | 6 | 100% | 1573 | 0.975 | 82.2 (62.1–91.6) | 0.000 (28.031; 5) | 0.000 (28.031; 5) | ||
7F | 6 | 6 | 100% | 1575 | 0.000 | 0 (0–74.6) | 0.537 (4.084; 5) | 0.537 (4.084; 5) |
FIGURE 21.
Trial-level GMRs for serotype 4 post-primary vaccination series. Each solid line in the figure shows the GMR from each trial. Blue boxes and lines show the point estimates and CIs for GMRs comparing vac1 vs. vac2. Concomitant vaccines are vaccines co-administered with PCV primary vaccine series. Information on co-administered vaccine is not always available (e.g. study ID 11 and 23). Concomitant vaccines in the bracket are those administered in some but not all of the study sites. enr 3–6 m, 4–8 w int, enrolment at 3–6 months of age and at 4–8 weeks interval of three doses primary vaccines in total; N/A, not applicable.

FIGURE 22.
Trial-level GMRs for serotype 6B post-primary vaccination series. Each solid line in the figure shows the GMR from each trial. Blue boxes and lines show the point estimates and CIs for GMRs comparing vac1 vs. vac2. Concomitant vaccines are vaccines co-administered with PCV primary vaccine series. Information on co-administered vaccine is not always available (e.g. study ID 11 and 23). Concomitant vaccines in the bracket are those administered in some but not all of the study sites. enr 3–6 m, 4–8 w int, enrolment at 3–6 months of age and at 4–8 weeks interval of three doses primary vaccines in total; N/A, not applicable.
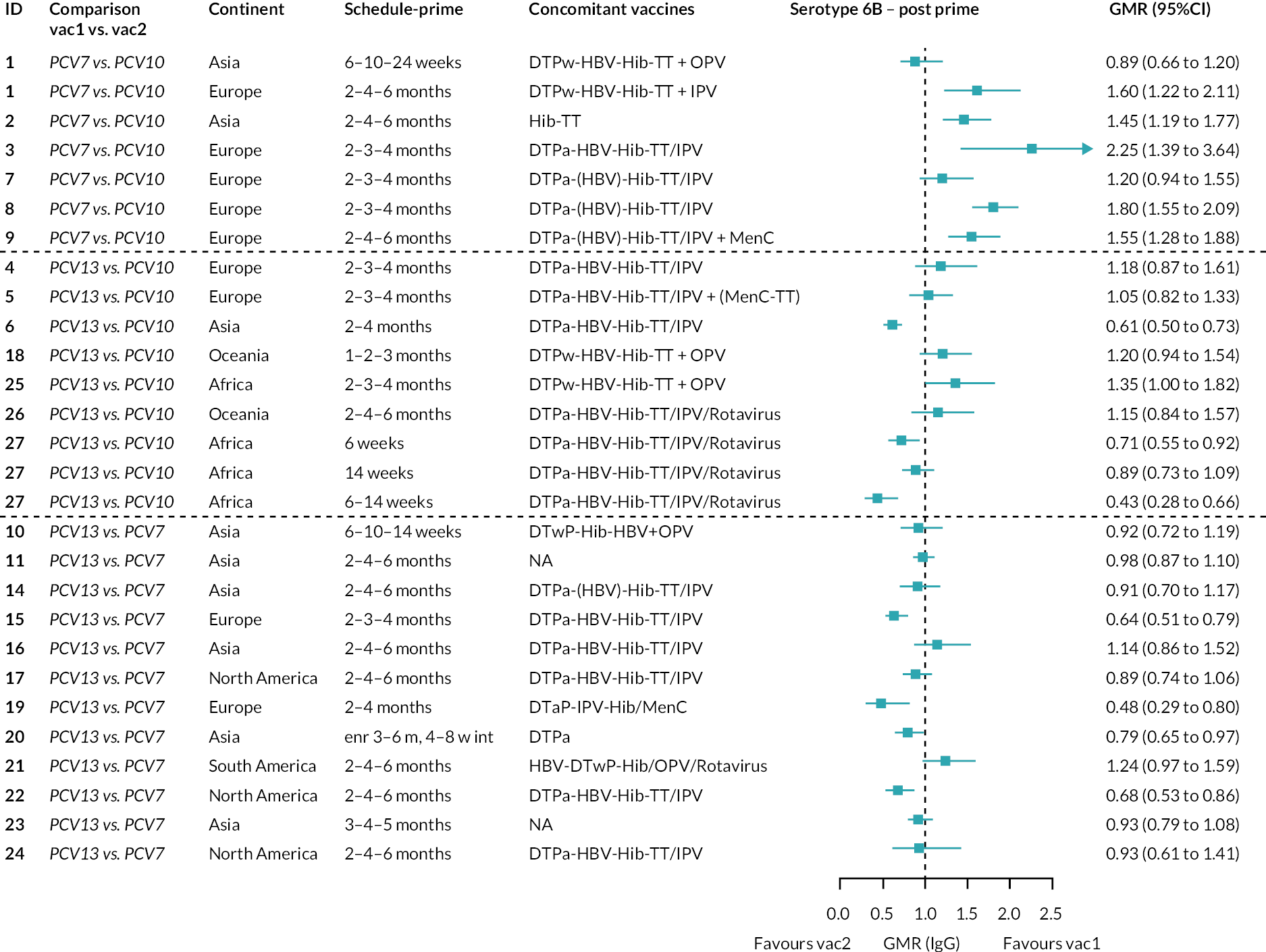
FIGURE 23.
Trial-level GMRs for serotype 9V post-primary vaccination series. Each solid line in the figure shows the GMR from each trial. Blue boxes and lines show the point estimates and CIs for GMRs comparing vac1 vs. vac2. Concomitant vaccines are vaccines co-administered with PCV primary vaccine series. Information on co-administered vaccine is not always available (e.g. study ID 11 and 23). Concomitant vaccines in the bracket are those administered in some but not all of the study sites. enr 3–6 m, 4–8 w int, enrolment at 3–6 months of age and at 4–8 weeks interval of three doses primary vaccines in total; N/A, not applicable.

FIGURE 24.
Trial-level GMRs for serotype 14 post-primary vaccination series. Each solid line in the figure shows the GMR from each trial. Blue boxes and lines show the point estimates and CIs for GMRs comparing vac1 vs. vac2. Concomitant vaccines are vaccines co-administered with PCV primary vaccine series. Information on co-administered vaccine is not always available (e.g. study ID 11 and 23). Concomitant vaccines in the bracket are those administered in some but not all of the study sites. enr 3–6 m, 4–8 w int, enrolment at 3–6 months of age, and at 4–8 weeks interval of three doses primary vaccines in total; N/A, not applicable.

FIGURE 25.
Trial-level GMRs for serotype 18C post-primary vaccination series. Each solid line in the figure shows the GMR from each trial. Blue boxes and lines show the point estimates and CIs for GMRs comparing vac1 vs. vac2. Concomitant vaccines are vaccines co-administered with PCV primary vaccine series. Information on co-administered vaccine is not always available (e.g. study ID 11 and 23). Concomitant vaccines in the bracket are those administered in some but not all of the study sites. enr 3–6 m, 4–8 w int, enrolment at 3–6 months of age and at 4–8 weeks interval of three doses primary vaccines in total; N/A, not applicable.

FIGURE 26.
Trial-level GMRs for serotype 19F post-primary vaccination series. Each solid line in the figure shows the GMR from each trial. Blue boxes and lines show the point estimates and CIs for GMRs comparing vac1 vs. vac2. Concomitant vaccines are vaccines co-administered with PCV primary vaccine series. Information on co-administered vaccine is not always available (e.g. study ID 11 and 23). Concomitant vaccines in the bracket are those administered in some but not all of the study sites. enr 3–6 m, 4–8 w int, enrolment at 3–6 months of age and at 4–8 weeks interval of three doses primary vaccines in total; N/A, not applicable.

FIGURE 27.
Trial-level GMRs for serotype 23F post-primary vaccination series. Each solid line in the figure shows the GMR from each trial. Blue boxes and lines show the point estimates and CIs for GMRs comparing vac1 vs. vac2. Concomitant vaccines are vaccines co-administered with PCV primary vaccine series. Information on co-administered vaccine is not always available (e.g. study ID 11 and 23). Concomitant vaccines in the bracket are those administered in some but not all of the study sites. enr 3–6 m, 4–8 w int, enrolment at 3–6 months of age and at 4–8 weeks interval of three doses primary vaccines in total; N/A, not applicable.

FIGURE 28.
Trial-level GMRs for serotype 1 post-primary vaccination series. Each solid line in the figure shows the GMR from each trial. Blue boxes and lines show the point estimates and CIs for GMRs comparing vac1 vs. vac2. Concomitant vaccines are vaccines co-administered with PCV primary vaccine series. Information on co-administered vaccine is not always available. Concomitant vaccines in the bracket are those administered in some but not all of the study sites. enr 3–6 m, 4–8 w int, enrolment at 3–6 months of age and at 4–8 weeks interval of three doses primary vaccines in total; N/A, not applicable.
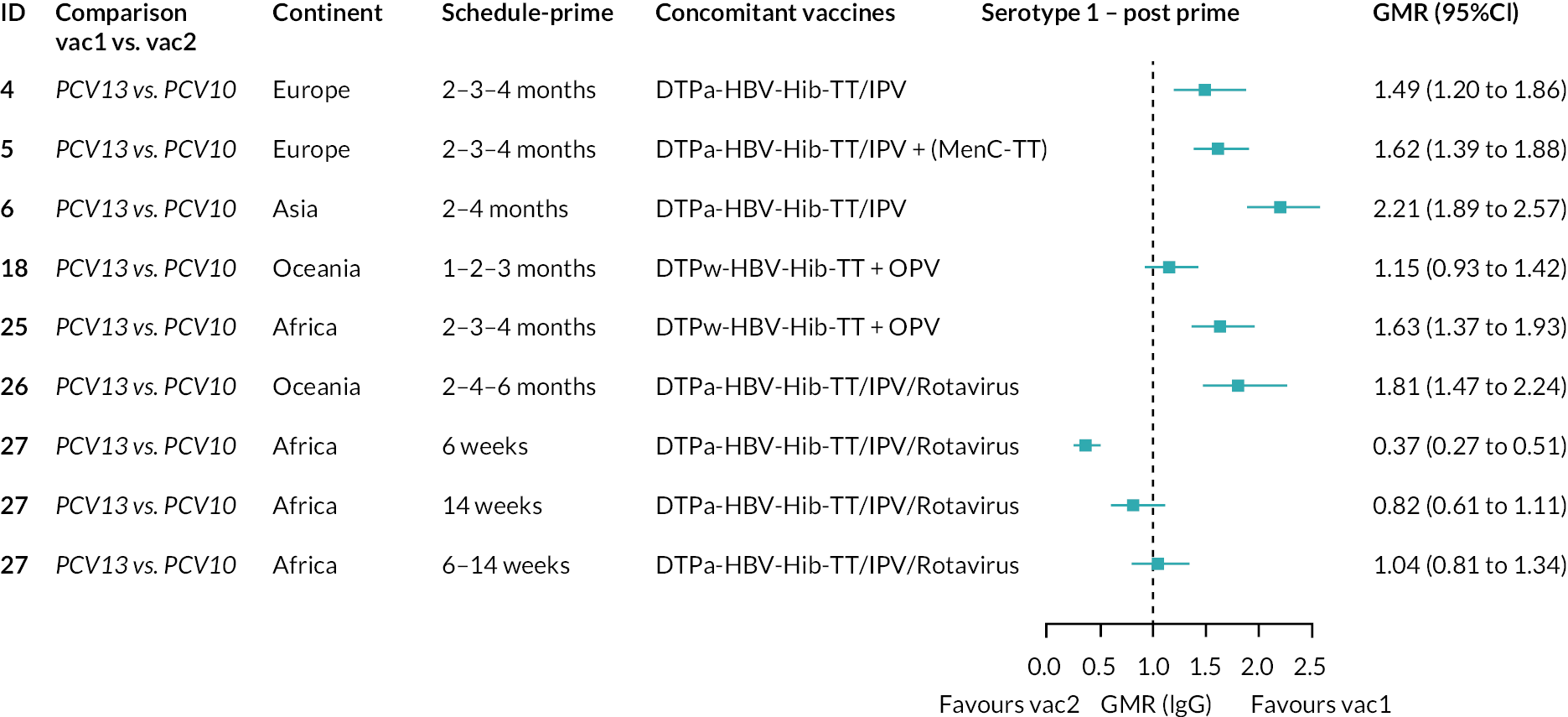
FIGURE 29.
Trial-level GMRs for serotype 5 post-primary vaccination series. Each solid line in the figure shows the GMR from each trial. Blue boxes and lines show the point estimates and CIs for GMRs comparing vac1 vs. vac2. Concomitant vaccines are vaccines co-administered with PCV primary vaccine series. Information on co-administered vaccine is not always available. Concomitant vaccines in the bracket are those administered in some but not all of the study sites. enr 3–6 m, 4–8 w int, enrolment at 3–6 months of age and at 4–8 weeks interval of three doses primary vaccines in total; N/A, not applicable.

FIGURE 30.
Trial-level GMRs for serotype 7F post-primary vaccination series. Each solid line in the figure shows the GMR from each trial. Blue boxes and lines show the point estimates and CIs for GMRs comparing vac1 vs. vac2. Concomitantvaccines are vaccines co-administered with PCV primary vaccine series. Information on co-administered vaccine is notalways available. Concomitant vaccines in the bracket are those administered in some but not all of the study sites. enr 3–6 m, 4–8 w int, enrolment at 3–6 months of age and at 4–8 weeks interval of three doses primary vaccines in total; N/A, not applicable.

FIGURE 31.
Trial-level GMRs for serotype 3 post-primary vaccination series. Each solid line in the figure shows the GMR from each trial. Blue boxes and lines show the point estimates and CIs for GMRs comparing vac1 vs. vac2. Concomitant vaccines are vaccines co-administered with PCV primary vaccine series. Information on co-administered vaccine is not always available. Concomitant vaccines in the bracket are those administered in some but not all of the study sites. enr 3–6 m, 4–8 w int, enrolment at 3–6 months of age and at 4–8 weeks interval of three doses primary vaccines in total; N/A, not applicable.
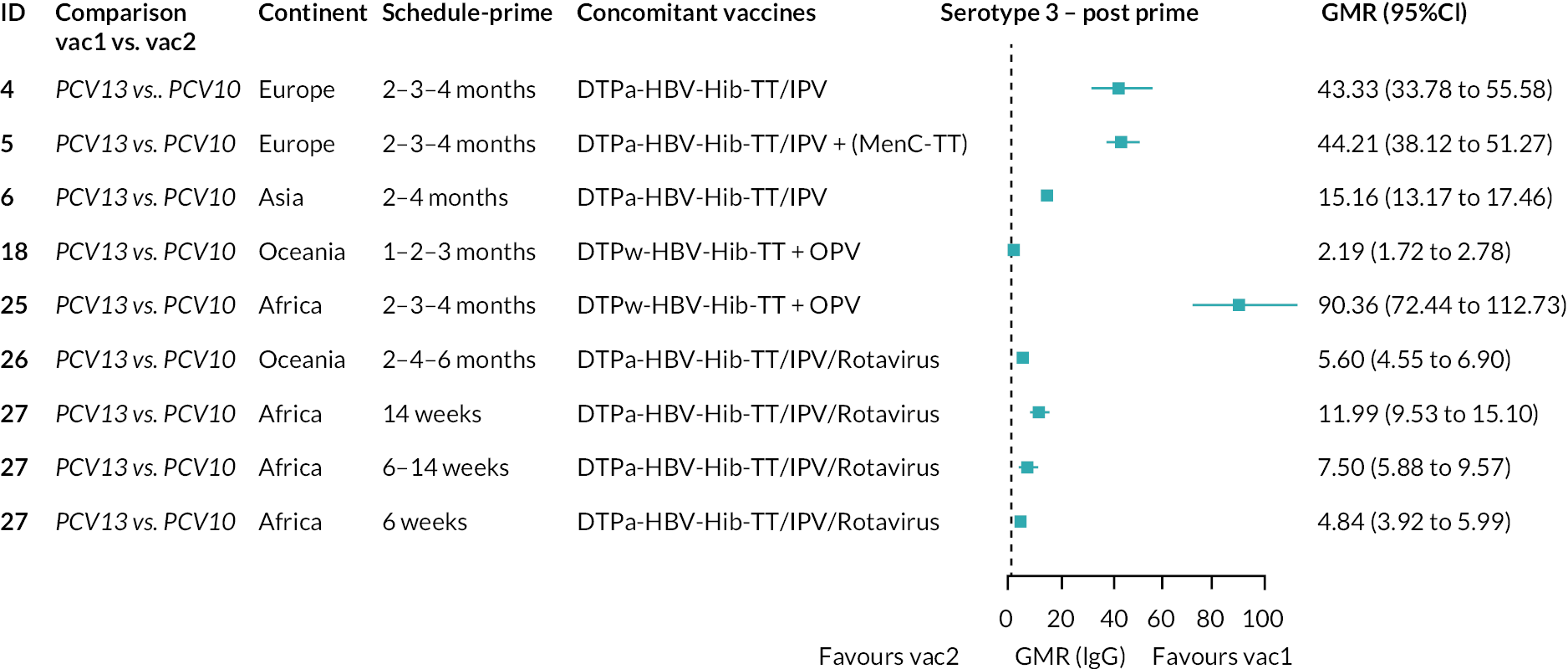
FIGURE 32.
Trial-level GMRs for serotype 6A post-primary vaccination series. Each solid line in the figure shows the GMR from each trial. Blue boxes and lines show the point estimates and CIs for GMRs comparing vac1 vs. vac2. Concomitant vaccines are vaccines co-administered with PCV primary vaccine series. Information on co-administered vaccine is not always available. Concomitant vaccines in the bracket are those administered in some but not all of the study sites. enr 3–6 m, 4–8 w int, enrolment at 3–6 months of age and at 4–8 weeks interval of three doses primary vaccines in total; N/A, not applicable.
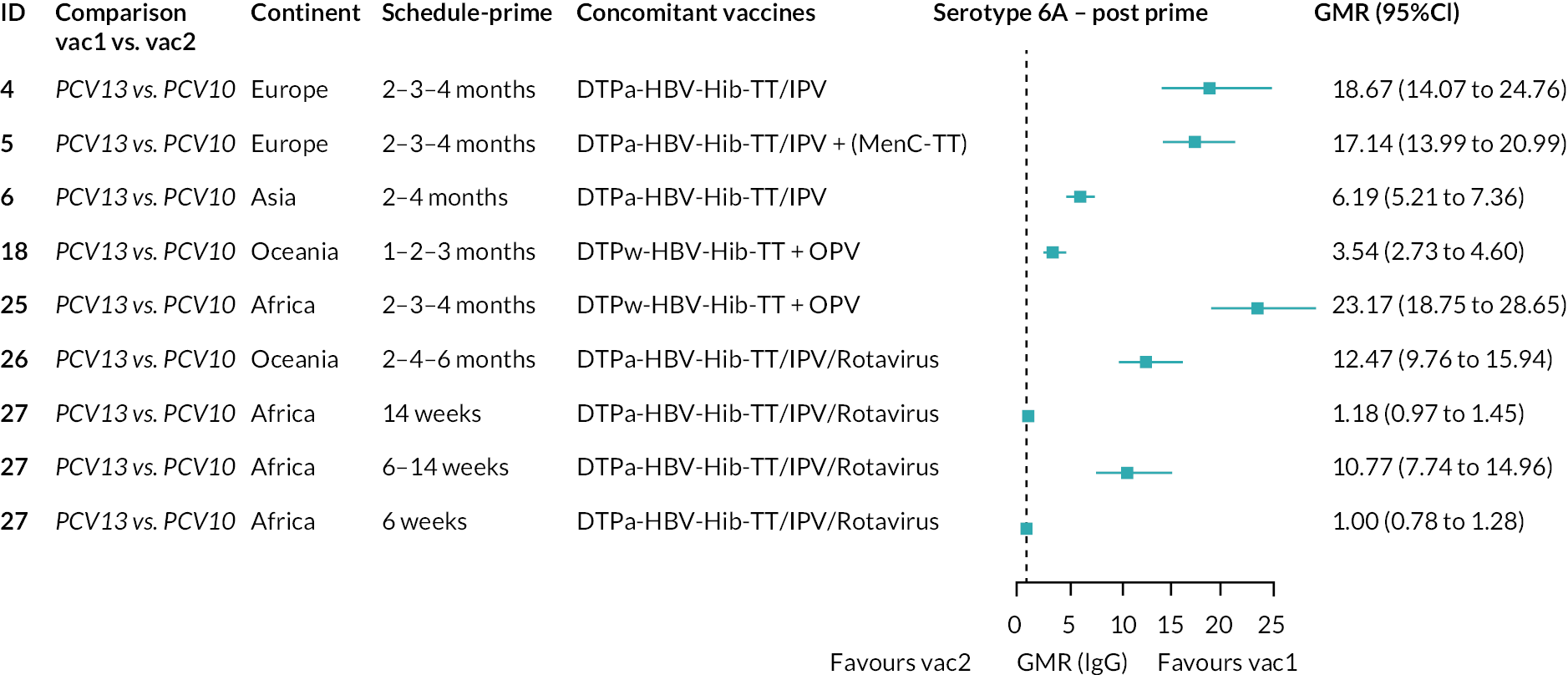
FIGURE 33.
Trial-level GMRs for serotype 19A post-primary vaccination series. Each solid line in the figure shows the GMR from each trial. Blue boxes and lines show the point estimates and CIs for GMRs comparing vac1 vs. vac2. Concomitant vaccines are vaccines co-administered with PCV primary vaccine series. Information on co-administered vaccine is not always available. Concomitant vaccines in the bracket are those administered in some but not all of the study sites. enr 3–6 m, 4–8 w int, enrolment at 3–6 months of age and at 4–8 weeks interval of three doses primary vaccines in total; N/A, not applicable.

FIGURE 34.
Trial-level GMRs for serotype 4 pre booster. Each solid line in the figure shows the GMR from each trial. Blue boxes and lines show the point estimates and CIs for GMRs comparing vac1 vs. vac2. Concomitant vaccines are vaccines co-administered with PCV primary vaccine series. Information on co-administered vaccine is not always available. Concomitant vaccines in the bracket are those administered in some but not all of the study sites. enr 3–6 m, 4–8 w int, enrolment at 3–6 months of age and at 4–8 weeks interval of three doses primary vaccines in total; N/A, not applicable.

FIGURE 35.
Trial-level GMRs for serotype 6B pre booster. Each solid line in the figure shows the GMR from each trial. Blue boxes and lines show the point estimates and CIs for GMRs comparing vac1 vs. vac2. Concomitant vaccines are vaccines co-administered with PCV primary vaccine series. Information on co-administered vaccine is not always available. Concomitant vaccines in the bracket are those administered in some but not all of the study sites. bOPV, bivalent oral poliovirus vaccine; enr 3–6 m, 4–8 w int, enrolment at 3–6 months of age and at 4–8 weeks interval of three doses primary vaccines in total; MR, measles and rubella combined vaccine; N/A, not applicable; YF, yellow fever vaccine.

FIGURE 36.
Trial-level GMRs for serotype 9V pre booster. Each solid line in the figure shows the GMR from each trial. Blue boxes and lines show the point estimates and CIs for GMRs comparing vac1 vs. vac2. Concomitant vaccines are vaccines co-administered with PCV primary vaccine series. Information on co-administered vaccine is not always available. Concomitant vaccines in the bracket are those administered in some but not all of the study sites. bOPV, bivalent oral poliovirus vaccine; enr 3–6 m, 4–8 w int, enrolment at 3–6 months of age and at 4–8 weeks interval of three doses primary vaccines in total; MR, measles and rubella combined vaccine; N/A, not applicable; YF, yellow fever vaccine.

FIGURE 37.
Trial-level GMRs for serotype 14 pre booster. Each solid line in the figure shows the GMR from each trial. Blue boxes and lines show the point estimates and CIs for GMRs comparing vac1 vs. vac2. Concomitant vaccines are vaccines co-administered with PCV primary vaccine series. Information on co-administered vaccine is not always available. Concomitant vaccines in the bracket are those administered in some but not all of the study sites. bOPV, bivalent oral poliovirus vaccine; enr 3–6 m, 4–8 w int, enrolment at 3–6 months of age and at 4–8 weeks interval of three doses primary vaccines in total; MR, measles and rubella combined vaccine; N/A, not applicable; YF, yellow fever vaccine.
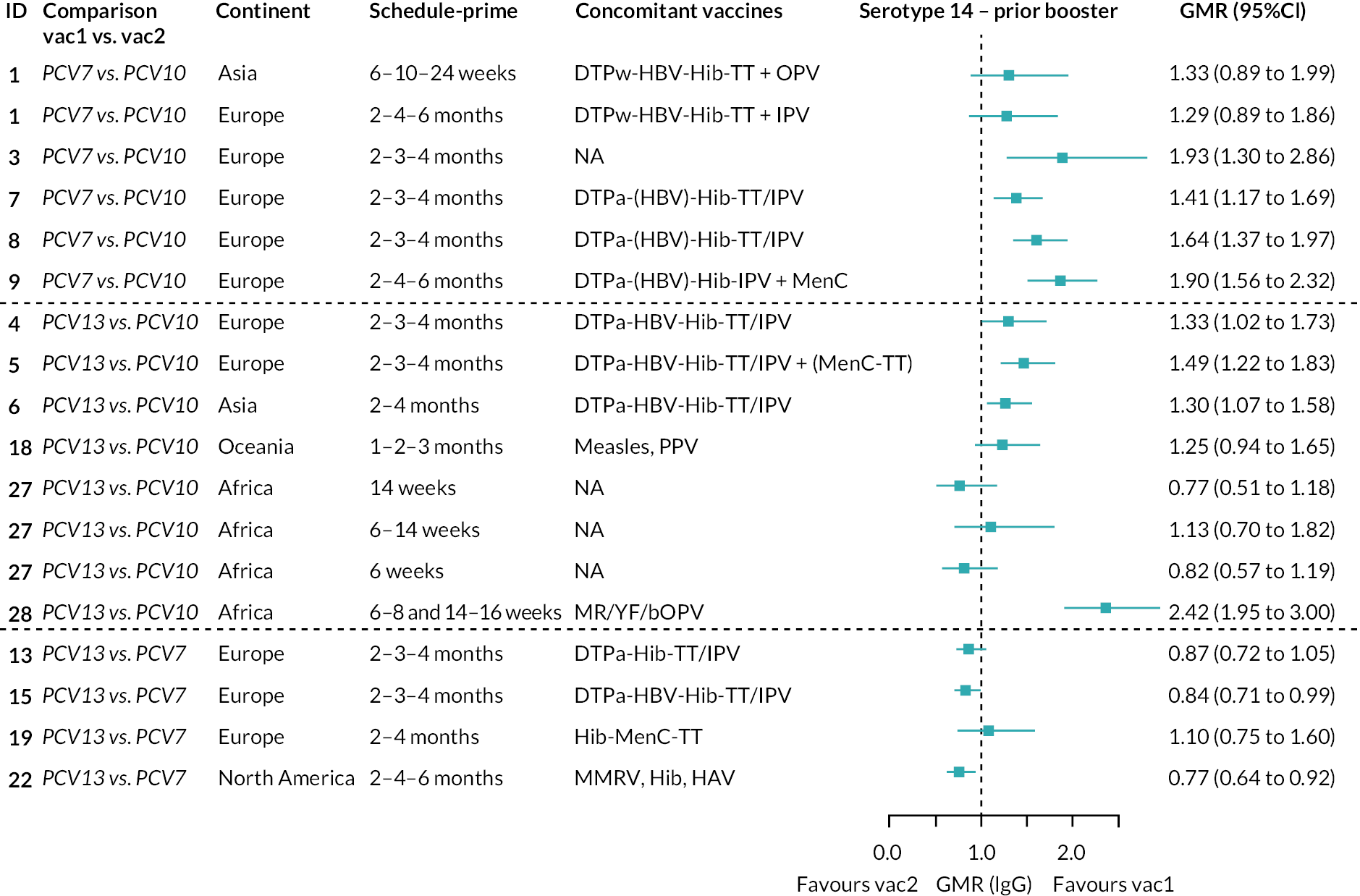
FIGURE 38.
Trial-level GMRs for serotype 18C pre booster. Each solid line in the figure shows the GMR from each trial. Blue boxes and lines show the point estimates and CIs for GMRs comparing vac1 vs. vac2. Concomitant vaccines are vaccines co-administered with PCV primary vaccine series. Information on co-administered vaccine is not always available. Concomitant vaccines in the bracket are those administered in some but not all of the study sites. enr 3–6 m, 4–8 w int, enrolment at 3–6 months of age and at 4–8 weeks interval of three doses primary vaccines in total; N/A, not applicable.

FIGURE 39.
Trial-level GMRs for serotype 19F pre booster. Each solid line in the figure shows the GMR from each trial. Blue boxes and lines show the point estimates and CIs for GMRs comparing vac1 vs. vac2. Concomitant vaccines are vaccines co-administered with PCV primary vaccine series. Information on co-administered vaccine is not always available. Concomitant vaccines in the bracket are those administered in some but not all of the study sites. bOPV, bivalent oral poliovirus vaccine; enr 3–6 m, 4–8 w int, enrolment at 3–6 months of age and at 4–8 weeks interval of three doses primary vaccines in total; MR, measles and rubella combined vaccine; N/A, not applicable; YF, yellow fever vaccine.

FIGURE 40.
Trial-level GMRs for serotype 23F pre booster. Each solid line in the figure shows the GMR from each trial. Blue boxes and lines show the point estimates and CIs for GMRs comparing vac1 vs. vac2. Concomitant vaccines are vaccines co-administered with PCV primary vaccine series. Information on co-administered vaccine is not always available. Concomitant vaccines in the bracket are those administered in some but not all of the study sites. bOPV, bivalent oral poliovirus vaccine; enr 3–6 m, 4–8 w int, enrolment at 3–6 months of age and at 4–8 weeks interval of three doses primary vaccines in total; MR, measles and rubella combined vaccine; N/A, not applicable; YF, yellow fever vaccine.
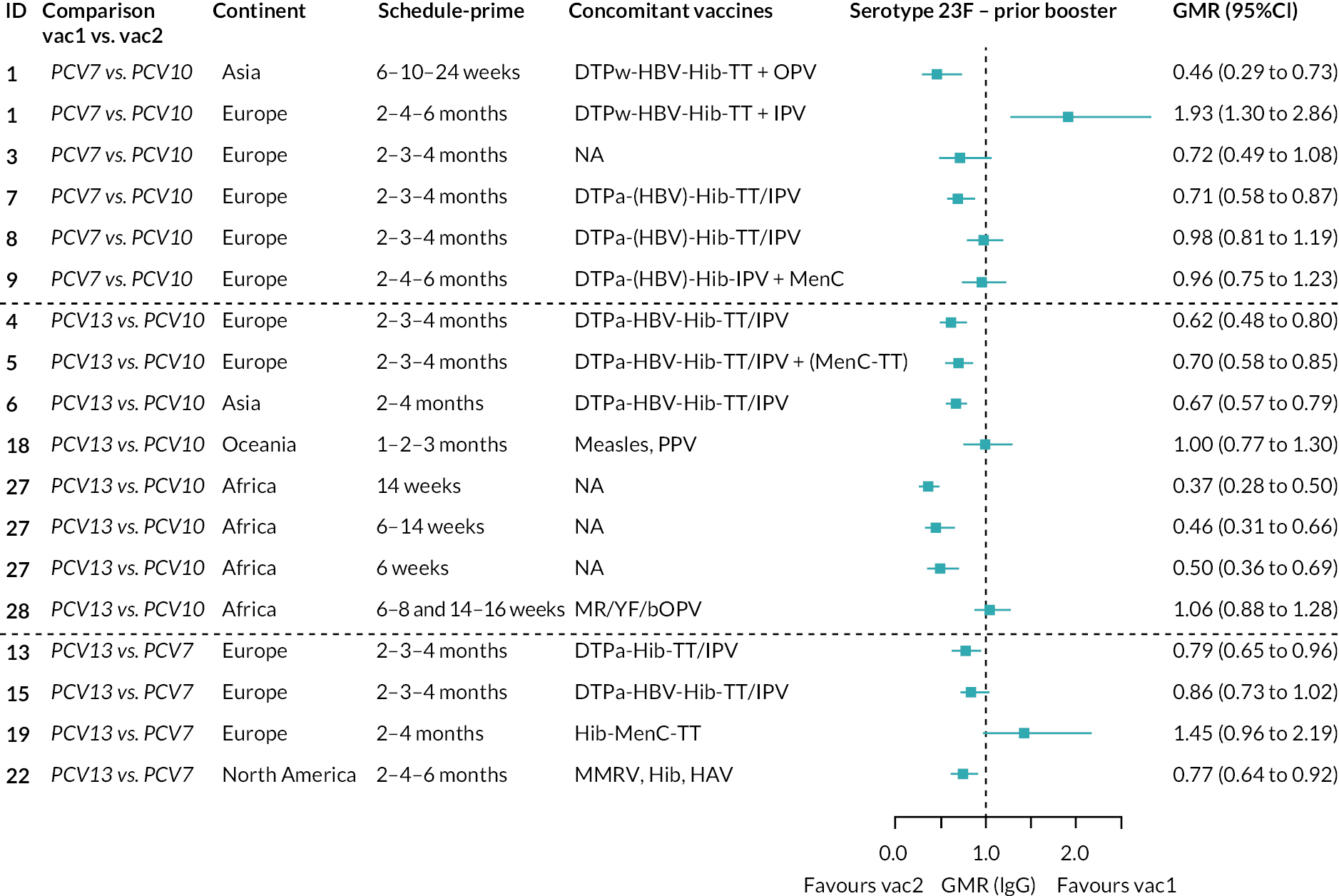
FIGURE 41.
Trial-level GMRs for serotype 1 pre booster. Each solid line in the figure shows the GMR from each trial. Blue boxes and lines show the point estimates and CIs for GMRs comparing vac1 vs. vac2. Concomitant vaccines are vaccines co-administered with PCV primary vaccine series. Information on co-administered vaccine is not always available. Concomitant vaccines in the bracket are those administered in some but not all of the study sites. bOPV, bivalent oral poliovirus vaccine; enr 3–6 m, 4–8 w int, enrolment at 3–6 months of age and at 4–8 weeks interval of three doses primary vaccines in total; MR, measles and rubella combined vaccine; N/A, not applicable; YF, yellow fever vaccine.

FIGURE 42.
Trial-level GMRs for serotype 5 pre booster. Each solid line in the figure shows the GMR from each trial. Blue boxes and lines show the point estimates and CIs for GMRs comparing vac1 vs. vac2. Concomitant vaccines are vaccines co-administered with PCV primary vaccine series. Information on co-administered vaccine is not always available. Concomitant vaccines in the bracket are those administered in some but not all of the study sites. bOPV, bivalent oral poliovirus vaccine; enr 3–6 m, 4–8 w int, enrolment at 3–6 months of age and at 4–8 weeks interval of three doses primary vaccines in total; MR, measles and rubella combined vaccine; N/A, not applicable; YF, yellow fever vaccine.

FIGURE 43.
Trial-level GMRs for serotype 7F pre booster. Each solid line in the figure shows the GMR from each trial. Blue boxes and lines show the point estimates and CIs for GMRs comparing vac1 vs. vac2. Concomitant vaccines are vaccines co-administered with PCV primary vaccine series. Information on co-administered vaccine is not always available. Concomitant vaccines in the bracket are those administered in some but not all of the study sites. bOPV, bivalent oral poliovirus vaccine; enr 3–6 m, 4–8 w int, enrolment at 3–6 months of age and at 4–8 weeks interval of three doses primary vaccines in total; MR, measles and rubella combined vaccine; N/A, not applicable; YF, yellow fever vaccine.
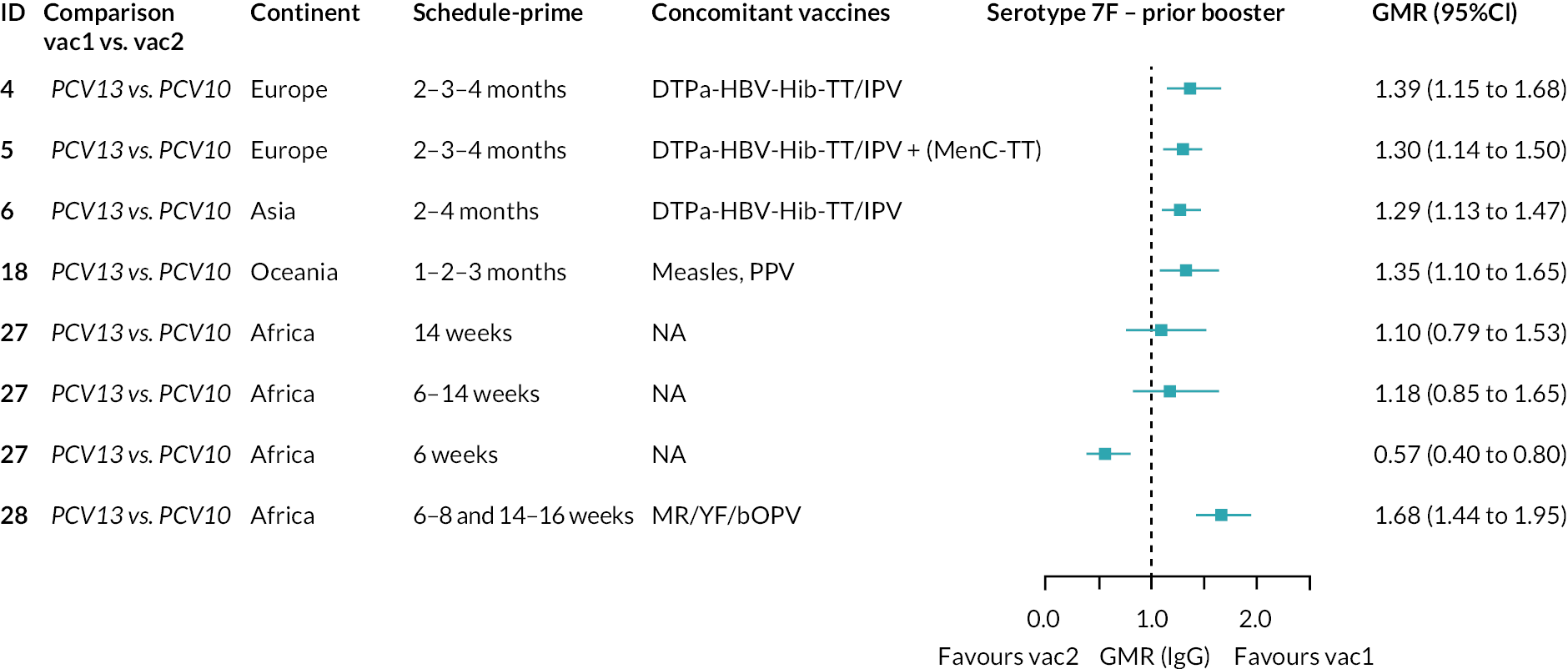
FIGURE 44.
Trial-level GMRs for serotype 3 pre booster. Each solid line in the figure shows the GMR from each trial. Blue boxes and lines show the point estimates and CIs for GMRs comparing vac1 vs. vac2. Concomitant vaccines are vaccines co-administered with PCV primary vaccine series. Information on co-administered vaccine is not always available. Concomitant vaccines in the bracket are those administered in some but not all of the study sites. enr 3–6 m, 4–8 w int, enrolment at 3–6 months of age and at 4–8 weeks interval of three doses primary vaccines in total; N/A, not applicable.

FIGURE 45.
Trial-level GMRs for serotype 6A pre booster. Each solid line in the figure shows the GMR from each trial. Blue boxes and lines show the point estimates and CIs for GMRs comparing vac1 vs. vac2. Concomitant vaccines are vaccines co-administered with PCV primary vaccine series. Information on co-administered vaccine is not always available. Concomitant vaccines in the bracket are those administered in some but not all of the study sites. bOPV, bivalent oral poliovirus vaccine; enr 3–6 m, 4–8 w int, enrolment at 3–6 months of age and at 4–8 weeks interval of three doses primary vaccines in total; MR, measles and rubella combined vaccine; N/A, not applicable; YF, yellow fever vaccine.

FIGURE 46.
Trial-level GMRs for serotype 19A pre booster. Each solid line in the figure shows the GMR from each trial. Blue boxes and lines show the point estimates and CIs for GMRs comparing vac1 vs. vac2. Concomitant vaccines are vaccines co-administered with PCV primary vaccine series. Information on co-administered vaccine is not always available. Concomitant vaccines in the bracket are those administered in some but not all of the study sites. bOPV, bivalent oral poliovirus vaccine; enr 3–6 m, 4–8 w int, enrolment at 3–6 months of age and at 4–8 weeks interval of three doses primary vaccines in total; MR, measles and rubella combined vaccine; N/A, not applicable; YF, yellow fever vaccine.

FIGURE 47.
Trial-level GMRs for serotype 4 post booster. Each solid line in the figure shows the GMR from each trial. Blue boxes and lines show the point estimates and CIs for GMRs comparing vac1 vs. vac2. Concomitant vaccines are vaccines co-administered with PCV primary vaccine series. Information on co-administered vaccine is not always available. Concomitant vaccines in the bracket are those administered in some but not all of the study sites. enr 3–6 m, 4–8 w int, enrolment at 3–6 months of age and at 4–8 weeks interval of three doses primary vaccines in total; N/A, not applicable.

FIGURE 48.
Trial-level GMRs for serotype 6B post booster. Each solid line in the figure shows the GMR from each trial. Blue boxes and lines show the point estimates and CIs for GMRs comparing vac1 vs. vac2. Concomitant vaccines are vaccines co-administered with PCV primary vaccine series. Information on co-administered vaccine is not always available. Concomitant vaccines in the bracket are those administered in some but not all of the study sites. bOPV, bivalent oral poliovirus vaccine; enr 3–6 m, 4–8 w int, enrolment at 3–6 months of age and at 4–8 weeks interval of three doses primary vaccines in total; MR, measles and rubella combined vaccine; N/A, not applicable; YF, yellow fever vaccine.
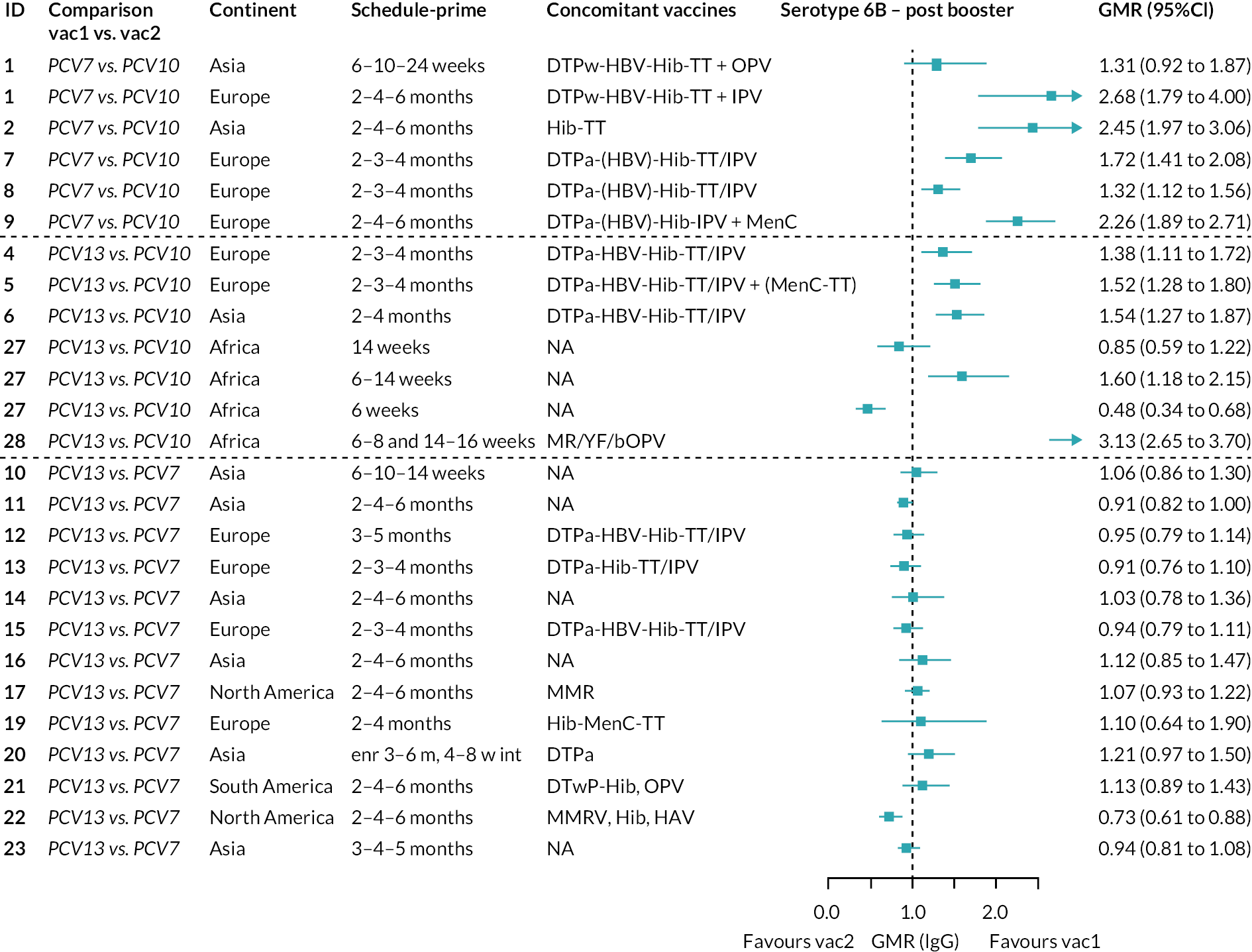
FIGURE 49.
Trial-level GMRs for serotype 9V post booster. Each solid line in the figure shows the GMR from each trial. Blue boxes and lines show the point estimates and CIs for GMRs comparing vac1 vs. vac2. Concomitant vaccines are vaccines co-administered with PCV primary vaccine series. Information on co-administered vaccine is not always available. Concomitant vaccines in the bracket are those administered in some but not all of the study sites. bOPV, bivalent oral poliovirus vaccine; enr 3–6 m, 4–8 w int, enrolment at 3–6 months of age and at 4–8 weeks interval of three doses primary vaccines in total; MR, measles and rubella combined vaccine; N/A, not applicable; YF, yellow fever vaccine.

FIGURE 50.
Trial-level GMRs for serotype 14 post booster. Each solid line in the figure shows the GMR from each trial. Blue boxes and lines show the point estimates and CIs for GMRs comparing vac1 vs. vac2. Concomitant vaccines are vaccines co-administered with PCV primary vaccine series. Information on co-administered vaccine is not always available. Concomitant vaccines in the bracket are those administered in some but not all of the study sites. bOPV, bivalent oral poliovirus vaccine; enr 3–6 m, 4–8 w int, enrolment at 3–6 months of age and at 4–8 weeks interval of three doses primary vaccines in total; MR, measles and rubella combined vaccine; N/A, not applicable; YF, yellow fever vaccine.

FIGURE 51.
Trial-level GMRs for serotype 18C post booster. Each solid line in the figure shows the GMR from each trial. Blue boxes and lines show the point estimates and CIs for GMRs comparing vac1 vs. vac2. Concomitant vaccines are vaccines co-administered with PCV primary vaccine series. Information on co-administered vaccine is not always available. Concomitant vaccines in the bracket are those administered in some but not all of the study sites. enr 3–6 m, 4–8 w int, enrolment at 3–6 months of age and at 4–8 weeks interval of three doses primary vaccines in total; N/A, not applicable.

FIGURE 52.
Trial-level GMRs for serotype 19F post booster. Each solid line in the figure shows the GMR from each trial. Blue boxes and lines show the point estimates and CIs for GMRs comparing vac1 vs. vac2. Concomitant vaccines are vaccines co-administered with PCV primary vaccine series. Information on co-administered vaccine is not always available. Concomitant vaccines in the bracket are those administered in some but not all of the study sites. bOPV, bivalent oral poliovirus vaccine; enr 3–6 m, 4–8 w int, enrolment at 3–6 months of age and at 4–8 weeks interval of three doses primary vaccines in total; MR, measles and rubella combined vaccine; N/A, not applicable; YF, yellow fever vaccine.
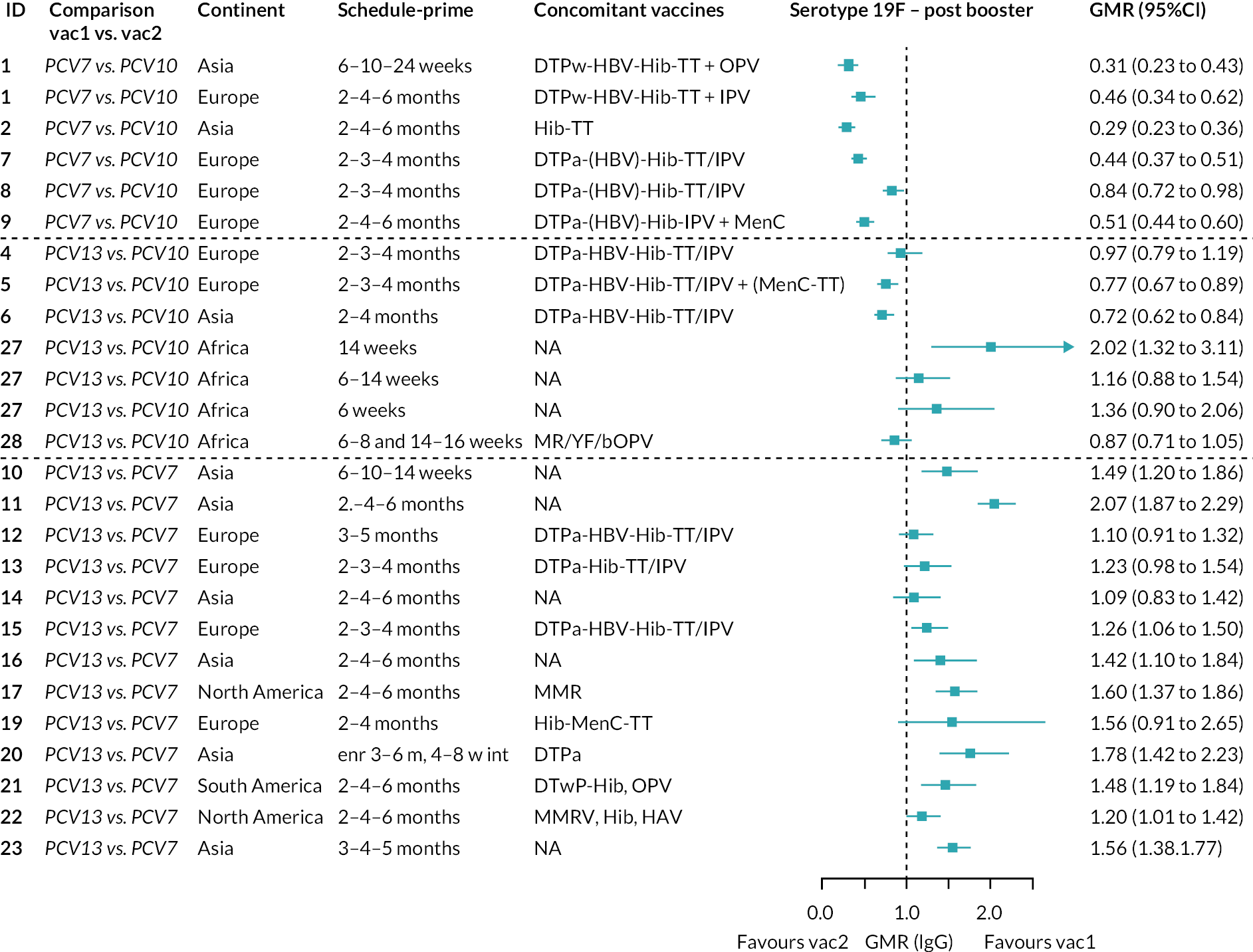
FIGURE 53.
Trial-level GMRs for serotype 23F post booster. Each solid line in the figure shows the GMR from each trial. Blue boxes and lines show the point estimates and CIs for GMRs comparing vac1 vs. vac2. Concomitant vaccines are vaccines co-administered with PCV primary vaccine series. Information on co-administered vaccine is not always available. Concomitant vaccines in the bracket are those administered in some but not all of the study sites. bOPV, bivalent oral poliovirus vaccine; enr 3–6 m, 4–8 w int, enrolment at 3–6 months of age and at 4–8 weeks interval of three doses primary vaccines in total; MR, measles and rubella combined vaccine; N/A, not applicable; YF, yellow fever vaccine.

FIGURE 54.
Trial-level GMRs for serotype 1 post booster. Each solid line in the figure shows the GMR from each trial. Blue boxes and lines show the point estimates and CIs for GMRs comparing vac1 vs. vac2. Concomitant vaccines are vaccines co-administered with PCV primary vaccine series. Information on co-administered vaccine is not always available. Concomitant vaccines in the bracket are those administered in some but not all of the study sites. bOPV, bivalent oral poliovirus vaccine; enr 3–6 m, 4–8 w int, enrolment at 3–6 months of age and at 4–8 weeks interval of three doses primary vaccines in total; MR, measles and rubella combined vaccine; N/A, not applicable; YF, yellow fever vaccine.

FIGURE 55.
Trial-level GMRs for serotype 5 post booster. Each solid line in the figure shows the GMR from each trial. Blue boxes and lines show the point estimates and CIs for GMRs comparing vac1 vs. vac2. Concomitant vaccines are vaccines co-administered with PCV primary vaccine series. Information on co-administered vaccine is not always available. Concomitant vaccines in the bracket are those administered in some but not all of the study sites. bOPV, bivalent oral poliovirus vaccine; enr 3–6 m, 4–8 w int, enrolment at 3–6 months of age and at 4–8 weeks interval of three doses primary vaccines in total; MR, measles and rubella combined vaccine; N/A, not applicable; YF, yellow fever vaccine.
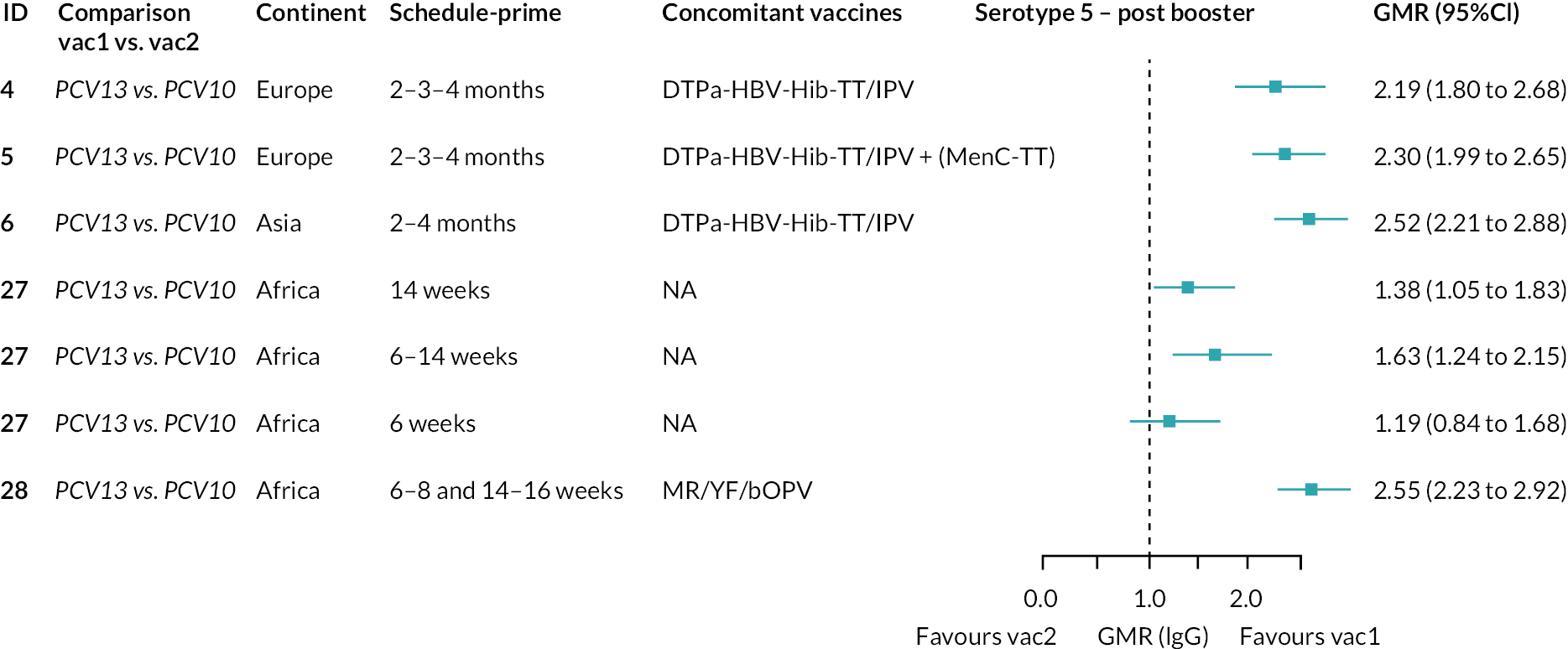
FIGURE 56.
Trial-level GMRs for serotype 7F post booster. Each solid line in the figure shows the GMR from each trial. Blue boxes and lines show the point estimates and CIs for GMRs comparing vac1 vs. vac2. Concomitant vaccines are vaccines co-administered with PCV primary vaccine series. Information on co-administered vaccine is not always available. Concomitant vaccines in the bracket are those administered in some but not all of the study sites. bOPV, bivalent oral poliovirus vaccine; enr 3–6 m, 4–8 w int, enrolment at 3–6 months of age and at 4–8 weeks interval of three doses primary vaccines in total; MR, measles and rubella combined vaccine; N/A, not applicable; YF, yellow fever vaccine.

FIGURE 57.
Trial-level GMRs for serotype 3 post booster. Each solid line in the figure shows the GMR from each trial. Blue boxes and lines show the point estimates and CIs for GMRs comparing vac1 vs. vac2. Concomitant vaccines are vaccines co-administered with PCV primary vaccine series. Information on co-administered vaccine is not always available. Concomitant vaccines in the bracket are those administered in some but not all of the study sites. enr 3–6 m, 4–8 w int, enrolment at 3–6 months of age and at 4–8 weeks interval of three doses primary vaccines in total; N/A, not applicable.
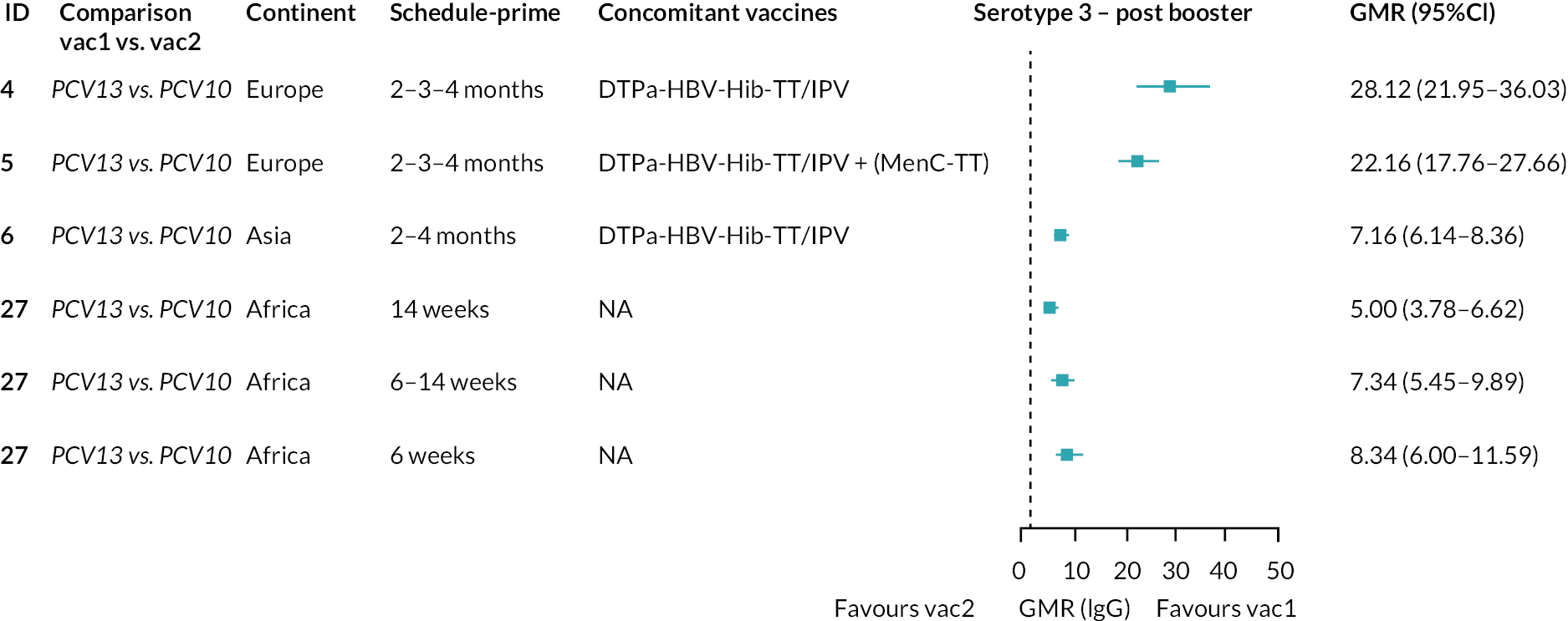
FIGURE 58.
Trial-level GMRs for serotype 6A post booster. Each solid line in the figure shows the GMR from each trial. Blue boxes and lines show the point estimates and CIs for GMRs comparing vac1 vs. vac2. Concomitant vaccines are vaccines co-administered with PCV primary vaccine series. Information on co-administered vaccine is not always available. Concomitant vaccines in the bracket are those administered in some but not all of the study sites. bOPV, bivalent oral poliovirus vaccine; enr 3–6 m, 4–8 w int, enrolment at 3–6 months of age and at 4–8 weeks interval of three doses primary vaccines in total; MR, measles and rubella combined vaccine; N/A, not applicable; YF, yellow fever vaccine.

FIGURE 59.
Trial-level GMRs for serotype 19A post booster. Each solid line in the figure shows the GMR from each trial. Blue boxes and lines show the point estimates and CIs for GMRs comparing vac1 vs. vac2. Concomitant vaccines are vaccines co-administered with PCV primary vaccine series. Information on co-administered vaccine is not always available. Concomitant vaccines in the bracket are those administered in some but not all of the study sites. bOPV, bivalent oral poliovirus vaccine; enr 3–6 m, 4–8 w int, enrolment at 3–6 months of age and at 4–8 weeks interval of three doses primary vaccines in total; MR, measles and rubella combined vaccine; N/A, not applicable; YF, yellow fever vaccine.

FIGURE 60.
Trial-level RR for serotype 4. Each solid line in the figure shows the RR from each trial. Blue boxes and lines show the point estimates and CIs for RRs comparing vac1 vs. vac2. Co-adm vaccines are vaccines co-administered with PCV primary vaccine series. Information on co-administered vaccine is not always available. Concomitant vaccines in the bracket are those administered in some but not all of the study sites.

FIGURE 61.
Trial-level RR for serotype 6B. TT, tetanus toxoid conjugate; NA, not applicable. Each solid line in the figure shows the RR from each trial. Blue boxes and lines show the point estimates and CIs for RRs comparing vac1 vs. vac2. Co-adm vaccines are vaccines co-administered with PCV primary vaccine series. Information on co-administered vaccine is not always available. Concomitant vaccines in the bracket are those administered in some but not all of the study sites.

FIGURE 62.
Trial-level RR for serotype 9V. Each solid line in the figure shows the RR from each trial. Blue boxes and lines show the point estimates and CIs for RRs comparing vac1 vs. vac2. Co-adm vaccines are vaccines co-administered with PCV primary vaccine series. Information on co-administered vaccine is not always available. Concomitant vaccines in the bracket are those administered in some but not all of the study sites.
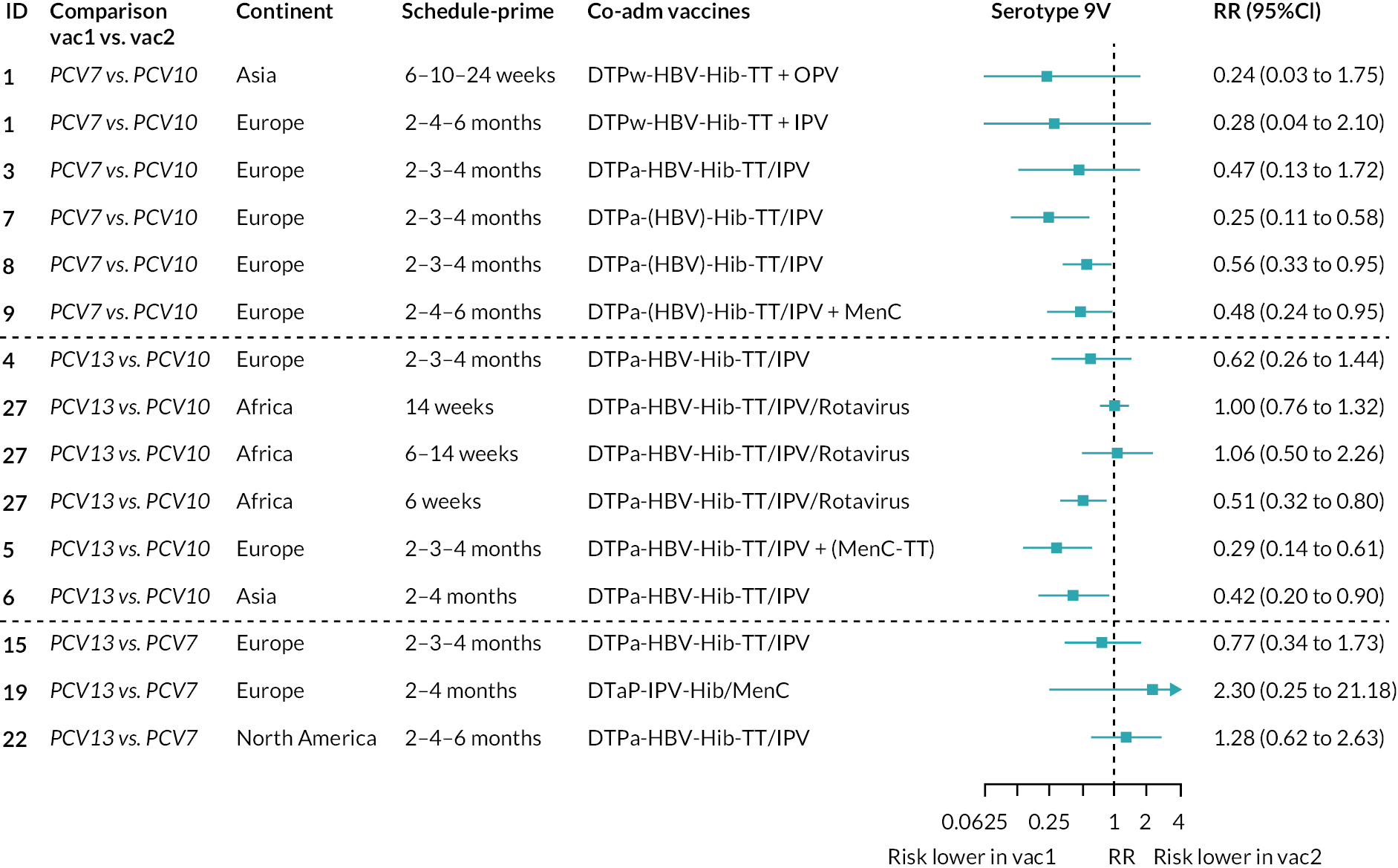
FIGURE 63.
Trial-level RR for serotype 14. Each solid line in the figure shows the RR from each trial. Blue boxes and lines show the point estimates and CIs for RRs comparing vac1 vs. vac2. Co-adm vaccines are vaccines co-administered with PCV primary vaccine series. Information on co-administered vaccine is not always available. Concomitant vaccines in the bracket are those administered in some but not all of the study sites.
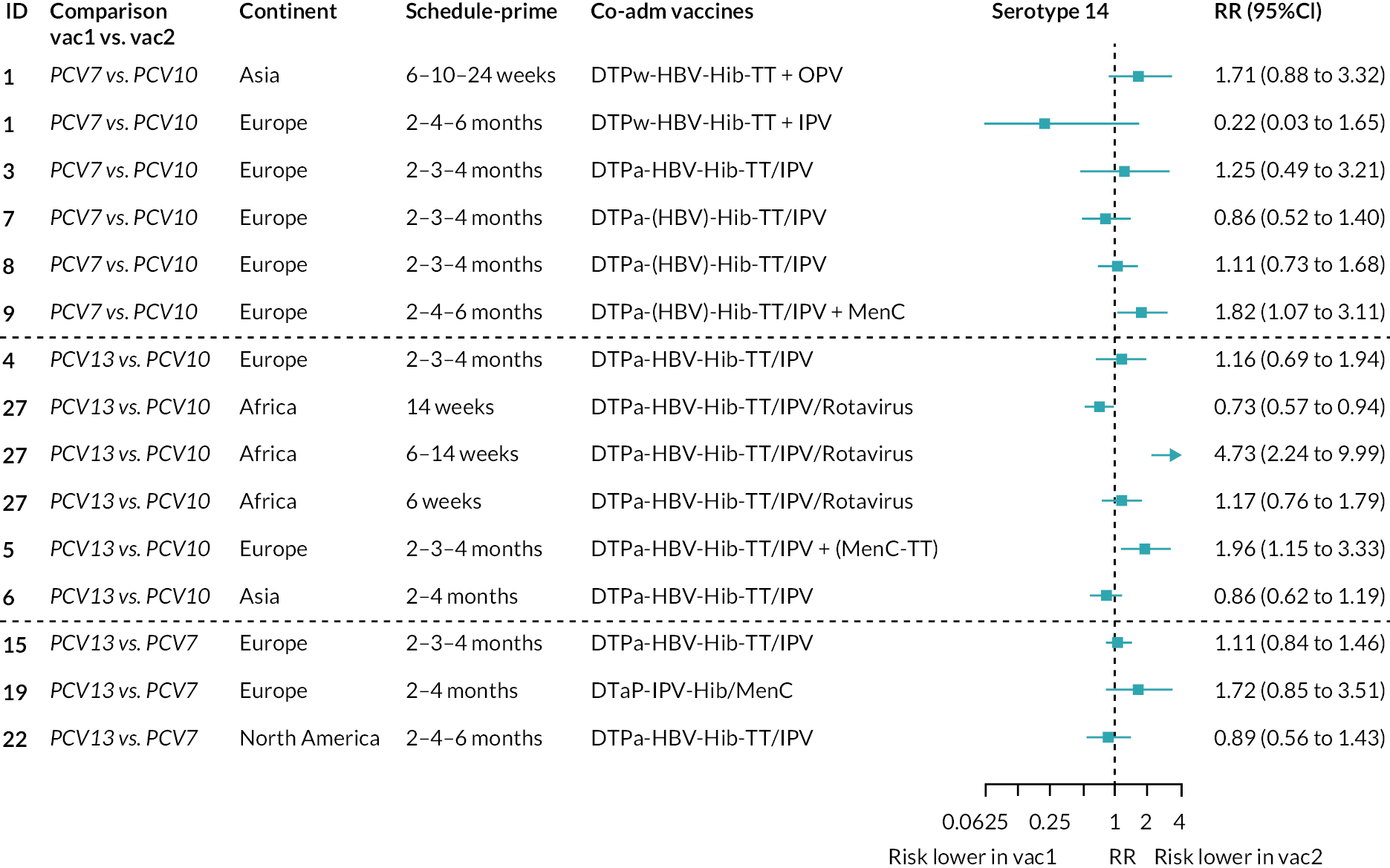
FIGURE 64.
Trial-level RR for serotype 18C. Each solid line in the figure shows the RR from each trial. Blue boxes and lines show the point estimates and CIs for RRs comparing vac1 vs. vac2. Co-adm vaccines are vaccines co-administered with PCV primary vaccine series. Information on co-administered vaccine is not always available. Concomitant vaccines in the bracket are those administered in some but not all of the study sites.

FIGURE 65.
Trial-level RR for serotype 4. Each solid line in the figure shows the RR from each trial. Blue boxes and lines show the point estimates and CIs for RRs comparing vac1 vs. vac2. Co-adm vaccines are vaccines co-administered with PCV primary vaccine series. Information on co-administered vaccine is not always available. Concomitant vaccines in the bracket are those administered in some but not all of the study sites.

FIGURE 66.
Trial-level RR for serotype 23F. Each solid line in the figure shows the RR from each trial. Blue boxes and lines show the point estimates and CIs for RRs comparing vac1 vs. vac2. Co-adm vaccines are vaccines co-administered with PCV primary vaccine series. Information on co-administered vaccine is not always available. Concomitant vaccines in the bracket are those administered in some but not all of the study sites.

FIGURE 67.
Trial-level RR for serotype 1. Each solid line in the figure shows the RR from each trial. Blue boxes and lines show the point estimates and CIs for RRs comparing vac1 vs. vac2. Co-adm vaccines are vaccines co-administered with PCV primary vaccine series. Information on co-administered vaccine is not always available. Concomitant vaccines in the bracket are those administered in some but not all of the study sites.

FIGURE 68.
Trial-level RR for serotype 5. Each solid line in the figure shows the RR from each trial. Blue boxes and lines show the point estimates and CIs for RRs comparing vac1 vs. vac2. Co-adm vaccines are vaccines co-administered with PCV primary vaccine series. Information on co-administered vaccine is not always available. Concomitant vaccines in the bracket are those administered in some but not all of the study sites.
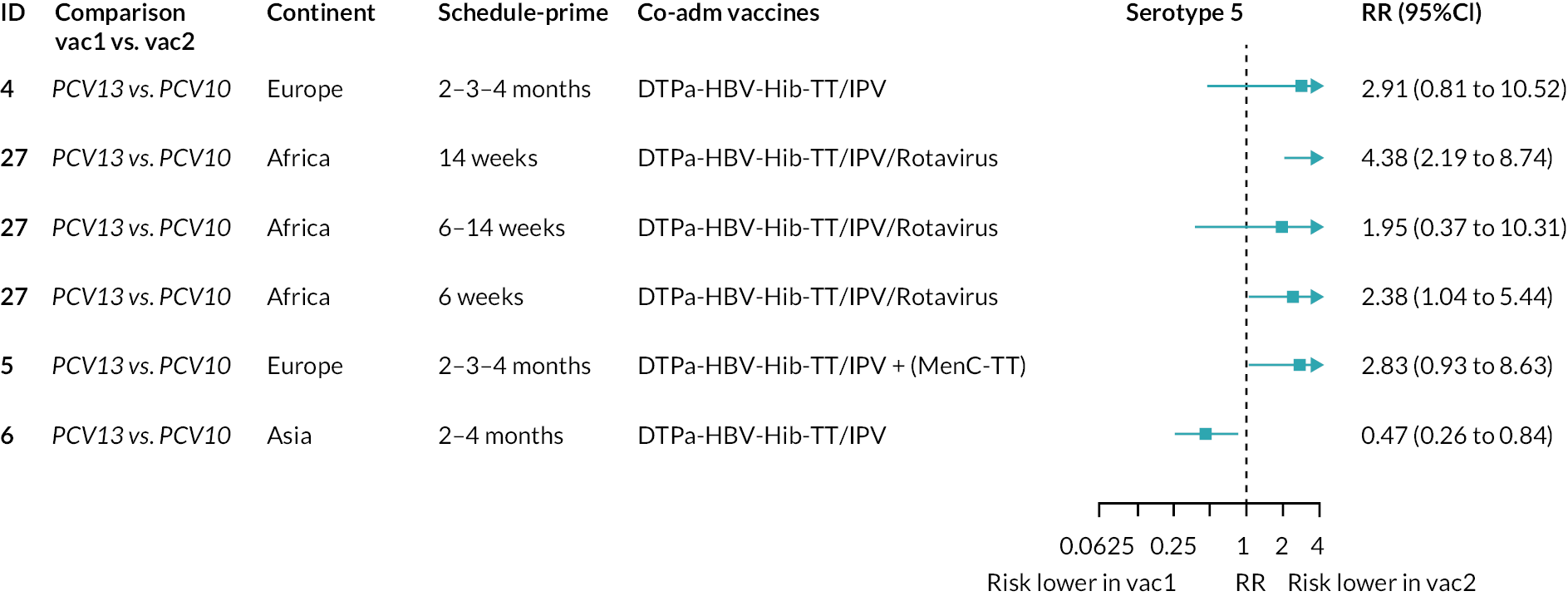
FIGURE 69.
Trial-level RR for serotype 7F. Each solid line in the figure shows the RR from each trial. Blue boxes and lines show the point estimates and CIs for RRs comparing vac1 vs. vac2. Co-adm vaccines are vaccines co-administered with PCV primary vaccine series. Information on co-administered vaccine is not always available. Concomitant vaccines in the bracket are those administered in some but not all of the study sites.
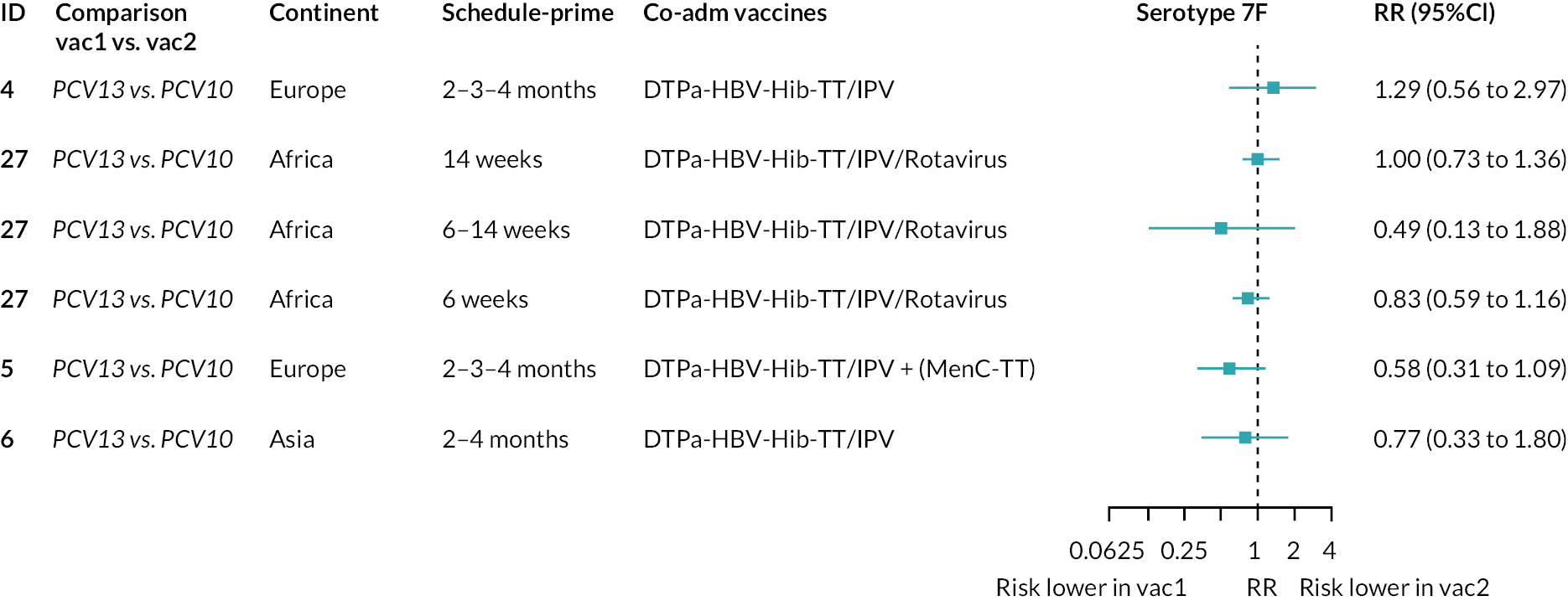
Appendix 6 Sensitivity analyses
FIGURE 70.
Geometric mean ratios from sensitivity analysis restricted to studies providing data for all three time points: (a) post-primary vaccination series, (b) pre boost and (c) post boost. Each line in the figure shows the output from NMAs (PCV7 serotypes) or direct meta-analyses (PCV13 but non-PCV7 serotypes). Blue boxes and blue lines show the point estimates and CIs for GMRs comparing PCV13 vs. PCV10. Points to the right of the vertical line are those with higher antibody responses in the PCV13 arm of the study, and points to the left are those with higher antibody responses in the PCV10 arm. The direct evidence column shows the percentage of evidence from studies directly comparing PCV13 vs. PCV10 that contributes to the estimates presented in the figure in blue (PCV13 vs. PCV10). GMR of PCV13 vs. PCV10 for PCV10 and PCV13 serotypes are from a meta-analysis of only studies comparing PCV13 with PCV10. SA, sensitivity analysis.
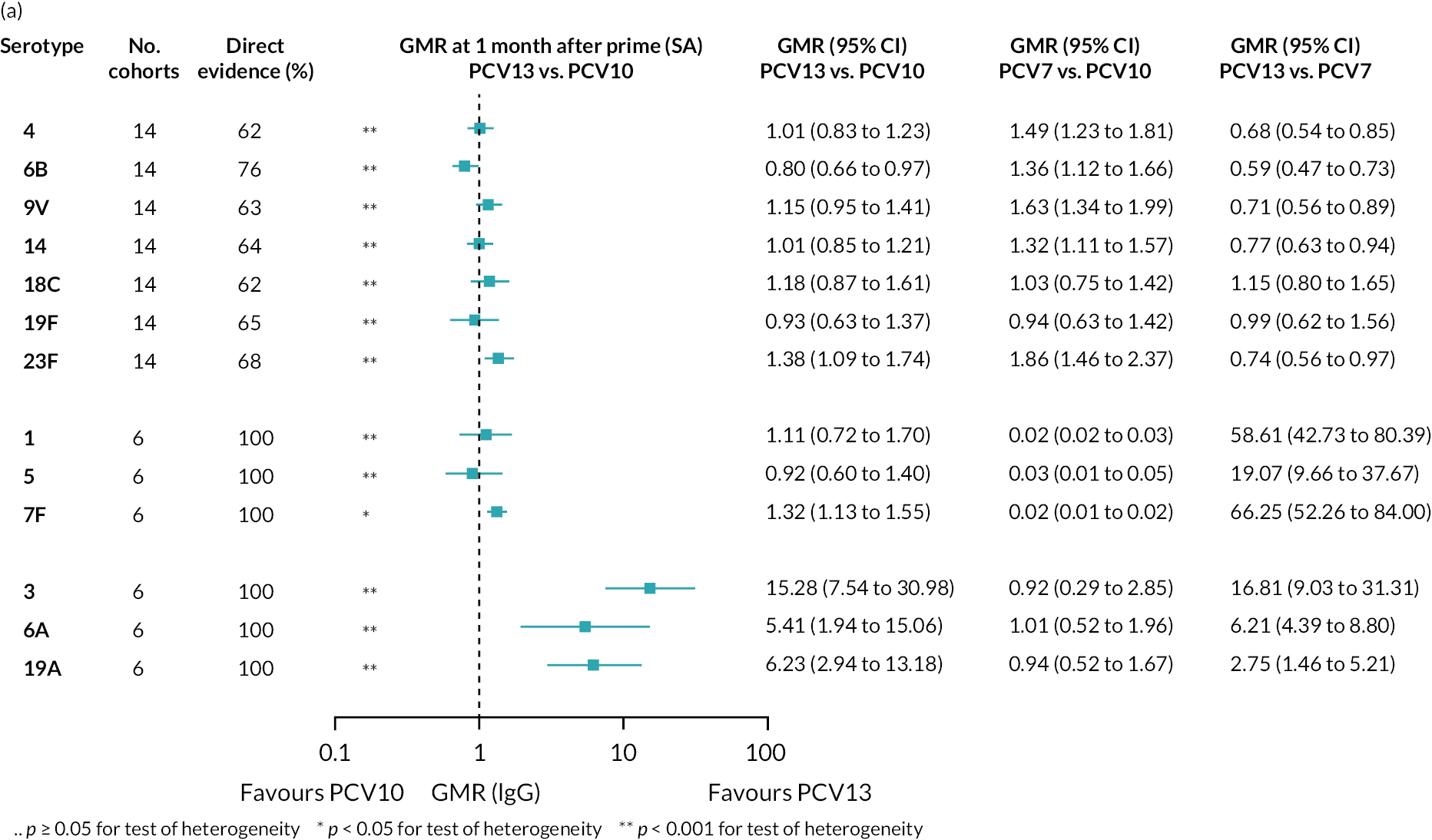
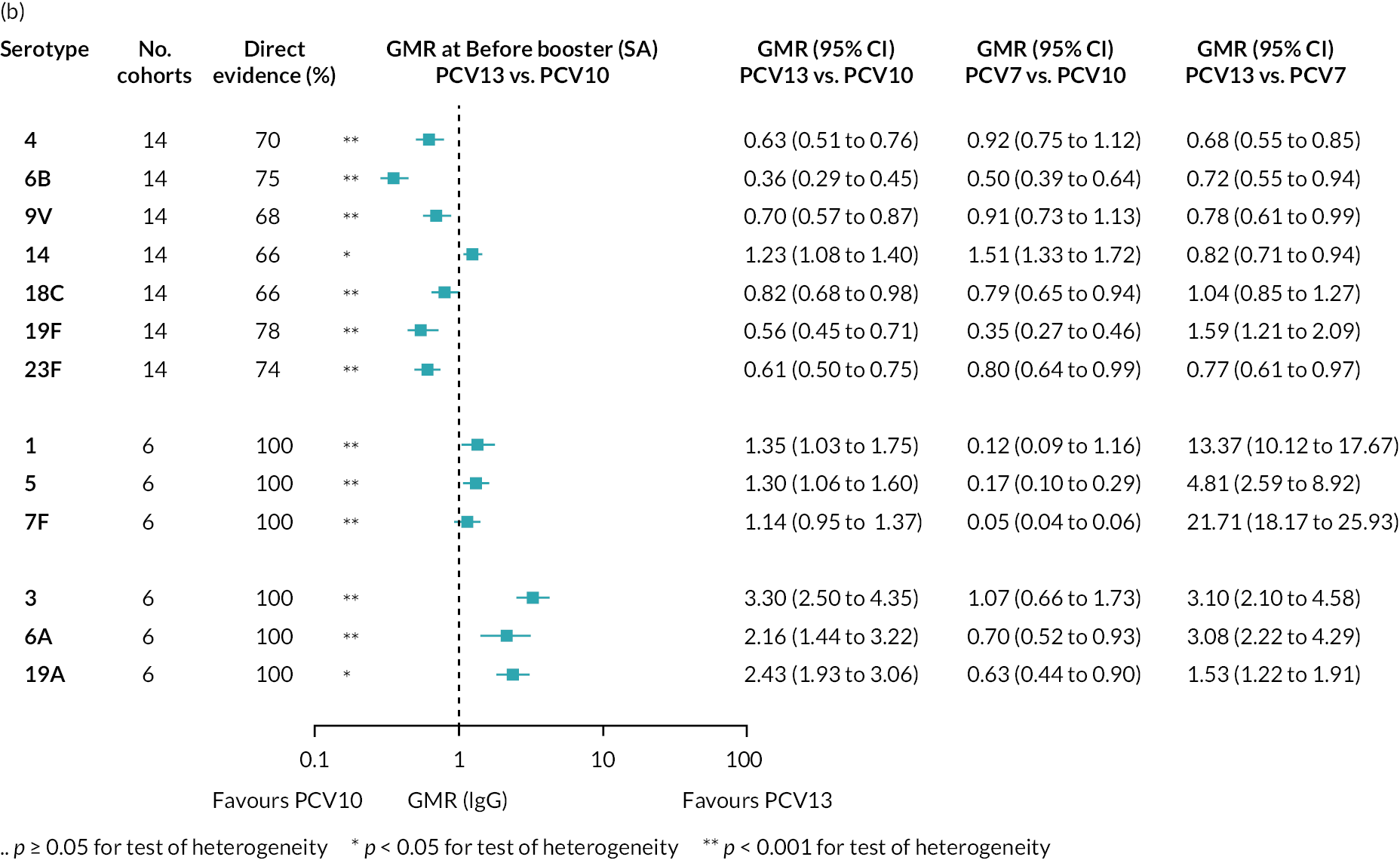

FIGURE 71.
Geometric mean ratios from sensitivity analyses restricted to studies conducted in Europe at (a) post-primary vaccination series, (b) pre boost and (c) post boost. Each line in the figure shows the output from NMSs (PCV7 serotypes) or direct meta-analyses (PCV13 but non-PCV7 serotypes). Blue boxes and blue lines show the point estimates and CIs for GMRs comparing PCV13 vs. PCV10. Points to the right of the vertical line are those with higher antibody responses in the PCV13 arm of the study, and points to the left are those with higher antibody responses in the PCV10 arm. The direct evidence column shows the percentage of evidence from studies directly comparing PCV13 vs. PCV10 that contributes to the estimates presented in the figure in blue (PCV13 vs. PCV10). GMR of PCV13 vs. PCV10 for PCV10 and PCV13 serotypes are from a meta-analysis of only studies comparing PCV13 with PCV10.
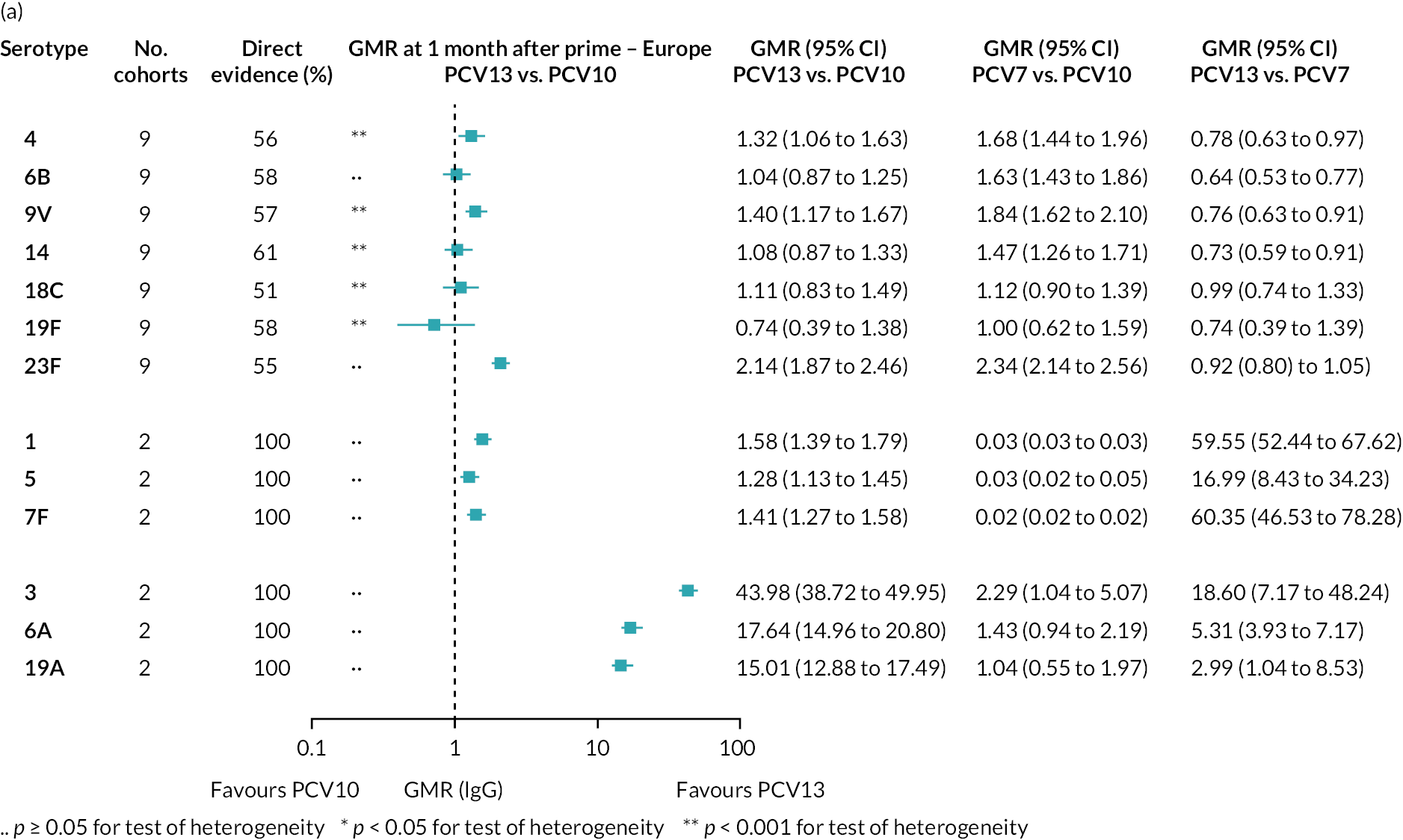
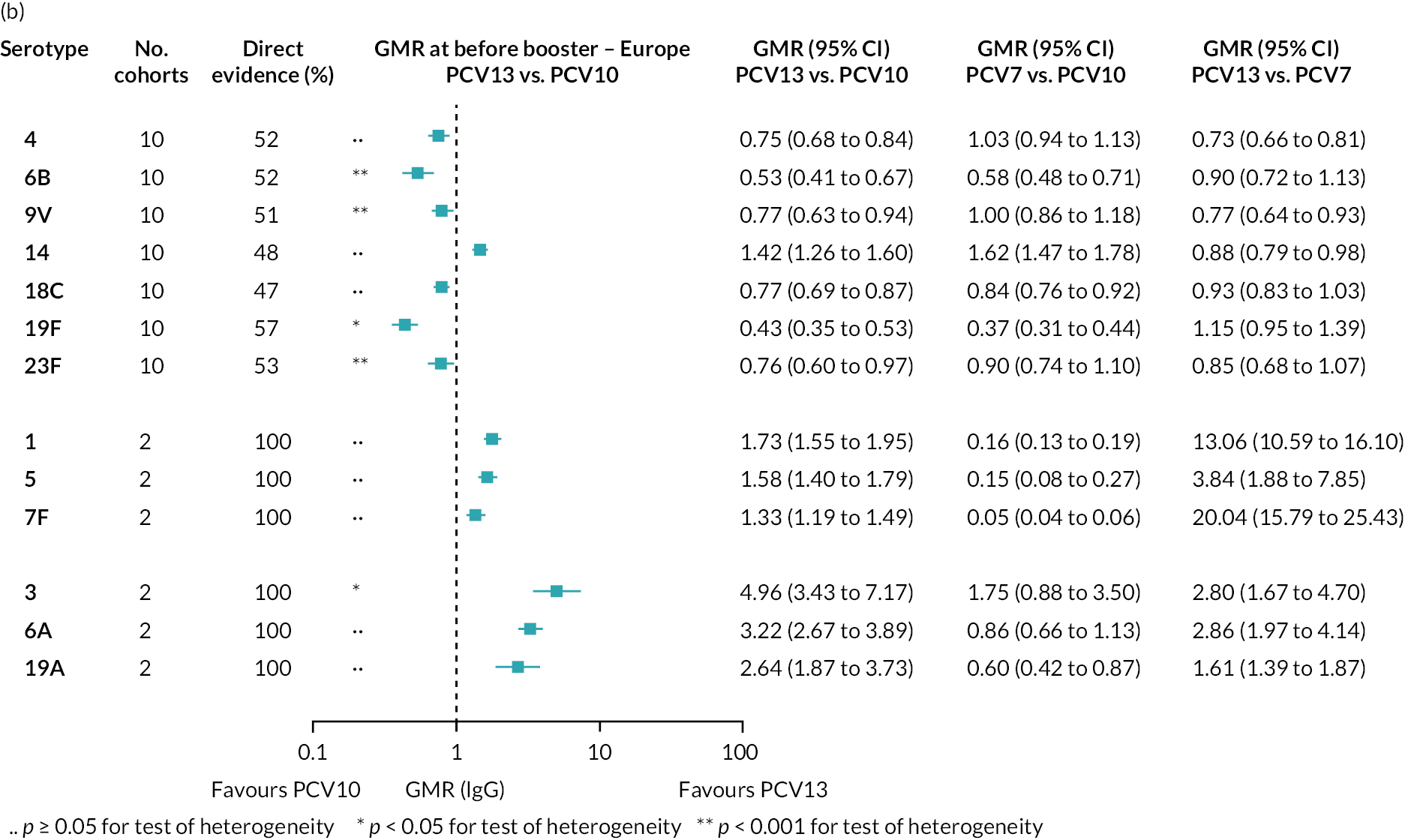

FIGURE 72.
Geometric mean ratios from sensitivity analyses of studies conducted in Asia at (a) post-primary vaccination series and (b) post boost. Each line in the figure shows the output from NMAs (PCV7 serotypes) or direct meta-analyses (PCV13 but non-PCV7 serotypes). Blue boxes and blue lines show the point estimates and CIs for GMRs comparing PCV13 vs. PCV10. Points to the right of the vertical line are those with higher antibody responses in the PCV13 arm of the study, and points to the left are those with higher antibody responses in the PCV10 arm. The direct evidence column shows the percentage of evidence from studies directly comparing PCV13 vs. PCV10 that contributes to the estimates presented in the figure in blue (PCV13 vs. PCV10). GMR of PCV13 vs. PCV10 for PCV10 and PCV13 serotypes are from a meta-analysis of only studies comparing PCV13 with PCV10.


FIGURE 73.
Geometric mean ratios from sensitivity analyses of studies that used a 3 + 1 schedule at (a) post-primary vaccination series, (b) pre boost and (c) post boost. Each line in the figure shows the output from NMAs (PCV7 serotypes) or direct meta-analyses (PCV13 but non-PCV7 serotypes). Blue boxes and blue lines show the point estimates and CIs for GMRs comparing PCV13 vs. PCV10. Points to the right of the vertical line are those with higher antibody responses in the PCV13 arm of the study, and points to the left are those with higher antibody responses in the PCV10 arm. The direct evidence column shows the percentage of evidence from studies directly comparing PCV13 vs. PCV10 that contributes to the estimates presented in the figure in blue (PCV13 vs. PCV10). GMR of PCV13 vs. PCV10 for PCV10 and PCV13 serotypes are from a meta-analysis of only studies comparing PCV13 with PCV10.

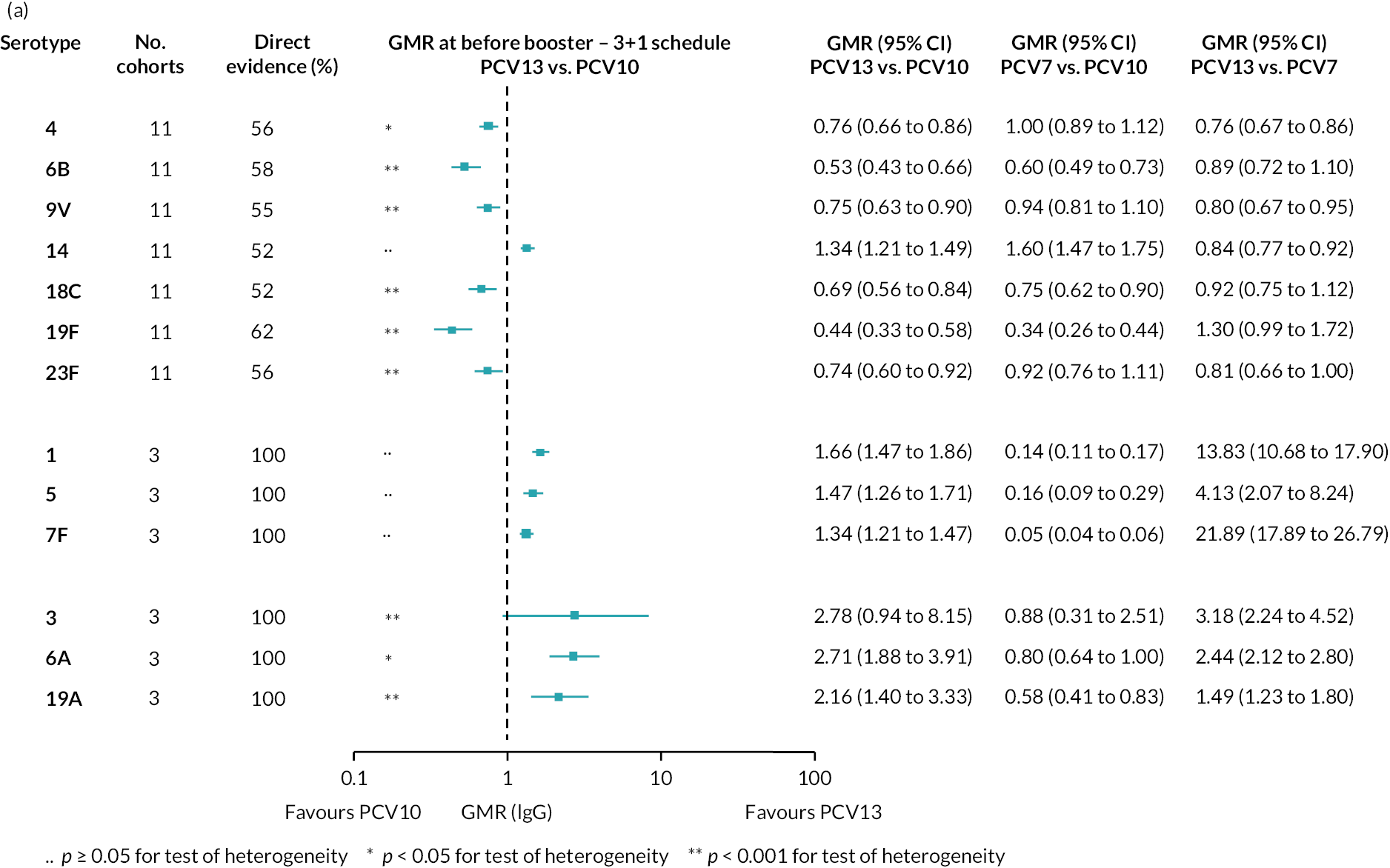

FIGURE 74.
Sensitivity analysis of RR of seroinfection for studies conducted in Europe. Each line in the figure shows the output from NMAs (PCV7 serotypes) or direct meta-analyses (PCV10 serotypes). Blue boxes and blue lines show the point estimates and CIs of RR of seroinfection comparing PCV13 vs. PCV10. The direct evidence column shows the percentage of evidence from studies directly comparing PCV13 vs. PCV10. Results for PCV10 serotypes are from a meta-analysis of only studies comparing PCV13 with PCV10; therefore, estimates of PCV7 vs. PCV10 and PCV13 vs. PCV7 were not available.

FIGURE 75.
Sensitivity analysis of RR of seroinfection for studies using a 3 + 1 schedule. Each line in the figure shows the output from NMAs (PCV7 serotypes) or direct meta-analyses (PCV10 serotypes). Blue boxes and blue lines show the point estimates and CIs of RR of seroinfection comparing PCV13 vs. PCV10. The direct evidence column shows the percentage of evidence from studies directly comparing PCV13 vs. PCV10. Results for PCV10 serotypes are from a meta-analysis of only studies comparing PCV13 with PCV10; therefore, estimates of PCV7 vs. PCV10 and PCV13 vs. PCV7 were not available.
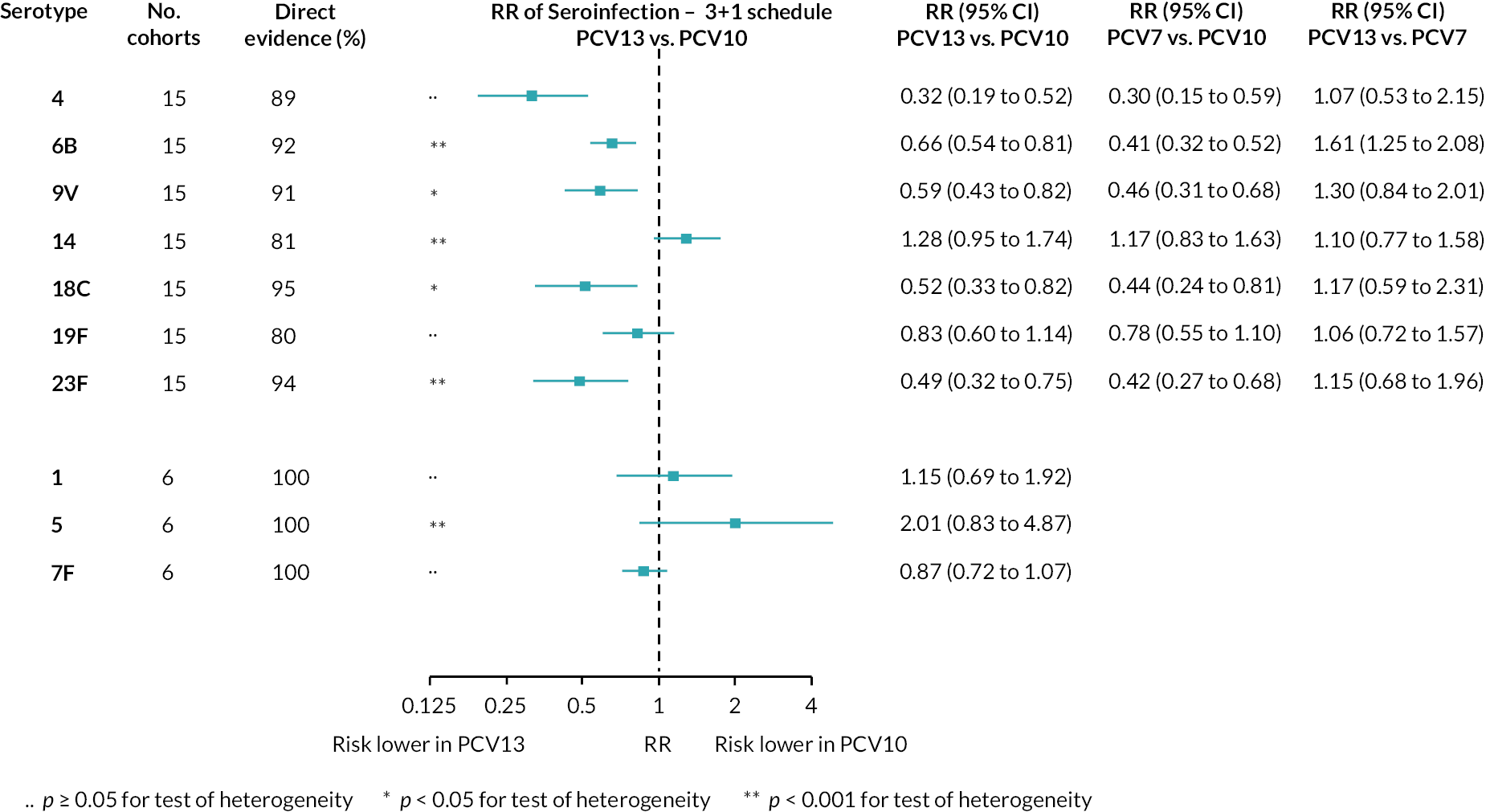
Appendix 7 R syntax
-
# network meta-analysis
-
library(netmeta)
-
net.pcv <- netmeta(TE = lgGMR, seTE = lgGMR.se, treat1 = vac2, treat2 = vac1, studlab = cohort.ID, data = data, sm = ‘MD’, reference.group = ‘pcv10’)
-
#Split direct and indirect evidence to evaluate inconsistency
-
net.pcv.split <- netsplit(net.pcv)
-
#format output
-
ind <- net.pcv.split$random
-
ind <- ind[which(ind$comparison %in% c(‘pcv10:pcv13’, ‘pcv13:pcv10’)),]
-
output.nma$random.nma <- round(ind$TE, 3)
-
output.nma$random.lower <- round(ind$lower, 3)
-
output.nma$random.upper <- round(ind$upper, 3)
-
output.nma$random.se <- round(ind$seTE, 3)
-
output.nma$random.CI <- paste(sprintf(‘%0.3f’, ind$TE), ‘ (‘, sprintf(‘%0.3f’, ind$lower), ‘, ‘, sprintf(‘%0.3f’, ind$upper), ‘)’, sep = ‘‘)
-
# Association between immunogenicity and seroefficacy
-
library(lme4)
-
#fit linear mixed effects model
-
random <- lmer(logRR ~ lgGMR * sero.type + (1 | cohort.ID), data = data, weights = study.weights)
List of abbreviations
- AIC
- Akaike information criterion
- CCR
- case : carrier ratio
- CDSR
- Cochrane Database of Systematic Reviews
- CET
- cost-effectiveness threshold
- CENTRAL
- Cochrane Central Register of Controlled Trials
- CRM
- cross-reacting material
- DTaP
- diphtheria, tetanus, acellular pertussis
- DTwP
- diphtheria, tetanus, whole-cell pertussis
- ELISA
- enzyme-linked immunosorbent assay
- GMC
- geometric mean concentration
- GMR
- geometric mean ratio
- HBV
- hepatitis B vaccine
- Hib-TT
- Haemophilus influenzae type b tetanus toxoid conjugate vaccine
- ICTRP
- International Clinical Trials Registry Platform
- IgG
- immunoglobulin G
- iNMB
- incremental net monetary benefit
- IPV
- inactivated polio vaccine
- mcg/ml
- micrograms per millilitre
- MenC
- group C meningococcal vaccine
- NCT
- National Clinical Trial
- NMA
- network meta-analysis
- OPV
- oral polio vaccine
- PCV
- pneumococcal conjugate vaccine
- PCV10-SII
- Pneumosil
- QALY
- quality-adjusted life-year
- RCT
- randomised controlled trial
- RoB
- risk of bias
- RR
- relative risk
- SE
- standard error
- TT
- tetanus toxoid
- WHO
- World Health Organization